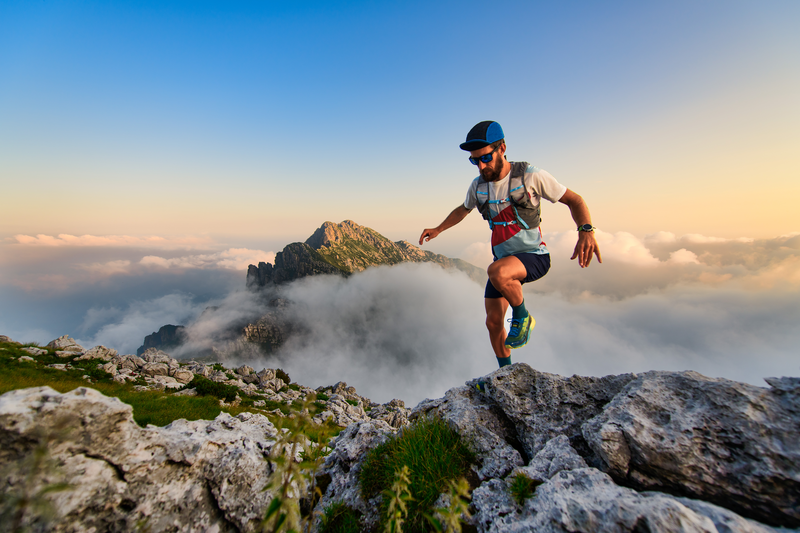
94% of researchers rate our articles as excellent or good
Learn more about the work of our research integrity team to safeguard the quality of each article we publish.
Find out more
ORIGINAL RESEARCH article
Front. Vet. Sci. , 07 March 2023
Sec. Veterinary Infectious Diseases
Volume 10 - 2023 | https://doi.org/10.3389/fvets.2023.1128863
This article is part of the Research Topic Emerging Swine Infectious Diseases View all 10 articles
Porcine Reproductive and Respiratory Syndrome Virus (PRRSV) and Classical Swine Fever Virus (CSFV) are two important pathogens, which cause serious impact on swine industry worldwide. In our previous research, rPRRSV-E2, the recombinant PRRSV expressing CSFV E2 protein, could provide sufficient protection against the lethal challenge of highly pathogenic PRRSV and CSFV, and could maintained genetically stable in vitro. Here, to evaluate the virulence reversion potential risk, rPRRSV-E2 had been continuously passaged in vivo, the stability of E2 expression and virulence of the passage viruses were analyzed. The results showed that no clinical symptoms or pathological changes could be found in the inoculated groups, and there were no significant differences of viraemia among the test groups. Sequencing and IFA analysis showed that the coding gene of exogenous CSFV E2 protein existed in the passaged viruses without any sequence mutations, deletions or insertions, and could expressed steadily. It could be concluded that the foreign CSFV E2 gene in the genome of rPRRSV-E2 could be maintained genetically stable in vivo, and rPRRSV-E2 strain had relatively low level of potential risk for virulence reversion.
Porcine reproductive and respiratory syndrome (PRRS) is characterized by late-term reproductive failure in sows and severe pneumonia in neonatal pigs (1–3). PRRSV is the causative pathogen of PRRS, which seriously affects the swine herds and causes huge economic losses to the pig industry worldwide (4–6). PRRSV is a positive-sense single-stranded RNA virus, belonging to the family Arteriviridae and genus Arterivirus. The 15-kb genome encoded at least 10 open reading frames (ORFs), which expressed at least 12 non-structural proteins (Nsps) and eight structural proteins (7–11). Although the level of bio-safety elevated since the ASF outbreak in 2018, PRRS is still one of the most significant threats to pig production in China (12–16).
Immunization is an effective measure to prevent and control PRRS, in which inactivated vaccines and subunit vaccines could not provide effective protection against PRRSV (17, 18). Live PRRS vaccines could stimulate cellular and humoral immune responses and provide respectable immune efficacy against heterologous PRRSV strains (19–23). In China, many live vaccines have been developed and used for PRRS control and contributed to the development of the pig industry (24, 25). However, the risk of virulence reversion of live vaccines has attracted extensive concerns and became one of the most important preclinical results in the innovation and development of a new vaccine (26–28).
