- 1Veterinary Diagnostic and Production Animal Medicine, Iowa State University, Ames, IA, United States
- 2AMVC, Frankfort, IN, United States
- 3Programme in Emerging Infectious Diseases, Duke-NUS Medical School, Singapore, Singapore
A porcine reproductive and respiratory syndrome virus (PRRSV) type 2 (PRRSV-2) isolate was obtained from lung samples collected from a 4.5-month-old pig at a wean-to-finish site in Indiana, USA, although no gross or microscopic lesions suggestive of PRRSV infection were observed in the lung tissue. Phylogenetic and molecular evolutionary analyses based on the obtained virus sequences indicated that PRRSV USA/IN105404/2021 was a natural recombinant isolate from Ingelvac PRRS® MLV and Prevacent® PRRS, which are PRRSV-2-modified live virus vaccines commercially available in the United States. This study is the first to report the detection of a PRRSV-2 recombinant strain consisting entirely of two modified live virus vaccine strains under field conditions. Based on clinical data and the absence of lung lesions, this PRRSV-2 recombinant strain was not virulent in swine, although its pathogenicity needs to be confirmed by clinical trials.
1. Introduction
Porcine reproductive and respiratory syndrome virus (PRRSV) is the etiologic agent of PRRS, a systemic swine disease (1). The PRRSV was first isolated in the early 1990s at a similar time in North America (USA) and Europe (the Netherlands) (2, 3). Since the emergence of PRRSV, the virus has continued to spread globally (1). The PRRSV is an enveloped, single-stranded, positive-sense RNA virus of the order Nidovirales and family Arteriviridae (4) and is classified into two distinct species: Betaarterivirus suid 1 which is colloquially known as PRRSV-1 or the European type, and Betaarterivirus suid 2 which is colloquially known as PRRSV-2 or the North American type (3–9). The entire PRRSV genome comprises ~ 15.1 kilobases consisting of at least 10 open reading frames (ORFs). Between the two PRRSV species, the ORF5 gene shares approximately 60% nucleotide identity (1, 4, 10).
Porcine reproductive and respiratory syndrome (PRRS) is one of the major swine diseases, causing significant economic losses associated with infection and clinical disease. Annual losses attributed to PRRSV are $664 million in the USA (11), $150 million in Canada (12), and between €75.72 and €650.09 per sow in Europe (13). The major clinical problem associated with PRRSV is reproductive failure in sows and respiratory distress, primarily observed not only in growing pigs but also in adult swine (1). Immunization with PRRS-modified live virus (MLV) vaccines has been used as a strategy to reduce the impact associated with PRRSV systemic disease and seeks to reduce clinical signs, viral shedding, and reproductive and respiratory losses associated with infection (14–16). PRRS MLV vaccines are the predominant immunological solution(s) used in breeding herds in the USA, with an increased number of production systems using this method of exposure (17–20). The use of PRRS MLV vaccines in breeding herds does not cause significant changes in production parameters following the first PRRS MLV exposure (21, 22).
Co-circulation of multiple PRRSV strains in a breeding herd is a reality (23). The emergence of new PRRSV strains can be due to low fidelity during RNA virus replication, recombination events, or random mutations (1, 24). A recombination event can occur between wild-type vs. wild-type PRRSV strains, wild-type vs. PRRSV MLV vaccine strains, or PRRSV MLV vaccine vs. PRRSV MLV vaccine strains. Co-infection by two or more strains in the same host and cell is a prerequisite for the occurrence of a recombination event (25, 26). Novel pathogenic PRRSV-2 strains derived from recombining wild-type and PRRSV MLV vaccine strains have been reported in China (27–29). PRRSV-2 recombinant strains derived from wild-type vs. PRRSV MLV vaccine strains have been reported in the USA (23, 30, 31) and Canada (32). PRRSV-2 recombinant strains derived from two wild-type strains have also been reported in the United States (23). In Denmark and France, it has been reported that pathogenic PRRSV-1 strains originated from the recombination between two PRRSV-1 MLV vaccine strains (33–35). Nevertheless, recombination between two PRRSV-2 MLV vaccine strains has not been reported in the USA. This study reports the detection of a recombinant PRRSV-2 isolate derived from two commercially available PRRSV-2 MLV vaccines in the USA under field conditions.
2. Methods
2.1. Overview of the clinical case and PRRS MLV exposure
At a wean-to-finish site located in Indiana, USA, where 4,882 4.5-month-old pigs were initially housed, a clinical history of coughing and increased mortality was observed. Overall wean-to-finish closeout mortality reached 21.06% (1,028 of 4,882). An assorted set of tissues (lung, spleen, and lymph nodes) were collected from three pigs, and one lung sample was collected individually from one pig (hereon referred to as “lung”). In addition, oral fluid samples (n = 2) were also collected. Samples were received at the Iowa State University Veterinary Diagnostic Laboratory (ISU-VDL) for routine disease investigation and diagnosis at the beginning of December 2021. Wean-to-finish piglets originating from a single sow farm flow had a history of endemic respiratory pathogens, including Mycoplasma hyopneumoniae (MHP) and influenza A virus (IAV), and were exposed to Prevacent® PRRS MLV vaccine, following the production system vaccination protocol. All breeding females were intramuscularly vaccinated with Ingelvac PRRS® MLV (GenBank AF066183) on 6 January 2021 in response to a PRRSV outbreak that had occurred in November 2020. Weaning-age pigs were placed in the wean-to-finish site and were intramuscularly vaccinated with Prevacent® PRRS (GenBank KU131568) between 16 August 2021 and 24 August 2021, i.e., at placement time.
2.2. Diagnostic testing
The assorted set of tissues, the individual lung sample, and oral fluid samples was submitted for ancillary testing, including routine bacterial culture evaluation, real-time polymerase chain reaction (rtPCR) or reverse transcription-real-time PCR (RT-rtPCR) testing (collectively referred to as PCR), and histopathology (assorted tissues and individual lung sample). Tissues and oral fluid samples were tested for PRRSV-1, PRRSV-2, IAV, MHP, African swine fever, and classical swine fever by PCR assays routinely used at the ISU-VDL. Specifically, the commercial PRRSV PCR, VetMAXTM PRRSV NA&EU Reagent (Thermo Fisher Scientific), targeting the conserved ORF6 and ORF7 genomic regions, was used as a screening PCR to detect and distinguish PRRSV-1 and PRRSV-2 in samples.
