- Hubei Key Laboratory of Animal Nutrition and Feed Science, Engineering Research Centre of Feed Protein Resources on Agricultural By-Products, Ministry of Education, Wuhan Polytechnic University, Wuhan, China
This study was to investigate the impact of α-terpineol (α-TPN) and Bacillus coagulans (B. coagulans) on weaned piglets infected with Enterotoxigenic Escherichia coli (ETEC). Thirty-two weaned piglets were assigned into four treatments: Control group (basal diet), STa group (basal diet + 1 × 1010 CFU ETEC), TPN+STa group (basal diet + 0.01% α-TPN + ETEC) and BC+STa group (basal diet + 2 × 106 CFU B. coagulans + ETEC). Result showed that both α-TPN and B. coagulans could alleviate diarrhea (decreased diarrhea rate), intestinal injury (improved intestinal morphology, decreased blood I-FABP concentration, increased protein expression level of Occludin), oxidative stress (increased GSH-Px activity and decreased MDA content) and inflammation (altered concentration of TNF-α, IL-1β in blood) induced by ETEC infection. Mechanism investigation further demonstrated that the beneficial effects of α-TPN and B. coagulans supplementation upon ETEC infection may be achieved by decreasing the protein expression levels of caspase-3, AQP4 and p-NF-κB and decreasing the gene expression levels of INSR and PCK1. Besides, α-TPN supplementation could specifically decreased expression level of gene b0,+AT, and B. coagulans supplementation could specifically decreased expression level of gene AQP10 and protein HSP70 in ETEC-infected weaned piglets. These results suggested that α-TPN and B. coagulans can be used as antibiotic alternatives against ETEC infection in weaned piglets.
1. Introduction
Enterotoxigenic Escherichia coli (ETEC) strains are the most common bacterial pathogens that cause diarrhea, which is one of the most important diseases in young farm animals, also the second leading cause of death in children under 5 years old (1, 2). ETEC-induced post-weaning diarrhea in piglets mainly occurs within the 1st week after weaning (3). ETEC infection could result in great economic losses to the swine industry due to reduced growth performance as well as increased morbidity and mortality of piglets. Over two thirds of diarrhea in piglets caused by ETEC are resulted from STa (heat-stable toxin A)-producing ETEC strains, thereby the most heated studies of ETEC frequently focus on STa (4). In order to study the pathology and toxicology of STa, a recombinant E. coli expressing STa, named LMG194-STa, was constructed by using E. coli strain LMG194 and the plasmid pBAD202/Dtopo in our previous work. E. coli LMG194-STa has been demonstrated to induce diarrhea and intestinal injury in piglets (5, 6).
Since the use of antibiotic in livestock has been reducing in China, many studies on piglet diarrhea have devoted to exploring the possible alternatives to antibiotics against ETEC infection (7). For which, essential oils and probiotics have been considered as ideal alternatives to antibiotics, due to their natural activities against pathogenic bacteria. Plant essential oils contain a wide variety of secondary metabolites that can inhibit or slow the growth of bacteria, yeasts, and molds (8). α-Terpineol (α-TPN) is a volatile monoterpene alcohol, a major component of the essential oils from various plant species (9). Research has demonstrated that it has insecticidal, antimicrobial, antispasmodic, anticonvulsant, antinociceptive and immunostimulant properties (10). It is present in the essential oil of several medicinal plants, such as Punica granatum L. (Pomegranate), Rosmarinus officinalis L. (Rosemary), Psidium guajava L. (Guava), which is popularly used for treating diarrhea. The antidiarrheal activity of α-TPN has been evaluated in mice (11). This evidence suggested that α-TPN could be used to reduce diarrhea and resist ETEC infection and STa toxin.
Bacillus coagulans (B. coagulans) is a lactic acid producing bacterial species, which is catalase positive, spore forming, motile, and a facultative anaerobe (12). Because of the strong resistance, resurrection, and stability, spores of B. coagulans could be activated in the acidic environment of the stomach, then germinate and proliferate in small intestines (13). Such spores could adapt to the gastrointestinal environment and reach the gut smoothly, and thereby play the beneficial impacts in the intestines (13). Due to these characteristics, B. coagulans has been frequently used in animal nutrition, particularly as a probiotic in poultry, cattle and shrimp (14). Our previous works have also demonstrated that supplementation of B. coagulans modulated metabolism and microbiome, alleviate intestinal integrity, oxidative stress and diarrhea (15), which may be also a good option for the treatment of ETEC infection.
Based on our previous studies on the recombinant E. coli LMG194-STa, the present study was conducted to investigate the protective effects of α-TPN and B. coagulans on intestinal function in weaned piglets infected with LMG194-STa, thereby further exploring the possible mechanisms. Findings of this study can contribute to the development of alternatives to antibiotics, and provide a novel strategy against ETEC infection.
