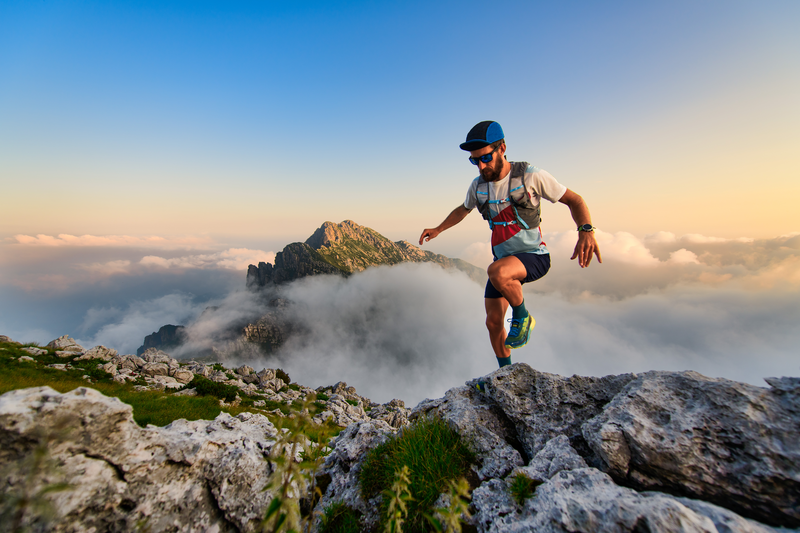
94% of researchers rate our articles as excellent or good
Learn more about the work of our research integrity team to safeguard the quality of each article we publish.
Find out more
REVIEW article
Front. Vet. Sci. , 15 March 2023
Sec. Veterinary Neurology and Neurosurgery
Volume 10 - 2023 | https://doi.org/10.3389/fvets.2023.1114798
This article is part of the Research Topic Chemotherapy and Other Pharmacotherapies for Canine Neurological Disorders View all 7 articles
“Meningoencephalomyelitis of unknown origin” (MUO)—a collective term for a group of clinically-indistinguishable (but pathologically distinct) autoimmune diseases of the CNS—has become increasingly commonly recognized throughout the world. In the 1960s−1980s the focus was primarily on the pathological description of these conditions and, largely anecdotally, their response to glucocorticoids. The subsequent availability of magnetic resonance imaging for companion animals led to a focus on imaging characteristics and response of MUO to various immunosuppressive medications. Previous reviews have not found clear evidence of superiority of any specific treatment regimen. Here, we review outcomes in a further 671 dogs treated with various combinations of glucocorticoids and immunosuppressive drugs and reported since 2009, aiming to determine whether recommendations can be drawn from the material published during more recent decades. We observe that: (i) there is more complete information on outcome of MUO-affected dogs solely receiving glucocorticoids and these reports provide evidence to undermine the dogma that MUO inevitably requires treatment with glucocorticoids plus an immunosuppressive drug; (ii) there is far more information on the pharmacokinetics of cytarabine delivered by a variety of routes, revealing that previous dosing and duration of administration in dogs with MUO may not have been optimal; and, (iii) there is a large number of cases that could be available for entry into multi-institutional randomized controlled trials. Finally, we suggest new research avenues that might aid future clinical trials in MUO through improved understanding of etiological triggers and individual patterns of immune response, such as the impact of the gut microbiome, the potential of CSF flow cytometry, and the establishment of robust clinical scores for evaluation of treatment success.
Immune-mediated meningoencephalomyelitis in dogs has been recognized for at least six decades (1) and what we now label as meningoencephalomyelitis of unknown origin (MUO) was first reported as a distinct disease in dogs in the 1970s (2–4). The term “MUO” is more recent; initial reports refer to the condition as “reticulosis,” which avoided defining it as either neoplastic or inflammatory (4). Subsequently, meningoencephalomyelitis lacking an identifiable etiology was often described as “granulomatous meningoencephalomyelitis” (GME) (5–7) and the first descriptions use this term in preference to the more recent “MUO” umbrella term for the clinical constellation of idiopathic (autoimmune) meningoencephalomyelitis in dogs (8). Although MUO is used as a synonym for autoimmune meningoencephalomyelitis in dogs, it specifically excludes steroid-responsive meningitis [which is recognized as a primary disease of the meninges rather than the central nervous system (CNS) itself] and (the rather uncommon) eosinophilic meningoencephalomyelitis. Conditions such as idiopathic generalized tremor syndrome, idiopathic hypertrophic pachymeningitis or even immune-mediated disease centered on neurotransmitter receptors could conceivably also be part of a spectrum of “MUO” (if defined as “immune-mediated disease of the central nervous system”), but are sufficiently distinct in presentation to be recognized as separate disease entities and are not discussed further here.
During pathological examination it is possible to designate various specific types and severities of meningeal, white and gray matter inflammation and necrosis into subtypes of MUO (8, 9). However, a recent article has implied that pathologic differences may be predominantly of academic interest, because different subtypes can even occur together within the brains of individual affected dogs (10), suggesting there may be little clinical gain from attempting to define the different pathological sub-types in living animals. Instead, in the absence of biopsy confirmation, most cases are treated based on signalment, imaging and cerebrospinal fluid (CSF) findings alone.
Treatment of MUO was limited at first because the cause of the myriad possible neurological signs was unclear and there were reasons for possible confusion with distemper and infectious meningoencephalitis (11). However, during the 1990s MUO was sufficiently well-recognized in the clinic and pathologically described as an idiopathic inflammatory condition that treatment with anti-inflammatory agents was commenced. In one of the earlier summaries of clinical features and outcomes, radiation, which also has a well-recognized anti-inflammatory effect at low doses (12), appeared effective (13). The diagnosis of MUO increased in frequency during the 1990s until the present day, which could be a result of increased recognition, recent increase of popularity of certain breeds (e.g., Pugs, French bulldogs) as well as genuinely increased prevalence. It is difficult to distinguish between these possibilities because this time period also corresponds to that in which veterinary access to magnetic resonance imaging (MRI) rapidly increased. Nevertheless, it is commonly thought that autoimmune disease is likely becoming more prevalent in pet dogs in the West, as it is in people (14); and, in dogs, may also be caused through poorly controlled breeding that reduces genetic diversity (15). It is therefore probable that this is genuinely a more common disease than it was in the 1980s and before.
Non-infectious (immune-mediated) meningoencephalomyelitis is common in humans [e.g., neurosarcoidosis, neuromyelitis optica, acute demyelinating encephalomyelitis, etc. (16)] and most widely apparent in the high prevalence (especially in specific geographical regions) of multiple sclerosis (MS) (17–19). The clinical signs of MS are similar to those of MUO in dogs, so that many different syndromes are recognized to be part of a constellation of clinical signs. In MS the most common presentations relate to visual deficits and spinal cord dysfunction [which also occur, albeit at lower frequency, in MUO-affected dogs (20)], but cognitive deficits have become more widely appreciated recently (17, 21). Pathologically, MS in humans and MUO in dogs also are broadly similar, consisting of inflammatory cell infiltration, often along vascular pathways; in MS both T and B cell infiltration is recognized, whereas MUO appears to be predominantly T cell dominated (22). The recognition of association of dog leucocyte antigen class II and necrotizing meningoencephalitis (in pugs) also demonstrates strong parallels with aggressively progressive forms of MS (23). On the other hand, demyelination—the hallmark pathological feature of MS—is rarely a dominant feature in MUO (8), although the recognition of regions of Schwann cell remyelination in MUO lesions (24), provides clear evidence that it does, at least sometimes, occur.
Because of the huge importance of MS in human neurology there are laboratory models of the disease in experimental animals, collectively known as “experimental autoimmune encephalomyelitis” (EAE) (25). This is a rapidly-developing paralytic disease of rats and mice (and can also be created in other species) that is induced by injection of processed components of the CNS together with an adjuvant to trigger widespread inflammation in the CNS. Pathologically, this condition bears even closer resemblance to MUO in dogs than MS in humans, for instance in the lower prominence of demyelination. The parallels in terms of neurological signs and pathological features of both EAE and MS with MUO can be useful in determining useful therapeutic strategies for affected dogs.
The etiology of MUO is by definition unknown but, in addition to individual genetic predisposition, many autoimmune diseases are strongly suspected of having a trigger, which may be infectious, environmental or neoplastic (26). In contrast to recent developments in MS (27, 28) and despite extensive searches for infectious triggers, none have so far been identified for MUO in dogs (29–36) and neither have broad environmental risks been identified in association with canine immune-mediated disease more generally (37), and so the etiology is assumed to be an idiopathic self-directed immune response. Nevertheless, the complicated immune-mimicry that underpins the relationship between Epstein-Barr virus and MS in humans implies that a similar relationship between an as yet unidentified pathogen and MUO cannot be ruled out. Detailed case-control studies on large populations of dogs would be required to identify such relationships.
Genetic causes have long been suspected in dogs with MUO because of the high prevalence in specific breeds, namely Pug, Maltese, Yorkshire terrier, Pekingese, Chihuahua, Papillon, Shih tzu and others (38), and the underlying genetic risk factors in these breeds are beginning to become more apparent: (i) ~75% of Pugs with necrotizing encephalitis have a specific combination of alleles coding for major histocompatibility complex class II molecules on chromosome 12 containing exons for the dog leukocyte antigen and conferring on them a relative risk of 5.45 of developing the disease (39); and (ii) in Maltese dogs, ILR7 on chromosome 4 and FBXW7 on chromosome 15, both involved in the immune system regulation, could be implicated in necrotizing encephalitis (40).