Upon a PRRSV-2 PCR positive result, the individual lung sample was tested using Ingelvac PRRS® MLV vaccine-like PCR (targeting the nsp2 region) and Prevacent® PRRS vaccine-like PCR (targeting the nsp2 region), as previously described (36). A PRRSV ORF5 CLAMP sequencing assay, targeting the conserved ORF5 genomic region, was applied to investigate the presence of a wild type PRRSV strain. CLAMP blocks the targeted vaccine ORF5 amplification and enhances the amplification of wild-type sequences that may be present in lower concentrations, with positive results indicating the presence of a wild-type PRRSV strain in the sample and negative results indicating the presence of a vaccine-like PRRSV strain (36, 37). PRRSV open reading frame 5 (ORF5) sequencing was performed using the Sanger technique (38, 39). PRRSV virus isolation (VI) was attempted from the lung sample following the previously described procedures (40). In brief, a 10% lung homogenate was prepared, filtered through a 0.45 μm pore-size filter, and inoculated (200 μl) into each cell of 24-well plates containing either MARC-145 or ZMAC cells. The cell plates were incubated for 1 h at 37°C with 5% CO2, after which the inoculum was removed and 1 ml of cell culture medium was added to each well. MARC-145 cells were examined daily for up to 5 days and ZMAC cells for 3 days for the development of cytopathic effect (CPE), after which the cell culture supernatants were harvested and the plates were fixed with 80% cold acetone for 10 min at room temperature, followed by immunofluorescence staining using PRRSV nucleocapsid protein-specific monoclonal antibodies (Rural Technologies Inc., South Dakota, USA) to detect the presence of PRRSV (40). If the first passage (P0) was negative, supernatants were inoculated into the respective cell lines for the second passage (P1). VI was considered negative if no growth was observed after two passages (40). Indigenous lung samples and the virus isolate obtained in ZMAC cells (P0) were both submitted for PRRSV whole genome sequencing via next-generation sequencing (NGS) technology, as previously described (39). All tests were performed on a fee-for-service basis at ISU-VDL.
2.3. PRRSV genetic comparisons and recombination analysis
The complete PRRSV genome sequence recovered from the virus isolate USA/IN105404/2021 was compared with a selected number of US wild-type and commercially available PRRSV MLV vaccine strains. The commercial PRRSV MLV vaccines currently available in the USA included in the comparison are Fostera® PRRS (GenBank AF494042), Ingelvac PRRS® MLV (GenBank AF066183), Ingelvac PRRS® ATP (GenBank DQ988080), Prevacent® PRRS (GenBank KU131568), Prime Pac® PRRS (GenBank DQ779791), and PRRSGard® (ISU-VDL in house vaccine bottle recovered sequence).
For sequence comparison and phylogenetic analysis, the NGS sequence recovered in this study, PRRS MLV vaccine sequences, and wild-type strain whole genome sequences were uploaded in Geneious Prime 2022.1.1 (https://www.geneious.com) and aligned using MAFFT v7.450 default settings (41, 42). The aligned sequences were used to construct a phylogenetic tree. Using default settings, a neighbor-joining consensus tree was built using the Tamura–Nei genetic distance with a bootstrap resampling method run with 100 replications in Geneious Tree Builder. Genes (ORF1-7) and non-structural proteins (nsp1-12) were annotated based on the ATCC VR-2332 strain (GenBank U87392.3), extracted and aligned using the MAFFT v7.450, and pairwise distances computed between USA/IN105404/2021 and all other compared sequences.
The investigation of the recombination event followed a previously described methodology (23). Aligned sequences were entered and used to detect recombination events and breakpoints using the recombination detection program RDP4 software version v.4.101 (43). A recombination event was considered if it was supported by at least six of the seven available RDP4 default parameters, namely, RDP, GENECONV, BootScan, MaxChi, Chimera, SiScan, and 3Seq. The complete PRRSV sequence with detected recombination events was further plotted using SimPlot version 3.5.1 (44) to confirm and visualize recombination breakpoints using a 200-bp window that slides with the genome alignment (20-bp step size).
3. Results
Ancillary diagnostic testing in oral fluid samples was PCR negative for IAV and PRRSV-1 and PCR positive for PRRSV-2 (Ct 36.0 and 35.7) and MHP (Ct 32.7 and 33.0). The spleen was PCR negative for classical swine fever virus and African swine fever virus. There was moderate growth of Streptococcus suis in the lung. The lung sample was negative by IAV PCR, PRRSV-1 screening PCR, and PRRSV Ingelvac MLV vaccine-like PCR but positive by MHP PCR (Ct 21.6). In addition, the lung was PRRSV-2 screening PCR positive (Ct 15.2) and PRRSV Prevacent vaccine-like PCR positive (Ct 18.0). Sanger sequencing was performed two times on the lung sample and recovered a 100% nucleotide identity. PRRSV-2 ORF5 gene sequence was classified as Lineage 5 RFLP 2-5-2, which had 99.0% similarity with the Ingelvac PRRS® MLV vaccine virus. PRRSV Ingelvac MLV ORF5 CLAMP sequencing was conducted to rule out the presence of a wild-type PRRSV, with the detection of only the Ingelvac PRRSV MLV ORF5. Microscopic evaluation of the lung tissue found numerous bronchioles surrounded by moderate-sized cuffs of lymphocytes. Alveoli in affected areas commonly contained a moderate number of neutrophils. No remarkable lesions were identified in the spleen and lymph nodes. The observed changes are consistent with MHP infection with secondary bacterial bronchopneumonia consistent with the MHP detected in the lung via PCR. Although PRRSV-2 was detected in the lung via PCR at a low Ct (Ct 15.2), no lesions suggestive of viral interstitial pneumonia were apparent in the examined sections.
