- Faculty of Veterinary Medicine, University of Ljubljana, Ljubljana, Slovenia
Regenerative medicine has opened the door to the exploration of new therapeutic methods for the treatment of various diseases, especially those associated with local or general disregulation of the immune system. In pulmonary diseases, new therapeutic strategies have emerged that are aimed at restoring functional lung tissue rather than alleviating symptoms. These strategies focus on tissue regeneration using stem cells and/or their derivatives or replacement of dysfunctional tissue using biomedical engineering. Animal health can directly benefit from regenerative therapy strategies and also serve as a translational experimental model for human disease. Several clinical trials have been conducted to evaluate the effects of cellular treatment on inflammatory lung disease in animals. Data reported to date show several beneficial effects in ex vivo and in vivo models; however, our understanding of the mechanisms that regenerative therapies exert on diseased tissues remains incomplete.
Introduction
Several chronic respiratory diseases in humans and animals remain incurable. Treatments have been relatively successful in relieving some symptoms, but they all ultimately lead to a poorer quality of life and are one of the leading causes of death worldwide (1). Intense and persistent inflammation leads to loss of functional tissue and pulmonary tissue remodeling, which in turn leads to loss of respiratory function. Over time, lung tissue changes are so severe that euthanasia is required in animals or lung transplantation is the only viable option to prolong life in humans (2–9).
The lung has an exceptional ability to respond and regenerate after the tissue injury (7, 9, 10). However, regeneration of lung tissue can often lead to pathological tissue remodeling and subsequent impairment of lung function (10). These changes in the lung can potentially be reversed through regenerative medicine in the form of cellular therapy, extracellular vesicle therapy (ECV), or even tissue engineering (4, 5, 7, 9–12). Several animal studies addressed regenerative therapeutic modalities in the lung in experimental models (13–38) and in clinical trials (39–41) (Table 1).
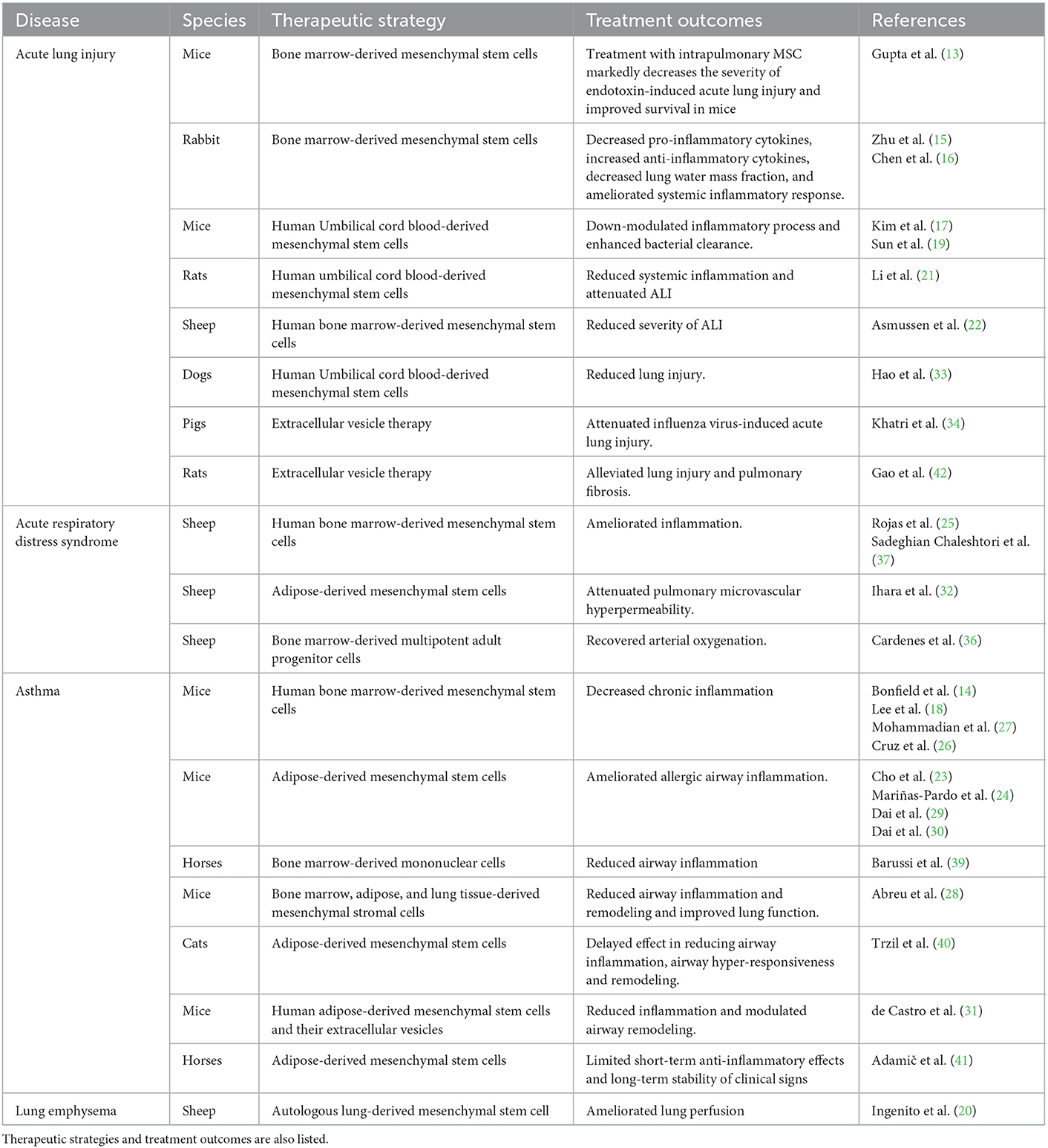
Table 1. List of selected references reporting the use of regenerative treatments for respiratory diseases in animals.
Although inflammation is the reason for damage to the airways, it is also critical for initiating tissue regeneration and restoration. Inflammatory cells flooding the airways are important for phagocytosis and for stimulating resident progenitor cells through secreted cytokines and growth factors. Some resident cell populations do not appear to exist in a healthy lung, but emerge only in response to lung injury. A more detailed knowledge of this relationship will likely enable new therapeutic options to stimulate lung regeneration and self-repair (43).
Data sources and searches
An online literature search was performed using the PubMed® (U.S. National Library of Medicine and National Institutes of Health) search engine (https://pubmed.ncbi.nlm.nih.gov/), evaluating reports from January 1, 1990, to October 31, 2022. Reference lists of relevant articles were also reviewed to find additional studies.
