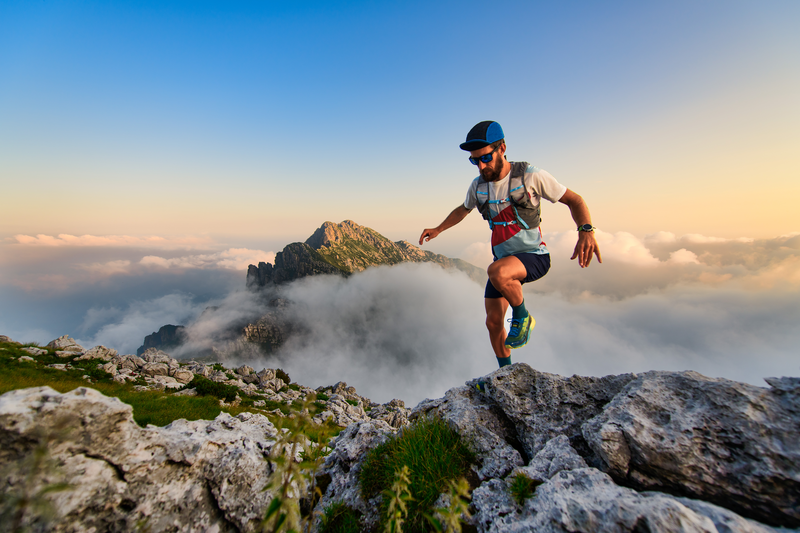
94% of researchers rate our articles as excellent or good
Learn more about the work of our research integrity team to safeguard the quality of each article we publish.
Find out more
ORIGINAL RESEARCH article
Front. Vet. Sci. , 12 May 2023
Sec. Veterinary Epidemiology and Economics
Volume 10 - 2023 | https://doi.org/10.3389/fvets.2023.1149876
Introduction: Honey bee gut microbiota have an important role in host health, nutrition, host-symbiont interaction, and interaction behavior with the surrounding environment. Recent discoveries of strain-level variation, characteristics of protective and nutritional capabilities, and reports of eco-physiological significance to the microbial community have emphasized the importance of honey bee gut microbiota. Many regions of Asia and Africa are inhabited by the dwarf honey bee, Apis florea. Studying its microflora and potential for pollination is therefore of foremost importance.
Methods: In the present investigation, we aimed to explore the gut bacteriobiome composition of two distinct honey bee species, Apis florea and Apis cerana indica using high throughput sequencing. Functional predictions of bee gut bacterial communities using PICRUSt2 was carried out.
Results and discussion: The phylum Proteobacteria dominated the bacterial community in both A. cerana indica (50.1%) and A. florea (86.7%), followed by Firmicutes (26.29 and 12.81%), Bacteroidetes (23.19 and 0.04%) and Actinobacteria (0.4 and 0.02%) respectively. The gut bacteria of A. cerana indica was more diverse than that of A. florea. The observed variations in bacterial genomic diversity among these critical pollinator species may have been influenced by the apiary management techniques, ecological adaptation factors or habitat size. These variations can have a significant effect in understanding host-symbiont interactions and functioning of gut microbiota highlighting the importance of metagenomic survey in understanding microbial community ecology and evolution. This is the first comparative study on variation in bacterial diversity between two Asian honey bees.
Honey bees are key pollinators of many crops for human use and provide valuable commodities such as honey and wax, making the gut microbiota of honey bees an interesting community to explore (1). Gut bacteria accomplish a wide range of functions that are vital to the host’s metabolism and overall health. Many living organisms benefit from gut microbial populations, from digesting indigestible polysaccharides to immunomodulation (1). Honey bees and their bumble bee relatives are globally important as they pollinate a vast majority of wild flowering plants and crops in the world. Given these aspects, the gut microbiota of social bees offers a promising new paradigm for studies on genetic diversity and the evolution of host-associated bacterial communities. The rapid decline in the bee populations has set a focus on possible factors that affect their health, especially their microbiota (2–4). There are significant functional differences in the gut bacterial communities of relative host species of honey bees. The gut microbiota of honey bees is becoming a prominent model for investigating genetic variation as well as the features that have led to the evolution of bacterial populations that are associated with hosts.
