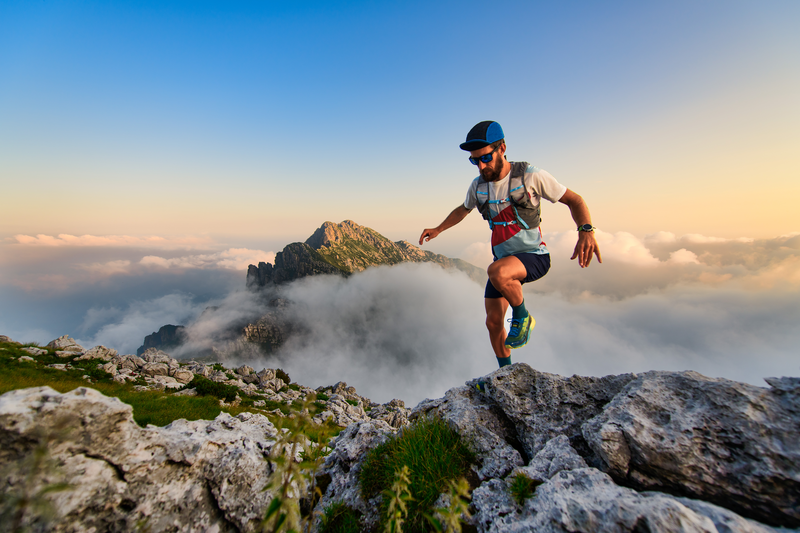
94% of researchers rate our articles as excellent or good
Learn more about the work of our research integrity team to safeguard the quality of each article we publish.
Find out more
ORIGINAL RESEARCH article
Front. Vet. Sci. , 30 March 2023
Sec. Animal Nutrition and Metabolism
Volume 10 - 2023 | https://doi.org/10.3389/fvets.2023.1098579
This article is part of the Research Topic Towards Plant-Based Foods: From Plant Biodiversity to Discovery of Sensorically Active Compounds View all 6 articles
Background: The effects of cinnamaldehyde, carvacrol and thymol complex (CCT) on the growth performance and intestinal function of piglets challenged with lipopolysaccharide (LPS) were determined. Colistin sulphate (CS) was as a positive control.
Method: Piglets (n = 24, 32 days of age) were allocated to four treatments: Control group (fed basal diet), LPS group (fed basal diet), CS+LPS group (fed basal diet + 50 mg/kg CS), and CCT+LPS group (fed basal diet + 50 mg/kg CCT).
Results: Results showed that diarrhea rates of piglets were significantly reduced by CCT and CS supplementation respectively. Further research showed that CS supplementation tended to improve the intestinal absorption function in LPS-challenged piglets. Moreover, CS supplementation significantly reduced the contents of cortisol in blood and malondialdehyde in the duodenum and the activities of inducible nitric oxide synthase in the duodenum and ileum and total nitric oxide synthase in the ileum in LPS-challenged piglets. CS supplementation significantly increased the activities of sucrase in the ileum and myeloperoxidase in the jejunum in LPS-challenged piglets. CS supplementation significantly alleviated the reduced mRNA levels of immune-related genes (IL-4, IL-6, IL-8, IL-10) in mesenteric lymph nodes and jejunum and mucosal growth-related genes (IGF-1, mTOR, ALP) in LPS-challenged piglets. These results suggested that CS supplementation improved the intestinal function in LPS-challenged piglets by improving intestinal oxidative stress, immune stress, and absorption and repair function. However, although CCT supplementation improved oxidative stress by reducing (p < 0.05) the content of malondialdehyde and the activity of nitric oxide synthase in the duodenum, CCT supplementation tended to aggravate the intestinal absorption dysfunction in LPS-challenged piglets. Furthermore, compared with the control and LPS groups, CCT supplementation remarkably elevated the content of prostaglandin in plasma and the mRNA levels of pro-inflammatory factor IL-6 in mesenteric lymph nodes and jejunum, and reduced the activity of maltase in the ileum in LPS-challenged piglets. These results suggested that CCT supplementation had a negative effect on intestinal function by altering intestinal immune stress response and reducing disaccharidase activity in LPS-challenged piglets.
Conclusions: Compared to CS, CCT supplementation exhibited a negative effect on intestinal function, suggesting whether CCT can be as an effective feed additive still needs further study.