Cellular therapy
Regenerative cell therapy is currently the most widely used method for stimulating the regeneration of damaged tissue, in which stem cells (SC) play a leading role. Stem cells are undifferentiated cells capable of self-renewal and transformation into other cell types (44). Traditionally, the therapeutic effect of SC has been associated with their migration to the affected area and their ability to replace damaged tissue (45). However, later discoveries recognized their complex immunomodulatory role through interaction with local cells of the immune system and paracrine signaling (46, 47). Currently, two ways of their potential use for therapeutic purposes are being investigated: (1) induction of endogenous differentiation and mobilization of resident progenitor cells and (2) ex vivo (exogenous) cultivation of SC and their application in patients (4, 11, 48, 49). The former is mainly related to tissue regeneration and repair through activation of resident cells (43, 48), while the latter is mainly associated with paracrine action and immunomodulatory effects (4).
Heterogeneous endogenous stem cells [cells capable of long-term self-renewal and differentiation into other progenitor cells or tissue-specific cells (4)] and progenitor cells [tissue-specific cells capable of differentiation into specific cell types, but are not capable of self-renewal or are capable of self-renewal only in the relatively short term (4)] of the lung, located in different regions of the airway, are capable of self-renewal and of forming one or more mature cell types, allowing local maintenance of epithelial integrity and repair of damage (4, 10, 50). They reside in their unique microenvironmental niches that allow them to maintain their progenitor properties and differentiate into different cell types (10). Several distinct populations of stem and progenitor cells are present in the airways, which can differentiate into different airway cell types (4, 10, 50).
Basal epithelial cells represent a population of stem/progenitor cells from which Club cells (formerly known as Clara cells) and ciliated cells can develop (51, 52). They may also serve as progenitors for multiciliated and goblet cells (10). Submucosal glandular progenitor cells are another group of cells capable of regenerating submucosal glandular tubules, ducts, and surface epithelium (10, 53). Neuroendocrine cells of the lung can also function as progenitor cells that differentiate into Club cells and ciliated cells upon injury (10, 54). Type 2 alveolar cells are critical for surfactant C production and secretion, but are also considered alveolar progenitor cells. They can self-renew and/or differentiate into type 1 alveolar cells, which are responsible for gas exchange (10). Differentiation, proliferation and expansion of type 2 alveolar cells after tissue injury is protracted and takes several months (50).
Resident stem cells, which are thought to share several properties with bone marrow-derived mesenchymal stem cells (BM-MSC), have also been found in the lung (20, 55–57). They are currently referred to as lung mesenchymal stem cells or lung mesenchymal stromal cells (L-MSC). Their potential physiological or pathophysiological functions are not yet known. Similar to BM-MSC, L-MSC secrete immunosuppressive molecules and therefore may influence the course of inflammation, tissue injury and repair (58, 59).
Attempts have also been made to derive the phenotype of structural lung cells for pulmonary vascular regeneration from adipose or bone marrow tissue or from embryonic SC. Despite the ability of SC to differentiate into lung cell types, results of such studies remain controversial because of inadequately derived or described methods (4).
The therapeutic potential of exogenous SC has been repeatedly noted in relation to their immunomodulatory effects. Their complex immunomodulatory role results from their interaction with local immune cells and paracrine signaling, leading to a reduction in proinflammatory stimulus and thus less tissue damage (46, 47, 60–63). Most research on SC therapies has focused on inflammatory airway diseases where conventional treatments have been unsuccessful, and they have been found to have several beneficial effects (36, 64–67). Various cell sources (e.g., BM-MSC, adipose-derived stem cells, embryonic stem cells, umbilical cord blood-derived mesenchymal stem cells), dosages, and delivery methods have been investigated to maximize the potential of their therapeutic use. However, there is not yet sufficient evidence to formulate precise guidelines for clinical use.
Tissue engineering
Pathologic changes in diseased lungs may progress to the point where cell therapy and stimulation of tissue regeneration alone are insufficient and tissue replacement is required to restore lung function. Suitable lung donors are not always available, or lung transplantation is contraindicated (68); therefore, in vitro-grown tissue may bridge the time to lung transplantation or serve as a definitive therapeutic modality.
Tissue engineering techniques are still insufficiently developed. The lung is composed of more than 40 different cell types that form a complex three-dimensional (3D) anatomic architecture (69). Generating lungs in vitro and mimicking their function is a major challenge that requires a high degree of cell specialization and complex tissue architecture (5, 48, 70, 71). They must provide a variety of organ functions, such as the diversity of airway cell types, the defense mechanisms that protect the upper airways (e.g., secretion of specifically composed mucus and active ciliary apparatus), and the coupling of the alveolar space with the surrounding systemic and pulmonary vasculature to ensure effective tissue perfusion and gas exchange (72).
Most preclinical studies have used biologically derived models or synthetic scaffolds seeded with an appropriate cell source to regenerate functional lung tissue (5, 7). Hybrid scaffolds combining biological materials (extracellular matrix (ECM) components) with synthetic scaffolds currently appear to have the greatest potential. These scaffolds are then seeded with autologous or allogeneic cells to generate functional tissue generation (5). An important advantage of using allogenic cells is the reduction of immunologic complications and tissue rejection (7, 12, 48). In this way, a miniaturized and simplified version of an organ can be produced in the laboratory, called an organoid. This is a 3D structure that replicates the microanatomy of the desired organ. The formation of organoids relies on the self-assembly of cells derived from adult tissues, embryonic stem cells, or induced pluripotent stem cells (70, 71, 73, 74).
Because they represent the overall architecture of the lung, organoids are important models for studying various physiological processes in the airway microenvironment and the effects of various effectors on airway tissue structure, including infectious agents and/or new therapeutic modalities. This is particularly important because the cellular and molecular response to chemical and physical signals in vivo and the properties of gene expression can be obscured or lost in more commonly used in vitro 2D cell culture systems (73, 74). Lung organoids are broadly divided into proximal lung organoids (containing cells that mimic the conducting airways), distal lung organoids (subsuming the alveoli), or proximal-distal organoids (74).