There remains considerable controversy around treatment of MUO in dogs, because a multitude of drugs have been prescribed, using many different dosing schedules and combinations. In this review we summarize the data available from publications since 2009 to follow-up on our previous review (38). The objectives of this current review are to assess the treatment results, in particular for those cases receiving glucocorticoids alone, and those reported in association with various dosing schedules of cytarabine, and how these correspond to recent evidence from pharmacological investigations. In addition, we suggest new research avenues that could investigate the etiology of the condition and may guide future treatment.
Because no specific directly-treatable etiology has been identified, treatment almost exclusively focuses on immunosuppression, although there is frequently a need for adjunctive treatment of associated neurological signs, such as seizures [that occur in 20–25% of cases (41–43)]. In this review we will focus only on treatment aimed at the underlying disease process rather than symptomatic therapy aimed specifically at controlling the resulting clinical signs.
When non-infectious meningoencephalomyelitis was first diagnosed, affected dogs were treated with glucocorticoids (GCs), predominantly dexamethasone, but often for relatively short periods of time and, upon relapse, were frequently euthanased—thereby permitting the diagnosis (11, 13, 38). In a large early study, some cases were also treated with radiation, which the authors associated with more prolonged survival after diagnosis (13). With hindsight, this was an unusual case series because the median survival period for dogs with multifocal disease (a common presentation) was extremely short (14 days, ranging from 1 to >1,215 days), which would nowadays be considered unusual, although it may also be at least partly attributable to the study inclusion requirement for definitive post-mortem diagnosis. Nevertheless, as stated by Zarfoss et al. (44), the prognosis at that time was widely considered to be poor, with Thomas and Eger (45) stating that most dogs with GME were euthanased or died within 3–6 months of presentation.
Glucocorticoids have been the mainstay of treatment in the intervening years, although the positive response to immunosuppression combined with the prominent adverse effects of high-dose GC therapy have prompted searches for substitutes. A large range of immunosuppressive therapies have been used in treatment of MUO (38, 46) with little apparent overview of what attributes of the medications might be desirable; for instance, which specific aspects of the immune response should be targeted. Cytosine arabinose (i.e., cytarabine) has become a favored medication for MUO, since its first use by Nuhsbaum et al. (47) and presentation by Cuddon at the 20th ACVIM forum in 2002 (48), for reasons that appear opaque. As with many other conditions in companion animals, there is a dearth of evidence on comparative efficacy of different drug regimens, because formally-designed randomized comparative trials are rare. Instead, veterinarians have tended to take the alternative approach of using a specific medication with which they feel comfortable and then, if it fails, to introduce another medication. This can be satisfactory as a way of managing individual dogs but is unsatisfactory in designating specific therapies as likely to be the optimal starting medication after diagnosis.
In our previous review (38) we noted that several immunosuppressive medications had been used for treatment of MUO, with little evidence to support any specific regimen over another. Since that time, more data has become available on responses to specific medications and specific regimes, although no formal trials of one medication vs. another have been conducted. Almost all protocols continue to use GCs as the first-line treatment, followed by addition of various other medications in attempts to spare GC use or to provide additional control of clinical signs, or both. We have searched the literature from 2009 onwards, using the same methods and key words described in our previous review [see (38)] and identified 15 studies for further review (Table 1). Here we summarize the results of treatment of any form of MUO as included by the authors. One publication detailed the outcomes for a contingent of dogs that presented with spinal cord signs only (62) and included dogs treated with GCs and/or cytarabine, with a median survival of 669 days. This report is not discussed below because of the clear distinction in presentation, although there is currently little reason to suppose that outcomes differ with different distributions of lesions at presentation.
Table 1. Summary of treatments and outcomes in dogs with meningoencephalitis of unknown origin published since 2009.
Although there is considerable weight of opinion that sole use of GCs is not as efficient a therapy for MUO as more complicated regimens there is, at present, no definitive evidence in support of such claims. Furthermore, it is often difficult to distinguish relative effects of other agents because they are almost exclusively used together with GCs and, in many instances, are not commenced until after a specified period (during which many severely affected dogs may already have succumbed to the disease or improved so as not to be treated with a second medication). In our previous review (38), we found only 26 dogs treated solely with GCs and these had a reported median survival of 36 days, possibly explaining the belief that treatment with GCs alone is inappropriate. However, the survival of those dogs was extremely variable, ranging from 2 to 1,200 days.
Since 2009, three groups have provided much additional data on sole therapy of MUO with GCs. Mercier et al. (49) reported on 16 dogs that received intravenous methylprednisolone at 30 mg/kg then 15 mg/kg 3 ho later, then 10 mg/kg 2 h after that, followed by prednisone or methylprednisolone at 2 mg/kg/day. Seven were designated as “responders” based on second CSF analysis and three non-responders received adjunctive therapy. They recorded a median survival of 602 days for all included dogs. Paušová et al. (42) reported on a cohort of 168 dogs, although it is important to note that 14 dogs that could have been included were euthanased upon diagnosis (therefore implying that subsequent survival analysis is biased by their exclusion) and these received intravenous dexamethasone at 2 mg/kg/day for 3–5 days, then prednisone at decreasing dose starting at 2 mg/kg/day. Relapse occurred in 19 dogs during therapy and in 45 after stopping therapy. Median survival time was 570 days (the dogs that were immediately euthanased upon diagnosis were not included in this analysis). Lawn and Harcourt-Brown (50) also reported short-term outcomes on 63 dogs that had received GCs alone in a non-randomized retrospective cohort study. Following an initial dose of 2 mg/kg/day prednisolone (or dexamethasone equivalent) seven of 63 dogs (11%) died within 7 days, which was equivalent to the proportion dying in a cytarabine and GCs group at the same institution (see below), and 40 dogs were alive at the 100-day study termination point.
This immunosuppressive medication had been reported in only 4 dogs for treatment of MUO in our previous review and there are now three further reports.
Barnoon et al. (51) reported on 25 dogs that were given 4 mg/kg/day prednisone as soon as possible after diagnosis, plus 20 mg/kg mycophenolate twice daily; doses were tapered with time. Five dogs showed complications thought to be caused by mycophenolate, which was then withdrawn and these dogs were not included in survival outcomes. For the remaining 20 dogs, median survival was 250 days. Apart from acute problems with gastroenteric signs the long-term adverse effects were not severe, although there were five recorded instances of disease that may have been induced by long-term immunosuppression.
Woolcock et al. (52) reported on 25 dogs (although a further two were excluded through euthanasia because of cost / prognosis at <7 days). Each dog received prednisone at 2 mg/kg/day or more, plus mycophenolate at 20 mg/kg per day. Some dogs also received cytarabine and / or cyclosporine and other medications. Median survival was 731 days. Two dogs showed mild adverse effects thought to be associated with mycophenolate.
A publication by Song et al. (53) included 86 dogs for follow-up (although eight were removed because of adverse effects of mycophenolate); each was given prednisone at 2 mg/kg/day plus mycophenolate at ~20 mg/kg twice daily. Median survival for all dogs was 558 days. Almost 50% dogs had adverse effects from treatment—mainly gastrointestinal signs acutely but then long-term evidence of adverse immunosuppressive effects (infections) and hematological abnormalities (~50% dogs).
Cytosine arabinose (i.e., cytarabine) has been used as a therapy for MUO first in 2002 in one dog (47) and started subsequently to be reported in case series from 2006 (44) and 2008 (63). Many of the subsequent clinical investigations of efficacy reviewed here have originated from the group lead by Lowrie in the UK and which included dogs free from other medications before diagnosis of MUO. The first of this series of publications (54) reported on 39 dogs in which treatment, similarly to Zarfoss et al. (44) and Menaut et al. (63), consisted of a combination of prednisolone commencing at 2 mg/kg/day for 4 weeks and decreasing thereafter, plus cytarabine at 50 mg/m2 twice daily subcutaneously for each of 2 days at progressively longer intervals (i.e., 3 weeks, then 4 weeks, 5 weeks etc.). Thirteen dogs died or were euthanased within 3 days of diagnosis and the overall median survival was 26 days. A follow-up study by the same group (55) compared the outcomes reported in 2013 with outcomes of a similar second cohort of 41 dogs that received the same GC regimen but initially received cytarabine as an intravenous dose of 100 mg/m2 over 24 h rather than subcutaneously (but subsequent treatments were the same). The outcomes were strikingly different, in that only four dogs of the new cohort died or were euthanased by 3 months (compared with 22 in the subcutaneous cytarabine cohort) and all the remaining 37 dogs were still alive at 12 months. In the third report in this series (56) a cohort of 42 dogs was treated with the same GC regimen plus a single cytarabine 24-h continuous rate infusion (CRI) of 100 mg/m2 at diagnosis. Treatment response appeared good, with 28/42 dogs reported as successful at 34 weeks and, overall, six dogs died (or were euthanased) because of their disease during a follow-up of 36 months. Median survival time was not available for each of these last two reports because so large a proportion of dogs survived, but the median time to relapse was similar (299 vs. 285 days, respectively) between those that received only a single cytarabine dose and those that received that plus subsequent subcutaneous doses.
This interesting series of reports raises many questions. First, “historic controls” are controversial, the problem being that it can be difficult to ensure that the populations recruited at different time periods are truly similar at entry to the study and that subsequent clinical decisions are made in the same way in each study sample. Broadly, in this series the study populations can be seen to be similar (although “how similar?” cannot be properly evaluated by statistical testing), but this cannot exclude the possibility of “unmeasured confounders” that, in contrast, will also be equivalent in sample populations in large randomized trials (the gold standard for such comparative studies). The median survival in the first report was unusually short for a modern cohort of MUO cases; such unusual results are more common when small populations are analyzed and are difficult to interpret without a randomly-allocated contemporary control group.