The microbiota is most common in the distal gastrointestinal system, where it assists in the breakdown and degradation of plant cell wall components (5). Gut bacteria are primarily transferred through social interactions in both bees and primates (6). Many invertebrate gut communities, on the other hand, have unpredictable compositions dominated by bacteria from environmental sources (7, 8). The reared species of honey bee have occupied a dominant position in commercial pollination around the world, as these are highly social bees. Yet, on the other hand, wild bees are useful pollinators, too. It is an efficient pollinator of numerous crops worldwide. Because of the honey bee’s significance to the agro-ecosystems in its native habitat, its conservation is highly anticipated (9). Due to limited understanding of their behavioral mechanism for nesting, perhaps their contribution toward pollination is underestimated (10). Modern apiculture, however, today suffers from several challenges, including parasitic mites, honey bee diseases, the inability of honey bees to function at low temperatures and adverse weather conditions. The general utility of honey bees as agricultural pollinators is confronted by these problems (10). This adds to the concern of beekeepers, insect-pollinated crop breeders and policy officials over fast growing reductions in honey bee populations (Colony Collapse Disorder) (11). Dwarf honeybees potential role in natural and agricultural systems offers a multidisciplinary perspective on various aspects. Production of lesser honey is the main limitation of A. florea, and hence not much attention has been given. However, the emendable pollination efficiency of this bee has considerably contributed to the varied flora in the tropical region, when considering how pollinator loss affects ecosystem stability and food security worldwide, the role of the dwarf honeybee Apis florea acquires vital significance (12).
The bacterial communities of wild flies are more diverse and vary in comparison to domesticated species (13), involving honey bee gut bacteria (14). Significant differences in microbiota diversity observed in these essential pollinator species could be associated with changes in bee management strategies, habitat size, or differential ecological evolution (15). Given the importance of metagenomic efforts to study the ecology and evolution of microbial populations, such alterations are expected to have an impact on gut flora interactions between hosts and symbionts. Despite advances in understanding of host-associated bacterial community diversity and its functional consequences, little is known about host species diversity variations or the underlying mechanisms that regulate and maintain diversification within or among hosts (15).
Among the few studies that have explained the bacterial community associated with the dwarf honey bee are as follows; an observation on lactic acid bacteria (LAB) in the crop of A. florea, assessment of bacterial communities in A. florea larvae (16, 17), and diversification of gut bacteria of a closely related species, Apis andreniformis (18).
Using targeted 16S rRNA gene amplicon sequencing, bacterial community composition profile of A. cerana indica and A. florea from the Indian subcontinent was studied. The microbial communities of A. florea, a pollinator, were compared with A. cerana indica with emphasis on ecosystem stabilization and commercial purposes, and we aimed to study the significant difference between the gut microbial compositions as well as major differences in functions.
A total of 60 worker honey bees (30 of each species) of A. cerana indica (four bee colonies from an apiary) and from three A. florea colonies were obtained in the Western Ghats of southern India’s forest region (Lat:12.14455 DMS N 12° 8′ 40.38″, Long: 75.9382 DMS E 75° 56′ 17.519″, College of Forestry, Ponnampet, Kodagu, India and Lat:12.55821 DMS N 12° 33′ 29.556″, Long:75.95338 DMS E 75° 57′ 12.167″, Mavina Halla Forest, Kodagu, Karnataka, India respectively). The sampling sites were located far among the bee colonies. The method used for sampling was followed as per the available literature (15, 19). During the same day, live honey bees were transferred in small cages to the laboratory for dissection; all honey bees were cold anaesthetized and were surface-sterilized in sterile falcon tubes, with sodium hypochlorite (7%) and ethanol (70%), then washed four times using sterile phosphate-buffered saline (PBS) (20). The complete alimentary canals of honey bees were dissected under aseptic conditions using saline (0.9%) after the stinger was removed with sterilized forceps. The dissected whole guts were transferred directly to phosphate-buffered saline (PBS) and preserved at −20°C for further analysis.
In two separate tubes, the alimentary tracts of worker honey bees (n = 60, 30 for each species of A. cerana indica and A. florea) were collected in 1 mL PBS. Genomic DNA was extracted using Qiagen Tissue kit (Genomic DNA extraction kit, United States) and the subsequent steps were carried out according to the manufacturer’s instructions. As detailed by (21), the concentration of DNA was determined using a NanoDropND-1000 spectrophotometer (Willington, United States) and the quality was determined using a 0.8% agarose gel. The DNA was kept at −20°C until further processing.
To study the bacterial diversity in honey bee guts, NGS libraries were constructed by targeting V4 region of 16S rRNA gene with primers 515F (5′-GTGCCAGCMGCCGGTAA-3′) and 806R (5′-GGACTACHVGGGTWTCTAAT-3′) (21). The library construction of 16S rRNA gene amplicons was carried out in accordance with Illumina protocols (United States). Purified libraries were subsequently pooled in equimolar concentrations and sequenced on the Illumina MiSeq system using 2 × 250 bp v2 Chemistry.
The raw reads obtained from high-throughput sequence analysis were assembled using the FLASH tool (22). The pre-processing of raw Fastq files were carried out using MOTHUR v1.32 to remove adaptors, low quality and ambiguous reads which minimizes the occurrence of erroneous sequences to the dataset. The reference-based OTU picking method was used to select operational taxonomic units (OTUs) against SILVA (v132) (23). QIIME v1.8 was used to calculate α-biodiversity indices such as Chao1, ACE and Shannon (diversity), once the sequencing depth has been set for all samples (24). Furthermore, PICRUSt2 tool was used to perform imputed metagenomics for the functional analysis of bacterial communities (25). The obtained dataset was analyzed using the KEGG (Kyoto Encyclopedia of Genes and Genomes) database (26).