2. Material and methods
2.1. Animals and design
The animal use protocol for the present study was approved by the Animal Care and Use Committee at Wuhan Polytechnic University (0328-2017-0117). Thirty-two crossbred healthy piglets (Duroc × Landrace × Yorkshire) were weaned at 21 days of age. Each piglet was individually housed in a 1.20 × 1.10 m2 steel metabolic cage with eight replicates per treatment. After weaning, piglets had a 3-day adaptation. During this period, piglets had free access to the basal diet for adapting to solid food. Then piglets were assigned randomly on the basis of body weight (5.62 ± 0.66 kg) and litter origin to 4 groups: 1) control group-piglets were fed the basal diet; 2) STa group-piglets were fed the basal diet and challenged with 1 × 1010 E. coli LMG194-STa; 3) TPN+STa group-piglets were fed the basal diet supplemented with 0.01% α-TPN, and challenged with 1 × 1010 CFU E. coli LMG194-STa and; 4) BC+STa group-piglets were fed the basal diet supplemented with 2 × 106 CFU B. coagulans, and challenged with 1 × 1010 CFU E. coli LMG194-STa. The composition and nutrient levels of the basal diet are shown in Table 1. From day 1 to day 10 of the trial, piglets were fed the basal diet with or without α-TPN or B. coagulans. All diets were isocaloric. On day 11 and day 12, each piglet was orally inoculated with 1 × 1010 CFU of E. coli LMG194-STa at both 8:00 and 20:00. The dose of ETEC and B. coagulans were determined based on our previous studies (15, 16). The dose of α-TPN was chosen according to the result of a preliminary experiment. The recombinant E. coli LMG194-STa were constructed by our previous work, and cultured in Lysogeny broth liquid medium (5, 6). B. coagulans were produced by fermenting; the conditions were 200 r/min of stirring speed and 350 L/h of throughput. The products of fermentation were filtered by organic ceramic membrane filtration equipment, then mixed with mineral adsorbent by 1:1. Final products were a dry powder that were counted by the plate counting method to determine the dosage and then were mixed in the diet. On the 15th day of the trial, blood samples were collected from the anterior vena cava and transferred into a tube with heparin sodium for anticoagulation. Plasma and serum were separated by centrifugation at 3,500 rpm for 15 min and stored at −20°C. All piglets were then slaughtered under anesthesia. Intestine, and intestinal content were collected and stored at −80°C until assay.
2.2. Plasma parameters analysis
The activity of superoxidase dismutase (SOD) and glutathione peroxidase (GSH-Px), and the content of malondialdehyde (MDA) in plasma were determined by spectrophotometry using commercially available kits (Jiancheng Bioengineering Institute, Nanjing, China). The concentration of tumor necrosis factor alpha (TNF-α), interleukin 1 beta (IL-1β), and intestinal fatty-acid binding protein (I-FABP) was determined by ELISA using Quantikine® Assay Kits (Fisher Scientific Inc., Minneapolis, MN, USA). Assays were performed in triplicate.
2.3. Intestinal morphology
Intestinal tissue samples used for the morphometric study were dehydrated and embedded in paraffin, sectioned at a thickness of 4 mm, and stained with haematoxylin and eosin. Morphological measurements were carried out using a light microscope (Leica microsystems, Wetzlar, Germany) equipped with the Leica Application Suite image analysis software (Leica microsystems, Wetzlar, Germany). Intestinal villus height and width, as well as crypt depth, were measured to calculate both the villus crypt ratio and villous surface area.
2.4. Real-time PCR
The gene expression levels were quantitated by the method of real-time PCR. Firstly, RNA from the frozen samples were isolated using the Trizol Reagent protocol (Invitrogen, Carlsbad, CA). Then cDNA was synthesized using a PrimeScript® RT reagent kit (Takara, Dalian, China) with gDNA Eraser. Finally, the real-time PCR was carried out with the SYBR® Premix Ex Taq™ (Takara, Dalian, China) on 7,500 Fast Real-Time PCR System (Applied Biosystems, Foster City, CA, USA). Ribosomal protein L4 (RPL4) was used as the reference gene. Data was analyzed by using the 2−ΔΔCt method as described previously (17). The primer sequences are shown in Table 2.
2.5. Western blot
The expression levels of proteins were performed using western blot. Tissue extracts were prepared using RIPA buffer. After electrophoresis, proteins were transferred onto PVDF membranes. Then the membrane was blocked for 1 h at room temperature with 5% milk solution, and incubated with primary antibodies at 4°C overnight. The following antibodies were used in this study: NF-κB (6956S, Cell Signaling Technology), pNF-κB (3033, Cell Signaling Technology), Mx1 (ab79609, Abcam), IFN-α (ab230993, Abcam), HSP70 (ADI-SPA-810-F, Enzo Life Sciences), I-FABP (sc-16063, Santa Cruz Biotechnology), Caspase-3 (9661S, Cell Signaling Technology), Villin (sc-7672, Santa Cruz Biotechnology), AQP4 (ab46182, Abcam), AQP3 (ab125219, Abcam), Claudin (RF217968, Invitrogen), and Occludin (TC259714, Invitrogen), β-actin (PA1-46296, Invitrogen). Subsequently, appropriate HRP-conjugated secondary antibodies were used followed by incubation at room temperature for 1 h. Blots were visualized using a chemiluminescence kit (Amersham Biosciences, Uppsala, Sweden) and image forming system (Alpha Innotech, New York, NY, USA).
2.6. Statistical analysis
All data, expressed as means ± SD, were analyzed by one-way analysis of variance (ANOVA). The normality and constant variance for experimental data were tested by the Levene's test. Differences among treatment means were determined by Duncan's multiple range tests. All statistical analyses were performed using SPSS 24.0 software (Chicago, IL, USA). P-values < 0.05 were taken to indicate statistical significance.
3. Results
3.1. Growth performance
During the experimental period, the average daily gain (ADG), average daily feed intake (ADFI), and diarrhea rate (DR) was recorded and calculated (Table 3). There was no significant difference in ADG, ADFI and F/G among these treatments throughout the experiment. However, ETEC infection significantly increased diarrhea rate during day 11 to 15 of the trial, whereas the supplementation of both α-TPN and B. coagulans significantly alleviated diarrhea (P < 0.05).