In the first passage (P0), a virus isolate was recovered from the lung sample in both MARC-145 and ZMAC cells. The isolate obtained in ZMAC cells (P0) was submitted for NGS examination, and a whole PRRSV genome was recovered from the isolate. Three genome fragments, i.e., contigs, were recovered from the indigenous lung sample. Contig length, nucleotide identity, and start and end point comparisons with the isolate USA/IN105404/2021 were as follows: (a) 3,467 base pairs (bp), 99.97%, position 61–3,527; (b) 3,152 bp, 99.94%, position 3,607–6,758; and (c) 8,204 bp, 100%, position 6,794–14,997. Recombination analysis indicated that the isolate was a recombinant between the Ingelvac PRRS® MLV and Prevacent® PRRS vaccine viruses. A putative recombination breakpoint was identified at position 7,664 of the isolate, which is located in the nsp8 encoding region (Figure 1). Compared with the PRRSV-2 VR-2332 referent strain, the breakpoint was located at position 7,634 without gaps in the VR-2332 strain (GenBank U87392.3). A neighbor-joining tree revealed that the recombinant virus had closer proximity with the Prevacent® PRRS major parental strain at the whole genome level (Figure 2A) and the genomic region before the breakpoint (Figure 2B), whereas it had closer proximity with the Ingelvac® PRRS MLV minor parental strain at the genomic region after the breakpoint (Figure 2C). Nucleotide dissimilarity for genes (ORFs) and non-structural proteins (NSP) with additional PRRS MLV vaccines and wild-type strains provided supporting evidence of recombination between Ingelvac® PRRS MLV and Prevacent® PRRS (Table 1). In addition, more than 1,000 raw sequencing reads from the whole genome sequencing of the isolate USA/IN105404/2021 crossing the breakpoint were identified and illustrated by using the Integrative Genomics Viewer (Figure 3). A high-level nucleotide identity for the ORF1b-ORF7 genes was also obtained between the isolate USA/IN105404/2021 and the wild-type PRRSV-2 VR-2332 strain. A high level of similarity with the VR-2332 strain was expected since the VR-2332 strain was the parental strain used to develop the Ingelvac® PRRS MLV vaccine. The contribution of the wild-type VR-2332 strain in the recombination event is unlikely since this strain was recovered 30 years ago (3), and no history of contact or infection with wild-type PRRSV strain(s) has been previously identified in the pig herd examined in this study.
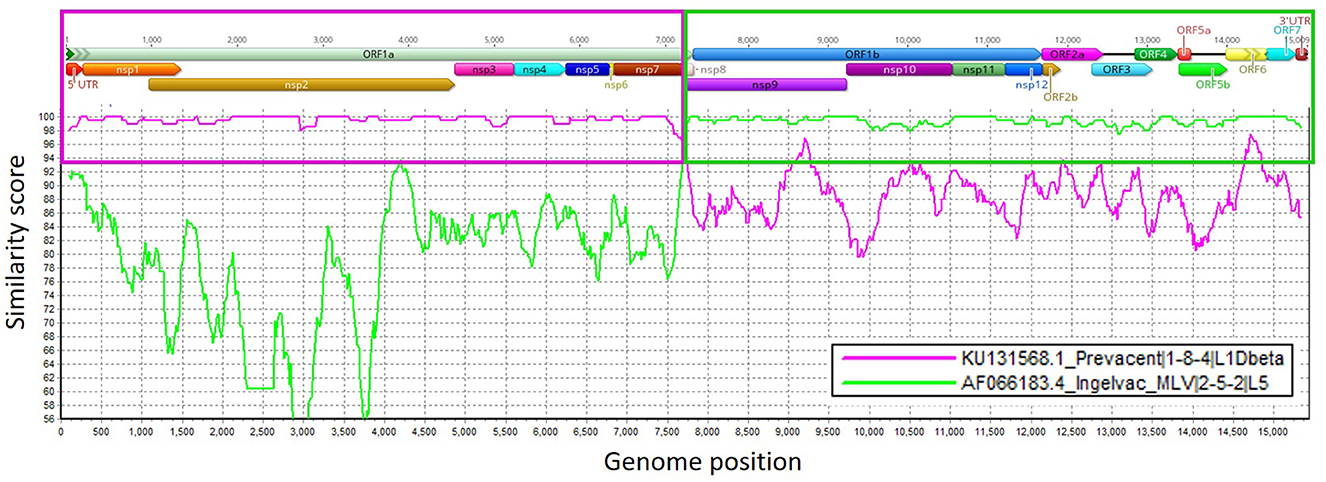
Figure 1. Similarity plot analysis using the isolate USA/IN105404/2021 strain as the query sequence against Ingelvac® PRRS MLV and Prevacent® PRRS. Genome regions (ORFs) and non-structural protein (NSP) genome regions of the recombinant strain are presented at the top of the plot. Purple and green boxes outline the recombination breakpoints, similarity scores, and genome regions that were derived from Prevacent® PRRS (major) and Ingelvac® PRRS MLV (minor) parental strains. The closer the similarity score is to 100 (y-axis), the more similar the compared strains are. Genome regions were plotted in Geneious, and recombination events were determined using RDP4 and confirmed in SimPlot (1).
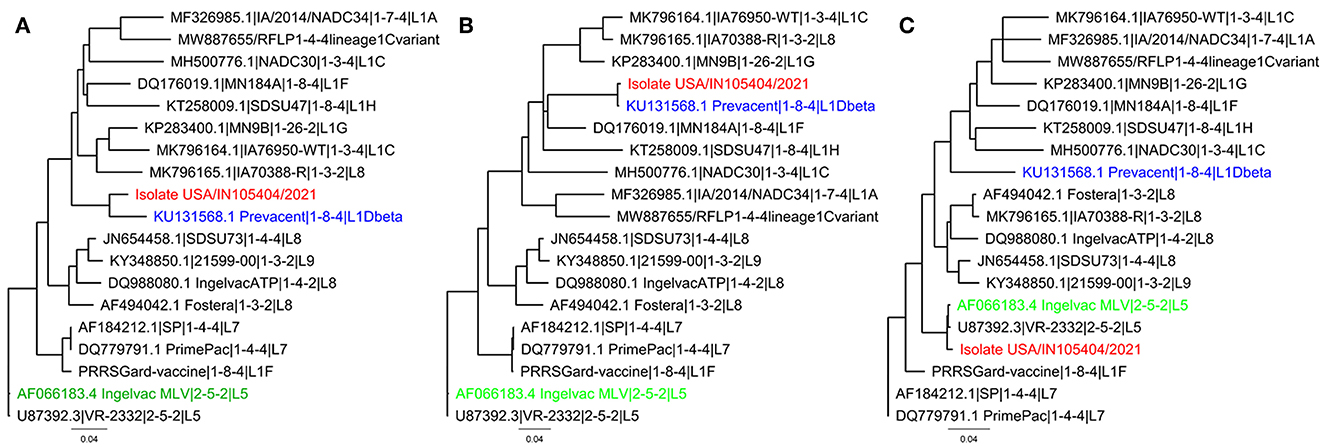
Figure 2. Neighbor-joining phylogenetic tree representing the recombinant isolate USA/IN105404/2021 (red), the major parental strain Prevacent® PRRS (blue), and the minor parental strain Ingelvac® PRRS MLV (green). Strains for additional PRRSV-modified live vaccines commercially available in the United States and other PRRSV-2 wild-type strains are presented as references. (A) Whole genome neighbor-joining phylogenetic tree; (B) neighbor-joining phylogenetic tree for the genome portion before the recombination breakpoint. (C) Neighbor-joining phylogenetic for the genome portion after the recombination breakpoint.