High-throughput sequencing and the quality editing of the 16S rRNA gene in A. florea and A. cerana indica yielded 0.29 million quality reads, those were used for further data analysis. Sequences were taxonomically assigned using the reference database, yielding 867 operational taxonomic units (OTUs). For 16S rRNA amplicon sequencing, the mean Good’s coverage of A. florea and A. cerana indica samples was 82.81 ± 17.19% (mean ± SD), indicating that the majority of diversity was captured. When assessing bacterial diversity, the alpha diversity assessment of A. florea and A. cerana indica guts by means of species richness and the non-parametric estimation of shannon index indicated significant differences between them (Table 1). The bacterial species richness of A. florea (850.84) worker bees was found to be higher than that of A. cerana indica (498.60). The non-parametric estimation of shannon index for bacterial communities also differed between A. florea gut (3.12) and A. cerana indica gut (3.29). This finding suggests that the gut of A. cerana indica has more bacterial diversity than A. florea. This observation showed that both the species shared 36.3% OTUs at the genus level (Figure 1).
Figure 1. Venn diagram indicating unique and shared OTUs at genus level among A. cerana indica and A. florea of Asian honey bees.
Amplicon sequencing of the 16S rRNA gene detected 16 and 12 bacterial phyla in the guts of A. cerana indica and A. florea, respectively (Figure 2A). In A. cerana indica gut bacterial phyla distributions were Proteobacteria (50.09%), Firmicutes (26.29%), Bacteroidetes (23.19%), Actinobacteria (0.4%) and the remaining (0.03%) comprised the minor phyla. Acidobacteria, Euryarchaeota, Cyanobacteria, Chloroflexi, Saccharibacteria, Verrucomicrobia, Planctomycetes, Gemmatimonadetes, Thaumarchaeota, Armatimonadetes, FBP and Tenericutes. The following bacterial phyla were found in the gut of A. florea: Proteobacteria and Firmicutes (86.86 and 12.81% respectively), Actinobacteria (0.27%), Bacteroidetes (0.04%) were the predominant phyla, and the rest (0.02%). Acidobacteria, Verrucomicrobia, Cyanobacteria, Nitrospirae, Chloroflexi, Planctomycetes, Tectomicrobia, and Lentisphaerae are the minor phyla. The proportion of composition was not reported in Figure 2A because the abundance of 12 minor phyla was quite less. The phylum of bacteria Proteobacteria outnumbered all other bacterial phyla in the whole gut of honey bee, A. florea, whereas Proteobacteria, Firmicutes and Bacteroidetes were found to be the major phyla in the gut of A. cerana indica.
Figure 2. Bacterial taxa distributions of phylum, family and genus at different phylogenetic levels in the gut of A. cerana indica and A. florea. (A) Bacterial phyla. (B) Bacterial family. (C) Bacterial genera in the gut of A. cerana indica. (D) Bacterial genera in the gut of A. florea.
OTUs with ≥0.1% abundance that differed significantly between A. cerana indica and A. florea were filtered at the family level (Figure 2B). The family-level distributions of bacteria in the whole gut of A. cerana indica were Lactobacillaceae (26.39%), Flavobacteriaceae (23.21%), Orbaceae (19.12%), Neisseriaceae (16.3%), Oxalobacteraceae (13.91%), Enterobacteriaceae (0.53%), Bifidobacteriaceae (0.27%), Moraxellaceae (0.15%) and Nocardiaceae (0.12%). In the whole gut of honey bee, A. florea, the bacterial families were classified as Enterobacteriaceae (79.47%) and Lactobacillaceae (12.75%) as dominant families; Oxalobacteraceae (7.45%) as next dominant family, and the minor families were Nocardiaceae, Micrococcaceae, Bifidobacteriaceae, Staphylococcaceae, and Prevotellaceae. The bacterial family’s composition percentage, which was <1%, was not included in Figure 2B.
At genera level, OTUs with ≥0.1% abundance were filtered (Figures 2C,D) and distributions of the bacterial genera in the whole gut of A. cerana indica were Lactobacillus (26.41%), Apibacter (23.22%), unidentified bacteria (20.58%), Massilia (13.92%), Orbus (11.86%), Gilliamella (3.08%), Bifidobacterium (0.26%), Escherichia-Shigella (0.24%), Rhodococcus (0.12%) and Alkanindiges (0.11%) (Figure 2C). Genera in A. florea gut were Enterobacter and Lactobacillus (58.49 and 14.47% respectively) as predominant taxa, Escherichia-Shigella (13.29%), Massilia (8.45%) and Klebsiella (1.71%) as major genera; Citrobacter, Pantoea, Serratia, Rhodococcus and Morganella genera are less prevalent (Figure 2D). The bacterial genera composition percentage, which was <1%, was not shown in Figures 2C,D.