Piglet feeding is one of the most critical parts of modern pig industry (1). However, piglets have a low immunity and an underdeveloped intestinal system, and piglets are prone to various stress responses (2). Moreover, the changes of dietary from easily digestible milk to less digestible solid feeds led to intestinal dysfunction in piglets and increased sensitivity of piglets to pathogenic microorganisms (3). Therefore, weaning leads to high morbidity and mortality in piglets. Although the use of antibiotics can significantly reduce the morbidity and mortality of piglets, a series of side effects such as drug resistance and drug residues also appear (4). The European Union and other countries banned the use of antibiotic growth-promoting agents in 2006 (5). Subsequently, China banned the use of antibiotic growth-promoting agents in 2020 (6). Faced with a series of health problems in piglet farming after the ban on antibiotics, it is crucial for swine industry to develop high-quality, safe and efficient additives that can replace antibiotics.
In recent years, natural plant extracts have been widely studied as potential feed additives (7). Among them, plant essential oils were the most widely studied and used (8). Previous studies have shown that plant essential oils supplemented in diet can enhance the growth performance of piglets by increasing feed intake, promoting digestion, exerting antibacterial, anti-inflammatory and antioxidant effects, and enhancing immune functions (9, 10).
Oleum cinnamomi, oregano and thyme oil were widely studied in feed production. Oleum cinnamomi has been reported to exhibit potential antibacterial activity and metabolic-modulating effects (11, 12) And Oleum cinnamomi can protect the intestine from damage under conditions of oxidative stress, inflammation, and infections (13). Cinnamaldehyde as a potent inhibitor is the predominant bioactive compound in oleum cinnamomi, which can inhibit filamentous molds, bacteria, and yeast (14). Cinnamaldehyde has been regarded as a potent antibiotic alternative in livestock production. Previous studies showed that cinnamaldehyde increased the antioxidant activity of rat kidneys by increasing the activity of the antioxidant enzyme (15). Cinnamaldehyde added to the diet may reduce the effects of stress and promote intake in feedlot cattle particularly early in the feeding period (16). Cinnamaldehyde also plays an anti-inflammatory role in Helicobacter pylori-induced gastric inflammation (17). Oregano has also been reported to exhibit potential antibacterial activity and antioxidant properties (18). Carvacrol, as the predominant bioactive in oregano, has antimicrobial, antioxidant, anti-inflammatory, anti-osteoclastic, and anti-diabetic properties combined with magnolol (19). Thyme oil has also been reported to exhibit potential antibacterial activity (18). Carvacrol and thymol, as the predominant bioactive in thyme oil, can improve absorption capacity by enhancing the digestive enzyme activity. And carvacrol and thymol also contribute to the relief from pathogen pressure by stimulating intestinal mucus production (20). Furthermore, previous studies have been reported that carvacrol and thymol supplementation promoted growth and improved meat and egg quality through metabolic-modulating, anti-oxidative, anti-inflammatory, and antimicrobial effects in poultry production (21). These 3 plant extracts have been reported to alter membrane permeability to hydrogen ions and reduce the number of pathogens in the intestinal tract (22).
In view of the good effect of cinnamaldehyde, carvacrol and thymol in metabolic-modulating, anti-oxidative, anti-inflammatory, and antimicrobial effects, whether the combination of cinnamaldehyde, carvacrol and thymol can better promote animal growth has not been studied yet. To evaluate the effects of dietary supplementation with cinnamaldehyde, carvacrol and thymol complex (CCT) on growth performance and intestinal function in weaned piglets, a model of piglet intestinal injury induced by lipopolysaccharide (LPS) stimulation was used in this study. LPS, as an immune stressor, can disrupt bowel function by affecting the expression of genes related to intestinal immune responses, growth, absorption, mucosal energy metabolism, and mucosal barrier function (23). The model of intestinal injury induced by LPS stimulation has been widely used to screen antibiotic substitutes (24–27). To further evaluate whether CCT can be used as a potential antibiotic substitute in feed, colistin sulfate (CS), as an antibiotic growth promoter which has been extensively used as a feed additive to reduce pathogen infections and improve growth performance after weaning (28, 29), was served as a positive control in this study.
Therefore, this study investigated the effects of dietary supplementation with CCT on the growth performance and intestinal function in weaned piglets challenged by LPS.