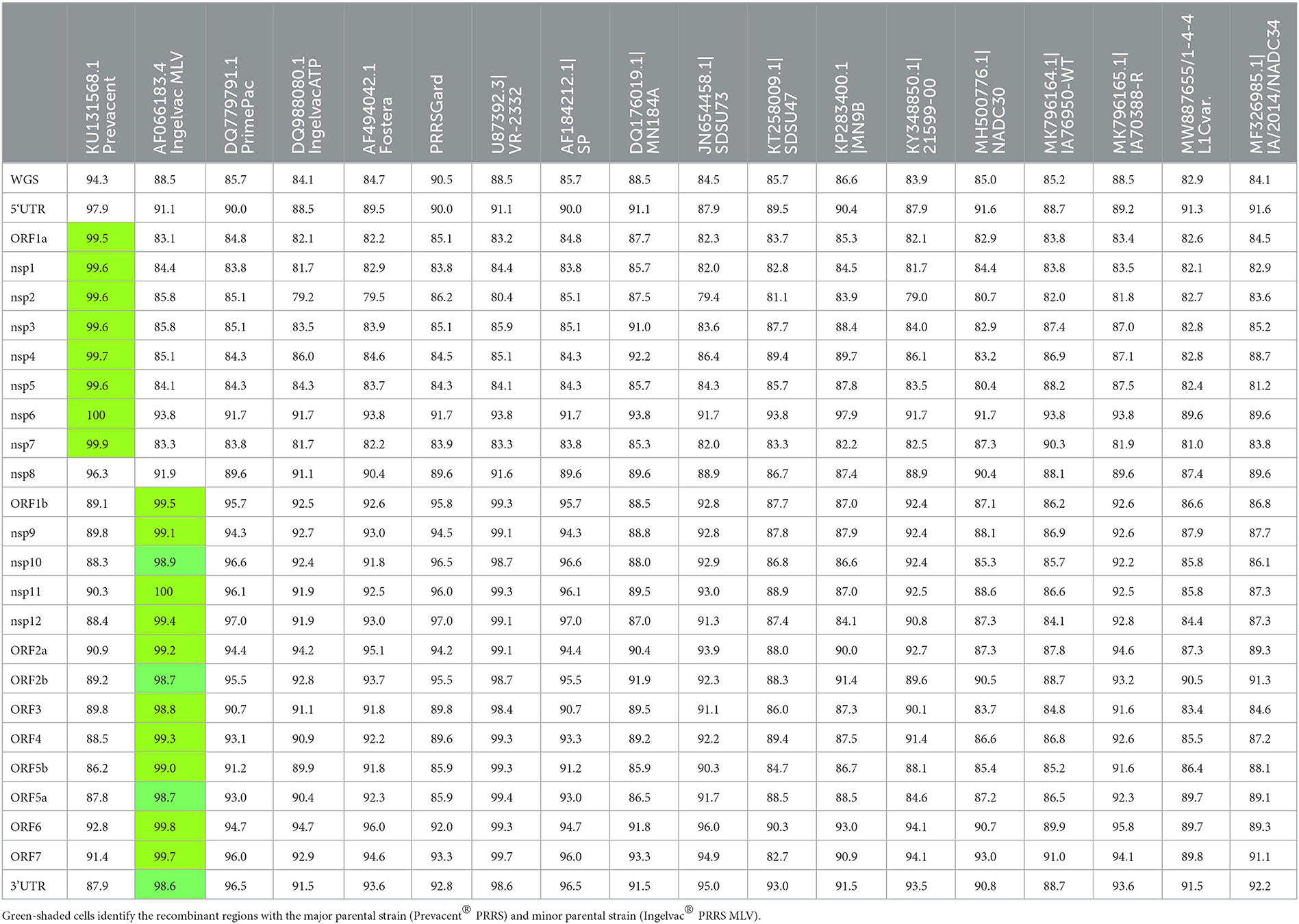
Table 1. Genome regions and nucleotide identity between isolate USA/IN105404/2021 and other vaccine and wild-type strains.
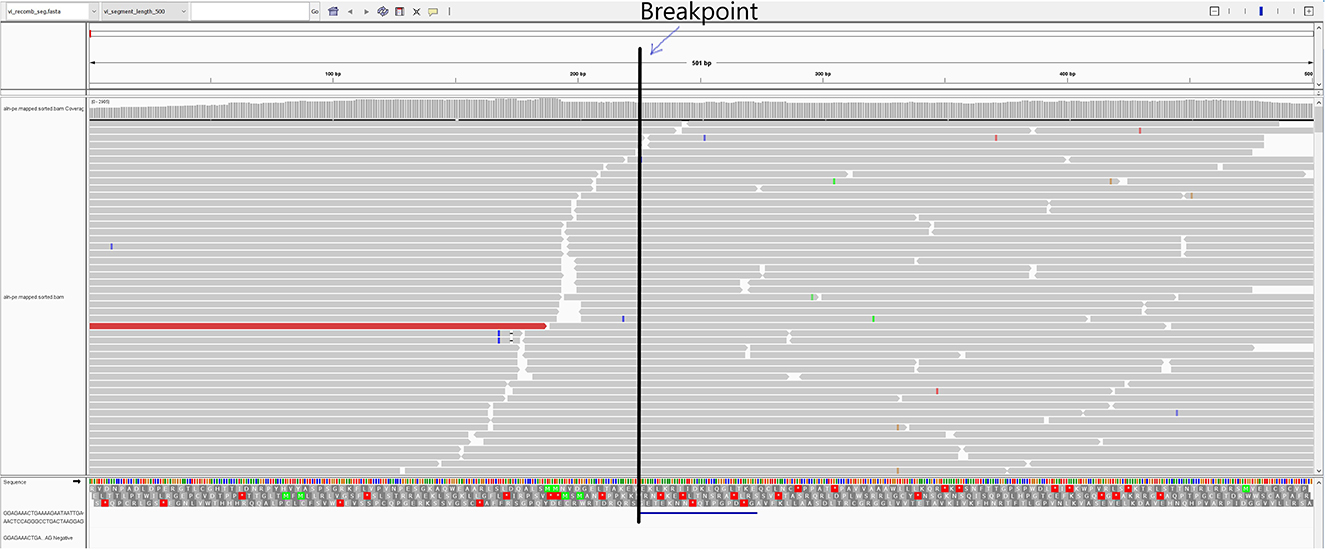
Figure 3. IGV representation of the mapped reads and virus isolate USA/IN105404/2021. The vertical line represents the recombination breakpoint identified at the 7,243 nt position. The reads marked before the breakpoint line were identical to the Prevacent® PRRS vaccine strain, whereas the reads after that matched the Ingelvac® PRRS MLV vaccine strain.