The creation of a functional epithelial tissue appropriately connected to the vascular component is particularly important for the future development of therapeutically beneficial engineered pulmonary tissues. A more ambitious model of tissue engineering is based on decellularization of the original organ, in which all cells and cellular materials are removed from the entire lung, resulting in an intact three-dimensional scaffold. This represents the innate ECM, preserving the natural structure of the airways and blood vessels, providing an optimal platform for transplantation of lung cells (48, 74). Lung ECM (collagen and elastic fibers enriched in proteoglycans, glycosaminoglycans, and fibronectin) not only provides a sophisticated scaffold for potential lung organogenesis, but also combines biochemical and mechanical signals that further guide SC behavior during lung re-development and regeneration (74). To generate functional lung tissue ex vivo, one would need to define more than 40 different cell types and perhaps hundreds to thousands of different cell subtypes (5).
Nichols et al. (35) transplanted a bioengineered porcine lung, which was generated using autologous cells. The bioengineered lungs successfully formed alveolar tissue and were ventilated, well vascularized, and developed a microbiome similar to that of the natural lung. The authors also noted no evidence of graft rejection (35). However, Yanagiya et al. (38) reported marked bullous changes in the transplanted tissue of bioengineered lungs when they examined unilateral transplantation of porcine lungs generated from autologous cells. They also reported comparable oxygen exchange between the bioengineered lung transplant group and the allograft recipient group, whereas CO2 exchange was significantly lower in the bioengineered lung transplant group than in the allograft group (38).
Airway anatomy and physiology are highly species-dependent, making it necessary to create species-specific models. In a recent review of mammalian lung organoids, Archer et al. (72) highlighted that the cells lining the bronchiolar or more distal part of the tracheobronchial tree differ considerably between species in terms of their abundance, the cell types present, the ultrastructural features of these cells in adult animals, and the secretory products they produce (72). Mouse models, for example, are not particularly well suited for studying human respiratory diseases. On the other hand, sheep lungs are most commonly used as models for human lungs because of their anatomy and the uniform distribution of differentiated cells at a given age of maturity. These elements make sheep a valuable model for human respiratory physiology and disease (72).
Cell-free therapeutical strategies
Extracellular vesicles are membrane-protected carriers of many substances, including microRNA (miRNA), messenger RNA, proteins, and mitochondria. Extracellular vesicles are broadly classified into exosomes (vesicles of endocytotic origin with a diameter of 30–150 nm, surrounded by a plasma membrane), microvesicles (diameter of 100–1,000 nm, not of endocytotic origin), and apoptotic bodies [diameter of 50 nm−5 μm; they are released by apoptotic cells during membrane budding (blebbing)] (75). The use of ECV offers several important advantages over cell therapy. Due to their smaller size, ECV can penetrate deeper into the airways and potentially be delivered by inhalation techniques (76). In addition, their membrane envelope makes them stable in tissues and body fluids. They also have low immunogenicity and toxicity compared to cell therapies (77, 78). A major obstacle to the therapeutic use of ECV is the lack of standardized methods for isolation and purification of ECV. The lack of standardized methods for isolating exosomes means that exosomes cannot be separated from other ECV of similar size. There is also a lack of standardization of methods for measuring ECV purity (47, 79). In this context, it is advisable to use the generic term “extracellular vesicle” when using ECV therapeutically and to avoid nominal categorization into subtypes. If the name of a single subspecies is used, extraction and selection must be precisely defined.
Confirmation of the functionality of ECV therapy requires that the therapeutic effect occurs without intercellular contact and that this is not achieved by ECV-unrelated soluble paracrine factors (80). Extracellular vesicles are involved in several intercellular signaling pathways, making them critical molecular messengers in various processes responsible for normal homeostasis and disease development. In the regeneration process, they also influence the response of stem/progenitor cells and other cells within their niche (78).
Numerous studies have demonstrated the benefits of systemic administration of ECV in mitigating allergic airway hyperreactivity and resulting inflammation and tissue remodeling (26, 31). Extracellular vesicle treatment has been shown to be beneficial in the treatment of lung injury and pulmonary fibrosis in rats. After intratracheal administration, there was a reduction in apoptosis and necrosis of type 2 alveolar epithelial cells and alleviation of lung injury. Extracellular vesicles decreased reactive oxygen species levels and inflammation in the airways. The authors were able to attribute some of the beneficial effects to a specific miRNA, let-7d-5p (42). Antounians et al. (81) also attributed the therapeutic effects of ECV to miRNA when they investigated its influence on the regenerative capacity of undeveloped fetal lungs in an experimental rodent model. Following ECV treatment, enhanced morphogenesis and alveolarization, restoration of lung tissue homeostasis, and differentiation of epithelial cells and fibroblasts were observed in association with the release of RNA cargo (81). In addition, ECV treatment may limit viral respiratory infections by affecting viral replication and virus-induced apoptosis in lung epithelial cells, which is also thought to depend on the transfer of RNA from ECV to epithelial cells (34).
In addition to the cell-free regenerative medicine options described above, several indirect therapeutic options have been described to stimulate local cells and tissue regeneration in the airways. For example, all-trans-retinoic acid, a derivative of vitamin A (retinol), has been described as a possible candidate to promote alveologenesis (48, 82, 83). It is also suggested that nanoparticles of integrins may influence the regeneration of collapsed alveoli (84). Another area of research is regenerative photobiostimulation, which aims to stimulate resident stem cells with electromagnetic radiation to trigger growth factor production, inhibition of inflammation, and stimulation of angiogenesis (85).
Most probable therapeutic application in animals
Benefits of cell treatments have been reported for the treatment of asthma; experimentally in mouse models (14, 18, 23, 24, 26–31) and animals with natural asthma, such as cats (40) and horses (39, 41). The treatment effects of SC, identified in preclinical studies of asthma treatment, are related to the reduction of airway inflammation through the regulation of inflammatory cytokines. The results of these studies differ in terms of cytokine expression and translation, but all consistently reported a reduction in airway inflammation. The influence of SC on tissue remodeling may play the critical role in the treatment of asthma (18, 24, 26, 31, 86, 87).
Ingenito et al. (20) investigated the effect of autologous L-MSC on experimentally induced lung emphysema in sheep to evaluate their ability to regenerate functional tissue. Animals received endoscopically either cellularized biological scaffolds or scaffolds alone. At four-week follow-up, no immune response to the grafts was detected, but significant improvement in tissue mass (in terms of increased cellularity and extracellular matrix content) and lung perfusion was observed in sheep receiving L-MSC compared with the control group. Detection of labeled L-MSC in the alveolar septum and peribronchial interstitium was also reported. L-MSC therefore have the potential for regeneration of emphysematous lungs (20).