3.2. Plasma immunity, intestinal injury, and antioxidant ability parameters
Inflammatory, intestinal injury and redox indexes in plasma are present in Table 4. Compared with control group, ETEC infection significantly increased TNF-α and MDA concentration, decreased GSH-Px activity in plasma (P < 0.05). IL-1β and I-FABP concentration had a tend to be increased by ETEC infection. Compared with STa group, TPN+STa group significantly decreased the concentration of TNF-α, IL-1β, and MDA, and increased GSH-Px activity, I-FABP concentration had a tend to be decreased by α-TPN supplementation; BC+STa group significantly decreased the concentration of IL-1β, I-FABP and MDA in plasma (P < 0.05), and tend to increase GSH-Px activity.
3.3. Intestinal morphology
The microscopic pictures of intestinal mucosal are shown in Figure 1, and the results of morphology is summarized in Table 5. The statistical analysis showed that compared to control group, ETEC infection significantly decreased villus height, width, surface area and the ratio of villus height to crypt depth in the duodenum, jejunum and ileum, increased crypt depth in the ileum (P < 0.05). Compared to STa group, α-TPN supplementation significantly increased villus surface area in the duodenum, increased villus height, surface area and villus height/crypt depth ratio in the ileum; B. coagulans supplementation significantly increased villus height in the duodenum, villus height, villus width and surface area in the jejunum, villus height and width and villus height/crypt depth ratio in the ileum, decreased crypt depth in the ileum (P < 0.05).
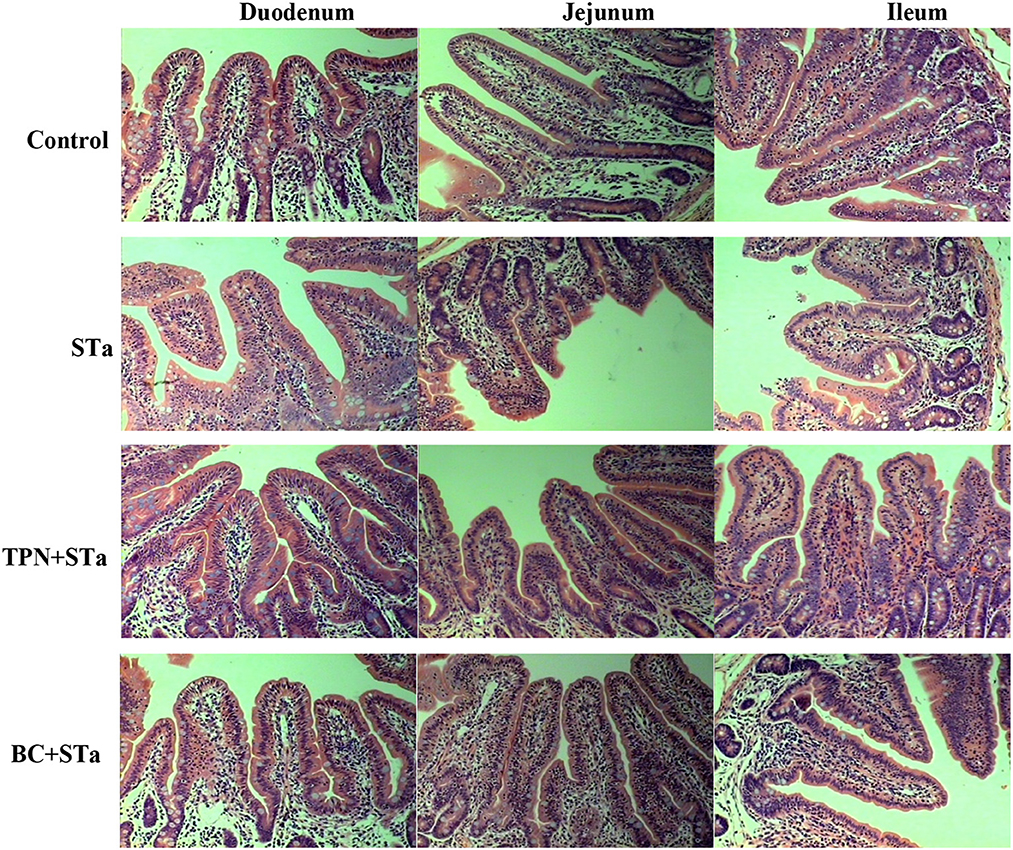
Figure 1. Intestinal mucosal morphology observed by H&E staining under an optical microscope. Magnification x 200.
3.4. Gene expression by real-time PCR
Expression level of genes associated with intestinal barrier, transport and metabolism are shown in Table 6. Compared to control group, ETEC infection significantly decreased expression level of genes matrix metalloproteinase-3 (MMP3) and Lipoprotein lipase (LPL) in the jejunum and colon, increased expression level of villin, I-FABP, b0,+AT, aquaporin 10 (AQP10), and insulin receptor (INSR) in the jejunum and colon as well as KCNJ13 and phosphoenolpyruvate carboxykinase 1 (PCK1) in the jejunum (P < 0.05). Compared to STa group, α-TPN supplementation significantly increased expression level of LPL in the jejunum, decreased expression level of villin, I-FABP, b0,+ amino acid transporter (b0,+AT), INSR, and PCK1 in the jejunum and colon as well as MMP3 in the colon; B. coagulans supplementation increased expression level of b0,+AT in the colon, decreased expression levels of villin, MMP3, AQP10, INSR and PCK1 in the jejunum colon and I-FABP in the colon (P < 0.05).