Based on reverse genetic manipulation, several regions of the PRRSV genome could be used to insert foreign genes, to perform basic research or vaccine innovation (29–32). In the previous study, rPRRSV-E2-expressing CSFV E2 protein with the backbone of vaccine strain HuN4-F112 was engineered, and its genetic stability could be maintained in vitro for at least 25 consecutive cell passages (32). In this study, an in vivo trial was designed, and the potential risk for virulence reversion of rPRRSV-E2 was evaluated.
African green monkey kidney cells (MARC-145 cells) were cultured in minimum essential medium (MEM; Invitrogen Corporation, Carlsbad, CA, USA) and supplemented with 10% fetal bovine serum (FBS; Life Technologies Inc., Gibco/Brl Division, Grand Island, NY, USA). It was maintained in MEM supplemented with 2% FBS at 37°C in a humidified atmosphere of 5% CO2, as described previously (33). The virus titer of recombinant strain rPRRSV-E2 was determined by performing standard median tissue culture infective dose (TCID50) assays in 96-well-plates. According to the Reed and Muench method (34), rPRRSV-E2 for experimental usage in this study is the fifth passage, and the virus titer is 106.0TCID50/mL.
The expression of PRRSV N protein and CSFV E2 protein in the experimental samples was determined by immunofluorescence assays (IFAs). MARC-145 cells grew to 70% confluence in six-well-plates and were infected with rPRRSV-E2 samples and mock controls, as reported. After 48 h of infection, cell monolayers were fixed with ice-cold methanol at room temperature for 10 min, blocked with 0.1% bovine serum albumin for 30 min, and incubated with 1:600 anti-PRRSV N (SR30A, Rural Technologies) or 1:1000 anti-E2 monoclonal antibody (prepared and preserved by our laboratory) at 37°C for 2 h. After washing with PBS five times, cells were incubated at 37°C for 1 h with Alexa Fluor 594-labeled goat anti-mouse IgG (H+L) (Invitrogen Corporation). After washing with PBS, fluorescence was visualized using an inverted fluorescence microscope (model IX741; Olympus Corporation, Tokyo, Japan), as previously mentioned (35).
All pig experimental programs follow the guidelines for the Care and Use of Experimental Animals and are approved by the Ethics Committee of the Shanghai Veterinary Research Institute, Chinese Academy of Agricultural Sciences, with the number SHvri-pi-20190067. Fifty piglets at 30 days of age, which were PRRSV-free, CSFV-free, PCV2-free, and PRV-free, were purchased and bred. In the first generation, the recombinant vaccine strain rPRRSV-E2 was inoculated intramuscularly at a dose of 105.0TCID50, and the serum samples with the highest viral load were selected to be inoculated with the second-generation to the fifth-generation virus at a dose of 2 mL. Five piglets per generation were inoculated with virus/serum samples (named R1–R5 groups, respectively, and each generation of pigs was one-to-one corresponded during the experiment). Another three piglets per generation served as the control group. When the virus was transmitted to the fifth generation, the serum samples of each pig in the fifth generation at the highest level of viremia were mixed, and then five piglets were inoculated. The original generation virus was inoculated at the same time, and the clinical manifestations were observed and recorded. After vaccination, the clinical manifestations of pigs were observed and recorded every day, such as feed intake, mental state, and abnormal conditions of the ears, eyelids, and body surfaces. After feeding, rectal temperature measurement was performed every morning until necropsy. Clinical anatomy observation: The experimental pigs were killed and necropsied on the 21st day after inoculation, and the pathological changes in inguinal lymph nodes, spleen, lung, kidney, and other tissues and organs were observed. Histopathological observation: The tissues of the inguinal lymph nodes, spleen, lung, and kidney of inoculated pigs were collected and their histopathological examination was carried out.
Blood samples and serum samples were collected 0, 7, 14, and 21 days after immunization, and the serum was split into four centrifuge tubes. RNA was extracted from one tube for real-time RT-qPCR from a blood sample using the QIAprep viral RNA Kit (Qiagen, Hilden, Germany) according to the manufacturer's instructions, as previously described. The other three test tubes were immediately frozen in a refrigerator at −70°C. RT-qPCR was used to detect and analyze the viremia of serum samples. Viral RNA was extracted from 140 μL of pig serum sample. Primers PNPF/PNPR and the NP probe were used for PRRSV RT-qPCR, and the sequences of primer pairs and probes are listed in Table 1.
Re-isolation of virus: The first–fifth generation pig sera were taken and filtrated using a 0.22-μm filter, then inoculated with single-layer MARC-145 cells and adsorbed in an incubator at 37°C for 1 h. The inoculated serum was discarded, and DMEM culture solution containing 2% FBS was replaced and cultured at 37°C. The virus was collected when 60–70% CPE occurred, and RT-PCR amplification and the whole genome sequence determination were performed with PRRSV primers, of which sequences were listed in Table 1.