Treatment with SC also significantly affects inflammatory responses and lung tissue regeneration in acute lung injury (ALI) and acute respiratory distress syndrome (ARDS) (36, 88–91). Aside from symptomatic therapy, no specific treatment for these diseases have been defined that would substantially improve short- and long-term outcomes. Positive effects in terms of reducing pulmonary edema and inflammation and improving gas exchange have been reported after cell treatment in experimentally induced ARDS in sheep (22, 25, 32, 36, 37). Currently, research on the effect of SC on the treatment of ARDS caused by respiratory viruses is particularly relevant due to the COVID-19 pandemic (67, 90, 92). Reductions in oxidative stress and inflammation and resulting lung injury and mortality following treatment with SC have been observed in mice (13, 17, 19, 21), rabbits (15, 16) and dogs (33) with experimentally induced lung injury.
In addition to cell therapy, treatment with ECV has also successfully treated acute airway inflammation caused by viral infection. Khatri et al. (34) investigated the effects of intratracheally administered ECV on influenza virus-induced acute lung injury in pigs. ECV treatment significantly reduced viral secretion (detected in nasal swabs), viral replication in the lungs, and virus-induced inflammatory cytokine formation in the lungs of infected pigs 12 h after viral infection. The authors concluded that intratracheal treatment with ECV attenuates influenza virus-induced ALI in pigs (34).
Conclusions
Further evidence from appropriately designed clinical trials is needed before regenerative therapy is considered an accepted therapeutic modality in respiratory medicine. To date, the use of SC or ECV for the treatment of respiratory disease has consistently been described as relatively safe after local and systemic application. Apart from mild local reactions after administration of cells of allogeneic origin, no severe adverse events have been observed (41, 47, 93–96). The interaction between SC/ECV and the immune system may also provide better insight into the pathophysiology of immune system dysregulation in the respiratory system.
It is also important to focus on a detailed understanding of the functional heterogeneity of each cell type in the respiratory system and the development of protocols for targeted cell differentiation and maturation (70). This is particularly true for tissue engineering, which is less explored compared to SC and ECV due to its anatomical and functional complexity. The creation of a functional epithelial tissue suitably linked to the vascular component is particularly important for the future development of respiratory physiology and medicine.
Author contributions
All authors listed have made a substantial, direct, and intellectual contribution to the work and approved it for publication.
Funding
The authors acknowledge the financial support from the Slovenian Research Agency (P4-0053).
Conflict of interest
The authors declare that the research was conducted in the absence of any commercial or financial relationships that could be construed as a potential conflict of interest.
Publisher's note
All claims expressed in this article are solely those of the authors and do not necessarily represent those of their affiliated organizations, or those of the publisher, the editors and the reviewers. Any product that may be evaluated in this article, or claim that may be made by its manufacturer, is not guaranteed or endorsed by the publisher.
References
1. World Health Organization. Chronic Respiratory Diseases. (2022). Available online at: https://www.who.int/health-topics/chronic-respiratory-diseases#tab=tab_3 (accessed November 2, 2022).
2. Rhind S, Gunn-Moore D. Desquamative form of cryptogenic fibrosing alveolitis in a cat. J Comp Pathol. (2000) 123:226–9. doi: 10.1053/jcpa.2000.0412
3. Lavoie JP. “Recurrent airway obstruction (Heaves) and summer-pasture-associated obstructive pulmonary disease,” In: McGorum BC, Dixon PM, Robinson NE, Schumacher J, eds Equine Respiratory Medicine and Surgery. Edinburgh: W.B. Saunders (2007). p. 565–89. doi: 10.1016/B978-0-7020-2759-8.50046-5
4. Lau AN, Goodwin M, Kim CF, Weiss DJ. Stem cells and regenerative medicine in lung biology and diseases. Mol Ther. (2012) 20:1116–30. doi: 10.1038/mt.2012.37
5. De Santis MM, Bölükbas DA, Lindstedt S, Wagner DE. How to build a lung: latest advances and emerging themes in lung bioengineering. Eur Respir J. (2018) 52:1601355. doi: 10.1183/13993003.01355-2016
6. Boiron L, Hopper K, Borchers A. Risk factors, characteristics, and outcomes of acute respiratory distress syndrome in dogs and cats: 54 cases. J Vet Emerg Crit Care San Antonio. (2019) 29:173–9. doi: 10.1111/vec.12819
7. Melo-Narváez MC, Stegmayr J, Wagner DE, Lehmann M. Lung regeneration: implications of the diseased niche and ageing. Eur Respir Rev. (2020) 29:200222. doi: 10.1183/16000617.0222-2020
8. Trzil JE. Feline asthma: diagnostic and treatment update. Vet Clin North Am Small Anim Pract. (2020) 50:375–91. doi: 10.1016/j.cvsm.2019.10.002
9. Khedoe PPPSJ, Wu X, Gosens R, Hiemstra PS. Repairing damaged lungs using regenerative therapy. Curr Opin Pharmacol. (2021) 59:85–94. doi: 10.1016/j.coph.2021.05.002
10. Parekh KR, Nawroth J, Pai A, Busch S, Senger C, Ryan A. Stem cells and lung regeneration. Am J Physiol Cell Physiol. (2020) 319:C675–93. doi: 10.1152/ajpcell.00036.2020
11. Terzic A, Nelson TJ. Advancing regenerative medicine. Nat Med. (2014) 20:795. doi: 10.1038/nm.3658
12. Edgar L, Pu T, Porter B, Aziz JM, La Pointe C, Asthana A, et al. Regenerative medicine, organ bioengineering and transplantation. Br J Surg. (2020) 107:793–800. doi: 10.1002/bjs.11686
13. Gupta N, Su X, Popov B, Lee J, Serikov V, Matthay M. Intrapulmonary delivery of bone marrow-derived mesenchymal stem cells improves survival and attenuates endotoxin-induced acute lung injury in mice. J Immunol. (2007) 179:1855–63. doi: 10.4049/jimmunol.179.3.1855
14. Bonfield TL, Koloze M, Lennon DP, Zuchowski B, Yang SE, Caplan AI. Human mesenchymal stem cells suppress chronic airway inflammation in the murine ovalbumin asthma model. Am J Physiol Lung Cell Mol Physiol. (2010) 299:L760–70. doi: 10.1152/ajplung.00182.2009
15. Zhu F, Guo G, Chen W, Peng Y, Xing J, Wang N. Effect of bone marrow-derived mesenchymal stem cells transplantation on the inflammatory response and lung injury in rabbit with inhalation injury. Zhonghua Shao Shang Za Zhi. (2010) 26:360–5. Available online at: http://open.oriprobe.com/articles/25470091/Effect_of_bone_marrow_derived_mesenchymal_stem_cel.htm
16. Chen W, Zhu F, Guo G, Zhan J. Effect of bone marrow mesenchymal stem cells engraftment on secretion of inflammatory cytokine in the early stages of smoke inhalation injury in rabbits. Zhongguo Wei Zhong Bing Ji Jiu Yi Xue. (2011) 23:21–3.