Second, a reasonable conclusion from this series of studies would be that cytarabine has little overall benefit beyond that given by GCs because the repeated dosing in the first two studies did not have benefit over the single dose given in the third study [this conclusion is also supported in the way cytarabine is used in humans, in whom it is administered over many consecutive days]. The possibility remains from this series of reports in dogs that the single initial dose has benefit, but recent data has called that too into question. In a non-randomized retrospective study in which dogs received prednisone and cyclosporine at the time of diagnosis either with or without cytarabine (200 mg/m2 as a CRI, or as four subcutaneous injections over 2 days) there was no apparent benefit of the cytarabine (57). Similarly, Lawn and Harcourt-Brown (50) did not detect benefit of cytarabine in their retrospective cohort study. However, it must be considered that the possible benefit of cytarabine in those reports might have been masked by the (beneficial) effect of concurrent GCs and/or cyclosporine but this finding implies there is little or no additional benefit.
Lastly, there is also the question of whether the administered dose of cytarabine was sufficiently high to achieve therapeutically useful blood levels. This specific issue has been examined in many recent publications but, specifically, it was shown that 200 mg/m2 [double the dose used in (55)] CRI cytarabine over 24 h was unreliable at producing durable blood levels above 1μg/mL (the presumed therapeutic level) (64). Nevertheless, whether the efficacy of cytarabine is time- (above threshold) or concentration-dependent is still not established. A reasonable overall conclusion is that the small case numbers included in many of the reports implies greater likelihood of more extreme results and so the reported differences between outcomes in different study protocols are likely to represent random variation rather than true differences in effects of different treatment regimens.
Important recent work has focused on cytarabine pharmacokinetics, especially that related to administration by different routes. This is especially pertinent because intravenous infusions imply a high cost to the owners (because it is typically given over at least 8 h and requiring hospitalization of the animal), thereby incurring an elevated risk of financially-driven euthanasia of affected animals. Many questions remain regarding the optimal method of delivering cytarabine for treating dogs affected by MUO. Many of these are directed at the important question of whether it is possible to use this therapy without needing the owners to attend with their dogs in person for intravenous injections.
The use of cyatarabine is predicated on the notion that it is necessary to attain a blood level of 1μg/mL (64), based on its uptake by cancer cells in vitro (65) resulting in their death (66). However, data from human medicine suggest that CSF:plasma ratio of cytarabine is ~10–25% and that intravenous infusions of 400 mg/m2 are required to attain a CSF concentration of 1.2 mmol (approximately that required for cytotoxicity) (67). Most veterinary studies on cytarabine regimens have been conducted to determine how well they attain concentrations of 1μg/mL, although it remains unknown how long it is necessary for the blood level to exceed that value.
Fortunately, the consensus from these studies is that (modeled) repeated subcutaneous injections can attain just as prolonged periods of high blood concentrations as intravenous delivery (68, 69) and a single high-dose subcutaneous injection provides a (marginally) higher overall drug exposure (in blood) than repeated lower dose injections (70). A recent technological development (the “Omnipod” system) opens the possibility of delivering high dose cytarabine via subcutaneous infusions that can be monitored by owners at home (71).
Flegel et al. (58) reported on 43 dogs with various categories of MUO that received prednisone at variable dosage (some quite high), plus lomustine at a median dose of ~60 mg/m2 every 6 weeks. Median overall survival is difficult to extract from this report because the survival was categorized by the individual sub-type of MUO, but all were between 91 and 457 days. Adverse effects appeared to be limited, although there was a tendency for treated dogs to show anemia; one dog developed leucopenia and died of septic shock (this complication was attributed to lomustine).
Pakozdy et al. (59) reported on 14 dogs, some treated with ciclosporin 3 mg/kg twice daily as well as GCs (which was often given at high dose). Median survival with ciclosporin was 620 days (vs. 28 days for GCs alone). Adverse effect in one ciclosporin dog was severe: life-threatening gastrointestinal hemorrhage that required a blood transfusion. The comparison with GC treatment alone is difficult to interpret because the two regimens were not parallel or allocated randomly.
Brady et al. (60) included 40 dogs that were treated with prednisone at 1 mg/kg/day and often started with intravenous dexamethasone; ciclosporin (3–5 mg/kg twice daily) was added to the treatment regimen and the prednisone dosage increased to 2 mg/kg/day when the infectious disease results were known, although no dogs were lost to treatment or survival analysis through this mechanism (Brady, personal communication). Median survival was reported to be 1,345 days; few dogs died in the first few weeks after diagnosis (in contrast with most other studies) and, notably, the shortest survival was 487 days. Four dogs had cytarabine added (one because of ciclosporin adverse effects). No other adverse effects were reported.
Wong et al. (61) reported on 40 dogs treated with prednisone 2 mg/kg/day plus azathioprine at 2 mg/kg/day, followed by recommended decreasing dosage, and some dogs also received dexamethasone. Azathioprine was only commenced after diagnostic tests were complete. Median survival of the included dogs was 1,834 days and dogs that died soon after diagnosis and before initiation of azathioprine were not included in this analysis. Few adverse effects that could be attributed to azathioprine were recorded, and even those that might have been attributed to azathioprine were generally mild.
Stem cells have long been noted to show anti-inflammatory effects and have previously been used for this effect in experimental models and human autoimmune disease [e.g., (72)]. In 2015, Zeira et al. (73) reported on a series of eight dogs that received autologous bone marrow mesenchymal stem cells after failing to respond adequately, or having developed adverse effects owing, to conventional therapy with GCs, with or without adjunctive cytarabine. The cells were administered intrathecally and via systemic blood vessels (carotid artery or intravenous). The dogs were reported to gradually improve over a 6-month period and the seven long-term survivors (one dog died of intercurrent disease) became neurologically normal or near-normal, although were also continuing to receive prednisone.
Low-dose radiation has an anti-inflammatory effect and there are a few reports on its use for MUO. Recently, Beckmann et al. (74) delivered 30 Gray over 10 fractions in 2 weeks to the whole brain or the lesioned region of six dogs that completed the therapy (one other was withdrawn because it probably had another diagnosis). Five dogs improved and one was unchanged at the end of therapy. Radiotherapy was initiated at between 3 and 56 days after starting medical therapy with prednisone at ~2–4 mg/kg/day and, in one case, cytarabine. Twelve-month outcomes were good in five of the six cases (one relapsed and was euthanased), with normal or near-normal neurologic status.
Although cytarabine readily crosses the blood-brain barrier there can be advantages to intrathecal (i.e., subarachnoid space) delivery because it allows higher concentrations to be available to cross the CSF-brain barrier and longer half-life. Subarachnoid delivery of cytarabine is used in human medicine for treating various malignancies and has a good safety record (67). Genoni et al. (75) used intrathecal delivery in a series of 112 dogs with MUO at 100 mg per dog (a calculated dose range of between 70 and 450 mg/m2), and in some animals this was accompanied by methotrexate (2.5 mg per dog). One dog developed seizures that were easily controlled. Whether the treatment was of benefit is not recorded and it is not reported whether the intrathecal treatment was repeated. According to a study of cytarabine administration to rat brain (76), intrathecal delivery would appear to have little benefit over systemic administration in achieving widespread high brain tissue concentration. Although intrathecal delivery does circumvent the blood-brain barrier it still does not place drug directly into the brain and traverse of the CSF-brain barrier is still required. Instead, the advantage may lie in the ability to achieve high CSF concentration for a prolonged period (because liver degradation is avoided) but it appears that depth of penetration into brain tissue is still limited.
The conclusion of our previous analysis of treatment for MUO was that there was no specific regimen that appeared to show overall superiority compared with others. It appears that, despite the addition of more recent reports, we are still in much the same position now. There are many protocols that have been used by different centers, but no direct comparisons between different approaches.
Glucocorticoids as a single therapy have now been reported in a larger number of dogs (26 cases before 2009 vs. 247 cases since 2009) compared to our previous review and the filling of this knowledge gap suggests survival periods of similar magnitude to dogs receiving GCs plus an adjunctive therapy and with extremely wide ranges, although direct comparison between studies is difficult. Despite the use of a wide range of different immunosuppressive agents there is still not reliable evidence that any of these are superior to GCs alone and there are potential benefits to owners to relying upon GCs alone, such as the ease of access, cheap cost and ease of administration. Nevertheless, this must also be balanced against the high rate of adverse effects of high-dose GC administration. Even so, there is also the potential for many owners to request euthanasia of their affected dog because of the costs associated with frequent revisits (for cytarabine injections or for blood tests). Financial exhaustion is a common cause of euthanasia in veterinary medicine (77).
Radiotherapy appears likely to be effective based on the small numbers of cases reported, in that it appears able to “rescue” dogs that had previously been progressing poorly, but might not be accessible—geographically or financially—for many owners. Furthermore, it can be difficult to commence radiotherapy as an emergency treatment, at least in some geographical locations, but is often required because of the recognized high rate of death soon after diagnosis (38, 46). Dogs in the recent report (74) had been treated medically for between 2 and 35 days before starting radiotherapy which suggests the possibility that the included cases were (inadvertently) selected for longevity (because of the need to survive for at least 48 h after diagnosis). Nevertheless, this same caveat also applies to many reports on medical therapies.