SPSS 14.0 software and GraphPad Prism 6.0 were used to perform all the data analysis and charting. Results: It was considered that there was a significant difference when the p < 0.05, and an extremely significant difference when the p < 0.01 or <0.001 (26).
The rPRRSV-E2 strain isolated in vivo was passaged on MARC-145 cells to detect the stability of foreign genes in the process of virus passage. The stability of the marker gene CSFV E2 during the passage of the virus was determined. The MARC-145 cells infected by the virus were detected by IFA, and the expression of the CSFV E2 gene was observed.
After the inoculation of each generation of virus/serum samples, the feed intake and mental state of the test pigs were normal, and there was no significant difference between the test and the mock control groups. There were no clinical symptoms such as anorexia, cough, diarrhea, congestion, or ulcer spots in the ears and body surface, no swelling of eyelids, and no obvious increase of eye secretion in pigs. After inoculation, all the pigs showed no symptoms of persistent hyperthermia (the body temperature was over 40.5°C, which was determined as persistent hyperthermia for 3 days). The temperature changes of the tested pigs are shown in Figure 1A. The serum samples were collected on the 7th, 14th, and 21st days after inoculation, which was used to determine the viral load in serum. The results showed that the virus load in pig serum of each group reached a peak in 7–14 days after inoculation (Figure 1B). However, the viremia level of R1–R5 groups gradually decreased, especially R5 group, their viremia levels were all below the negative value. In addition, viral shedding was also conducted to assess the risk. The results showed that the viral shedding levels were all below the negative threshold line (data not shown).
Figure 1. Safety identification of rPRRSV-E2 vaccination group and pig–pig in vivo serial passage sera inoculation group. The values of mock groups of each generation were indicated together. (A) Daily rectal temperature record of the rPRRSV-E2 inoculation group and the in vivo passage groups. (B) Viral RNA replication in serum samples detection for the rPRRSV-E2 inoculation group and the pig–pig in vivo generation sera inoculation group. Five piglets in each generation were inoculated with virus/serum samples, named R1–R5 groups, respectively, and each generation of pigs was one-to-one corresponded during the experiment. (C) Gross pathological changes of organs and tissues (spleen, lung, kidney, and lymph node) of rPRRSV-E2 inoculation group and in vivo passage sera inoculation group after necropsy. (D) Histopathological observation of organs and tissues (spleen, lung, kidney, and lymph node) of rPRRSV-E2 inoculation group and in vivo serial passage sera inoculation group after necropsy. Scale bar = 200 μm.
On the 21st day after inoculation, the experimental piglets were killed and necropsied. The dissection observation results showed that there were no visible pathological changes in the internal organs of each piglet of the rPRRSV-E2 in vivo passage group, and there was no significant difference compared with the blank control group (mock group) (Figure 1C). Histopathological examination was carried out on each group of organs, and the results showed that there was no obvious pathological damage to all kinds of parenchymal organs (spleen, lung, kidney, and lymph nodes) (Figure 1D).
The genetic stability analysis for rPRRSV-E2 was carried out in MARC-145 cells in vitro, and P5, P10, P15, and P20 viruses were sequenced. The sequencing results of the samples showed that the inserted CSFV E2 protein gene could exist stably in the viral genome, and no nucleotide variation occurred. The results of IFA showed that the E2 gene of CSFV could be expressed normally (Figure 2A). The whole genome of each generation of the virus during in vivo passage was sequenced. The results of stability analysis of the CSFV E2 gene showed that compared with its parental strain, the virus isolated in vivo (R1–R5) had no nucleotide mutation in the E2 insertion region (Figure 2B). Only a few base mutations were scattered in the whole genome. Neither base insertion nor deletion was found. IFA for CSFV E2 protein of the isolated virus showed that E2 protein was stably and abundantly expressed (Figure 2C).
Figure 2. The genetic stability of foreign genes in rPRRSV-E2 in vitro and in vivo. (A) Indirect immunofluorescence results of CSFV E2 protein of in vitro generation of P5, P10, P15, and P20 viruses. (B) Sequence alignments of CSFV E2 protein insertion region of the isolated virus of various passages in vivo. (C) Indirect immunofluorescence assay of CSFV E2 protein expression of the isolated virus after passage in vivo. Scale bar = 20 μm.