Functional predictions of A. florea and A. cerana indica gut bacterial communities using PICRUSt2 revealed higher metabolism-related gene families, inferring that these bacterial populations play a significant role in the breakdown of complex macromolecules. Additionally, gene families involved in environmental info. proc. (Information processing), genetic info. proc. related and cellular processes were identified (Figure 3).
Figure 3. Metagenomic imputation. The distribution of metabolism, environmental information processing (Environmental Info. Proc.), genetic information processing (Genetic Info. Proc.) and cellular processes-related gene families in the gut bacterial taxa of A. florea and A. cerana indica.
Assortment of each functional module such as metabolism is correspondingly prevalent in carbohydrate metabolism (32.4%) and amino acid metabolism (16.43%) respectively: Vitamin and cofactor metabolism (12.77%), nucleotide metabolism (11.13%), energy metabolism (9.1%), lipid metabolism (5.6%), glycan biosynthesis and metabolism (5.35%) and metabolism of other amino acids (4.05%). Similarly, Membrane Transport (64.65%), Signal transduction (34.42%); Translation (48.53%), Replication and repair (29.69%); Cellular motility (39.66%) were identified as significant contributors to environmental info. proc. and genetic info. proc. and cellular functions, respectively (Figure 4).
Figure 4. Heat map detailing the functional imputations. It represents the differential abundance of gene families among the gut bacterial communities of A. florea and A. cerana indica.
Our study highlights the gut bacterial diversity of two different species of honey bees of India using a high throughput approach. There were quantitative differences in the prevalence of bacterial taxa among A. cerana indica and A. florea.
Lactobacillus, Apibacter, Massilia, Orbus, Gilliamella, Bifidobacterium, Escherichia-Shigella, Rhodococcus and Alkanindiges were the predominant bacterial genera observed in the gut of A. cerana indica. Lactobacillus and Bifidobacterium, which are often present in honey bee guts, have antibacterial properties and are involved in the fermentation of carbohydrates (27). The genus Apibacter occur as host-specific members of the bee gut microbiota and various Apibacter species or strains can be found in different bee hosts (27).
In the gut of A. mellifera, Snodgrassella alvi as well as Gilliamella apicola are abundant (28). In our analysis, G. apicola was found abundant whereas S. alvi were found in traces. G. apicola can degrade pectin and other cell wall components of pollen grains (29).
Enterobacter, Lactobacillus, Klebsiella, Escherichia-Shigella, Citrobacter, Massilia, Pantoea, Serratia, Morganella and Rhodococcus, are the most common taxa discovered in the guts of A. florea worker bees. Because of anaerobic and haloalkaliphilic environments, Prevotella and Massilia cannot be cultivated in standard laboratory settings. Prevotella, Rhodococcus, Massilia, and Morganella have not been reported in gut microbiota of honey bee in the literature. However, Massilia and Prevotella are gut mutualists of Red Mason Bee, Osmia bicornis and bark beetle (Dendroctonus rhizophagus) (30, 31) respectively, known to interact with arthropods providing fitness benefits and are the most common gut bacteria.
Firmicutes and Proteobacteria were discovered in A. florea in our investigation, which was consistent with previous studies in A. florea and A. cerana indica (17, 32). In the present study, Enterobacter occur predominantly in A. florea gut followed by the genus Klebsiella. In comparison to A. cerana indica, A. florea encountered higher environmental bacterial taxa, opportunistic in nature, such as Klebsiella and Enterobacter species. Because they are wild pollinators and cannot be domesticated, the presence of non-core gut microbes in A. florea is linked to exposure to a diverse habitat and floral diversity (33). Production of protease and lipase enzymes have been found in Klebsiella and Enterobacter genera (34–36). The gut associated Klebsiella sp. found to produce multiple polysaccharide degrading enzymes in apple snail and wild dwarf bee A. florea (37, 38). In the gut of honey bees, these bacteria may aid in the digestion of pollen grains (32). On the other hand, the gut of A. cerana indica found to share bacterial species with other Apis clade that were already reported in the literature.