It would appear that the reliance on cytarabine perhaps mainly derives from a small number of cases reported in the mid-2000s, in which a subcutaneous dose of 50 mg/m2 twice a day for 2 days and then repeated every 3 weeks was empirically administered, perhaps because it appeared against a background of an expected largely dismal prognosis. Although specific evidence for efficacy is lacking, there is evidence that it is not associated with a high incidence of adverse effects (78), possibly reflecting the low dosages used in MUO cases. Subsequent recording of prolonged survival of MUO-affected dogs when treated with many other immunosuppressive regimens (including GCs alone) imply that the cytarabine regimen used in these reports may not have been as efficacious as originally interpreted. For instance, treatment also included concurrent prednisone (1–2 mg/kg twice daily) as well as commencing with a high dose of dexamethasone. Moreover, more recent publications (see above) examining the pharmacokinetics of cytarabine raise questions about the appropriateness of previous apparently-effective dosing regimens, including that reported by Zarfoss et al. (44), implying that the impact of this therapy might be difficult to extract from the overall immunosuppressive effect of the high GC dose. Of course, it may be that cytarabine is specifically useful but further evidence is needed regarding the ideal dose and dosing interval. An argument against cytarabine as an agent in MUO is that there are many protocols that have been advocated but there is little that is known about how best to dose this medication, and specifically whether cytarabine crosses the blood-brain barrier via time- or concentration-dependent mechanisms. Cytarabine is primarily administered in people (typically for treatment of acute myeloid leukemia) via twice daily subcutaneous injection or daily intravenous infusion for at least 7 consecutive days (79, 80) using doses ranging from 100 to 200 mg/m2, creating prolonged high blood concentrations (67) and this could perhaps be explored further in MUO. There appears to be plenty of scope to increase the dose because, so far, few adverse effects have been reported (78). Rescue protocols in people consist of administration of enormous doses up to 3,000 mg/m2, with 1,000 mg/m2 being seen as a safer approach (81). Many of the veterinary regimens seem to have marginal probability of reaching the therapeutic threshold required to achieve adequate CSF concentration. On the other hand, in human medicine there is evidence that the therapeutic ceiling (for acute myeloid leukemia, at least) is reached with quite low doses (200 mg/m2) anyway and so higher doses might just expose patients to higher risk of adverse events for no benefit (81).
Adverse effects of medications do not seem to be limiting for most of the immunosuppressive agents used, with the possible exception of high-dose mycophenolate (i.e., when used at 20 mg/kg twice daily), which appears to be associated with a relatively high rate of unacceptable complications. Glucocorticoids themselves are probably responsible for most of the adverse effects we see on a day-to-day basis. Indeed, the rationale for using most of the other immunosuppressive agents is largely to avoid the long-term effects of the GCs, which do themselves often “work” very well in this condition.
In comparison with therapy for MS in humans (see below) the only medication apart from high-dose GC therapy that is available and similar in veterinary medicine is leflunomide. In MS, teriflunomide (the active metabolite of leflunomide) is the only agent to which veterinarians have routine access that is considered to have value in treatment of MS (82). There are, currently, few reports on use of leflunomide in MUO (83).
As mentioned above, there are many reasons to consider MUO as “dog multiple sclerosis” although the analogy is imperfect because, as mentioned above, there are differences in pathological detail between dogs and humans. However, there are even differences in character and severity of EAE between different strains of rat, attributable to differences in genetic or environmental background, or both (84–87). Therefore, it is perhaps not unreasonable to consider that differences in lesion detail resulting from a parallel immune phenomenon in the two species may result from the large differences in genetic and environmental factors between humans and dogs. While MUO may have some value as a model condition for human medicine, conversely, because of the considerably more advanced knowledge of pathogenesis of MS and the recent rapid development of new therapies for affected humans, it is more realistic for veterinarians to consider human MS as the model disease for MUO. Several different subtypes of MS are recognized, based on their progression dynamics and, following this approach, we might consider that MUO is most similar to primary progressive MS, which has a relatively poor prognosis in humans (17).
In primary progressive MS, although GCs may be used initially, the mainstay of therapy has now been revolutionized by monoclonal antibodies that modulate immune cell function. Following successful clinical trials, ocrelizumab, a monoclonal antibody against the CD20 antigen on B cells, is now the recommended first-line treatment (82, 88). Other, more veterinary-accessible, agents have either been shown not to be useful [glatiramer acetate (89)] or have uncertain benefits [interferon-beta (90, 91)] in clinical trials in human primary progressive MS.
The problem for veterinarians is that monoclonal antibodies could be applied in dogs [as they have been for dermatologic disorders (92)], but there are many obstacles of time, cost and the relatively small market for these products, that might limit our access in future to parallel “canine-ized” medications for treatment of autoimmune CNS disorders.
In 2011, following a tradition of modeling MS with the experimental disease EAE in rodents (25), it was found that germ-free rats are far less susceptible to development of EAE than those that have a normal gut microbiome (93) and subsequent exploration has revealed that there are gut bacteria that promote pro- or anti- inflammatory immune environments in the CNS. There are various mechanisms underlying these effects, but it is thought that short-chain fatty acid production in the gut is of major importance (94). In turn, these anti-inflammatory products are the result of metabolism of gut contents—predominantly dietary fiber—by specific categories of bacteria, notably Prevotellacae and Faecalibactirum prausnitzii, although there are undoubtedly almost unquantifiable numbers of bacteria (and other microbes) that also play roles in regulating systemic immune responses.
More recently, evidence has accrued that in dogs with MUO (95), as well as in people with MS (96), there is relatively low abundance of these anti-inflammatory bacteria in the gut microbiome, raising the question as to whether some type of microbial transfer, or possibly even a diet change, might aid in control of both diseases. Initially, fecal microbial transfer was carried out by enema or similar methods (97) but, more recently, oral delivery of lyophilized and selected bacteria has become more widely available and is less cumbersome to administer (98). A current clinical trial (Jeffery, unpublished data) in dogs is investigating whether fecal microbial transfer might aid control of MUO in dogs (Table 2 illustrates the normalization of fecal microbiome associated with fecal microbial transfer).
Currently, diagnosis of MUO is largely presumptive and relies on cross-sectional imaging such as MRI and analysis of CSF, as reviewed elsewhere (38, 46). Definitive diagnosis requires biopsy and analysis of inflammatory brain lesions but, despite being safe (99–101), this is costly and so seldom used. Magnetic resonance imaging also remains imperfect with some studies finding that as many as 40% of lesion are not detected and there are large variations in lesion appearance [reviewed by (46)]. An interesting approach to use MRI for more precise diagnosis is to determine the permeability of the blood-brain barrier in dogs with MUO (102).
Imaging is best complemented with analysis of CSF, and a myriad of biomarkers (e.g., acute phase proteins, antibodies and cytokines) have been investigated in the last decade in an attempt to identify and quantify the molecules that might help classify and identify the different forms of MUO. However, this approach remains laborious (a few biomarkers out of thousands of possible candidates are typically tested at one time on a small number of affected dogs), access to CNS tissue to compare circulating and in situ biomarkers is rare, and most markers lack specificity, as highlighted by Andersen-Ranberg et al. (103). Since that review, five further studies (104–108) revisited blood neutrophil-to-lymphocyte ratio or various biomarkers that showed promise. In general, many biomarker comparisons have been made but few studies have examined sensitivity and specificity (rather than simple statistical tests of difference). Sometimes it may also be difficult to determine whether the elevation of specific biomarkers coincide or precede clinical deterioration, thereby limiting their diagnostic utility. A further limitation is that, with some exceptions (105), comparisons have often not been made between findings in MUO and other neurological conditions. On the other hand, the more sophisticated technique of “immunosignaturing,” which determines patterns of serum antibody binding to a large array of peptides, was shown to discriminate between healthy dogs, dogs with brain tumors and dogs with MUO, including in a validation set (109), suggesting promise in discriminating different types of MUO in future (see below).
In order to more precisely tailor immunotherapy for each individual dog (and to design more targeted clinical trials) it would be helpful to define the nature of the disease in each individual. For instance, to define an immune phenotype, based on the balance between B and T cell responses, or suggest more precise therapy based on the balance between T-helper and natural killer cells. Such discrimination might be made by examining the phenotypes of the cells available in the CSF. This approach, termed immune profiling, is well-known in neuro-inflammatory diseases and MS in humans (110, 111) and flow cytometry (112) is used to label and characterize inflammatory cells in the CSF. This technique has now been developed for CSF from MUO cases (Figure 1, courtesy of Prof. Wooldrige and Dr. Milodowski) and is currently being investigated to determine whether specific patterns of response are associated with specific disease types or prognosis. In time, this may allow more complex analysis of T-cell receptor usage and antigen specificity along with the study of genes leading to expression of specific receptors. This “genotyping” of the immune response together with screening tools derived from the “omics” [e.g., genomics, proteomics, metabolomics (114)] may help identify disease triggers and therefore target therapy. A concrete example is the use of plasmapheresis in humans to treat encephalitis with known antibodies directed against intracellular onconeuronal antigens such as ANNA-1/anti-Hu, and representing markers of T-cell mediated immunity (115).
Figure 1. An example of CSF flow cytometry results in a dog with MUO: a population of live lymphocytes is sorted, and two antibodies used to differentiate their subtypes. The procedure requires the CSF to be centrifuged and the resultant cells to be washed and then suspended in phosphate buffer saline with fetal calf serum before being incubated with primary antibodies (e.g., CD3, CD21) that are fluorescently labeled. Subsequent flow cytometry classifies the cells according to their fluorescence, size and shape. With further validation, CSF flow cytometry may help to define the relative importance of B- vs. T- lymphocyte-mediated inflammation and so guide the choice of immunosuppressive medication [e.g., cyclosporine mainly has suppressive effect on T-lymphocytes (113)] and, in future, guide development of appropriate monoclonal antibodies against specific types of lymphocytes. SSC, side scatter; FSC, forward scatter.