After inoculation, there was no redness and inflammation in the inoculated parts of piglets in the fifth-generation virus inoculation group and the original virus inoculation group. The feed intake and mental state of the experimental piglets inoculated with the fifth-generation virus were normal, and there was no significant difference between the blank control group and the fifth-generation virus inoculation group. There was no congestion or ulcer spots on the ears and body surfaces of inoculated piglets, no swelling of eyelids, and no obvious increase in the secretions of eyes. All the piglets did not show symptoms of persistent hyperthermia after inoculation (Figure 3A). Autopsy results showed that no visible pathological changes were found in the parenchymal organs and the lymph nodes of the piglets in each group (Figure 3B). Histopathological observation showed that there was no obvious pathological damage to the spleen, lung, kidney, lymph nodes, and other parenchymal organs of the pigs in each group (Figure 3C).
Figure 3. Safety comparison test between in vivo passage serum mixture inoculated group and original virus inoculated group. The changes in rectal temperature of pigs in each group after inoculation, autopsy results, and histopathological observation. (A) Daily rectal temperature record of rPRRSV-E2 inoculation group compared with in vivo passage sera inoculation group. (B) Gross pathological changes of organs and tissues (spleen, lung, kidney, and lymph node) of rPRRSV-E2 inoculation group (1) and in vivo passage mixed-sera inoculation group (2) after necropsy. (C) Histopathological observation of organs and tissues (spleen, lung, kidney, and lymph node) of rPRRSV-E2 inoculation group (1) and in vivo passage mixed sera inoculation group (2) after necropsy. Scale bar = 200 μm.
Since it emerged in the late 1980's, many kinds of vaccines have been developed for PRRS control, including inactive, subunit, and attenuated live vaccines. However, only live vaccines showed sufficient immune protection and are used in major pig breeding areas all over the world (17, 18, 20, 23). Although PRRS spreads and evolves rapidly, immunization still plays a very positive role in the prevention and control of PRRS (36, 37). On the other hand, live vaccines still have many defects to be improved, including the risk of virulence reversion, which has been reported by many studies and has become one of our foremost concerns of PRRSV vaccine innovation and development (26–28).
In this study, the recombinant PRRSV live vector vaccine rPRRSV-E2 strain was concerned. The E2 gene, an important protective antigen of CSFV, was inserted into the intergenic region of ORF1b and ORF2 of PRRSV by reverse genetic manipulation. Previous studies had proved that the vaccine could provide good immune protection for both HP-PRRSV and CSFV (32). The potential risk of virulence reversion for rPRRSV-E2 was assessed in this study. The results of this study showed that during the observation period, piglets inoculated P1–P5 rPRRSV-E2/serum did not show any PRRS-related clinical symptoms or visible pathological lesions in the lungs, kidneys, lymph nodes, and spleens, which demonstrated the safety of the passaged viruses and indicated that rPRRSV-E2 had no virulence reversion of in vivo, at least in these five passage processes. The stability of the PRRSV genome was very important for virulence. In the evolution history of PRRSV, the virulence change is always accompanied by great changes in the genome (38–40). The results showed that the coding gene of exogenous CSFV E2 protein existed in the passage viruses, without any sequence mutations, deletions, or insertions, and it could be stably expressed. Combined with the previous study, full-length sequencing of P5, P10, P15, P20, and P25 rPRRSV-E2 virus stocks of MARC-145 cells showed that there were no mutations in the region where foreign genes were expressed, and they remained stable at least for 25 consecutive cell passages (32). The genome of rPRRSV-E2 was stable in vivo and in vitro, and this will reduce the possibility of virulence reversion.