4. Discussion
This study provides solid evidence for the emergence of a natural recombinant PRRSV-2 field strain derived from two commercially available PRRSV-2 MLV vaccines available in the USA. Even though PCR detected a high PRRSV viral load (Ct 15.2) in the lung suggesting the ability of this recombinant virus to replicate in the host, microscopic lesions associated with PRRSV interstitial pneumonia were not observed. Under the conditions of this study, it appears that the recombinant strain was not virulent, although it is also possible that previous vaccination may provide protective immunity. Nonetheless, the pathogenicity of this recombinant virus needs to be unequivocally determined by an experimental inoculation study in pigs.
Porcine reproductive and respiratory syndrome virus (PRRSV) has previously been shown to persist for long periods in infected pigs (45, 46). Under experimental conditions, PRRSV was recovered from tonsils at 150 days post-infection and had the ability to develop viremia and seroconversion in a subsequent bioassay study (47). Co-infection with two (or more) strains in the same host and the same cell at the same time is required for a recombination event to occur (26). Nevertheless, exposure to both vaccine viruses has occurred in this pig flow, i.e., Ingelvac® PRRS MLV in the breeding herd and Prevacent® PRRS in the piglets. Recovery of the recombinant isolate occurred 11 months after the last Ingelvac® PRRS MLV exposure in the breeding herd and approximately 4 months after exposure of the piglets to Prevacent® PRRS. In another study in a breeding herd subsequently exposed to Ingelvac® PRRS MLV, detection of PRRSV in processing fluid samples was intermittent and associated with stress factors, e.g., the introduction of additional pathogens, after the third whole-herd exposure to the vaccine virus that occurred at 39 weeks after initial exposure (22). It would be possible that the recombinant PRRSV identified in this study occurred after some Ingelvac® PRRS MLV leakage from the breeding herd, providing the opportunity for co-circulation of both strains in the wean-to-finish population and creating the conditions necessary for a recombination event. The possibility that this recombination event of two PRRSV MLV strains could have occurred in another site or a different population of pigs and been subsequently introduced into the herd has not been explored.
Considering that co-infection with two (or more) strains in the same host and the same cell simultaneously is required for recombination events, consistent usage of a single PRRSV MLV vaccine may help prevent MLV vaccine-associated recombination events. In the case of this study, if the PRRSV MLV exposure strategy used in pigs at the wean-to-finish site was with Ingelvac® PRRS MLV or if the breeding herd was exposed to Prevacent® PRRS, keeping the vaccination strategies consistent in both populations, this recombination event could have been potentially prevented. Live virus inoculation (LVI) is still a practice used in the USA for breeding herd exposure, and further testing of LVI material prior to its use, e.g., using an NGS approach to investigate the presence of a single or multiple wild-type PRRSV strain(s) is recommended. Not using LVI material containing multiple PRRSV strains can reduce the intentional exposure of pigs to more than one PRRSV strain, decreasing the opportunity for co-infection of the same host and cell with distinct PRRSV wild-type or MLV vaccine strains. Routine testing of gilts to avoid the introduction of PRRSV-infected gilts with a farm-distinct wild-type strain(s) should be encouraged. Moreover, whenever possible, reducing the commingling of piglets infected with distinct PRRSV strains can help reduce the emergence of new recombinant strains. These are examples of measures that could help reduce the opportunity presented by emerging virulent PRRSV recombinant strains such as the L1C variant RFLP 1-4-4 strain that emerged in the USA in recent years (48, 49).
In this study, PCR results were initially confusing due to the discrepancy between screening PCR, vaccine-like PCR results, and the ORF5 sequence obtained from the lung. This prompted virus isolation and further investigation using NGS on the virus isolate and the original lung sample. The whole genome sequence was obtained from the isolate USA/IN105404/2021 and the three contig sequences were obtained from the indigenous lung sample via NGS to help align with the PRRSV screening PCR, vaccine-specific PCR, and ORF5 Sanger sequencing results. The Ingelvac MLV-like PCR yielded a negative result, whereas the Elanco Prevacent-like PCR was positive. Both of these vaccine-like PCRs target the nsp2 region of the genome, and that portion of the isolate USA/IN105404/2021 genome had high nucleotide identity (99.6%) with the Prevacent® PRRS vaccine sequence. In contrast, the PRRSV Ingelvac MLV ORF5 CLAMP and Sanger sequencing targeting the ORF5 gene recovered an Ingelvac MLV strain consistent with the whole genome sequence, considering that a portion of the whole genome (ORF1b–ORF7) had a high nucleotide identity (99%) with the Ingelvac® PRRS MLV sequence. The conflicting results between a Prevacent® PRRS PCR positive outcome and detection of an Ingelvac MLV ORF5 sequence, together with the additional NGS results, clearly suggested the presence of a recombinant PRRSV strain in the lung sample and the derived isolate.