Since our previous review on MUO in dogs there has still only been one prospective clinical trial [on “COP” vs. cytarabine (116)] to compare therapies for MUO and that investigated only a small population of affected dogs (although it did strongly suggest one of the options was not useful). There does appear to be a widespread resistance to trials on this condition, which perhaps partly reflects the overall reluctance of veterinarians to engage with randomization but might also be because each dog can be treated as an “n-of-1” therapeutic trial (a single subject clinical trial in which an individual patient is the sole unit of observation in a study investigating the effect of a treatment). Nevertheless, it would still be helpful to know which was the best therapy to start with, or to know whether it is preferable to commence therapy with two medications rather than just one.
A basic requirement of a clinical trial is to have a reliable outcome measure. For MUO a common reported end-point has been survival. This is indeed an important outcome but death from MUO may happen many months, if not years, following diagnosis, which can make it difficult to avoid large losses to follow-up. An alternative is to record neurologic deficits, but that then raises the question of how to compare heterogeneous patients with widely varying presenting signs. A solution that we have suggested previously (116) is a simple scoring scheme that aims to capture clinically-important worsening of disease and then using that outcome to declare a dog “worse” than when it presented and therefore a “failure of treatment.” The purpose of this alternative measure is that the “failure” of a treatment will occur long before the final demise of the animal and so will speed attainment of meaningful end-points to compare effectiveness between therapies. Gonçalves et al. (117) proposed recently a neurodisability scale for dogs with MUO, designed from the retrospective study of 100 cases and then tested prospectively on 31 new cases, with apparent good reliability. An alternative approach, that has been exploited in a recent unpublished trial (Harcourt-Brown, personal communication) is to compare the response to different treatment regimens over a very short follow-up period. This has the twin merits of drastically reducing loss to follow-up and also identifying interventions that protect dogs from dying in the immediate period after diagnosis, when a large proportion of overall mortality occurs.
During the past decade many reports on treatment of MUO have appeared but, unfortunately, it is hard to draw strong overall conclusions from these studies and no single medication appears advantageous over others, as evidenced by the enormous survival range of a few days to hundreds of days regardless of treatment protocol.
This impasse is in part due to the lack of comparison of medications in randomized controlled trials, but possibly also to the use of survival as the outcome measure, which may prevent detection of more subtle differential medication benefits. It therefore appears that examining response to treatment in the future should also rely on scoring systems, as previously proposed and currently in further development.
The lack of discernible differences between treatments may be because of a mixture of “responders” and “non-responders” in each cohort of dogs, and the design of future trials might be enhanced by identifying specific patient profiles or combination of baseline variables that could be used for stratification. Immune profiling of MUO cases (e.g., using CSF flow cytometry) would be especially powerful in this regard because it permits: (i) splitting canine MUO into more distinct groups (thus stratifying for trials); and (ii) post-hoc analysis of treatment outcomes in light of the immune footprint. In addition, such in-depth disease analysis may identify pathophysiological mechanisms in MUO and potential triggers. New approaches to therapy might then become more apparent, in particular those that can address disease triggers rather than treat the end-stage of the disease as we may have been doing so far.
NJ: format of article, writing manuscript, and literature search and analysis of published data, Table 2. NG: writing the manuscript and literature search and analysis of published literature, Table 1 and Figure 1. NJ and NG contributed to and approved the final manuscript, figure, and tables.
Figure 1 originates from work by NG and Prof. Linda Wooldridge funded by the Langford Trust.
The authors declare that the research was conducted in the absence of any commercial or financial relationships that could be construed as a potential conflict of interest.
All claims expressed in this article are solely those of the authors and do not necessarily represent those of their affiliated organizations, or those of the publisher, the editors and the reviewers. Any product that may be evaluated in this article, or claim that may be made by its manufacturer, is not guaranteed or endorsed by the publisher.
CNS, central nervous system; CSF, cerebrospinal fluid; EAE, experimental autoimmune/allergic encephalomyelitis; GCs, glucocorticoids; MS, multiple sclerosis; MRI, magnetic resonance imaging; MUO, meningoencephalomyelitis of unknown origin.
1. Koestner A, Zeman W. Primary reticulosis of the central nervous system in dogs. Am J Vet Res. (1962) 23:381–93.
2. Fankhauser R, Fatzer R, Luginbühl H. Reticulosis of the central nervous system (CNS) in dogs. Adv Vet Sci Comp Med. (1972) 16:35–71.
3. Russo ME. Primary reticulosis of the central nervous system in dogs. J Am Vet Med Assoc. (1979) 174:492–500.
4. Vandevelde M. Primary reticulosis of the central nervous system. Vet Clin North Am Small Anim Pract. (1980) 10:57–63. doi: 10.1016/S0195-5616(80)50003-3
5. Braund KG, Vandevelde M, Walker TL, Redding RW. Granulomatous meningoencephalomyelitis in six dogs. J Am Vet Med Assoc. (1978) 172:1195–200.
6. Cordy DR. Canine granulomatous meningoencephalomyelitis. Vet Pathol. (1979) 16:325–33. doi: 10.1177/030098587901600306
8. Coates JR, Jeffery ND. Perspectives on meningoencephalomyelitis of unknown origin. Vet Clin North Am Small Anim Pract. (2014) 44:1157–85. doi: 10.1016/j.cvsm.2014.07.009
9. Uchida K, Park E, Tsuboi M, Chambers JK, Nakamyama H. Pathological and immunological features of canine necrotizing meningoencephalitis and granulomatous meningoencephalitis. Vet J. (2016) 213:72–7. doi: 10.1016/j.tvjl.2016.05.002
10. Nessler JN, Oevermann A, Schawacht M, Gerhauser I, Spitzbarth I, Bittermann S, et al. Concomitant necrotizing encephalitis and granulomatous meningoencephalitis in four toy breed dogs. Front Vet Sci. (2022) 9:957285. doi: 10.3389/fvets.2022.957285
11. Sarfaty D, Carrillo JM, Greenlee PG. Differential diagnosis of granulomatous meningoencephalomyelitis, distemper, and suppurative meningoencephalitis in the dog. J Am Vet Med Assoc. (1986) 188:387–92.
12. Arenas M, Sabater S, Hernández V, Rovirosa A, Lara PC, Biete A, et al. Anti-inflammatory effects of low-dose radiotherapy. Indications, dose, and radiobiological mechanisms involved. Strahlenther Onkol. (2012) 188:975–81. doi: 10.1007/s00066-012-0170-8
13. Muñana KR, Luttgen PJ. Prognostic factors for dogs with granulomatous meningoencephalomyelitis: 42 cases (1982–1996). J Am Vet Med Assoc. (1998) 212:1902–6.
14. Dinse GE, Parks CG, Weinberg CR, Co CA, Wilkerson J, Zeldin DC, et al. Increasing prevalence of antinuclear antibodies in the United States. Arthritis Rheumatol. (2022) 74:2032–41. doi: 10.1002/art.42330
15. Ehrensperger F. Autoimmune diseases in dogs and their impact for breeding programs with special reference of the Nova Scotia Duck Tolling Retriever. Schweiz Arch Tierheilkd. (2018) 160:711–7. doi: 10.17236/sat00186