During nectar and pollen gathering, insects acquire and discharge microorganisms on floral surfaces, shaping the flower microbiome. These microbes found in flowers are mostly bacteria. According to a recent study, these floral occupants can act as facilitators in plant-pollinator interaction (39). Insect pollination is a natural process that involves the transfer of bacteria between flowers to seeds. Varieties of bacterial taxa that may be transferred by insects have been found by previous community-profiling techniques used on the seed microbiome of diverse plant species. Enterobacteriaceae spp. are present both in seeds, flowers and insect visitors (A. mellifera), indicating a potential microbial transmission from insect pollinators through the floral route to the seed. Insect pollinators may play a role in determining the composition of the seed microbiome which is yet to be understood (39). Cultivated bacteria belonged to the phyla Proteobacteria, Actinobacteria, and Firmicutes, with notable species differences amongst pollen species. Proteobacteria was shown to be the predominant phylum in almost pollen species, followed by Actinobacteria, Firmicutes and Acidobacteria, according to next generation sequencing. In our study, also we observed the maximal presence of Proteobacteria, Firmicutes and Actinobacteria which are found in the other pollinator species (40).
The structure and diversity of pollen microbiota were significantly impacted by plant species and pollination type. Insect-pollinated species had more similar microbiome than wind-pollinated species, implying a leveling impact by insect vectors (40). Acinetobacter spp. stimulates pollen germination and induces growth density along with releasing proteins demonstrating that germination stimulation improves bacterial fitness. Acinetobacter directly induces and benefits from pollen germination and bursting. Further investigation into microbe-pollen interactions could help to improve several elements of pollination ecology, such as floral microbial ecology, pollinator nutrition absorption from pollen, and pollen germination cues for plant reproduction (41).
Similarly, gut microbial communities were found to fall into two separate compositional categories in a 16S rRNA investigation of 28 bumble bee species in China, with varying frequencies between species (42). The first type was the common corbiculate bee-specific microbial community, which included S. alvi, G. apicola, Lactobacillus Firm4 and Lactobacillus Firm5. The second type was dominated by Enterobacteriaceae and other Proteobacteria, as well as Lactobacillus sp. found in the environment (14). Overall, it signifies the influence of gut microbiota on bee health and provides a good base for the study model to analyze evolutionary pattern of gut symbionts.
The shift to non-core bacterial species occurs in wild bees, according to in-depth approaches using experimental colonies of the Bombus terrestris, a European bumble bee, as they are exposed to stress and protruding colonizers from environmental sources, and they are more likely to encounter non-core Enterobacteriaceae in their guts than bees from indoor colonies (43–45). These findings indicate that young adults acquire the characteristic gut symbionts, resulting in a rather stable ‘normal’ microbial community of co-adapted bacteria, but that individual bee microbial communities can be conquered by opportunistic bacteria over time. The cause of these aggravations is unknown (14).
The findings of this study prompted an interest in learning more about habitat functional biodiversity. The functional diversity of bacterial gene pool in whole guts of A. cerana indica and A. florea was determined using the PICRUSt2 tool. The results revealed a diverse range of genetic variation involved in numerous essential processes viz., environmental information: signaling, signal transduction, membrane transport related gene families; genetic information: replication and repair, transcription and translation; cellular processes: cell growth and death, cellular motility, transport and catabolism, and carbohydrate, protein, and other biomolecule metabolism, comprising the production of some secondary metabolites. In the bacterial communities of A. cerana indica and A. florea, we discovered a ~61% greater congregation of gene families with metabolic functions.
The gut bacterial taxa of A. cerana indica are more diversified than those of A. florea. The high bacterial diversification in the whole gut microbiota of A. cerana indica may provide greater metabolic flexibility, allowing feeding on a wider range of pollen sources and consequently adaptation to varying environmental circumstances more rapidly. It is also feasible that a gut microbiota with less diversity but better adaptation to local conditions will be more advantageous than one with higher variety. These assumptions can be evaluated in honey bees, revealing new information about the function of bacterial diversity in host-associated bacterial populations and honey bee health. Dwarf bee Apis florea is an excellent pollinator of many important crops. The gut bacterial diversity analysis revealed that Apis florea comprises more bacteria taxa that inhabit pollination environment. Hence, Apis florea is greater pollinator compared to Apis cerana indica. It is important to assess A. florea’s potential for pollinating the native vegetation and for maintaining plant biodiversity in both forests and arid environments. It is also necessary to investigate the possibilities for making use of the bee’s capability for pollination, honey production, and improved agricultural economy. The value of A. florea as a pollinator considerably outweighs the value of the honey; hence, it should be allowed to persist in its natural habitats. Understanding the basic properties of symbiotic gut microbes has the potential to reveal previously unknown mechanisms and assist in the identification of pollinator species and their potential role in pollination.
The datasets presented in this study can be found in online repositories. The names of the repository/repositories and accession number(s) can be found in the article/Supplementary material.
DG conducted the research, performed the data analyses, and wrote and reviewed the manuscript. HS, KK, and HG edited and reviewed the manuscript. YS provided experimental support. AS planned, supervised, organized the experiment, and wrote and reviewed the manuscript. All authors contributed to the article and approved the submitted version.
The authors extend their appreciation to the Ministry of Education in KSA for funding this research work through project number KKU-IFP2-DA-7.