3.5. Protein expression by Western blot
Expression level of proteins associated with intestinal barrier, immunity and metabolism in the jejunum are shown in Figure 2. Compared to control group, ETEC infection significantly increased expression levels of proteins p-NF-κB, Mx1, IFN-α, HSP70, Caspase-3, Villin, and AQP4, decreased expression level of NF-κB, I-FABP, Occludin and AQP3 (P < 0.05). Compared to STa group, α-TPN supplementation significantly increased expression level of proteins NF-κB, Occludin and AQP3, decreased expression level of p-NF-κB, Mx1, Caspase-3, Villin, and AQP4; B. coagulans supplementation increased expression level of NF-κB, Occludin and AQP3, decreased expression level of p-NF-κB, Mx1, HSP70, I-FABP, Caspase-3, Villin and AQP4 (P < 0.05).
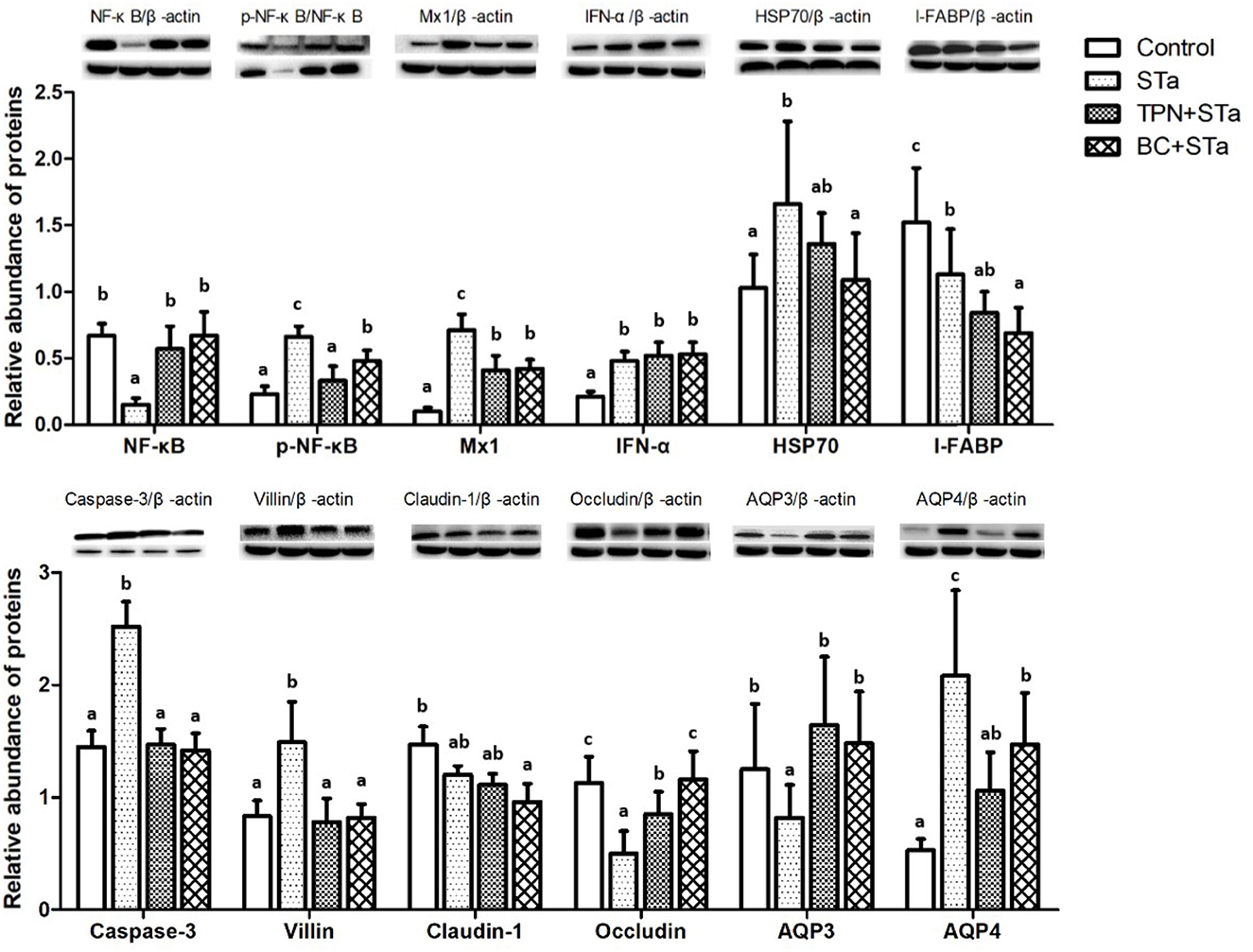
Figure 2. Effects of TPN and B. coagulans supplementation on the expression of proteins associated with intestinal barrier, immunity and metabolism in the jejunum of ETEC infected weaned piglets. Bars not sharing a common lowercase letter differ significantly (p < 0.05).
4. Discussion
Diarrhea in piglets is one of the most challenging problems in the pig industry, which causes a huge economic loss nowadays (18). A major finding of this study is that diarrhea rate was markedly decreased by both α-TPN and B. coagulans supplementation, indicating that α-TPN and B. coagulans supplementation could effectively alleviate diarrhea in the weaned piglets. Furthermore, diarrhea rate in the TPN+STa group was lower than that in the BC+STa groups, suggesting that supplementation of α-TPN had a greater impact on alleviating diarrhea in the piglets infected with ETEC. The anti-diarrheal activity of α-TPN was also observed in mice, and this activity may due to its ability to block PGE2 and GM1 receptors (11). Further studies are needed to verify its anticholinergic action in piglets.