17. Kim ES, Chang YS, Choi SJ, Kim JK, Yoo HS, Ahn SY, et al. Intratracheal transplantation of human umbilical cord blood-derived mesenchymal stem cells attenuates Escherichia coli-induced acute lung injury in mice. Respir Res. (2011) 12:108. doi: 10.1186/1465-9921-12-108
18. Lee SH, Jang AS, Kwon JH, Park SK, Won JH, Park CS. Mesenchymal stem cell transfer suppresses airway remodeling in a toluene diisocyanate-induced murine asthma model. Allergy Asthma Immunol Res. (2011) 3:205–11. doi: 10.4168/aair.2011.3.3.205
19. Sun J, Han ZB, Liao W, Yang SG, Yang Z, Yu J, et al. Intrapulmonary delivery of human umbilical cord mesenchymal stem cells attenuates acute lung injury by expanding CD4+CD25+ Forkhead Boxp3 (FOXP3)+ regulatory T-cells and balancing anti- and pro-inflammatory factors. Cell Physiol Biochem. (2011) 27:587–96. doi: 10.1159/000329980
20. Ingenito EP, Tsai L, Murthy S, Tyagi S, Mazan M, Hoffman A. Autologous lung-derived mesenchymal stem cell transplantation in experimental emphysema. Cell Transplant. (2012) 21:175–89. doi: 10.3727/096368910X550233
21. Li J, Li D, Liu X, Tang S, Wei F. Human umbilical cord mesenchymal stem cells reduce systemic inflammation and attenuate LPS-induced acute lung injury in rats. J Inflamm Lond. (2012) 9:33. doi: 10.1186/1476-9255-9-33
22. Asmussen S, Ito H, Traber DL, Lee JW, Cox RA, Hawkins HK, et al. Human mesenchymal stem cells reduce the severity of acute lung injury in a sheep model of bacterial pneumonia. Thorax. (2014) 69:819–25. doi: 10.1136/thoraxjnl-2013-204980
23. Cho KS, Park MK, Kang SA, Park HY, Hong SL, Park HK, et al. Adipose-derived stem cells ameliorate allergic airway inflammation by inducing regulatory T-cells in a mouse model of asthma. Mediators Inflamm. (2014) 2014:436476. doi: 10.1155/2014/436476
24. Mariñas-Pardo L, Mirones I, Amor-Carro O, Fraga-Iriso R, Lema-Costa B, Cubillo I, et al. Mesenchymal stem cells regulate airway contractile tissue remodeling in murine experimental asthma. Allergy. (2014) 69:730–40. doi: 10.1111/all.12392
25. Rojas M, Cárdenes N, Kocyildirim E, Tedrow JR, Cáceres E, Deans R, et al. Human adult bone marrow-derived stem cells decrease severity of lipopolysaccharide-induced acute respiratory distress syndrome in sheep. Stem Cell Res Ther. (2014) 5:42. doi: 10.1186/scrt430
26. Cruz FF, Borg ZD, Goodwin M, Sokocevic D, Wagner DE, Coffey A, et al. Systemic administration of human bone marrow-derived mesenchymal stromal cell extracellular vesicles ameliorates Aspergillus hyphal extract-induced allergic airway inflammation in immunocompetent mice. Stem Cells Transl Med. (2015) 4:1302–16. doi: 10.5966/sctm.2014-0280
27. Mohammadian M, Boskabady MH, Kashani IR, Jahromi GP, Omidi A, Nejad AK, et al. Effect of bone marrow derived mesenchymal stem cells on lung pathology and inflammation in ovalbumin-induced asthma in mouse. Iran J Basic Med Sci. (2016) 19:55–63. doi: 10.22038/IJBMS.2016.6415
28. Abreu SC, Antunes MA, Xisto DG, Cruz FF, Branco VC, Bandeira E, et al. Bone marrow, adipose, and lung tissue-derived murine mesenchymal stromal cells release different mediators and differentially affect airway and lung parenchyma in experimental asthma. Stem Cells Transl Med. (2017) 6:1557–67. doi: 10.1002/sctm.16-0398
29. Dai R, Liu J, Cai S, Zheng C, Zhou X. Delivery of adipose-derived mesenchymal stem cells attenuates airway responsiveness and inflammation in a mouse model of ovalbumin-induced asthma. Am J Transl Res. (2017) 9:2421–8. Available online at: https://e-century.us/web/journal_search.php?journal=ajtr&q=Delivery%20of%20adipose-derived%20mesenchymal%20stem%20cells%20attenuates%20airway%20responsiveness%20and%20inflammation%20in%20a%20mouse%20model%20of%20ovalbumin-induced%20asthma
30. Dai R, Yu Y, Yan G, Hou X, Ni Y, Shi G. Intratracheal administration of adipose derived mesenchymal stem cells alleviates chronic asthma in a mouse model. BMC Pulm Med. (2018) 18:131. doi: 10.1186/s12890-018-0701-x
31. de Castro LL, Xisto DG, Kitoko JZ, Cruz FF, Olsen PC, Redondo PAG, et al. Human adipose tissue mesenchymal stromal cells and their extracellular vesicles act differentially on lung mechanics and inflammation in experimental allergic asthma. Stem Cell Res Ther. (2017) 8:151. doi: 10.1186/s13287-017-0600-8
32. Ihara K, Fukuda S, Enkhtaivan B, Trujillo R, Perez-Bello D, Nelson C, et al. Adipose-derived stem cells attenuate pulmonary microvascular hyperpermeability after smoke inhalation. PLoS One. (2017) 12:e0185937. doi: 10.1371/journal.pone.0185937
33. Hao Y, Ran Y, Lu B, Li J, Zhang J, Feng C, et al. Therapeutic effects of human umbilical cord-derived mesenchymal stem cells on canine radiation-induced lung injury. Int J Radiat Oncol Biol Phys. (2018) 102:407–16. doi: 10.1016/j.ijrobp.2018.05.068
34. Khatri M, Richardson LA, Meulia T. Mesenchymal stem cell-derived extracellular vesicles attenuate influenza virus-induced acute lung injury in a pig model. Stem Cell Res Ther. (2018) 9:17. doi: 10.1186/s13287-018-0774-8
35. Nichols J, La Francesca S, Niles J, Vega S, Argueta L, Frank L, et al. Production and transplantation of bioengineered lung into a large-animal model. Sci Transl Med. (2018) 10:eaao3926. doi: 10.1126/scitranslmed.aao3926