The authors declare that the research was conducted in the absence of any commercial or financial relationships that could be construed as a potential conflict of interest.
All claims expressed in this article are solely those of the authors and do not necessarily represent those of their affiliated organizations, or those of the publisher, the editors and the reviewers. Any product that may be evaluated in this article, or claim that may be made by its manufacturer, is not guaranteed or endorsed by the publisher.
1. Hall, AB, Tolonen, AC, and Xavier, RJ. Human genetic variation and the gut microbiome in disease. Nat Rev Genet. (2017) 18:690–9. doi: 10.1038/nrg.2017.63
2. Cox-Foster, DL, Conlan, S, Holmes, EC, Palacios, G, Evans, JD, Moran, NA, et al. A metagenomic survey of microbes in honey bee colony collapse disorder. Science. (2007) 318:283–7. doi: 10.1126/science.1146498
3. Fürst, MA, McMahon, DP, Osborne, JL, Paxton, RJ, and Brown, MJF. Disease associations between honeybees and bumblebees as a threat to wild pollinators. Nature. (2014) 506:364–6. doi: 10.1038/nature12977
4. Crotti, E, Sansonno, L, Prosdocimi, EM, Vacchini, V, Hamdi, C, Cherif, A, et al. Microbial symbionts of honeybees: a promising tool to improve honeybee health. New Biotechnol. (2013) 30:716–22. doi: 10.1016/j.nbt.2013.05.004
5. Zheng, H, Perreau, J, Powell, JE, Han, B, Zhang, Z, Kwong, WK, et al. Division of labor in honey bee gut microbiota for plant polysaccharide digestion. Proc Natl Acad Sci U S A. (2019) 116:25909–16. doi: 10.1073/pnas.1916224116
6. Tung, J, Barreiro, LB, Burns, MB, Grenier, JC, Lynch, J, Grieneisen, LE, et al. Social networks predict gut microbiome composition in wild baboons. Elife. (2015) 4:e05224. doi: 10.7554/eLife.05224
7. Wong, ACN, Chaston, JM, and Douglas, AE. The inconstant gut microbiota of Drosophila species revealed by 16S rRNA gene analysis. ISME J. (2013) 7:1922–32. doi: 10.1038/ismej.2013.86
8. McFrederick, QS, Thomas, JM, Neff, JL, Vuong, HQ, Russell, KA, Hale, AR, et al. Flowers and wild Megachilid bees share microbes. Microb Ecol. (2017) 73:188–200. doi: 10.1007/s00248-016-0838-1
9. Sihag, RC. Crop hosts and pollination potential of the red dwarf honey bee (Apis florea F.) in the semi-arid environment of north West India. J Appl Sci. (2019) 19:551–6. doi: 10.3923/jas.2019.551.556
10. Torchio, PF. Osmia ribifloris, a native bee species developed as a commercially managed pollinator of highbush blueberry (Hymenoptera: Megachilidae). J Kansas Entomol Soc. (1990) 63:427–36.
11. Meena, T. Bees as pollinators - biodiversity and conservation. Int Res J Agric Sci Soil Sci. (2012) 2:1–7.
12. Abrol, DP. The future role of dwarf honey bees in natural and agricultural systems [Internet]. Boca Raton: Routledge & CRC Press (2020).
13. Chandler, JA, Lang, J, Bhatnagar, S, Eisen, JA, and Kopp, A. Bacterial communities of diverse Drosophila species: ecological context of a host-microbe model system. PLoS Genet. (2011) 7:e1002272. doi: 10.1371/journal.pgen.1002272
14. Kwong, WK, and Moran, NA. Gut microbial communities of social bees. Nat Rev Microbiol. (2016) 14:374–84. doi: 10.1038/nrmicro.2016.43
15. Ellegaard, KM, Suenami, S, Miyazaki, R, and Engel, P. Vast differences in strain-level diversity in the gut microbiota of two closely related honey bee species. Curr Biol. (2020) 30:2520–2531.e7. doi: 10.1016/j.cub.2020.04.070
16. Vásquez, A, Forsgren, E, Fries, I, Paxton, RJ, Flaberg, E, Szekely, L, et al. Symbionts as major modulators of insect health: lactic acid Bacteria and honeybees. PLoS One. (2012) 7:e33188. doi: 10.1371/journal.pone.0033188
17. Saraithong, P, Li, Y, Saenphet, K, Chen, Z, and Chantawannakul, P. Bacterial community structure in Apis florea larvae analyzed by denaturing gradient gel electrophoresis and 16S rRNA gene sequencing. Insect Sci. (2015) 22:606–18. doi: 10.1111/1744-7917.12155
18. Martinson, VG, Danforth, BN, Minckley, RL, Rueppell, O, Tingek, S, Moran, NA, et al. A simple and distinctive microbiota associated with honey bees and bumble bees. Mol Ecol. (2011) 20:619–28. doi: 10.1111/j.1365-294X.2010.04959.x
19. Anjum, SI, Shah, AH, Aurongzeb, M, Kori, J, Azim, MK, Ansari, MJ, et al. Characterization of gut bacterial flora of Apis mellifera from north-west Pakistan. Saudi J Biol Sci. (2018) 25:388–92. doi: 10.1016/j.sjbs.2017.05.008
20. Inglis, GD, Enkerli, J, and Goettel, MS. Laboratory techniques used for entomopathogenic fungi: Hypocreale In: LA Lacey, editor. Manual of techniques in invertebrate pathology. Amsterdam: Academic Press (2012). 189–253.