Intestinal morphology indexes such as villus height, surface area, crypt depth, and the ratio of villus height to crypt depth are the common indicators of intestinal morphologic development and intestinal morphological integrity (16). Usually, the increases in villus height, villus surface area, as well as the ratio of villus height to crypt depth reflect the improvement of intestinal absorption capacity and intestinal health (19). In this study, STa group decreased the height, width and surface area of villus and the ratio of villus height to crypt depth in the duodenum, jejunum and ileum, increased the crypt depth in the ileum, reflecting that ETEC infection markedly damaged intestinal integrity. However, both TPN+STa and BC+STa group increased villus height, width and surface area as well as the ratio of villus height to crypt depth, indicating that supplementation of α-TPN and B. coagulans can repair the intestinal injury caused by ETEC infection and enhance intestinal integrity. This was further supported by the finding that lower plasma I-FABP concentration was detected in BC+STa and TPN+ STa groups compared to STa group. As I-FABP leakages into the circulation from enterocytes only when intestinal mucosal damage occurs (20). Moreover, the improvement of intestinal morphology would partially explain the alleviation of diarrhea by α-TPN and B. coagulans.
Activation of the host immune system in response to pathogen infection results in the production of many cytokines that act as mediators of disease activity (21). In particular, proinflammatory cytokines, such as IL-1β, and TNF-α appear to play important roles in the pathophysiology of inflammatory diseases (22). In this study, STa group increased plasma TNF-α and IL-1β concentration, indicating that ETEC infection had obviously proinflammatory effect on piglets. Additionally, compared to STa group, TPN+STa group decreased the concentration of TNF-α and IL-1β; BC+STa group significantly decreased the concentration of IL-1β. These results suggested that both α-TPN and B. coagulans supplementation could relieve inflammation induced by ETEC infection and had anti-inflammatory properties.
Oxidant stress reflects the unbalance between the systematic phenomenon of reactive oxygen species and the capacity of biosystem to readily detoxicate the reactive intermediaries or to renovate the resulting injury (23). Nevertheless, cells protect themselves from hydroxyl radicals and other oxygenates by antioxidant enzymes, such as SOD and GSH-Px (24). MDA can induce noxious stress in cells and constitute homopolar protein adducts known as advanced lipoxidation end-products (ALEs), which is usually utilized as a marker to evaluate the oxidant stress levels (25). In the present study, STa group significantly increased MDA content and decreased GSH-Px activity in plasma, suggesting that ETEC infection could reduce the anti-oxidative capacity of piglets and induce oxidative stress. However, compared with STa group, both the TPN+STa and BC+STa group decreased the content of MDA and increased GSH-Px activity in plasma. These results indicated that α-TPN and B. coagulans supplementation could alleviant oxidative stress caused by ETEC infection, and supplementation of α-TPN and B. coagulans could enhance anti-oxidative capacity.
The injury and slow growth as well as the barrier function reducing of intestinal mucosa were probably associated with some genes such as villin, I-FABP, Occludin, and MMP3. Villin is one kind of actin binding protein and a marker of villus cell differentiation, which conduce to prop up the microfilaments of the microvilli of the mucosal villus (26). Occludin integrates such diverse processes as gene transcription, tumor suppression, and cell proliferation to modulate the intestinal mucosal structure and function (27). MMP3 as well as their inhibitors play a crucial role in the maintenance of extracorpuscular matrix homeostasis, which is expressed at high levels in the intestine of clinical IBD and celiac diseases (28). In response to stress, HSP70 is expressed at elevated levels to promote refolding and prevent aggregation of partially denatured proteins, thereby, protecting cells from injury (29). Results of this study showed that STa group decreased expression level of gene MMP3 and protein Occludin, increased expression levels of gene villin and I-FABP and protein HSP70 and I-FABP. Whereas, compared with STa group, the TPN+STa and BC+STa group increased expression level of protein Occludin, which is beneficial for the repairment of intestinal barrier. The BC+STa group decreased expression level of protein HSP70, indicating that B. coagulans supplementation could promote the recovery from ETEC infection.
Caspase-3 is one of the key components of the apoptotic pathway in the small intestine, this protein is either partially or totally responsible for the proteolytic cleavage of many key proteins (30). The expression of caspase-3 was increased by ETEC infection whereas decreased by α-TPN and B. coagulans supplementation, which might contribute to enterocyte proliferation.
NF-κB is involved in activation of an exceptionally large number of genes in response to infections, inflammation, and other stressful situations requiring rapid reprogramming of gene expression (31). NF-κB is normally sequestered in the cytoplasm of non-stimulated cells and consequently must be translocated into the nucleus to stimulate gene transcription. The subcellular location of NF-κB is controlled by a family of inhibitory proteins, IκBs, which bind NF-κB and mask its nuclear localization signal, thereby preventing nuclear uptake (32). Exposure of cells to a variety of extracellular stimuli leads to the rapid phosphorylation, ubiquitination, and ultimately proteolytic degradation of IκB, which frees NF-κB to translocate to the nucleus where it regulates gene transcription (33). Mx proteins form a family of interferon (IFN)-induced GTPases with potent antiviral activity against various single-stranded RNA viruses in mammals (34). In the present study, STa group decreased expression level of NF-κB, increased expression levels of Mx1 and p-NF-κB, whereas both the TPN+STa and BC+STa group increased expression level of NF-κB, decreased expression level of Mx1 and p-NF-κB. The decline of p-NF-κB expression was in accordance with the decrease of TNF-α and IL-1β concentration in plasma. Together, these results demonstrated that ETEC infection activated the immune response in the piglets, while α-TPN and B. coagulans supplementation could alleviate the immune stress in the piglets during ETEC infection.