36. Cardenes N, Aranda-Valderrama P, Carney JP, Sellares Torres J, Alvarez D, Kocydirim E, et al. Cell therapy for ARDS: efficacy of endobronchial vs. intravenous administration and biodistribution of MAPCs in a large animal model. BMJ Open Respir Res. (2019) 6:e000308. doi: 10.1136/bmjresp-2018-000308
37. Sadeghian Chaleshtori S, Mokhber Dezfouli MR, Abbasi J, Dehghan MM, Jabbari Fakhr M, Yadollahi S, et al. Prevention of LPS-induced acute respiratory distress syndrome in sheep by bone marrow-derived mesenchymal stem/stromal cells. Life Sci. (2020) 263:118600. doi: 10.1016/j.lfs.2020.118600
38. Yanagiya M, Kitano K, Yotsumoto T, Asahina H, Nagayama K, Nakajima J. Transplantation of bioengineered lungs created from recipient-derived cells into a large animal model. Semin Thorac Cardiovasc Surg. (2021) 33:263–71. doi: 10.1053/j.semtcvs.2020.03.005
39. Barussi FCM, Bastos FZ, Leite LMB, Fragoso FYI, Senegaglia AC, Brofman PRS, et al. Intratracheal therapy with autologous bone marrow-derived mononuclear cells reduces airway inflammation in horses with recurrent airway obstruction. Respir Physiol Neurobiol. (2016) 232:35–42. doi: 10.1016/j.resp.2016.07.002
40. Trzil JE, Masseau I, Webb TL, Chang CH, Dodam JR, Liu H, et al. Intravenous adipose-derived mesenchymal stem cell therapy for the treatment of feline asthma: a pilot study. J Feline Med Surg. (2016) 18:981–90. doi: 10.1177/1098612X15604351
41. Adamič N, Prpar Mihevc S, Blagus R, Kramarič P, Krapež U, Majdič G, et al. Effect of intrabronchial administration of autologous adipose-derived mesenchymal stem cells on severe equine asthma. Stem Cell Res Ther. (2022) 13:23. doi: 10.1186/s13287-022-02704-7
42. Gao Y, Sun J, Dong C, Zhao M, Hu Y. Jin F. Extracellular vesicles derived from adipose mesenchymal stem cells alleviate PM25-induced lung injury and pulmonary fibrosis. Med Sci Monit. (2020) 26:e922782. doi: 10.12659/MSM.922782
43. Yamada M, Fujino N, Ichinose M. Inflammatory responses in the initiation of lung repair and regeneration: their role in stimulating lung resident stem cells. Inflamm Regen. (2016) 36:15. doi: 10.1186/s41232-016-0020-7
44. Morrison SJ, Wandycz AM, Hemmati HD, Wright DE, Weissman IL. Identification of a lineage of multipotent hematopoietic progenitors. Development. (1997) 124:1929–39. doi: 10.1242/dev.124.10.1929
46. Fu X, Liu G, Halim A, Ju Y, Luo Q, Song AG. Mesenchymal stem cell migration and tissue repair. Cells. (2019) 8:784. doi: 10.3390/cells8080784
47. Voga M, Adamic N, Vengust M, Majdic G. Stem cells in veterinary medicine—current state and treatment options. Front Vet Sci. (2020) 7:278. doi: 10.3389/fvets.2020.00278
48. Lipsi R, Rogliani P, Calzetta L, Segreti A, Cazzola M. The clinical use of regenerative therapy in COPD. Int J Chron Obstruct Pulmon Dis. (2014) 9:1389–96. doi: 10.2147/COPD.S49519
49. Gugjoo MB. “Mesenchymal stem cells therapeutic applications in lung disorders,” In: Therapeutic Applications of Mesenchymal Stem Cells in Veterinary Medicine. Singapore: Springer. (2022). p. 279–96. doi: 10.1007/978-981-19-3277-9_8
50. Lynch TJ, Ievlev V, Parekh KR. “Heterogeneity of pulmonary stem cells,” In: Birbrair A, ed Stem Cells Heterogeneity in Different Organs. Advances in Experimental Medicine and Biology, vol 1169. Cham: Springer (2019). p. 95–117. doi: 10.1007/978-3-030-24108-7_6
51. Hong K, Reynolds S, Watkins S, Fuchs E, Stripp B. Basal cells are a multipotent progenitor capable of renewing the bronchial epithelium. Am J Pathol. (2004) 164:577–88. doi: 10.1016/S0002-9440(10)63147-1
52. Rock J, Onaitis M, Rawlins E, Lu Y, Clark C, Xue Y, et al. Basal cells as stem cells of the mouse trachea and human airway epithelium. Proc Natl Acad Sci USA. (2009) 106:12771–5. doi: 10.1073/pnas.0906850106
53. Hegab AE, Ha VL, Gilbert JL, Zhang KX, Malkoski SP, Chon AT, et al. Novel stem/progenitor cell population from murine tracheal submucosal gland ducts with multipotent regenerative potential. Stem Cells. (2011) 29:1283–93. doi: 10.1002/stem.680
54. Song YS, Lee HJ, Doo SH, Lee SJ, Lim I, Chang KT, et al. Mesenchymal stem cells overexpressing hepatocyte growth factor (HGF) inhibit collagen deposit and improve bladder function in rat model of bladder outlet obstruction. Cell Transplant. (2012) 21:1641–50. doi: 10.3727/096368912X637488
55. Lama VN, Smith L, Badri L, Flint A, Andrei AC, Murray S, et al. Evidence for tissue-resident mesenchymal stem cells in human adult lung from studies of transplanted allografts. J Clin Invest. (2007) 117:989–96. doi: 10.1172/JCI29713
56. Summer R, Fitzsimmons K, Dwyer D, Murphy J, Fine A. Isolation of an adult mouse lung mesenchymal progenitor cell population. Am J Respir Cell Mol Biol. (2007) 37:152–9. doi: 10.1165/rcmb.2006-0386OC
57. Hegab A, Kubo H, Fujino N, Suzuki T, He M, Kato H, et al. Isolation and characterization of murine multipotent lung stem cells. Stem Cells Dev. (2010) 19:523–36. doi: 10.1089/scd.2009.0287