16. Cavanagh JJ, Levy M. Differential diagnosis of multiple sclerosis. Presse Med. (2021) 50:104092. doi: 10.1016/j.lpm.2021.104092
17. Filippi M, Bar-Or A, Piehl F, Preziosa P, Solari A, Vukusic S, et al. Multiple sclerosis. Nat Rev Dis Primers. (2018) 4:43. doi: 10.1038/s41572-018-0041-4
18. Kimoff RJ, Kaminska M, Trojan D. Multiple sclerosis and related disorders. Handb Clin Neurol. (2022) 189:177–200. doi: 10.1016/B978-0-323-91532-8.00013-6
19. Lane J, Ng HS, Poyser C, Lucas RM, Tremlett H. Multiple sclerosis incidence: a systematic review of change over time by geographical region. Mult Scler Relat Disord. (2022) 63:103932. doi: 10.1016/j.msard.2022.103932
20. Smith SM, Westermeyer HD, Mariani CL, Gilger BC, Davidson MG. Optic neuritis in dogs: 96 cases (1983–2016). Vet Ophthalmol. (2018) 21:442–51. doi: 10.1111/vop.12528
21. Meca-Lallana V, Gascón-Giménez F, Ginestal-López RC, Higueras Y, Téllez-Lara N, Carreres-Polo J, et al. Cognitive impairment in multiple sclerosis: diagnosis and monitoring. Neurol Sci. (2021) 42:5183–93. doi: 10.1007/s10072-021-05165-7
22. Prümmer JK, Stein VM, Marti E, Lutterotti A, Jelcic I, Schüpbach-Regula G, et al. Assessment of oligoclonal bands in cerebrospinal fluid and serum of dogs with meningoencephalitis of unknown origin. PLoS ONE. (2023) 18:e0280864. doi: 10.1371/journal.pone.0280864
23. Greer KA, Wong AK, Liu H, Famula TR, Pedersen NC, Ruhe A, et al. Necrotizing meningoencephalitis of Pug dogs associates with dog leukocyte antigen class II and resembles acute variant forms of multiple sclerosis. Tissue Antigens. (2010) 76:110–8. doi: 10.1111/j.1399-0039.2010.01484.x
24. Kegler K, Spitzbarth I, Imbschweiler I, Wewetzer K, Baumgärtner W, Seehusen F. Contribution of Schwann cells to remyelination in a naturally occurring canine model of CNS neuroinflammation. PLoS ONE. (2015) 10:e0133916. doi: 10.1371/journal.pone.0133916
25. Constantinescu CS, Farooqi N, O'Brien K, Gran B. Experimental autoimmune encephalomyelitis (EAE) as a model for multiple sclerosis (MS). Br J Pharmacol. (2011) 164:1079–106. doi: 10.1111/j.1476-5381.2011.01302.x
26. Wahren-Herlenius M, Dörner T. Immunopathogenic mechanisms of systemic autoimmune disease. Lancet. (2013) 382:819–31. doi: 10.1016/S0140-6736(13)60954-X
27. Bjornevik K, Cortese M, Healy BC, Kuhle J, Mina MJ, Leng Y, et al. Longitudinal analysis reveals high prevalence of Epstein-Barr virus associated with multiple sclerosis. Science. (2022) 375:296–301. doi: 10.1126/science.abj8222
28. Lanz TV, Brewer RC, Ho PP, Moon J-S, Jude KM, Fernandez D, et al. Clonally expanded B cells in multiple sclerosis bind EBV EBNA1 and Glial CAM. Nature. (2022) 603:321–7. doi: 10.1038/s41586-022-04432-7
29. Schatzberg SJ, Li Q, Porter BF, Barber RM, Claiborne MK, Levine JM, et al. Broadly reactive pan-paramyxovirus reverse transcription polymerase chain reaction and sequence analysis for the detection of Canine distemper virus in a case of canine meningoencephalitis of unknown etiology. J Vet Diagn Invest. (2009) 21:844–9. doi: 10.1177/104063870902100613
30. Barber RM, Li Q, Diniz PP, Porter BF, Breitschwerdt EB, Claiborne MK, et al. Evaluation of brain tissue or cerebrospinal fluid with broadly reactive polymerase chain reaction for Ehrlichia, Anaplasma, spotted fever group Rickettsia, Bartonella, and Borrelia species in canine neurological diseases (109 cases). J Vet Intern Med. (2010) 24:372–8. doi: 10.1111/j.1939-1676.2009.0466.x
31. Barber RM, Porter BF, Li Q, May M, Claiborne MK, Allison AB, et al. Broadly reactive polymerase chain reaction for pathogen detection in canine granulomatous meningoencephalomyelitis and necrotizing meningoencephalitis. J Vet Intern Med. (2012) 26:962–8. doi: 10.1111/j.1939-1676.2012.00954.x
32. Han JI, Chang DW, Na KJ. A multiplex quantitative real-time polymerase chain reaction panel for detecting neurologic pathogens in dogs with meningoencephalitis. J Vet Sci. (2015) 16:341–7. doi: 10.4142/jvs.2015.16.3.341
33. Hoon-Hanks LL, McGrath S, Tyler KL, Owen C, Stenglein MD. Metagenomic Investigation of Idiopathic Meningoencephalomyelitis in Dogs. J Vet Intern Med. (2018) 32:324–30. doi: 10.1111/jvim.14877
34. Collinet A, Garcia G, Wellehan J, Childress A, Carrera-Justiz S. Investigation of astrovirus and bornavirus in the cerebrospinal fluid of dogs clinically diagnosed with meningoencephalitis of unknown etiology. J Vet Intern Med. (2020) 34:232–6. doi: 10.1111/jvim.15677
35. Nessler JN, Jo WK, Osterhaus ADME, Ludlow M, Tipold A. Canine meningoencephalitis of unknown origin-the search for infectious agents in the cerebrospinal fluid via deep sequencing. Front Vet Sci. (2021) 8:645517. doi: 10.3389/fvets.2021.645517
36. Barber RM, Li Q, Levine JM, Ruone SJ, Levine GJ, Kenny P, et al. Screening for viral nucleic acids in the cerebrospinal fluid of dogs with central nervous system inflammation. Front Vet Sci. (2022) 9:850510. doi: 10.3389/fvets.2022.850510
37. Jeffery U. Urban environment: a risk factor for canine immune-mediated disease? J Small Anim Pract. (2017) 58:639–44. doi: 10.1111/jsap.12724
38. Granger N, Smith PM, Jeffery ND. Clinical findings and treatment of non-infectious meningoencephalomyelitis in dogs: a systematic review of 457 published cases from 1962 to 2008. Vet J. (2010) 184:290–7. doi: 10.1016/j.tvjl.2009.03.031
39. Barber RM, Schatzberg SJ, Corneveaux JJ, Allen AN, Porter BF, Pruzin JJ, et al. Identification of risk loci for necrotizing meningoencephalitis in pug dogs. J Hered. (2011) 102:Suppl 1:S40–6. doi: 10.1093/jhered/esr048
40. Schrauwen I, Barber RM, Schatzberg SJ, Siniard AL, Corneveaux JJ, Porter BF, et al. Identification of novel genetic risk loci in maltese dogs with necrotizing meningoencephalitis and evidence of a shared genetic risk across toy dog breeds. PLoS ONE. (2014) 9:e112755. doi: 10.1371/journal.pone.0112755
41. Kaczmarska A, José-López R, Czopowicz M, Lazzerini K, Leblond G, Stalin C, et al. Postencephalitic epilepsy in dogs with meningoencephalitis of unknown origin: clinical features, risk factors, and long-term outcome. J Vet Intern Med. (2020) 34:808–20. doi: 10.1111/jvim.15687
42. Paušová TK, Tomek A, Šrenk P, Belašková S. Clinical presentation, diagnostic findings, and long-term survival time in 182 dogs with meningoencephalitis of unknown origin from Central Europe that were administered glucocorticosteroid monotherapy. Top Companion Anim Med. (2021) 44:100539. doi: 10.1016/j.tcam.2021.100539
43. Cornelis I, Volk HA, Van Ham L, De Decker S. Prognostic factors for 1-week survival in dogs diagnosed with meningoencephalitis of unknown aetiology. Vet J. (2016) 214:91–5. doi: 10.1016/j.tvjl.2016.05.008
44. Zarfoss M, Schatzberg S, Venator K, Cutter-Schatzberg K, Cuddon P, Pintar J, et al. Combined cytosine arabinoside and prednisone therapy for meningoencephalitis of unknown aetiology in 10 dogs. J Small Anim Pract. (2006) 47:588–95. doi: 10.1111/j.1748-5827.2006.00172.x
45. Thomas JB, Eger C. Granulomatous meningoencephalomyelitis in 21 dogs. J Small Anim Pract. (1989) 30:287–93. doi: 10.1111/j.1748-5827.1989.tb01558.x
46. Cornelis I, Van Ham L, Gielen I, De Decker S, Bhatti SFM. Clinical presentation, diagnostic findings, prognostic factors, treatment and outcome in dogs with meningoencephalomyelitis of unknown origin: a review. Vet J. (2019) 244:37–44. doi: 10.1016/j.tvjl.2018.12.007
47. Nuhsbaum MT, Powell CC, Gionfriddo JR, Cuddon PA. Treatment of granulomatous meningoencephalomyelitis in a dog. Vet Ophthalmol. (2002) 5:29–33. doi: 10.1046/j.1463-5224.2002.00215.x
48. Cuddon PA, Coates JR, Murray M. New treatments for granulomatous meningoencephalomyelitis. In: Proc 20th ACVIM Forum. Greenwood Village, CO: American College of Veterinary Internal Medicine (2002). p. 319–21.