In the present study, animal experiment was approved by the Institutional Animal Care and Use Committee at Wuhan Polytechnic University (2014-0514, May 10, 2014). All animal experiments were performed following the guidelines of the Research Ethics Committee of the College of Animal Science and Nutritional Engineering, Wuhan Polytechnic University. The animal experiments were also conducted in accordance with relevant guidelines and regulations. Twenty-four crossbred healthy female piglets (Duroc × Landrace × Yorkshire) were weaned after 21 days after birth. After 11 days of environmental adaptation, piglets (32 days old, 7.29 ± 0.77 kg) were housed individually in stainless steel metabolic cages (1.20 × 1.10m2), and environmental temperature was maintained at 22–25°C (30). Food and water can be freely obtained by piglets. The corn and soybean meal-based diet was formulated to meet National Research Council's (31) recommended requirements for all nutrients [National Research Council. Nutrient Requirements of pigs. National Academic Press: Washington, DC (2012)]. During the 11-day adaptation period, all piglets were fed the basal diet.
At 32 days old (set as the day 0 of the experiment), all piglets were divided freely into one of the four groups: (1) Control group (fed the basal diet and sterile saline was injected intraperitoneally); (2) LPS group (fed the basal diet and LPS was injected intraperitoneally); (3) Colistin sulfate (CS)+LPS group (fed the basal diet supplemented with 50 mg/kg CS and LPS was injected intraperitoneally); (4) cinnamaldehyde, carvacrol and thymol complex (CCT)+LPS group (fed basal diet supplemented with 50 mg/kg CCT complex and LPS was injected intraperitoneally).
The trial period was 21 days (day 0 to 20 days). The growth performance of piglets was statistically analyzed by recording the body weight (BW), feed intake and diarrhea rates (DR). The blood samples were gathered from the anterior vena cava of 12-h-fasted pigs into heparinized vacuum tubes (Becton Dickinson Vacutainer System) on day 21 of the trial, the collected blood samples were used for hematological evaluation and separating plasma by centrifuging (3,500 × g for 10 min at 4°C) (13). Subsequently, overnight fasted piglets in the LPS, CS+LPS and CCT+LPS groups were intraperitoneally injected with LPS (100 μg/kg BW, Escherichia coli serotype 055: B5; Sigma Chemical Inc., St. Louis, MO, USA), whereas those in the control group were intraperitoneally injected with the same volume of sterile saline (30). To determine intestinal absorptive function, all piglets was orally administrated D-xylose with the dose of 0.1 g/kg BW at 2 h post LPS challenge (32). At 3h after LPS challenge, blood samples were gathered and stored at −80°C until analysis. At 6h after LPS challenge, all piglets were killed under anesthesia with an intravenous injection of pentobarbital sodium (50 mg/kg BW), and then intestinal samples were collected.
In addition, cinnamaldehyde (St. Louis, MO, USA; Cat. W228613; CAS.8007-80-5), carvacrol (CAS.499-75-2) and thymol (CAS.89-83-8) were purchased from Sigma-Aldrich Chemicals. The dosage of 50 mg/kg CCT was chosen according to our previous study by Wang et al. (13). The proportion of the complex is determined according to the content of the main effective substances in Oleum cinnamomi, oregano oil and thyme oil (1:1:1).
The whole gastrointestinal tracts of piglets were immediately exposed by quickly opening abdomen from the sternum to the pubis (32). The small intestine dissected free of the mesentery was placed on a stainless steel tray (< 0°C). The different intestinal segments (10 cm) were collected from the distal duodenum, mid-jejunum and mid-ileum. And then the collected segments were opened longitudinally with scissors, and the exposed intestinal cavity surface was flushed with ice-cold PBS (30). A sterile glass microscope slide was used to scrape mucosal samples at 4°C, and then the scraped mucosal was rapidly frozen in liquid nitrogen and stored at −80°C until analysis. After piglets were killed, all samples were collected within 15 min.
The whole blood samples were analyzed by the ADVIA 2120i Hematology System (Siemens Healthcare Diagnostics, Deerfield, Illinois, USA) to determine hematology including the white blood cell (WBC), red blood cell (RBC), platelet (PLT), plateletcrit (PCT), hemoglobin (HGB), hematocrit (HCT), red blood cell distribution width (RDW), mean corpuscular volume (MCV), mean corpuscular hemoglobin (MCH), mean corpuscular hemoglobin concentration (MCHC), and mean platelet volume (MPV).