21. Kumbhare, SV, Dhotre, DP, Dhar, SK, Jani, K, Apte, DA, Shouche, YS, et al. Insights into diversity and imputed metabolic potential of bacterial communities in the continental shelf of Agatti Island. PLoS One. (2015) 10:e0129864. doi: 10.1371/journal.pone.0129864
22. Magoč, T, and Salzberg, SL. FLASH: Fast length adjustment of short reads to improve genome assemblies. Bioinformatics. (2011) 27:2957–63. doi: 10.1093/bioinformatics/btr507
23. Edgar, RC. Search and clustering orders of magnitude faster than BLAST. Bioinformatics. (2010) 26:2460–1. doi: 10.1093/bioinformatics/btq461
24. Bokulich, NA, Joseph, CML, Allen, G, Benson, AK, and Mills, DA. Next-generation sequencing reveals significant bacterial diversity of botrytized wine. PLoS One. (2012) 7:e36357. doi: 10.1371/journal.pone.0036357
25. Langille, MGI, Zaneveld, J, Caporaso, JG, McDonald, D, Knights, D, Reyes, JA, et al. Predictive functional profiling of microbial communities using 16S rRNA marker gene sequences. Nat Biotechnol. (2013) 31:814–21. doi: 10.1038/nbt.2676
26. Jani, K, Dhotre, D, Bandal, J, Shouche, Y, Suryavanshi, M, and Rale, V. World’s largest mass bathing event influences the bacterial communities of Godavari, a Holy River of India. Microb Ecol. (2018) 76:706–18. doi: 10.1007/s00248-018-1169-1
27. Kwong, WK, and Moran, NA. Apibacter adventoris gen. nov., sp. nov., a member of the phylum Bacteroidetes isolated from honey bees. Int J Syst Evol Microbiol. (2016) 66:1323–9. doi: 10.1099/ijsem.0.000882
28. Kwong, WK, and Moran, NA. Cultivation and characterization of the gut symbionts of honey bees and bumble bees: description of Snodgrassella alvi gen. nov., sp. nov., a member of the family Neisseriaceae of the betaproteobacteria, and Gilliamella apicola gen. nov., sp. nov., a memb. Int J Syst Evol Microbiol. (2013) 63:2008–18. doi: 10.1099/ijs.0.044875-0
29. Engel, P, Martinson, VG, and Moran, NA. Functional diversity within the simple gut microbiota of the honey bee. Proc Natl Acad Sci U S A. (2012) 109:11002–7. doi: 10.1073/pnas.1202970109
30. Briones-Roblero, CI, Hernández-García, JA, Gonzalez-Escobedo, R, Soto-Robles, LV, Rivera-Orduña, FN, and Zúñiga, G. Structure and dynamics of the gut bacterial microbiota of the bark beetle, Dendroctonus rhizophagus (Curculionidae: Scolytinae) across their life stages. PLoS One. (2017) 12:e0175470. doi: 10.1371/journal.pone.0175470
31. Keller, A, Grimmer, G, and Steffan-Dewenter, I. Diverse microbiota identified in whole intact nest chambers of the red Mason bee Osmia bicornis (Linnaeus 1758). PLoS One. (2013) 8:e78296. doi: 10.1371/journal.pone.0078296
32. Disayathanoowat, T, Young, JPW, Helgason, T, and Chantawannakul, P. T-RFLP analysis of bacterial communities in the midguts of Apis mellifera and Apis cerana honey bees in Thailand. FEMS Microbiol Ecol. (2012) 79:273–81. doi: 10.1111/j.1574-6941.2011.01216.x
33. Ganeshprasad, DN, Lone, JK, Jani, K, Shouche, YS, Khan, KA, Sayed, S, et al. Gut bacterial flora of open nested honeybee, Apis florea. Front Ecol Evol. (2022) 10:126. doi: 10.3389/fevo.2022.837381
34. Trishin, AV, Zhdanovich, MI, Savvateeva, LV, Toptygin, AI, Donenko, FV, Kiselevskiǐ, MV, et al. Protease activity of Klebsiella pneumoniae of different virulence. Zh Mikrobiol Epidemiol Immunobiol. (2004) 4:7–11.