58. Jarvinen L, Badri L, Wettlaufer S, Ohtsuka T, Standiford T, Toews G, et al. Lung resident mesenchymal stem cells isolated from human lung allografts inhibit T cell proliferation via a soluble mediator. J Immunol. (2008) 181:4389–96. doi: 10.4049/jimmunol.181.6.4389
59. Hoffman AM, Paxson JA, Mazan MR, Davis AM, Tyagi S, Murthy S, et al. Lung-derived mesenchymal stromal cell post-transplantation survival, persistence, paracrine expression, and repair of elastase-injured lung. Stem Cells Dev. (2011) 20:1779–92. doi: 10.1089/scd.2011.0105
60. Duffy MM, Ritter T, Ceredig R, Griffin MD. Mesenchymal stem cell effects on T-cell effector pathways. Stem Cell Res Ther. (2011) 2:34. doi: 10.1186/scrt75
61. Kyurkchiev D, Bochev I, Ivanova-Todorova E, Mourdjeva M, Oreshkova T, Belemezova K, et al. Secretion of immunoregulatory cytokines by mesenchymal stem cells. World J Stem Cells. (2014) 6:552–70. doi: 10.4252/wjsc.v6.i5.552
62. de Witte SFH, Luk F, Sierra Parraga JM, Gargesha M, Merino A, Korevaar SS, et al. Immunomodulation by therapeutic mesenchymal stromal cells (MSC) is triggered through phagocytosis of MSC by monocytic cells. Stem Cells Dayt Ohio. (2018) 36:602–15. doi: 10.1002/stem.2779
63. Hyvärinen K, Holopainen M, Skirdenko V, Ruhanen H, Lehenkari P, Korhonen M, et al. Mesenchymal stromal cells and their extracellular vesicles enhance the anti-inflammatory phenotype of regulatory macrophages by downregulating the production of interleukin (IL)-23 and IL-22. Front Immunol. (2018) 9:771. doi: 10.3389/fimmu.2018.00771
64. Antoniou KM, Karagiannis K, Tsitoura E, Bibaki E, Lasithiotaki I, Proklou A, et al. Clinical applications of mesenchymal stem cells in chronic lung diseases. Biomed Rep. (2018) 8:314–8. doi: 10.3892/br.2018.1067
65. Fujita Y, Kadota T, Araya J, Ochiya T, Kuwano K. Clinical application of mesenchymal stem cell-derived extracellular vesicle-based therapeutics for inflammatory lung diseases. J Clin Med. (2018) 7:355. doi: 10.3390/jcm7100355
66. Harrell CR, Sadikot R, Pascual J, Fellabaum C, Jankovic MG, Jovicic N, et al. Mesenchymal stem cell-based therapy of inflammatory lung diseases: current understanding and future perspectives. Stem Cells Int. (2019) 2019:4236973. doi: 10.1155/2019/4236973
67. Yen BL, Yen M, Wang L, Liu K, Sytwu H. Current status of mesenchymal stem cell therapy for immune/inflammatory lung disorders: gleaning insights for possible use in COVID-19. Stem Cells Transl Med. (2020) 9:1163–73. doi: 10.1002/sctm.20-0186
68. Weill D. Lung transplantation: indications and contraindications. J Thorac Dis. (2018) 10:4574–87. doi: 10.21037/jtd.2018.06.141
69. Franks T, Colby T, Travis W, Tuder R, Reynolds H, Brody A, et al. Resident cellular components of the human lung: current knowledge and goals for research on cell phenotyping and function. Proc Am Thorac Soc. (2008) 5:763–6. doi: 10.1513/pats.200803-025HR
70. Barkauskas CE, Chung MI, Fioret B, Gao X, Katsura H, Hogan BLM. Lung organoids: current uses and future promise. Development. (2017) 144:986–97. doi: 10.1242/dev.140103
71. Bourguignon C, Vernisse C, Mianné J, Fieldès M, Ahmed E, Petit A, et al. Les organoïdes pulmonaires [Lung organoids]. Med Sci (Paris). (2020) 36:382–8. doi: 10.1051/medsci/2020056
72. Archer F, Bobet-Erny A, Gomes M. State of the art on lung organoids in mammals. Vet Res. (2021) 52:77. doi: 10.1186/s13567-021-00946-6
73. Cunniff B, Druso J, van der Velden J. Lung organoids: advances in generation and 3D-visualization. Histochem Cell Biol. (2021) 155:301–8. doi: 10.1007/s00418-020-01955-w
74. Varghese B, Ling Z, Ren X. Reconstructing the pulmonary niche with stem cells: a lung story. Stem Cell Res Ther. (2022) 13:161. doi: 10.1186/s13287-022-02830-2
75. Kalra H, Drummen GPC, Mathivanan S. Focus on extracellular vesicles: introducing the next small big thing. Int J Mol Sci. (2016) 17:170. doi: 10.3390/ijms17020170
76. Han Y, Zhu Y, Youngblood H, Almuntashiri S, Jones T, Wang X, et al. Nebulization of extracellular vesicles: a promising small RNA delivery approach for lung diseases. J Control Release. (2022) 352:556–69. doi: 10.1016/j.jconrel.2022.10.052
77. Woods N, MacLoughlin R. Defining a regulatory strategy for ATMP/aerosol delivery device combinations in the treatment of respiratory disease. Pharmaceutics. (2020) 12:922. doi: 10.3390/pharmaceutics12100922
78. Kadota T, Fujita Y, Araya J, Ochiya T, Kuwano K. Extracellular vesicle-mediated cellular crosstalk in lung repair, remodelling and regeneration. Eur Respir Rev. (2022) 31:210106. doi: 10.1183/16000617.0106-2021
79. Reiner AT, Witwer KW, van Balkom BWM, de Beer J, Brodie C, Corteling RL, et al. Concise review: Developing best-practice models for the therapeutic use of extracellular vesicles. Stem Cells Transl Med. (2017) 6:1730–9. doi: 10.1002/sctm.17-0055
80. Théry C, Witwer KW, Aikawa E, Alcaraz MJ, Anderson JD, Andriantsitohaina R, et al. Minimal information for studies of extracellular vesicles 2018 (MISEV2018): a position statement of the International Society for Extracellular Vesicles and update of the MISEV2014 guidelines. J Extracell Vesicles. (2018) 7:1535750. doi: 10.1080/20013078.2018.1461450