Plasma biochemical parameters, such as aspartate transaminase (AST), total bilirubin (TBIL), alanine transaminase (ALT), triacylglycerol (TG), blood urea nitrogen (BUN), and alkaline phosphatase (ALP), were determined by an automatic biochemical analyzer (HITACHI 7020, Japan).
The plasma D-xylose was measured as described by Yi et al. (33). Fifty microliters of plasma was added to 5 mL of the phloroglucinol color reagent solution (Sigma Chemical Inc., St. Louis, MO, USA) and then heated at 100°C for 4min. The samples were cooled at room temperature. A D-xylose standard solution was prepared by dissolving D-xylose in saturated benzoic acid (prepared in deionized water) to obtain 0 mM, 0.7 mM, 1.3 mM, and 2.6 mM, which were consequently added to the color reagent solution alongside the samples. The absorbance of samples and standard solutions at 554nm was determined by using a spectrophotometer (Model 6100, Jenway Ltd., Felsted, Dunmow, CM6 3LB, Essex, England, UK). The standard solution of 0 mmol/L D-xylose was regarded as blank.
The intestinal samples (~200 mg) were homogenized with cooling saline and then centrifuged at 2,500 rpm for 10 min at 4 °C to collect the supernatant fluid for further assays. The concentration of inducible nitric oxide synthase (iNOS), total nitric oxide synthase (tNOS), hydrogen peroxide (H2O2), catalase (CAT), glutathione peroxidase (GSH-Px), malondialdehyde (MDA) and superoxide dismutase (SOD) and so on in the intestinal mucosae were determined by using commercially available kits (Nanjing Jiancheng Bioengineering Institute, Nanjing, China).
Each frozen mucosal sample (~100 mg) was powdered under liquid nitrogen using a mortar and pestle, and then homogenized in Trizol buffer. Total RNA was extracted by using the TRIzol Reagent protocol (Invitrogen, Carlsbad, CA, USA). Total RNA was quantified at an OD of 260 nm using the NanoDrop® ND-1000A UV-VIS spectrophotometer (Thermo Scientific, Wilmington, DE, USA), and its purity was evaluated by determining the OD260/OD280 ratio. When samples showed an OD260/OD280 = 1.8–2.2, they could be considered 90–100% pure nucleic acids (34). The RNA integrity in each sample was evaluated by using 1% denatured agarose gel electrophoresis. When the sample RNA had a 28S/18S rRNA ratio≥1.8, RNA can be used for quantitative RT-PCR analysis (35). According to the manufacturer's instruction, cDNA was synthesized by using a PrimeScript® RT reagent kit with gDNA Eraser (Takara, Dalian, China) to reverse-transcribe total RNA. And then the synthesized cDNA was stored at −20°C for further analysis.
The primer pairs (Table 1) used to amplify cDNA fragments were used for qPCR as previously described by Yi et al. (34). The SYBR® Premix Ex Taq™ (Takara, Dalian, China) on an Applied Biosystems 7500 Fast Real-Time PCR System (Foster City, CA, USA) was used to perform qPCR. The reaction mixture (50 μL) included 25 μL of SYBR® Premix Ex Taq™ (2 × ), 4 μL of cDNA and 0.2 μM of each primer. Each sample was conducted in triplicate. The reaction conditions of qPCR (two-step amplification): 95°C for 30 s, followed by 40 cycles of 95°C for 5 s and 60°C for 31 s. A subsequent melting curve (9 °C for 15 s, 60°C for 1 min and 95°C for 15 s) with continuous fluorescence measurement and final cooling to room temperature was processed. The melting curves of the products and the size of the amplicons were used to evaluate the specificity of qPCR (36). The ribosomal protein L4 (RPL4) and glyceraldehyde-3-phosphate dehydrogenase (GAPDH) were as references in this study (37). Results were analyzed by 2−ΔΔCt method (38).
All data were showed as means ± SD and analyzed using one-way ANOVA. The normality and constant variance for data were tested using Levene's test. Differences among treatment means were determined using Duncan's post-hoc tests. All statistical analyses were performed using the SPSS 17.0 software (SPSS, Inc.). P < 0.05 was considered to indicate statistical significance.