The process of first performing virus isolation and then applying NGS to the isolate is recommended to obtain more specific sequencing reads when performing NGS, making the results more conclusive for the presence of a single virus representing the recombinant strain. An indigenous clinical sample could potentially contain more than one PRRSV strain and could produce genetic sequences of either strain when submitted for Sanger or NGS sequencing. A virus isolation process favors the selection of one strain (50). Therefore, the NGS performed on a virus isolate made it conclusive for recovering and discerning the presence of a single recombinant strain in the sample. Although plaque-purification of a virus isolate was not attempted in this study, raw sequencing reads crossing the breakpoint were identified with one terminus most closely related to the Ingelvac PRRS® MLV sequence and the other terminus most closely related to the Prevacent® PRRS sequence, providing direct evidence that the assembled recombinant sequence was from a single molecule but not from two different genomes.
These findings contribute to our understanding of PRRSV evolution and epidemiology. Compared to the ORF5 sequence, which is only 603 bp and comprises ~ 4% of the entire genome, using the full-length PRRSV genome for epidemiological investigations has a competitive advantage to better characterize PRRSV strains across all genome regions. Full-length genome sequencing is necessary to detect recombination events. The NGS technology makes it more feasible nowadays to determine the full-length PRRSV genome sequences at a reasonable cost. The use of NGS also improves epidemiological investigations when whole genomes are compared versus the exclusive use of the ORF5 gene sequence (23).
Data availability statement
The PRRSV whole-genome sequence isolate USA/IN105404/2021 has been deposited in GenBank under accession no. OQ145436 (https://www.ncbi.nlm.nih.gov/nuccore/OQ145436). The Illumina MiSeq reads have been deposited in the SRA under BioProject accession no. SRR23173625 (https://www.ncbi.nlm.nih.gov/sra/?term=SRR23173625).
Ethics statement
This research was conducted on clinical diagnostic samples that had been submitted for diagnostic testing. Written informed consent was obtained from the owners for the participation of their animals in this study.
Author contributions
GT, DM, AW, JS, and PG contributed to the conception and design of the study. GT, MZ, and AS organized the database and performed the statistical analysis. GT wrote the first draft of the manuscript. KK, JZ, DM, and GL wrote sections of the manuscript. All authors contributed to the manuscript revision, read, and approved the submitted version.
Funding
Funding for the subsequent virus investigation of the clinical case and the analysis performed under this project was provided by the Iowa State University Veterinary Diagnostic Laboratory.
Conflict of interest
The authors declare that the research was conducted in the absence of any commercial or financial relationships that could be construed as a potential conflict of interest.
Publisher's note
All claims expressed in this article are solely those of the authors and do not necessarily represent those of their affiliated organizations, or those of the publisher, the editors and the reviewers. Any product that may be evaluated in this article, or claim that may be made by its manufacturer, is not guaranteed or endorsed by the publisher.
References
1. Zimmerman JJ, Dee SA, Holtkamp DJ, Murtaugh MP, Stadejek T, Stevenson GW, et al. Porcine reproductive and respiratory syndrome viruses (Porcine Arteriviruses). In: Zimmerman JJ, Karriker LA, Ramirez A, Schwartz KJ, Stevenson GW, Zhang J, editors. Diseases of Swine. 11 ed. Hoboken, NJ: Wiley Blackwell (2019). p. 685–708.
2. Wensvoort G, Terpstra C, Pol JM, ter Laak EA, Bloemraad M, de Kluyver EP, et al. Mystery swine disease in The Netherlands: the isolation of Lelystad virus. Vet Q. (1991) 13:121–30. doi: 10.1080/01652176.1991.9694296
3. Collins JE, Benfield DA, Christianson WT, Harris L, Hennings JC, Shaw DP, et al. Isolation of swine infertility and respiratory syndrome virus (isolate ATCC VR-2332) in North America and experimental reproduction of the disease in gnotobiotic pigs. J Vet Diagn Invest. (1992) 4:117–26. doi: 10.1177/104063879200400201
4. Kuhn JH, Lauck M, Bailey AL, Shchetinin AM, Vishnevskaya TV, Bào Y, et al. Reorganization and expansion of the nidoviral family Arteriviridae. Arch Virol. (2016) 161:755–68. doi: 10.1007/s00705-015-2672-z
5. Zhang J, Yoon K-J, Zimmerman J. Overview of viruses. In: Zimmerman J, Karriker L, Ramirez A, Schwartz K, Stevenson G, Zhang J, editors. Diseases of Swine. 11 ed. Hoboken, NJ: Wiley Blackwell (2019). p. 427–37.
6. Terpstra C, Wensvoort G, Pol JM. Experimental reproduction of porcine epidemic abortion and respiratory syndrome (mystery swine disease) by infection with Lelystad virus: Koch's postulates fulfilled. Vet Q. (1991) 13:131–6. doi: 10.1080/01652176.1991.9694297
7. Meulenberg JJ, Hulst MM, de Meijer EJ, Moonen PL, den Besten A, de Kluyver EP, et al. Lelystad virus, the causative agent of porcine epidemic abortion and respiratory syndrome (PEARS), is related to LDV and EAV. Virology. (1993) 192:62–72. doi: 10.1006/viro.1993.1008
8. Nelsen CJ, Murtaugh MP, Faaberg KS. Porcine reproductive and respiratory syndrome virus comparison: divergent evolution on two continents. J Virol. (1999) 73:270–80. doi: 10.1128/JVI.73.1.270-280.1999
9. Benfield DA, Nelson E, Collins JE, Harris L, Goyal SM, Robison D, et al. Characterization of swine infertility and respiratory syndrome (SIRS) virus (isolate ATCC VR-2332). J Vet Diagn Invest. (1992) 4:127–33. doi: 10.1177/104063879200400202
10. Allende R, Lewis TL, Lu Z, Rock DL, Kutish GF, Ali A, et al. North American and European porcine reproductive and respiratory syndrome viruses differ in non-structural protein coding regions. J Gen Virol. (1999) 80:307–15. doi: 10.1099/0022-1317-80-2-307
11. Holtkamp DJ, Kliebenstein JB, Neumann EJ, Zimmerman JJ, Rotto HF, Yoder TK, et al. Assessment of the economic impact of porcine reproductive and respiratory syndrome virus on United States pork producers. J Swine Health Prod. (2013) 21:72–84. doi: 10.31274/ans_air-180814-28
12. Mussel A. A Risk, Benefit, Strength, Weakness, Opportunity and Threat Analysis for the Control and Possible Eradication of Porcine Reproductive and Respiratory Syndrome (PRRS) Virus Within the Canadian Swine Herd. Ottowa, ON: Canadian Swine Health Board (2011).