49. Mercier M, Barnes Heller HL. Efficacy of glucocorticoid monotherapy for treatment of canine meningoencephalomyelitis of unknown etiology: a prospective study in 16 dogs. Vet Med Sci. (2015) 1:16–22. doi: 10.1002/vms3.4
50. Lawn RW, Harcourt-Brown TR. Risk factors for early death or euthanasia within 100 days of diagnosis in dogs with meningoencephalomyelitis of unknown origin. Vet J. (2022) 287:105884. doi: 10.1016/j.tvjl.2022.105884
51. Barnoon I, Shamir MH, Aroch I, Bdolah-Abram T, Srugo I, Konstantin L, et al. Retrospective evaluation of combined mycophenolate mofetil and prednisone treatment for meningoencephalomyelitis of unknown etiology in dogs: 25 cases (2005–2011). J Vet Emerg Crit Care. (2016) 26:116–24. doi: 10.1111/vec.12399
52. Woolcock AD, Wang A, Haley A, Kent M, Creevy KE, Platt SR. Treatment of canine meningoencephalomyelitis of unknown aetiology with mycophenolate mofetil and corticosteroids: 25 cases (2007–2012). Vet Med Sci. (2016) 2:125–35. doi: 10.1002/vms3.22
53. Song JH, Yu DH, Lee HC, Hwang TS, Kim YJ, An SJ, et al. Evaluation of treatment with a combination of mycophenolate mofetil and prednisolone in dogs with meningoencephalomyelitis of unknown etiology: a retrospective study of 86 cases (2009–2017). BMC Vet Res. (2020) 16:192. doi: 10.1186/s12917-020-02414-3
54. Lowrie M, Smith PM, Garosi L. Meningoencephalitis of unknown origin: investigation of prognostic factors and outcome using a standard treatment protocol. Vet Rec. (2013) 172:527. doi: 10.1136/vr.101431
55. Lowrie M, Thomson S, Smith P, Garosi L. Effect of a constant rate infusion of cytosine arabinoside on mortality in dogs with meningoencephalitis of unknown origin. Vet J. (2016) 213:1–5. doi: 10.1016/j.tvjl.2016.03.026
56. Stee K, Broeckx BJG, Targett M, Gomes SA, Lowrie M. Cytosine arabinoside constant rate infusion without subsequent subcutaneous injections for the treatment of dogs with meningoencephalomyelitis of unknown origin. Vet Rec. (2020) 187:e98. doi: 10.1136/vr.106019
57. Barber R, Downey Koos L. Treatment with cytarabine at initiation of therapy with cyclosporine and glucocorticoids for dogs with meningoencephalomyelitis of unknown origin is not associated with improved outcomes. Front Vet Sci. (2022) 9:925774. doi: 10.3389/fvets.2022.925774
58. Flegel T, Boettcher IC, Matiasek K, Oevermann A, Doherr MG, Oechtering G, et al. Comparison of oral administration of lomustine and prednisolone or prednisolone alone as treatment for granulomatous meningoencephalomyelitis or necrotizing encephalitis in dogs. J Am Vet Med Assoc. (2011) 238:337–45. doi: 10.2460/javma.238.3.337
59. Pakozdy A, Leschnik M, Kneissl S, Gumpenberger M, Gruber A, Tichy A, et al. Improved survival time in dogs with suspected GME treated with ciclosporin. Vet Rec. (2009) 164:89–90. doi: 10.1136/vr.164.3.89
60. Brady SL, Woodward AP, le Chevoir M. Survival time and relapse in dogs with meningoencephalomyelitis of unknown origin treated with prednisolone and ciclosporin: a retrospective study. Aust Vet J. (2020) 98:491–8. doi: 10.1111/avj.12994
61. Wong MA, Hopkins AL, Meeks JC, Clarke JD. Evaluation of treatment with a combination of azathioprine and prednisone in dogs with meningoencephalomyelitis of undetermined etiology: 40 cases (2000–2007). J Am Vet Med Assoc. (2010) 237:929–35. doi: 10.2460/javma.237.8.929
62. Cornelis I, Volk HA, Van Ham L, De Decker S. Clinical presentation, diagnostic findings and outcome in dogs diagnosed with presumptive spinal-only meningoen-cephalomyelitis of unknown origin. J Small Anim Pract. (2017) 58:174–82. doi: 10.1111/jsap.12622
63. Menaut P, Landart J, Behr S, Lanore D, Trumel C. Treatment of 11 dogs with meningoencephalomyelitis of unknown origin with a combination of prednisolone and cytosine arabinoside. Vet Rec. (2008) 162:241–5. doi: 10.1136/vr.162.8.241
64. Levitin HA, Foss KD, Li Z, Reinhart JM, Hague DW, Fan TM. Pharmacokinetics of a cytosine arabinoside subcutaneous protocol in dogs with meningoencephalomyelitis of unknown aetiology. J Vet Pharmacol Ther. (2021) 44:696–704. doi: 10.1111/jvp.12980
65. Mulder JK, Harrap KR. Cytosine arabinoside uptake by tumour cells in vitro. Eur J Cancer. (1975) 11:373–9. doi: 10.1016/0014-2964(75)90066-3
66. Pawlak A, Obmińska-Mrukowicz B, Zbyryt I, Rapak A. In vitro drug sensitivity in canine lymphoma. J Vet Res. (2016) 60:55–61. doi: 10.1515/jvetres-2016-0009
67. Balis FM, Poplack DG. Central nervous system pharmacology of antileukemic drugs. Am J Pediatr Hematol Oncol. (1989) 11:74–86. doi: 10.1097/00043426-198921000-00017
68. Crook KI, Early PJ, Messenger KM, Muñana KR, Gallagher R, Papich MG. The pharmacokinetics of cytarabine in dogs when administered via subcutaneous and continuous intravenous infusion routes. J Vet Pharmacol Ther. (2013) 36:408–11. doi: 10.1111/jvp.12008
69. Pastina B, Early PJ, Bergman RL, Nettifee J, Maller A, Bray KY, et al. The pharmacokinetics of cytarabine administered subcutaneously, combined with prednisone, in dogs with meningoencephalomyelitis of unknown etiology. J Vet Pharmacol Ther. (2018) 41:638–43. doi: 10.1111/jvp.12667
70. Jones A, McGrath S, Gustafson DL. The pharmacokinetics of cytarabine administered at three distinct subcutaneous dosing protocols in dogs with meningoencephalomyelitis of unknown origin. J Vet Pharmacol Ther. (2019) 42:588–92. doi: 10.1111/jvp.12809
71. Mancini SL, Early PJ, Slater BM, Olby NJ, Mariani CL, Munana KR, et al. Novel subcutaneous cytarabine infusion with the Omnipod system in dogs with meningoencephalomyelitis of unknown etiology. Am J Vet Res. (2022) 83:ajvr.22.03.0046. doi: 10.2460/ajvr.22.03.0046
72. Sun L, Akiyama K, Zhang H, Yamaza T, Hou Y, Zhao S, et al. Mesenchymal stem cell transplantation reverses multiorgan dysfunction in systemic lupus erythematosus mice and humans. Stem Cells. (2009) 27:1421–32. doi: 10.1002/stem.68
73. Zeira O, Asiag N, Aralla M, Ghezzi E, Pettinari L, Martinelli L, et al. Adult autologous mesenchymal stem cells for the treatment of suspected non-infectious inflammatory diseases of the canine central nervous system: safety, feasibility and preliminary clinical findings. J Neuroinflamm. (2015) 12:181. doi: 10.1186/s12974-015-0402-9
74. Beckmann K, Carrera I, Steffen F, Golini L, Kircher PR, Schneider U, et al. A newly designed radiation therapy protocol in combination with prednisolone as treatment for meningoencephalitis of unknown origin in dogs: a prospective pilot study introducing magnetic resonance spectroscopy as monitor tool. Acta Vet Scand. (2015) 57:4. doi: 10.1186/s13028-015-0093-3
75. Genoni S, Palus V, Eminaga S, Cherubini GB. Safety of intrathecal administration of cytosine arabinoside and methotrexate in dogs and cats. Vet Comp Oncol. (2016) 14:331–6. doi: 10.1111/vco.12109
76. Groothuis DR, Benalcazar H, Allen CV, Wise RM, Dills C, Dobrescu C, et al. Comparison of cytosine arabinoside delivery to rat brain by intravenous, intrathecal, intraventricular and intraparenchymal routes of administration. Brain Res. (2000) 856:281–90. doi: 10.1016/S0006-8993(99)02089-2
77. Boller M, Nemanic TS, Anthonisz JD, Awad M, Selinger J, Boller EM, et al. The effect of pet insurance on presurgical euthanasia of dogs with gastric dilatation-volvulus: a novel approach to quantifying economic euthanasia in veterinary emergency medicine. Front Vet Sci. (2020) 7:590615. doi: 10.3389/fvets.2020.590615
78. Keegan S, Rose JH, Khan Z, Liebel FX. Low frequency of pre-treatment and post-treatment haematological abnormalities in dogs with non-infectious meningoencephalitis treated with cytosine arabinoside and prednisolone. Vet Rec Open. (2019) 6:e000315. doi: 10.1136/vetreco-2018-000315
79. Murphy T, Yee KWL. Cytarabine and daunorubicin for the treatment of acute myeloid leukemia. Expert Opin Pharmacother. (2017) 18:1765–80. doi: 10.1080/14656566.2017.1391216
80. Kantarjian H, Kadia T, DiNardo C, Daver N, E, et al. Acute myeloid leukemia: current progress and future directions. Blood Cancer J. (2021) 11:41. doi: 10.1038/s41408-021-00425-3
81. Löwenberg B, Pabst T, Vellenga E, van Putten W, Schouten HC, Graux C, et al. Cytarabine dose for acute myeloid leukemia. N Engl J Med. (2011) 364:1027–36. doi: 10.1056/NEJMoa1010222
82. Hauser SL, Cree BAC. Treatment of multiple sclerosis: a review. Am J Med. (2020) 133:1380–90.e2. doi: 10.1016/j.amjmed.2020.05.049
83. Gregory CR, Stewart A, Sturges B, DeManvelle T, Cannon A, Ortega T, et al. Leflunomide effectively treats naturally occurring immune-mediated and inflammatory diseases of dogs that are unresponsive to conventional therapy. Transplant Proc. (1998) 30:4143–8. doi: 10.1016/S0041-1345(98)01373-6
84. Vukmanović S, Mostarica Stojković M, Lukić ML. Experimental autoimmune encephalomyelitis in “low” and “high” interleukin 2 producer rats. I. Cellular basis of induction. Cell Immunol. (1989) 121:237–46. doi: 10.1016/0008-8749(89)90022-1
85. Stevens DB, Gold DP, Sercarz EE, Moudgil KD. The Wistar Kyoto (RT1(l)) rat is resistant to myelin basic protein-induced experimental autoimmune encephalomyelitis: comparison with the susceptible Lewis (RT1(l)) strain with regard to the MBP-directed CD4+ T cell repertoire and its regulation. J Neuroimmunol. (2002) 126:25–36. doi: 10.1016/S0165-5728(02)00045-0