AQP4 and AQP10 are two of the most important water channel proteins and mainly distributed in intestinal epithelial cells and facilitated the transport of water across epithelial cell (35). KCNJ13 is an ATP-dependent calcium channel that transports calcium out of cells, which plays a very considerable role in calcium homeostasis (36). b0,+AT plays a role in the high-affinity and sodium-independent transport of cystine and neutral and dibasic amino acids and appears to function in the reabsorption of cystine in the kidney tubule (37). Increased level of genes b0,+AT, AQP10, KCNJ13, and protein AQP4 were observed in the STa group, indicated that ETEC infection could increase intestinal transportation, which could be ascribed to the damage of intestinal barrier and may the mechanism of diarrhea. Additionally, α-TPN and B. coagulans supplementation alleviated the intestinal transport dysfunction in the piglets during ETEC infection, as presented by decreased level of gene b0,+AT in the TPN+STa group and gene AQP10 in the BC+STa group, as well as protein AQP4 in both groups.
INSR is a transmembrane receptor which can binds insulin, IGF-I, and IGF-II and belongs to the large class of tyrosine kinase receptors. Binding of insulin or other ligands to this receptor activates the insulin signaling pathway, which regulates glucose uptake and release, as well as the synthesis and storage of carbohydrates, lipids and protein (38). PCK1 is a main control point for the regulation of gluconeogenesis and can be regulated by insulin, glucocorticoids, glucagon, cAMP, and diet (39). LPL is expressed in heart, muscle, and adipose tissue, which functions as both triglyceride hydrolase and ligand/bridging factor of receptor-mediated lipoprotein uptake (40). This study observed that STa group decreased expression level of LPL, increased expression levels of INSR and PCK1; whereas both the TPN+STa and BC+STa group decreased the level of INSR and PCK1 and the TPN+STa group increased expression level of LPL. The opposite results on metabolic regulation between ETEC and two supplements indicated the beneficial impact of α-TPN and B. coagulans on intestinal metabolism in piglets during ETEC infection.
5. Conclusion
The present study investigated the beneficial impact of dietary supplementation of α-TPN and B. coagulans in piglets infected with ETEC. Both α-TPN and B. coagulans supplementation could relieve diarrhea, inflammation, intestinal injury, oxidative and immune stress caused by ETEC infection, by means of modulation of genes and proteins associated with intestinal barrier, immune response, transport and metabolism. Findings of this study demonstrated that both α-TPN and B. coagulans can be used against ETEC infection, and could be considered as ideal alternatives to antibiotics.
Data availability statement
The original contributions presented in the study are included in the article/supplementary material, further inquiries can be directed to the corresponding author.
Ethics statement
The animal study was reviewed and approved by Animal Care and Use Committee at Wuhan Polytechnic University.
Author contributions
YH contributed to conception and design of the study. TW and QZ conducted the experiments and wrote the draft of the manuscript. HX and PL assisted the animal experiment. DZ and LW performed the statistical analysis. YH and DY revised the manuscript. All authors read and approved the submitted version.
Funding
This work was jointly supported by Hubei Provincial Key R&D Program (2021BBA083), Wuhan Knowledge Innovation Project (2022020801010392), and National Natural Science Foundation of China (32172763, 32072762).
Acknowledgments
We thank our students and technicians for their contributions to this research.
Conflict of interest
The authors declare that the research was conducted in the absence of any commercial or financial relationships that could be construed as a potential conflict of interest.
Publisher's note
All claims expressed in this article are solely those of the authors and do not necessarily represent those of their affiliated organizations, or those of the publisher, the editors and the reviewers. Any product that may be evaluated in this article, or claim that may be made by its manufacturer, is not guaranteed or endorsed by the publisher.
Abbreviations
ETEC, Enterotoxigenic Escherichia coli; STa, heat-stable toxin A; α-TPN, α-Terpineol; B. coagulans, Bacillus coagulans; SOD, superoxidase dismutase; GSH-Px, glutathione peroxidase; MDA, malondialdehyde; TNF-α, tumor necrosis factor alpha; IL-1β, interleukin 1 beta; I-FABP, intestinal fatty-acid binding protein; ADG, average daily gain; ADFI, average daily feed intake; DR: diarrhea rate; MMP3, matrix metalloproteinase-3; LPL, Lipoprotein lipase; b0,+AT, (b0,+ amino acid transporter); AQP10, aquaporin 10; INSR, insulin receptor; PCK1, phosphoenolpyruvate carboxykinase 1.