13. Nathues H, Alarcon P, Rushton J, Jolie R, Fiebig K, Jimenez M, et al. Cost of porcine reproductive and respiratory syndrome virus at individual farm level - an economic disease model. Prev Vet Med. (2017) 142:16–29. doi: 10.1016/j.prevetmed.2017.04.006
14. Pileri E, Mateu E. Review on the transmission porcine reproductive and respiratory syndrome virus between pigs and farms and impact on vaccination. Vet Res. (2016) 47:108. doi: 10.1186/s13567-016-0391-4
15. Cano JP, Dee SA, Murtaugh MP, Trincado CA, Pijoan CB. Effect of vaccination with a modified-live porcine reproductive and respiratory syndrome virus vaccine on dynamics of homologous viral infection in pigs. Am J Vet Res. (2007) 68:565–71. doi: 10.2460/ajvr.68.5.565
16. Linhares DC, Cano JP, Wetzell T, Nerem J, Torremorell M, Dee SA. Effect of modified-live porcine reproductive and respiratory syndrome virus (PRRSv) vaccine on the shedding of wild-type virus from an infected population of growing pigs. Vaccine. (2012) 30:407–13. doi: 10.1016/j.vaccine.2011.10.075
17. Arruda AG, Friendship R, Carpenter J, Greer A, Poljak Z. Evaluation of control strategies for porcine reproductive and respiratory syndrome (PRRS) in Swine breeding herds using a discrete event agent-based model. PLoS ONE. (2016) 11:e0166596. doi: 10.1371/journal.pone.0166596
18. Murtaugh MP, Genzow M. Immunological solutions for treatment and prevention of porcine reproductive and respiratory syndrome (PRRS). Vaccine. (2011) 29:8192–204. doi: 10.1016/j.vaccine.2011.09.013
19. Tousignant SJ, Perez A, Lowe JF, Yeske P, Morrison RB. Temporal and spatial dynamics of porcine reproductive and respiratory syndrome virus infection in the United States. Am J Vet Res. (2015) 7. doi: 10.2460/ajvr.76.1.70
20. Perez AM, Linhares DCL, Arruda AG, VanderWaal K, Machado G, Vilalta C, et al. Individual or common good? Voluntary data sharing to inform disease surveillance systems in food animals. Front Vet Sci. (2019) 6:194. doi: 10.3389/fvets.2019.00194
21. Moura CAA, Johnson C, Baker SR, Holtkamp DJ, Wang C, Linhares DCL. Assessment of immediate production impact following attenuated PRRS type 2 virus vaccination in swine breeding herds. Porcine Health Manag. (2019) 5:13. doi: 10.1186/s40813-019-0120-2
22. Trevisan G, Johnson C, Benjamin N, Bradner L, Linhares DCL. Description of changes of key performance indicators and PRRSV shedding over time in a naive breeding herd following a PRRS MLV exposure. Transbound Emerg Dis. (2021) 68:3230–5. doi: 10.1111/tbed.14327
23. Trevisan G, Zeller M, Li G, Zhang J, Gauger P, Linhares DCL. Implementing a user-friendly format to analyze PRRSV next-generation sequencing results and associating breeding herd production performance with number of PRRSV strains and recombination events. Transbound Emerg Dis. (2022) 69:e2214–29. doi: 10.1111/tbed.14560
24. Yuan S, Nelsen C, Murtaugh MP, Schmitt BV, Faaberg KS. Recombination between North American strains of porcine reproductive and respiratory syndrome virus. Virus Res. (1999) 61:87–98. doi: 10.1016/S0168-1702(99)00029-5
25. Liu D, Zhou R, Zhang J, Zhou L, Jiang Q, Guo X, et al. Recombination analyses between two strains of porcine reproductive and respiratory syndrome virus in vivo. Virus Res. (2011) 155:473–86. doi: 10.1016/j.virusres.2010.12.003
26. Mötz M, Stadler J, Kreutzmann H, Ladinig A, Lamp B, Auer A, et al. A conserved stem-loop structure within ORF5 is a frequent recombination hotspot for porcine reproductive and respiratory syndrome virus 1 (PRRSV-1) with a particular modified live virus (MLV) strain. Viruses. (2023) 15:258. doi: 10.3390/v15010258
27. Bian T, Sun Y, Hao M, Zhou L, Ge X, Guo X, et al. A recombinant type 2 porcine reproductive and respiratory syndrome virus between NADC30-like and a MLV-like: Genetic characterization and pathogenicity for piglets. Infect Genet Evol. (2017) 54:279–86. doi: 10.1016/j.meegid.2017.07.016
28. Liu J, Zhou X, Zhai J, Wei C, Dai A, Yang X, et al. Recombination in JXA1-R vaccine and NADC30-like strain of porcine reproductive and respiratory syndrome viruses. Vet Microbiol. (2017) 204:110–20. doi: 10.1016/j.vetmic.2017.04.017
29. Li B, Fang L, Xu Z, Liu S, Gao J, Jiang Y, et al. Recombination in vaccine and circulating strains of porcine reproductive and respiratory syndrome viruses. Emerg Infect Dis. (2009) 15:2032–5. doi: 10.3201/eid1512.090390
30. Wang A, Chen Q, Wang L, Madson D, Harmon K, Gauger P, et al. Recombination between vaccine and field strains of porcine reproductive and respiratory syndrome virus. Emerg Infect Dis. (2019) 25:2335–7. doi: 10.3201/eid2512.191111
31. Risser J, Ackerman M, Evelsizer R, Wu S, Kwon B, Hammer JM. Porcine reproductive and respiratory syndrome virus genetic variability a management and diagnostic dilemma. Virol J. (2021) 18:206. doi: 10.1186/s12985-021-01675-0
32. Lalonde C, Provost C, Gagnon CA. Whole-genome sequencing of porcine reproductive and respiratory syndrome virus from field clinical samples improves the genomic surveillance of the virus. J Clin Microbiol. (2020) 58:e0097–20. doi: 10.1128/JCM.00097-20
33. Kvisgaard LK, Kristensen CS, Ryt-Hansen P, Pedersen K, Stadejek T, Trebbien R, et al. A recombination between two type 1 porcine reproductive and respiratory syndrome virus (PRRSV-1) vaccine strains has caused severe outbreaks in Danish pigs. Transbound Emerg Dis. (2020). doi: 10.1111/tbed.13555
34. Eclercy J, Renson P, Lebret A, Hirchaud E, Normand V, Andraud M, et al. A field recombinant strain derived from two type 1 porcine reproductive and respiratory syndrome virus (PRRSV-1) modified live vaccines shows increased viremia and transmission in SPF pigs. Viruses. (2019) 11:296. doi: 10.3390/v11030296
35. Renson P, Touzain F, Lebret A, Le Dimna M, Quenault H, Normand V, et al. Complete genome sequence of a recombinant porcine reproductive and respiratory syndrome virus strain from two genotype 1 modified live virus vaccine strains. Genome Announc. (2017) 5:e00454–17. doi: 10.1128/genomeA.00454-17
36. Harmon K, Bradner L, Bieber M, Gauger P, Zhang J, editors. Put a CLAMP on It! PCR-Based Strategy to Selectively Sequence Wild-Type PRRSV in Vaccinated Herds. 50th Annual Meeting of the American Association of Swine Veterinarians. Orlando, FL: American Association of Swine Veterinarians (2019).