For live PRRSV vaccine, most studies consider that there are high risks of virulence reversion. The causes for this phenomenon should be considered from the following aspects: 1. Genetic stability of gene sequences; 2. Horizontal transmission capability of vaccine; and 3. The level and duration of viremia. In this study, the potential risk of virulence reversion of the rPRRSV-E2 strain was evaluated, and the results showed that the risk was relatively low. First, the genetic stability test in vitro and in vivo confirmed that the virus sequences of the rPRRSV-E2 strain, especially the sequences of foreign genes, were stable. In addition, rPRRSV-E2 had passed the transgenic safety evaluation. The experiment of horizontal transmission confirmed that the pigs immunized with the rPRRSV-E2 strain were kept in the same house as normal piglets and could not infect other non-immunized piglets (data not shown). There is no ability and risk of horizontal transmission. Moreover, compared with the parental vaccine strain vHuN4-F112, the rPRRSV-E2 strain has a shorter duration and a lower viremia level, because the insertion of the exogenous CSFV E2 gene makes it further weakened and has higher safety characteristics. In addition, previous studies also found that the virus shedding and virus load levels of rPRRSV-E2 in tissues were low. All these causes and factors determined that rPRRSV-E2 showed little potential risk in the assessment of virulence reversion. Furthermore, it is worth noting that the reverse genetic manipulation platform for full-length infectious cDNA clone of pHuN4-F112 is stable and convenient, and it exhibits a strong ability to accommodate foreign genes. Thus, far, we have found that multiple sites and foreign genes can be stably inserted based on this platform, and the maximum foreign gene size can reach 2,100 bp. Multiple foreign genes can be simultaneously and stably expressed. Therefore, stability is essential for PRRSV to be used as a platform for the development to develop new genetic engineering live vaccines. This may also be one of the reasons for the good performance of rPRRSV-E2 in the risk assessment of virulence reversion.
Virulence reversion is determined by many factors, including the frequency, correct use of the vaccine, selection of generation to be used as vaccines, and the characteristic of the candidate vaccine strain. This vaccine strain, which was reported with virulence reversion, mainly used the virus that has been passaged on MARC-145 cells for <100 generations (26–28). rPRRSV-E2 was generated by the full-length infectious clone of the vaccine strain HuN4-F112, which was made from the serial passage of wild-type HuN4 on MARC-145 cells for 112 generations (24, 41). HuN4-F112 was proved to be safe, and the genome was stable from generations 112–130, which increases the safety of rPRRSV-E2. We already exhibited the stability of the rPRRSV-E2 genome and the virulence of in vivo passage viruses. A previous study also demonstrated that there was no virus shedding after rPRRSV-E2 inoculation and no horizontal transmission among the co-inhabited piglets, which also indicated the safety of rPRRSV-E2 (32). Virulence reversion was determined by many factors, although the safety of rPRRSV-E2 is demonstrated, the scientific and rational usage of the vaccine still needs more attention, and the PRRS vaccine needs to be upgraded continuously.
In conclusion, rPRRSV-E2 is a candidate vaccine strain that is safe for immunization. The genome was observed to be stable in vivo and in vitro, and the level of potential risk for virulence reversion was relatively quite low.
The original contributions presented in the study are included in the article/supplementary material, further inquiries can be directed to the corresponding authors.
The animal study was reviewed and approved by Ethics Committee of the Shanghai Veterinary Research Institute, Chinese Academy of Agricultural Sciences.
This study was conceived and designed by GL and GT. YJ and FG performed the experiments and prepared the figures. FG, LL, and YZho wrote the main manuscript text. WT and LY analyzed the data. YZha, KZ, and HZ prepared the manuscript. GT made constructive comments on the experiments. All authors participated in the experiments, contributed to the article, and approved the submitted version.
We gratefully acknowledge support from the National Key Research and Development Program of China (2022YFD1800800 and 2021YFD1801401), Natural Science Foundation of Shanghai (21ZR1476900 and 21ZR1477200), Shanghai Municipal Agriculture Science and Technology Project (2022-02-08-00-12-F01115), External Collaborative Scientific Research Program (2022YJXTGCWQSX5006), the National Natural Science Foundation of China (31941017), and Central Public-interest Scientific Institution Basal Research Fund (Y2020YJ15, 2021JB01, and 2020JB01).
The authors declare that the research was conducted in the absence of any commercial or financial relationships that could be construed as a potential conflict of interest.
All claims expressed in this article are solely those of the authors and do not necessarily represent those of their affiliated organizations, or those of the publisher, the editors and the reviewers. Any product that may be evaluated in this article, or claim that may be made by its manufacturer, is not guaranteed or endorsed by the publisher.