35. Zhen-Qian, Z, and Chun-Yun, G. Screening for lipase-producing Enterobacter agglomerans for biodiesel catalyzation. Afr J Biotechnol. (2009) 8:1273–9.
36. Feng, W, Wang, XQ, Zhou, W, Liu, GY, and Wan, YJ. Isolation and characterization of lipase-producing bacteria in the intestine of the silkworm, Bombyx mori, reared on different forage. J Insect Sci. (2011) 11:1–10. doi: 10.1673/031.011.13501
37. Imran, M, Saida, B, Ghadi, SC, Verma, P, and Shouche, YS. The gut-associated Klebsiella sp. of the apple snail produces multiple polysaccharide degrading enzymes. Curr Sci. (2016) 110:2170–2. doi: 10.18520/cs/v110/i11/2170-2172
38. Ganeshprasad, DN, Karthik, Y, Sachin, HR, and Sneharani, AH. Polysaccharide hydrolyzing enzyme activity of bacteria, native to Apis florea gut. Biomedicine. (2021) 41:768–75. doi: 10.51248/.v41i4.1013
39. Prado, A, Marolleau, B, Vaissière, BE, Barret, M, and Torres-Cortes, G. Insect pollination: an ecological process involved in the assembly of the seed microbiota. Sci Rep. (2020) 10:3575. doi: 10.1038/s41598-020-60591-5
40. Ambika Manirajan, B, Ratering, S, Rusch, V, Schwiertz, A, Geissler-Plaum, R, Cardinale, M, et al. Bacterial microbiota associated with flower pollen is influenced by pollination type, and shows a high degree of diversity and species-specificity. Environ Microbiol. (2016) 18:5161–74. doi: 10.1111/1462-2920.13524
41. Christensen, SM, Munkres, I, and Vannette, RL. Nectar bacteria stimulate pollen germination and bursting to enhance microbial fitness. Curr Biol. (2021) 31:4373–4380.e6. doi: 10.1016/j.cub.2021.07.016
42. Li, J, Powell, JE, Guo, J, Evans, JD, Wu, J, Williams, P, et al. Two gut community enterotypes recur in diverse bumblebee species. Curr Biol. (2015) 25:R652–3. doi: 10.1016/j.cub.2015.06.031
43. Meeus, I, Parmentier, L, Billiet, A, Maebe, K, van Nieuwerburgh, F, Deforce, D, et al. 16S rRNA amplicon sequencing demonstrates that indoor-reared bumblebees (Bombus terrestris) harbor a Core subset of Bacteria normally associated with the wild host. PLoS One. (2015) 10:e0125152. doi: 10.1371/journal.pone.0125152
44. Parmentier, L, Meeus, I, Mosallanejad, H, de Graaf, DC, and Smagghe, G. Plasticity in the gut microbial community and uptake of Enterobacteriaceae (Gammaproteobacteria) in Bombus terrestris bumblebees’ nests when reared indoors and moved to an outdoor environment. Apidologie. (2016) 47:237–50. doi: 10.1007/s13592-015-0393-7
Keywords: dwarf honey bee, Apis cerana indica, Apis florea, gut microbiota, pollinator
Citation: Khan KA, Ganeshprasad DN, Sachin HR, Shouche YS, Ghramh HA and Sneharani AH (2023) Gut microbial diversity in Apis cerana indica and Apis florea colonies: a comparative study. Front. Vet. Sci. 10:1149876. doi: 10.3389/fvets.2023.1149876
Received: 23 January 2023; Accepted: 26 April 2023;
Published: 12 May 2023.
Edited by:
Muhammad Kashif Iqbal, Cholistan University of Veterinary and Animal Sciences, PakistanReviewed by:
Hammad Saleem, University of Veterinary and Animal Sciences, PakistanCopyright © 2023 Khan, Ganeshprasad, Sachin, Shouche, Ghramh and Sneharani. This is an open-access article distributed under the terms of the Creative Commons Attribution License (CC BY). The use, distribution or reproduction in other forums is permitted, provided the original author(s) and the copyright owner(s) are credited and that the original publication in this journal is cited, in accordance with accepted academic practice. No use, distribution or reproduction is permitted which does not comply with these terms.
*Correspondence: A. H. Sneharani, c25laGFyYW5pYWhAZ21haWwuY29t
†These authors have contributed equally to this work
Disclaimer: All claims expressed in this article are solely those of the authors and do not necessarily represent those of their affiliated organizations, or those of the publisher, the editors and the reviewers. Any product that may be evaluated in this article or claim that may be made by its manufacturer is not guaranteed or endorsed by the publisher.
Research integrity at Frontiers
Learn more about the work of our research integrity team to safeguard the quality of each article we publish.