37. Gauger P, Harmon K, editors. PRRS CLAMP: molecular diagnostic tools to distinguish wild-type and vaccine strains of PRRSV. In: 52nd Annual Meeting of the American Association of Swine Vetrinarians. Virtual Conference, (2021).
38. Sanger F, Nicklen S, Coulson AR, DNA. sequencing with chain-terminating inhibitors. Proc Natl Acad Sci U S A. (1977) 74:5463–7. doi: 10.1073/pnas.74.12.5463
39. Zhang J, Zheng Y, Xia XQ, Chen Q, Bade SA, Yoon KJ, et al. High-throughput whole genome sequencing of Porcine reproductive and respiratory syndrome virus from cell culture materials and clinical specimens using next-generation sequencing technology. J Vet Diagn Invest. (2017) 29:41–50. doi: 10.1177/1040638716673404
40. Yim-Im W, Huang H, Park J, Wang C, Calzada G, Gauger P, et al. Comparison of ZMAC and MARC-145 cell lines for improving porcine reproductive and respiratory syndrome virus isolation from clinical samples. J Clin Microbiol. (2021) 59:e01757–20. doi: 10.1128/JCM.01757-20
41. Katoh K, Standley D, MAFFT. Multiple sequence alignment software version 7: improvements in performance and usability. Mol Biol Evol. (2013) 30:772–80. doi: 10.1093/molbev/mst010
42. Katoh K, Misawa K, Kuma K, Miyata T, MAFFT. a novel method for rapid multiple sequence alignment based on fast Fourier transform. Nucleic Acids Res. (2002) 30:3059–66. doi: 10.1093/nar/gkf436
43. Martin DP, Murrell B, Golden M, Khoosal A, Muhire B. RDP4: Detection and analysis of recombination patterns in virus genomes. Virus Evol. (2015) 1:vev003. doi: 10.1093/ve/vev003
44. Lole KS, Bollinger RC, Paranjape RS, Gadkari D, Kulkarni SS, Novak NG, et al. Full-length human immunodeficiency virus type 1 genomes from subtype C-infected seroconverters in India, with evidence of intersubtype recombination. J Virol. (1999) 73:152–60. doi: 10.1128/JVI.73.1.152-160.1999
45. Henao-Diaz A, Ji J, Gimenez-Lirola L, Baum DH, Zimmerman J. Understanding and interpreting PRRSV diagnostics in the context of “disease transition stages”. Res Vet Sci. (2020) 131:173–6. doi: 10.1016/j.rvsc.2020.04.023
46. Lopez OJ, Osorio FA. Role of neutralizing antibodies in PRRSV protective immunity. Vet Immunol Immunopathol. (2004) 102:155–63. doi: 10.1016/j.vetimm.2004.09.005
47. Allende R, Laegreid WW, Kutish GF, Galeota JA, Wills RW, Osorio FA. Porcine reproductive and respiratory syndrome virus: description of persistence in individual pigs upon experimental infection. J Virol. (2000) 74:10834–7. doi: 10.1128/JVI.74.22.10834-10837.2000
48. Trevisan G, Li G, Moura CAA, Coleman K, Thomas P, Zhang J, et al. Complete coding genome sequence of a novel porcine reproductive and respiratory syndrome virus 2 restriction fragment length polymorphism 1-4-4 Lineage 1C variant identified in Iowa, USA. Microbiol Resour Announc. (2021) 10:e0044821. doi: 10.1128/MRA.00448-21
49. Pamornchainavakul N, Kikuti M, Paploski IAD, Makau DN, Rovira A, Corzo CA, et al. Measuring how recombination re-shapes the evolutionary history of PRRSV-2: a genome-based phylodynamic analysis of the emergence of a Novel PRRSV-2 variant. Front Vet Sci. (2022) 9:846904. doi: 10.3389/fvets.2022.846904
Keywords: PRRSV, NGS, MLV vaccines, recombination, evolution
Citation: Trevisan G, Magstadt D, Woods A, Sparks J, Zeller M, Li G, Krueger KM, Saxena A, Zhang J and Gauger PC (2023) A recombinant porcine reproductive and respiratory syndrome virus type 2 field strain derived from two PRRSV-2-modified live virus vaccines. Front. Vet. Sci. 10:1149293. doi: 10.3389/fvets.2023.1149293
Received: 21 January 2023; Accepted: 27 February 2023;
Published: 28 March 2023.
Edited by:
Waithaka Mwangi, Kansas State University, United StatesReviewed by:
Jesús Hernández, National Council of Science and Technology (CONACYT), MexicoMartí Cortey, Department of Animal Health and Anatomy, Universitat Autònoma de Barcelona, Spain
Copyright © 2023 Trevisan, Magstadt, Woods, Sparks, Zeller, Li, Krueger, Saxena, Zhang and Gauger. This is an open-access article distributed under the terms of the Creative Commons Attribution License (CC BY). The use, distribution or reproduction in other forums is permitted, provided the original author(s) and the copyright owner(s) are credited and that the original publication in this journal is cited, in accordance with accepted academic practice. No use, distribution or reproduction is permitted which does not comply with these terms.
*Correspondence: Giovani Trevisan, dHJldmlzYW5AaWFzdGF0ZS5lZHU=