2. Wensvoort G, de Kluyver EP, Pol JM, Wagenaar F, Moormann RJ, Hulst MM, et al. Lelystad virus, the cause of porcine epidemic abortion and respiratory syndrome: a review of mystery swine disease research at lelystad. Vet Microbiol. (1992) 33:185–93. doi: 10.1016/0378-1135(92)90046-V
3. Snijder EJ, Horzinek MC, Spaan WJ. The coronaviruslike superfamily. Adv Exp Med Biol. (1993) 342:235–44. doi: 10.1007/978-1-4615-2996-5_37
4. Holtkamp DJ, Kliebenstein JB, Neumann EJ, Zimmerman JJ, Rotto HF, Yoder TK, et al. Assessment of the economic impact of porcine reproductive and respiratory syndrome virus on United States pork producers. J Swine Health Product. (2013) 21:72–84. doi: 10.31274/ans_air-180814-28
5. Zhou L, Yang H. Porcine reproductive and respiratory syndrome in China. Virus Res. (2010) 154:31–7. doi: 10.1016/j.virusres.2010.07.016
6. Collins JE, Benfield DA, Christianson WT, Harris L, Hennings JC, Shaw DP, et al. Isolation of swine infertility and respiratory syndrome virus (isolate ATCC VR-2332) in North America and experimental reproduction of the disease in gnotobiotic pigs. J Vet Diagn Invest. (1992) 4:117–26. doi: 10.1177/104063879200400201
7. Bautista EM, Meulenberg JJ, Choi CS, Molitor TW. Structural polypeptides of the American (VR-2332) strain of porcine reproductive and respiratory syndrome virus. Arch Virol. (1996) 141:1357–65. doi: 10.1007/BF01718837
8. Snijder EJ, Meulenberg JJ. The molecular biology of arteriviruses. J Gen Virol. (1998) 79:961–79. doi: 10.1099/0022-1317-79-5-961
9. Firth AE, Zevenhoven-Dobbe JC, Wills NM, Go YY, Balasuriya UB, Atkins JF, et al. Discovery of a small arterivirus gene that overlaps the gp5 coding sequence and is important for virus production. J Gen Virol. (2011) 92:1097–106. doi: 10.1099/vir.0.029264-0
10. Li Y, Treffers EE, Napthine S, Tas A, Zhu L, Sun Z, et al. Transactivation of programmed ribosomal frameshifting by a viral protein. Proc Natl Acad Sci U S A. (2014) 111:E2172–81. doi: 10.1073/pnas.1321930111
11. van Aken D, Snijder EJ, Gorbalenya AE. Mutagenesis analysis of the Nsp4 main proteinase reveals determinants of arterivirus replicase polyprotein autoprocessing. J Virol. (2006) 80:3428–37. doi: 10.1128/JVI.80.7.3428-3437.2006
12. Zhao D, Liu R, Zhang X, Li F, Wang J, Zhang J, et al. Replication and virulence in pigs of the first african swine fever virus isolated in China. Emerg Microbes Infect. (2019) 8:438–47. doi: 10.1080/22221751.2019.1590128
13. Zhu Z, Yuan L, Hu D, Lian Z, Yao X, Liu P, et al. Isolation and genomic characterization of a Chinese NADC34-like PRRSV isolated from Jiangsu province. Transbound Emerg Dis. (2022) 69:e1015–e27. doi: 10.1111/tbed.14392
14. Xu Y, Ji X, Fu C, Hu D, Pang H, Wang T, et al. Evolution characterization and pathogenicity of a porcine reproductive and respiratory syndrome virus isolate from a pig farm in Shandong Province, China. Viruses. (2022) 14:1194. doi: 10.3390/v14061194
15. Xu H, Xiang L, Tang YD, Li C, Zhao J, Gong B, et al. Genome-wide characterization of QYYZ-like PRRSV during 2018-2021. Front Vet Sci. (2022) 9:945381. doi: 10.3389/fvets.2022.945381
16. Yu F, Liu L, Tian X, Chen L, Huang X, Sun Y, et al. Genomic analysis of porcine reproductive and respiratory syndrome virus 1 revealed extensive recombination and potential introduction events in China. Vet Sci. (2022) 9:450. doi: 10.3390/vetsci9090450
17. Prieto C, Martinez-Lobo FJ, Diez-Fuertes F, Aguilar-Calvo P, Simarro I, Castro JM. Immunisation of pigs with a major envelope protein sub-unit vaccine against porcine reproductive and respiratory syndrome virus (PRRSV) results in enhanced clinical disease following experimental challenge. Vet J. (2011) 189:323–9. doi: 10.1016/j.tvjl.2010.07.010
18. Scortti M, Prieto C, Alvarez E, Simarro I, Castro JM. Failure of an inactivated vaccine against porcine reproductive and respiratory syndrome to protect gilts against a heterologous challenge with PRRSV. Vet Rec. (2007) 161:809–13. doi: 10.1136/vr.161.24.809
19. Martinez-Lobo FJ, Diez-Fuertes F, Simarro I, Castro JM, Prieto C. The ability of porcine reproductive and respiratory syndrome virus isolates to induce broadly reactive neutralizing antibodies correlates with in vivo protection. Front Immunol. (2021) 12:691145. doi: 10.3389/fimmu.2021.691145