References
1. Zhu C, Ye JL, Yang J, Yang KM, Chen Z, Liang R, et al. Differential expression of intestinal ion transporters and water channel aquaporins in young piglets challenged with enterotoxigenic Escherichia coli K88. J Anim Sci. (2017) 95:5240–52. doi: 10.2527/jas2017.1806
2. Amcheslavsky A, Wallace AL, Ejemel M, Li Q, McMahon CT, Stoppato M, et al. Anti-CfaE nanobodies provide broad cross-protection against major pathogenic enterotoxigenic Escherichia coli strains, with implications for vaccine design. Sci Rep. (2021) 11:2751. doi: 10.1038/s41598-021-81895-0
3. Melkebeek V, Goddeeris BM, Cox E, ETEC. vaccination in pigs. Vet Immunol Immunopathol. (2013) 152:37–42. doi: 10.1016/j.vetimm.2012.09.024
4. Rocha LB, Ozaki CY, Horton DS, Menezes CA, Silva A, Fernandes I, et al. Different assay conditions for detecting the production and release of heat-labile and heat-stable toxins in enterotoxigenic Escherichia coli isolates. Toxins (Basel). (2013) 5:2384–402. doi: 10.3390/toxins5122384
5. Wu T, Lv Y, Li X, Zhao D, Yi D, Wang L, et al. Establishment of a recombinant Escherichia coli-induced piglet diarrhea model. Front Biosci. (2018) 23:1517–34. doi: 10.2741/4658
6. Lv Y, Li X, Zhang L, Shi Y, DU L, Ding B, et al. Injury and mechanism of recombinant E. coli expressing STa on piglets colon. J Vet Med Sci. (2018) 80:205–12. doi: 10.1292/jvms.17-0528
7. Hu YJ, Cowling BJ. Reducing antibiotic use in livestock, China. Bull World Health Organ. (2020) 98:360–1. doi: 10.2471/BLT.19.243501
8. Nazzaro F, Fratianni F, De Martino L, Coppola R, De Feo V. Effect of essential oils on pathogenic bacteria. Pharmaceuticals. (2013) 6:1451–74. doi: 10.3390/ph6121451
9. de Oliveira MG, Marques RB, de Santana MF, Santos AB, Brito FA, Barreto EO, et al. α-terpineol reduces mechanical hypernociception and inflammatory response. Basic Clin Pharmacol Toxicol. (2012) 111:120–5. doi: 10.1111/j.1742-7843.2012.00875.x
10. De Sousa DP, Quintans-Júnior LJ., de Almeida RN. Evolution of the anticonvulsant activity of α-terpineol. Pharm Biol. (2007) 45:69–70. doi: 10.1080/13880200601028388
11. Dos Santos Negreiros P, da Costa DS, da Silva VG, de Carvalho Lima IB, Nunes DB, de Melo Sousa FB, et al. Antidiarrheal activity of α-terpineol in mice. Biomed Pharmacother. (2019) 110:631–40. doi: 10.1016/j.biopha.2018.11.131
12. Payot T, Chemaly Z, Fick, M. Lactic acid production by Bacillus coagulans-Kinetic studies and optimization of culture medium for batch and continuous fermentations. Enzyme Microb Technol. (1999) 24:191–9. doi: 10.1016/S0141-0229(98)00098-2
13. Hung AT, Lin SY, Yang TY. Effects of Bacillus coagulans ATCC 7050 on growth performance, intestinal morphology, and microflora composition in broiler chickens. Animal Produ Sci. (2012) 52:874–9. doi: 10.1071/AN11332
14. Ripamonti B, Agazzi A, Baldi A, Balzaretti C, Bersani C, Pirani S, et al. Administration of Bacillus coagulans in calves: recovery from fecal samples and evaluation of functional aspects of spores. Vet Res Commun. (2009) 33:991–1001. doi: 10.1007/s11259-009-9318-0
15. Wu T, Zhang Y, Lv Y, Li P, Yi D, Wang L, et al. Beneficial Impact and Molecular Mechanism of Bacillus coagulans on Piglets' Intestine. Int J Mol Sci. (2018) 19:2084. doi: 10.3390/ijms19072084
16. Wu T, Li K, Yi D, Wang L, Zhao D, Lv Y, et al. Dietary Supplementation with Trihexanoin Enhances Intestinal Function of Weaned Piglets. Int J Mol Sci. (2018) 19:3277. doi: 10.3390/ijms19103277
17. Yi D, Hou Y, Wang L, Zhao D, Ding B, Wu T, et al. Gene expression profiles in the intestine of lipopolysaccharide-challenged piglets. Front Biosci. (2016) 21:487–501. doi: 10.2741/4404
18. Bhandari SK, Xu B, Nyachoti CM, Giesting DW, Krause DO. Evaluation of alternatives to antibiotics using an Escherichia coli K88+ model of piglet diarrhea: effects on gut microbial ecology. J Anim Sci. (2008) 86:836–47. doi: 10.2527/jas.2006-822
19. Hou Y, Wang L, Zhang W, Yang Z, Ding B, Zhu H, et al. Protective effects of N-acetylcysteine on intestinal functions of piglets challenged with lipopolysaccharide. Amino Acids. (2012) 43:1233–42. doi: 10.1007/s00726-011-1191-9
20. Lau E, Marques C, Pestana D, Santoalha M, Carvalho D, Freitas P, et al. The role of I-FABP as a biomarker of intestinal barrier dysfunction driven by gut microbiota changes in obesity. Nutr Metab. (2016) 13:31. doi: 10.1186/s12986-016-0089-7
21. Sekiyama KD, Yoshiba M, Thomson AW. Circulating proinflammatory cytokines. (IL-1 beta, TNF-alpha, and IL-6) and IL-1 receptor antagonist (IL-1Ra) in fulminant hepatic failure and acute hepatitis. Clin Exp Immunol. (1994) 98:71–7. doi: 10.1111/j.1365-2249.1994.tb06609.x
23. Nita M, Grzybowski A. The role of the reactive oxygen species and oxidative stress in the pathomechanism of the age-related ocular diseases and other pathologies of the anterior and posterior eye segments in adults. Oxid Med Cell Longev. (2016) 2016:3164734. doi: 10.1155/2016/3164734
24. Birben E, Sahiner UM, Sackesen C, Erzurum S, Kalayci O. Oxidative stress and antioxidant defense. World Allergy Organ J. (2012) 5:9–19. doi: 10.1097/WOX.0b013e3182439613
25. Del Rio D, Stewart AJ, Pellegrini N. A review of recent studies on malondialdehyde as toxic molecule and biological marker of oxidative stress. Nutr Metab Cardiovasc Dis. (2005) 15:316–28. doi: 10.1016/j.numecd
26. Chantret I, Barbat A, Dussaulx E, Brattain MG, Zweibaum A. Epithelial polarity, villin expression, and enterocytic differentiation of cultured human colon carcinoma cells: a survey of twenty cell lines. Cancer Res. (1988) 48:1936–42.