86. Storch MK, Weissert R, Steffer A, Birnbacher R, Wallström E, Dahlman I, et al. MHC gene related effects on microglia and macrophages in experimental autoimmune encephalomyelitis determine the extent of axonal injury. Brain Pathol. (2002) 12:287–99. doi: 10.1111/j.1750-3639.2002.tb00443.x
87. Stanisavljević S, Lukić J, Momčilović M, Miljković M, Jevtić B, Kojić M, et al. Gut-associated lymphoid tissue, gut microbes and susceptibility to experimental autoimmune encephalomyelitis. Benef Microbes. (2016) 7:363–73. doi: 10.3920/BM2015.0159
88. Wolinsky JS, Arnold DL, Brochet B, Hartung HP, Montalban X, Naismith RT, et al. Long-term follow-up from the ORATORIO trial of ocrelizumab for primary progressive multiple sclerosis: a post-hoc analysis from the ongoing open-label extension of the randomised, placebo-controlled, phase 3 trial. Lancet Neurol. (2020) 19:998–1009. doi: 10.1016/S1474-4422(20)30342-2
89. Wolinsky JS, Narayana PA, O'Connor P, Coyle PK, Ford C, Johnson K, et al. Glatiramer acetate in primary progressive multiple sclerosis: results of a multinational, multicenter, double-blind, placebo-controlled trial. Ann Neurol. (2007) 61:14–24. doi: 10.1002/ana.21079
90. Rojas JI, Romano M, Ciapponi A, Patrucco L, Cristiano E. Interferon beta for primary progressive multiple sclerosis. Cochrane Database Syst Rev. (2009) 21:CD006643. doi: 10.1002/14651858.CD006643.pub2
91. Tur C, Montalban X, Tintoré M, Nos C, Río J, Aymerich FX, et al. Interferon β-1b for the treatment of primary progressive multiple sclerosis: five-year clinical trial follow-up. Arch Neurol. (2011) 68:1421–7. doi: 10.1001/archneurol.2011.241
92. Souza CP, Rosychuk RAW, Contreras ET, Schissler JR, Simpson AC. A retrospective analysis of the use of lokivetmab in the management of allergic pruritus in a referral population of 135 dogs in the western USA. Vet Dermatol. (2018) 29:489-e164. doi: 10.1111/vde.12682
93. Berer K, Mues M, Koutrolos M, Rasbi ZA, Boziki M, Johner C, et al. Commensal microbiota and myelin autoantigen cooperate to trigger autoimmune demyelination. Nature. (2011) 479:538–41. doi: 10.1038/nature10554
94. Luu M, Pautz S, Kohl V, Singh R, Romero R, Lucas S, et al. The short chain fatty acid pentanoate suppresses autoimmunity by modulating the metabolic-epigenetic crosstalk in lymphocytes. Nat Commun. (2019) 10:760. doi: 10.1038/s41467-019-08711-2
95. Jeffery ND, Barker AK, Alcott CJ, Levine JM, Meren I, Wengert J, et al. The association of specific constituents of the fecal microbiota with immune-mediated brain disease in dogs. PLoS ONE. (2017) 12:e0170589. doi: 10.1371/journal.pone.0170589
96. Miyake S, Kim S, Suda W, Oshima K, Nakamura M, Matsuoka T, et al. Dysbiosis in the gut microbiota of patients with multiple sclerosis, with a striking depletion of species belonging to clostridia XIVa and IV clusters. PLoS ONE. (2015) 10:e0137429. doi: 10.1371/journal.pone.0137429
97. Fuentes S, de Vos WM. How to manipulate the microbiota: Fecal microbiota transplantation. Adv Exp Med Biol. (2016) 902:143–53. doi: 10.1007/978-3-319-31248-4_10
98. Cold F, Baunwall SMD, Dahlerup JF, Petersen AM, Hvas CL, Hansen LH. Systematic review with meta-analysis: encapsulated faecal microbiota transplantation - evidence for clinical efficacy. Therap Adv Gastroenterol. (2021) 14:17562848211041004. doi: 10.1177/17562848211041004
99. Koblik PD, LeCouteur RA, Higgins RJ, Fick J, Kortz GD, Sturges BK, et al. Modification and application of a Pelorus Mark III stereotactic system for CT-guided brain biopsy in 50 dogs. Vet Radiol Ultrasound. (1999) 40:424–33. doi: 10.1111/j.1740-8261.1999.tb00370.x
100. Moissonnier P, Blot S, Devauchelle P, Delisle F, Beuvon F, Boulha L, et al. Stereotactic CT-guided brain biopsy in the dog. J Small Anim Pract. (2002) 43:115–23. doi: 10.1111/j.1748-5827.2002.tb00041.x
101. Rossmeisl JH, Andriani RT, Cecere TE, Lahmers K, LeRoith T, Zimmerman KL, et al. Frame-based stereotactic biopsy of canine brain masses: technique and clinical results in 26 cases. Front Vet Sci. (2015) 2:20. doi: 10.3389/fvets.2015.00020
102. Hanael E, Baruch S, Chai O, Nir Z, Rapoport K, Ruggeri M, et al. Detection of blood-brain barrier dysfunction using advanced imaging methods to predict seizures in dogs with meningoencephalitis of unknown origin. J Vet Intern Med. (2022) 36:702–12. doi: 10.1111/jvim.16396
103. Andersen-Ranberg E, Berendt M, Gredal H. Biomarkers of non-infectious inflammatory CNS diseases in dogs - where are we now? Part I: meningoencephalitis of unknown origin. Vet J. (2021) 273:105678. doi: 10.1016/j.tvjl.2021.105678
104. Mariani CL, Niman ZE, Boozer LB, Ruterbories LK, Early PJ, Muñana KR, et al. Vascular endothelial growth factor concentrations in the cerebrospinal fluid of dogs with neoplastic or inflammatory central nervous system disorders. J Vet Intern Med. (2021) 35:1873–83. doi: 10.1111/jvim.16181
105. Park J, Lee D, Yun T, Koo Y, Chae Y, Kim H, et al. Evaluation of the blood neutrophil-to-lymphocyte ratio as a biomarker for meningoencephalitis of unknown etiology in dogs. J Vet Intern Med. (2022) 36:1719–25. doi: 10.1111/jvim.16512
106. Song JH, Yu DH, Hwang TS, Seung BJ, Sur JH, Kim YJ, et al. Expression of platelet-derived growth factor receptor-α/ß, vascular endothelial growth factor receptor-2, c-Abl, and c-Kit in canine granulomatous meningoencephalitis and necrotizing encephalitis. Vet Med Sci. (2020) 6:965–74. doi: 10.1002/vms3.314
107. Rozental AJ, McGrath S, Mooney AP, Hinson SR, McKeon A, Pittock SJ, et al. Evaluation of cell-based and tissue-based immunofluorescent assays for detection of glial fibrillary acidic protein autoantibodies in the cerebrospinal fluid of dogs with meningoencephalitis of unknown origin and other central nervous system disorders. Am J Vet Res. (2021) 82:132–7. doi: 10.2460/ajvr.82.2.132
108. Yun T, Koo Y, Chae Y, Lee D, Kim H, Kim S, et al. Neurofilament light chain as a biomarker of meningoencephalitis of unknown etiology in dogs. J Vet Intern Med. (2021) 35:1865–72. doi: 10.1111/jvim.16184
109. Lake BB, Rossmeisl JH, Cecere J, Stafford P, Zimmerman KL. Immunosignature differentiation of non-infectious meningoencephalomyelitis and intracranial neoplasia in dogs. Front Vet Sci. (2018) 5:97. doi: 10.3389/fvets.2018.00097
110. Lepennetier G, Hracsko Z, Unger M, Van Griensven M, Grummel V, Krumbholz M, et al. Cytokine and immune cell profiling in the cerebrospinal fluid of patients with neuro-inflammatory diseases. J Neuroinflamm. (2019) 16:1–11. doi: 10.1186/s12974-019-1601-6
111. Arun T, Pattison L, Palace J. Distinguishing neurosarcoidosis from multiple sclerosis based on CSF analysis: a retrospective study. Neurology. (2020) 94:e2545–54. doi: 10.1212/WNL.0000000000009491
112. Nemecek A, Zimmermann H, Rübenthaler J, Fleischer V, Paterka M, Luessi F, et al. Flow cytometric analysis of T cell/monocyte ratio in clinically isolated syndrome identifies patients at risk of rapid disease progression. Mult Scler. (2016) 22:483–93. doi: 10.1177/1352458515593821
113. Hess AD, Colombani PM, Esa AH. Cyclosporine and the immune response: basic aspects. Crit Rev Immunol. (1986) 6:123–49.
114. Hasin Y, Seldin M, Lusis A. Multi-omics approaches to disease. Genome Biol. (2017) 18:83. doi: 10.1186/s13059-017-1215-1
115. Abboud H, Probasco JC, Irani S, Ances B, Benavides DR, Bradshaw M, et al. Autoimmune encephalitis: proposed best practice recommendations for diagnosis and acute management. J Neurol Neurosurg Psychiatry. (2021) 92:757–68. doi: 10.1136/jnnp-2020-325300
Keywords: meningoencephalomyelitis, dog, therapy, glucocorticoid, cytarabine
Citation: Jeffery N and Granger N (2023) New insights into the treatment of meningoencephalomyelitis of unknown origin since 2009: A review of 671 cases. Front. Vet. Sci. 10:1114798. doi: 10.3389/fvets.2023.1114798
Received: 02 December 2022; Accepted: 17 February 2023;
Published: 15 March 2023.
Edited by:
R. Timothy Bentley, Purdue University, United StatesReviewed by:
Hanne Gredal, University of Copenhagen, DenmarkCopyright © 2023 Jeffery and Granger. This is an open-access article distributed under the terms of the Creative Commons Attribution License (CC BY). The use, distribution or reproduction in other forums is permitted, provided the original author(s) and the copyright owner(s) are credited and that the original publication in this journal is cited, in accordance with accepted academic practice. No use, distribution or reproduction is permitted which does not comply with these terms.
*Correspondence: Nick Jeffery, bmplZmZlcnlAY3ZtLnRhbXUuZWR1
Disclaimer: All claims expressed in this article are solely those of the authors and do not necessarily represent those of their affiliated organizations, or those of the publisher, the editors and the reviewers. Any product that may be evaluated in this article or claim that may be made by its manufacturer is not guaranteed or endorsed by the publisher.
Research integrity at Frontiers
Learn more about the work of our research integrity team to safeguard the quality of each article we publish.