20. Rawal G, Angulo J, Linhares DCL, Mah CK, Van Vlaenderen I, Poulsen Nautrup B. The efficacy of a modified live virus vaccine fostera(R) PRRS against heterologous strains of porcine reproductive and respiratory syndrome virus: a meta-analysis. Res Vet Sci. (2022) 150:170–8. doi: 10.1016/j.rvsc.2022.06.026
21. Proctor J, Wolf I, Brodsky D, Cortes LM, Frias-De-Diego A, Almond GW, et al. Heterologous vaccine immunogenicity, efficacy, and immune correlates of protection of a modified-live virus porcine reproductive and respiratory syndrome virus vaccine. Front Microbiol. (2022) 13:977796. doi: 10.3389/fmicb.2022.977796
22. Duerlinger S, Knecht C, Sawyer S, Balka G, Zaruba M, Ruemenapf T, et al. Efficacy of a modified live porcine reproductive and respiratory syndrome virus 1 (PRRSV-1) vaccine against experimental infection with PRRSV Aut15-33 in weaned piglets. Vaccines. (2022) 10:934. doi: 10.3390/vaccines10060934
23. Zuckermann FA, Garcia EA, Luque ID, Christopher-Hennings J, Doster A, Brito M, et al. Assessment of the efficacy of commercial porcine reproductive and respiratory syndrome virus (PRRSV) vaccines based on measurement of serologic response, frequency of Gamma-Ifn-producing cells and virological parameters of protection upon challenge. Vet Microbiol. (2007) 123:69–85. doi: 10.1016/j.vetmic.2007.02.009
24. Tian ZJ, An TQ, Zhou YJ, Peng JM, Hu SP, Wei TC, et al. An attenuated live vaccine based on highly pathogenic porcine reproductive and respiratory syndrome virus (HP-PRRSV) protects piglets against HP-PRRS. Vet Microbiol. (2009) 138:34–40. doi: 10.1016/j.vetmic.2009.03.003
25. Leng X, Li Z, Xia M, He Y, Wu H. Evaluation of the efficacy of an attenuated live vaccine against highly pathogenic porcine reproductive and respiratory syndrome virus in young pigs. Clin Vaccine Immunol. (2012) 19:1199–206. doi: 10.1128/CVI.05646-11
26. Jiang YF, Xia TQ, Zhou YJ, Yu LX, Yang S, Huang QF, et al. Characterization of three porcine reproductive and respiratory syndrome virus isolates from a single swine farm bearing strong homology to a vaccine strain. Vet Microbiol. (2015) 179:242–9. doi: 10.1016/j.vetmic.2015.06.015
27. Yu LX, Wang X, Yu H, Jiang YF, Gao F, Tong W, et al. The emergence of a highly pathogenic porcine reproductive and respiratory syndrome virus with additional 120aa deletion in Nsp2 region in Jiangxi, China. Transbound Emerg Dis. (2018) 65:1740–8. doi: 10.1111/tbed.12947
28. Wang J, Zhang M, Cui X, Gao X, Sun W, Ge X, et al. Attenuated porcine reproductive and respiratory syndrome virus regains its fatal virulence by serial passaging in pigs or porcine alveolar macrophages to increase its adaptation to target cells. Microbiol Spectr. (2022) 10:e0308422. doi: 10.1128/spectrum.03084-22
29. Wang Y, He W, Li Q, Xie X, Qin N, Wang H, et al. Generation of a porcine reproductive and respiratory syndrome virus expressing a marker gene inserted between ORF4 and ORF5a. Arch Virol. (2020) 165:1803–13. doi: 10.1007/s00705-020-04679-3
30. Li Y, Ren C, Li C, Xiao Y, Zhou Y. A recombinant porcine reproductive and respiratory syndrome virus stably expressing a Gaussia luciferase for antiviral drug screening assay and luciferase-based neutralization assay. Front Microbiol. (2022) 13:907281. doi: 10.3389/fmicb.2022.907281
31. Xu YZ, Zhou YJ, Zhang SR, Jiang YF, Tong W, Yu H, et al. Stable expression of foreign gene in nonessential region of nonstructural protein 2 (Nsp2) of porcine reproductive and respiratory syndrome virus: applications for marker vaccine design. Vet Microbiol. (2012) 159:1–10. doi: 10.1016/j.vetmic.2012.03.015
32. Gao F, Jiang Y, Li G, Zhou Y, Yu L, Li L, et al. Porcine reproductive and respiratory syndrome virus expressing E2 of classical swine fever virus protects pigs from a lethal challenge of highly-pathogenic PRRSV and CSFV. Vaccine. (2018) 36:3269–77. doi: 10.1016/j.vaccine.2018.04.079
33. Gao F, Yao H, Lu J, Wei Z, Zheng H, Zhuang J, et al. Replacement of the heterologous 5' untranslated region allows preservation of the fully functional activities of type 2 porcine reproductive and respiratory syndrome virus. Virology. (2013) 439:1–12. doi: 10.1016/j.virol.2012.12.013
34. Pizzi M. Sampling variation of the fifty percent end-point, determined by the reed-muench (Behrens) method. Hum Biol. (1950) 22:151–90.