81. Antounians L, Catania V, Montalva L, Liu B, Hou H, Chan C, et al. Fetal lung underdevelopment is rescued by administration of amniotic fluid stem cell extracellular vesicles in rodents. Sci Transl Med. (2021) 13:eaax5941. doi: 10.1126/scitranslmed.aax5941
82. Massaro G, Massaro D. Postnatal treatment with retinoic acid increases the number of pulmonary alveoli in rats. Am J Physio. (1996) 270:L305–10. doi: 10.1152/ajplung.1996.270.2.L305
83. McGowan S, Jackson S, Jenkins-Moore M, Dai H, Chambon P, Snyder J. Mice bearing deletions of retinoic acid receptors demonstrate reduced lung elastin and alveolar numbers. Am J Respir Cell Mol Biol. (2000) 23:162–7. doi: 10.1165/ajrcmb.23.2.3904
84. Horiguchi M, Kojima H, Sakai H, Kubo H, Yamashita C. Pulmonary administration of integrin-nanoparticles regenerates collapsed alveoli. J Control Release. (2014) 187:167–74. doi: 10.1016/j.jconrel.2014.05.050
85. Lin F, Josephs SF, Alexandrescu DT, Ramos F, Bogin V, Gammill V, et al. Lasers, stem cells, and COPD. J Transl Med. (2010) 8:16. doi: 10.1186/1479-5876-8-16
86. Ortiz LA, Gambelli F, McBride C, Gaupp D, Baddoo M, Kaminski N, et al. Mesenchymal stem cell engraftment in lung is enhanced in response to bleomycin exposure and ameliorates its fibrotic effects. Proc Natl Acad Sci U S A. (2003) 100:8407–11. doi: 10.1073/pnas.1432929100
87. Zhang LB, He M. Effect of mesenchymal stromal (stem) cell (MSC) transplantation in asthmatic animal models: a systematic review and meta-analysis. Pulm Pharmacol Ther. (2019) 54:39–52. doi: 10.1016/j.pupt.2018.11.007
88. Xu F, Hu Y, Zhou J, Wang X. Mesenchymal stem cells in acute lung injury: are they ready for translational medicine? J Cell Mol Med. (2013) 17:927–35. doi: 10.1111/jcmm.12063
89. McIntyre LA, Moher D, Fergusson DA, Sullivan KJ, Mei SHJ, Lalu M, et al. Efficacy of mesenchymal stromal cell therapy for acute lung injury in preclinical animal models: a systematic review. PLoS ONE. (2016) 11:e0147170. doi: 10.1371/journal.pone.0147170
90. Li JP, Wu KH, Chao WR, Lee YJ, Yang SF, Chao YH. Immunomodulation of mesenchymal stem cells in acute lung injury: from preclinical animal models to treatment of severe COVID-19. Int J Mol Sci. (2022) 23:8196. doi: 10.3390/ijms23158196
91. Horie S, Gonzalez HE, Laffey JG, Masterson CH. Cell therapy in acute respiratory distress syndrome. J Thorac Dis. (2018) 10:5607–20. doi: 10.21037/jtd.2018.08.28
92. Khoury M, Cuenca J, Cruz FF, Figueroa FE, Rocco PRM, Weiss DJ. Current status of cell-based therapies for respiratory virus infections: applicability to COVID-19. Eur Respir J. (2020) 55:2000858. doi: 10.1183/13993003.00858-2020
93. Bertoni L, Branly T, Jacquet S, Desancé M, Desquilbet L, Rivory P, et al. Intra-articular injection of 2 different dosages of autologous and allogeneic bone marrow- and umbilical cord-derived mesenchymal stem cells triggers a variable inflammatory response of the fetlock joint on 12 sound experimental horses. Stem Cells Int. (2019) 2019:9431894. doi: 10.1155/2019/9431894
94. Cabon Q, Febre M, Gomez N, Cachon T, Pillard P, Carozzo C, et al. Long-term safety and efficacy of single or repeated intra-articular injection of allogeneic neonatal mesenchymal stromal cells for managing pain and lameness in moderate to severe canine osteoarthritis without anti-inflammatory pharmacological support: pilot clinical study. Front Vet Sci. (2019) 6:10. doi: 10.3389/fvets.2019.00010
95. Magri C, Schramme M, Febre M, Cauvin E, Labadie F, Saulnier N, et al. Comparison of efficacy and safety of single vs. repeated intra-articular injection of allogeneic neonatal mesenchymal stem cells for treatment of osteoarthritis of the metacarpophalangeal/metatarsophalangeal joint in horses: a clinical pilot study. PLoS ONE. (2019) 14:e0221317. doi: 10.1371/journal.pone.0221317
96. Ursini TL, Amelse LL, Elkhenany HA, Odoi A, Carter-Arnold JL, Adair HS, et al. Retrospective analysis of local injection site adverse reactions associated with 230 allogenic administrations of bone marrow-derived mesenchymal stem cells in 164 horses. Equine Vet J. (2019) 51:198–205. doi: 10.1111/evj.12992
Keywords: lung, regenerative medicine, veterinary medicine, lung diseases, cellular therapies, biomedical engineering
Citation: Adamič N and Vengust M (2023) Regenerative medicine in lung diseases: A systematic review. Front. Vet. Sci. 10:1115708. doi: 10.3389/fvets.2023.1115708
Received: 04 December 2022; Accepted: 02 January 2023;
Published: 17 January 2023.
Edited by:
Janina Burk, Justus-Liebig-University Giessen, GermanyReviewed by:
Pratheesh Mankuzhy, Kerala Veterinary and Animal Sciences University, IndiaHaithem Ali Mohamed Ahmed Farghali, Cairo University, Egypt
Copyright © 2023 Adamič and Vengust. This is an open-access article distributed under the terms of the Creative Commons Attribution License (CC BY). The use, distribution or reproduction in other forums is permitted, provided the original author(s) and the copyright owner(s) are credited and that the original publication in this journal is cited, in accordance with accepted academic practice. No use, distribution or reproduction is permitted which does not comply with these terms.
*Correspondence: Modest Vengust, Modest.Vengust@vf.uni-lj.si