27. Schneeberger EE, Lynch RD. The tight junction: a multifunctional complex. Am J Physiol Cell Physiol. (2004) 286:C1213–28. doi: 10.1152/ajpcell.00558.2003
28. Bhuvarahamurthy V, Kristiansen GO, Johannsen M, Loening SA, Schnorr D, Jung K, et al. In situ gene expression and localization of metalloproteinases MMP1, MMP2, MMP3, MMP9, and their inhibitors TIMP1 and TIMP2 in human renal cell carcinoma. Oncol Rep. (2006) 15:1379–84. doi: 10.3892/or.15.5.1379
29. Buchmeier NA, Heffron F. Induction of Salmonella stress proteins upon infection of macrophages. Science. (1990) 248:730–2. doi: 10.1126/science.1970672
30. Wang Q, Hou Y, Yi D, Wang L, Ding B, Chen X, et al. Protective effects of N-acetylcysteine on acetic acid-induced colitis in a porcine model. BMC Gastroenterol. (2013) 13:133. doi: 10.1186/1471-230X-13-133
31. Sen R, Baltimore D. Inducibility of kappa immunoglobulin enhancer-binding protein Nf-kappa B by a posttranslational mechanism. Cell. (1986) 47:921–8. doi: 10.1016/0092-8674(86)90807-x
32. Baldwin AS Jr. The NF-kappa B and I kappa B proteins: new discoveries and insights. Annu Rev Immunol. (1996) 14:649–83. doi: 10.1146/annurev.immunol
33. Karin M, Ben-Neriah Y. Phosphorylation meets ubiquitination: the control of NF-[kappa]B activity. Annu Rev Immunol. (2000) 18:621–63. doi: 10.1146/annurev.immunol.18.1.621
34. Arnheiter H, Frese M, Kambadur R, Meier E, Haller O. Mx transgenic mice–animal models of health. Curr Top Microbiol Immunol. (1996) 206:119–47. doi: 10.1007/978-3-642-85208-4_8
35. Matsuzaki T, Tajika Y, Ablimit A, Aoki T, Hagiwara H, Takata K. Aquaporins in the digestive system. Med Electron Microsc. (2004) 37:71–80. doi: 10.1007/s00795-004-0246-3
36. Hejtmancik JF, Jiao X, Li A, Sergeev YV, Ding X, Sharma AK, et al. Mutations in KCNJ13 cause autosomal-dominant snowflake vitreoretinal degeneration. Am J Hum Genet. (2008) 82:174–80. doi: 10.1016/j.ajhg.2007.08.002
37. Romeo E, Dave MH, Bacic D, Ristic Z, Camargo SM, Loffing J, et al. Luminal kidney and intestine SLC6 amino acid transporters of B0AT-cluster and their tissue distribution in Mus musculus. Am J Physiol Renal Physiol. (2006) 290:F376–83. doi: 10.1152/ajprenal.00286.2005
38. Massoner P, Ladurner-Rennau M, Eder IE, Klocker H. Insulin-like growth factors and insulin control a multifunctional signaling network of significant importance in cancer. Br J Cancer. (2010) 103:1479–84. doi: 10.1038/sj.bjc.6605932
39. Sato M, Tokuji Y, Yoneyama S, Fujii-Akiyama K, Kinoshita M, Ohnishi M. Profiling of hepatic gene expression of mice fed with edible japanese mushrooms by DNA microarray analysis: comparison among Pleurotus ostreatus, Grifola frondosa, and Hypsizigus marmoreus. J Agric Food Chem. (2011) 59:10723–31. doi: 10.1021/jf2025659
Keywords: B. coagulans, α-terpineol, Enterotoxigenic Escherichia coli, intestinal function, antibiotic alternatives, weaned piglets
Citation: Wu T, Zhang Q, Xu H, Li P, Zhao D, Wang L, Yi D and Hou Y (2023) Protective effects of α-terpineol and Bacillus coagulans on intestinal function in weaned piglets infected with a recombinant Escherichia coli expressing heat-stable enterotoxin STa. Front. Vet. Sci. 10:1118957. doi: 10.3389/fvets.2023.1118957
Received: 09 December 2022; Accepted: 16 January 2023;
Published: 10 February 2023.
Edited by:
Mian Muhammad Awais, Bahauddin Zakariya University, PakistanCopyright © 2023 Wu, Zhang, Xu, Li, Zhao, Wang, Yi and Hou. This is an open-access article distributed under the terms of the Creative Commons Attribution License (CC BY). The use, distribution or reproduction in other forums is permitted, provided the original author(s) and the copyright owner(s) are credited and that the original publication in this journal is cited, in accordance with accepted academic practice. No use, distribution or reproduction is permitted which does not comply with these terms.
*Correspondence: Yongqing Hou, houyq@aliyun.com
†These authors have contributed equally to this work