35. Li L, Wei Z, Zhou Y, Gao F, Jiang Y, Yu L, et al. Host Mir-26a suppresses replication of porcine reproductive and respiratory syndrome virus by upregulating type I interferons. Virus Res. (2015) 195:86–94. doi: 10.1016/j.virusres.2014.08.012
36. Jiang Y, Li G, Yu L, Li L, Zhang Y, Zhou Y, et al. Genetic diversity of porcine reproductive and respiratory syndrome virus (PRRSV) from 1996 to 2017 in China. Front Microbiol. (2020) 11:618. doi: 10.3389/fmicb.2020.00618
37. Corzo CA, Mondaca E, Wayne S, Torremorell M, Dee S, Davies P, et al. Control and elimination of porcine reproductive and respiratory syndrome virus. Virus Res. (2010) 154:185–92. doi: 10.1016/j.virusres.2010.08.016
38. Zhou YJ, Hao XF, Tian ZJ, Tong GZ, Yoo D, An TQ, et al. Highly virulent porcine reproductive and respiratory syndrome virus emerged in China. Transbound Emerg Dis. (2008) 55:152–64. doi: 10.1111/j.1865-1682.2008.01020.x
39. Wang Y, Liang Y, Han J, Burkhart KM, Vaughn EM, Roof MB, et al. Attenuation of porcine reproductive and respiratory syndrome virus strain Mn184 using chimeric construction with vaccine sequence. Virology. (2008) 371:418–29. doi: 10.1016/j.virol.2007.09.032
40. Brockmeier SL, Loving CL, Vorwald AC, Kehrli ME, Baker RB, Nicholson TL, et al. Genomic sequence and virulence comparison of four type 2 porcine reproductive and respiratory syndrome virus strains. Virus Res. (2012) 169:212–21. doi: 10.1016/j.virusres.2012.07.030
Keywords: rPRRSV-E2, virulence reversion, genetic stability, in vivo passage, CSFV E2
Citation: Jiang Y, Gao F, Li L, Zhou Y, Tong W, Yu L, Zhang Y, Zhao K, Zhu H, Liu C, Li G and Tong G (2023) The rPRRSV-E2 strain exhibited a low level of potential risk for virulence reversion. Front. Vet. Sci. 10:1128863. doi: 10.3389/fvets.2023.1128863
Received: 21 December 2022; Accepted: 08 February 2023;
Published: 07 March 2023.
Edited by:
Tao Lin, New Hope Group, United StatesReviewed by:
Yanhua Li, Yangzhou University, ChinaCopyright © 2023 Jiang, Gao, Li, Zhou, Tong, Yu, Zhang, Zhao, Zhu, Liu, Li and Tong. This is an open-access article distributed under the terms of the Creative Commons Attribution License (CC BY). The use, distribution or reproduction in other forums is permitted, provided the original author(s) and the copyright owner(s) are credited and that the original publication in this journal is cited, in accordance with accepted academic practice. No use, distribution or reproduction is permitted which does not comply with these terms.
*Correspondence: Guoxin Li, Z3VveGlubGlAc2h2cmkuYWMuY24=; Guangzhi Tong, Z3p0b25nQHNodnJpLmFjLmNu
†These authors have contributed equally to this work
Disclaimer: All claims expressed in this article are solely those of the authors and do not necessarily represent those of their affiliated organizations, or those of the publisher, the editors and the reviewers. Any product that may be evaluated in this article or claim that may be made by its manufacturer is not guaranteed or endorsed by the publisher.
Research integrity at Frontiers
Learn more about the work of our research integrity team to safeguard the quality of each article we publish.