Compared with the control group, dietary supplementation with CCT and CS significantly decreased the DRs of weaned piglets (Table 2). Neither CS nor CCT could affect the average daily feed intake (ADFI), average daily gain (ADG), and feed/gain (F/G) of weaned piglets (Table 2).
Compared with the control group, dietary supplementation with CCT significantly increased the activity of alanine transaminase (ALT) and the concentrations of cholesterol (CHOL), triacylglycerol (TG), blood urea nitrogen (BUN) and blood chlorine (CL). While dietary supplementation with CCT significantly decreased the concentration of glucose (GLU) and the activity of alkaline phosphatase (ALP) in plasma compared with those of the control group. Dietary supplementation with CS significantly increased the concentration of CL in plasma compared with that of the control group (Table 3). Moreover, compared with the control group, dietary supplementation with CCT significantly elevated the monocytic cell count (MONO), mean platelet volume (MPV) and plateletcrit (PCT) levels in the blood. Dietary CS supplementation significantly elevated (P < 0.05) PCT levels in the blood compared with that of the control group (Table 3).
Table 3. Effects of CCT supplementation on blood biochemical parameters and hematological parameters in piglets before LPS challenge.
Compared with the control group, LPS challenge obviously increased the concentrations of total bilirubin (TBLL), TG, BUN, creatinine (Crea) and CL, and gamma-glutamyl transferase (GGT) activity. LPS challenge significantly decreased the concentration of GLU in the plasma compared with that of the control group. However, dietary supplementation of CCT significantly increased aspartate transaminase (AST) activity and TG level in LPS-challenged weaned pigs. Dietary supplementation of CCT significantly decreased GLU concentration, as well as the activities of GGT and ALP in LPS-challenged weaned pigs (Table 4). Compared with the control group, LPS challenge significantly decreased the levels of white blood cell (WBC), neutrophil count (NEU), lymphocyte count (LYM), MONO, eosinophile count (EOS), basophilic leukocyte count (BASO), and platelet count (PLT). However, dietary supplementation of CCT significantly reduced the number of PLT in the blood in LPS-challenged weaned piglets (Table 4).
Table 4. Effects of CCT supplementation on blood biochemical parameters and hematological parameters in weaned piglets after LPS challenge.
Compared with the control group, LPS challenge significantly reduced the concentrations of D-Xylose in the plasma. Dietary supplementation with CS tended to reduce the decreased content of D-Xylose in the plasma in LPS-challenged weaned piglets. However, compared with the CS+LPS group, dietary supplementation with CCT obviously increased the decreased content of D-Xylose in the plasma in LPS-challenged weaned piglets (Table 5). Compared with the control group, LPS challenge obviously increased the concentrations of cortisol (COR) and prostaglandin (PG) E2 in plasma. Dietary supplementation with CS significantly reduced the increased concentration of COR in plasma in LPS-challenged weaned piglets. However, dietary supplementation with CCT significantly increased the content of PGE2 in the plasma in LPS-challenged weaned piglets (Table 5).
Table 5. CCT and CS supplementation on the concentrations of D-xylose, COR (Cortisol), and PGE2 (Prostaglandin E2) in the plasma of LPS-challenged piglets.
Compared with the control group, LPS challenge significantly increased the activity of sucrase in the duodenum (Figure 1A), while significantly reducing the activities of sucrase and alkaline phosphatase in the jejunum (Figure 1B), alkaline phosphatase in the ileum (Figure 1C) and sucrase in the colon (Figure 1D). Dietary supplementation with CS obviously reduced the activity of lactase in the jejunum (Figure 1B) and significantly increased the activity of sucrase in the ileum (Figure 1C). Dietary supplementation with CCT obviously reduced the activity of lactase in the jejunum (Figure 1B) and maltase in the ileum (Figure 1C), and increased the activity of sucrase in the colon (Figure 1D).
Figure 1. Effects of CCT and CS supplementation on the activities of intestinal disaccharidases in LPS-challenged piglets. The activities of maltase (p = 0.062), lactase (p = 0.882), sucrase (p = 0.004) and alkaline phosphatase (p = 0.006) in duodenum (A), the activities of maltase (p = 0.440), lactase (p = 0.002), sucrase (p = 0.003) and alkaline phosphatase (p = 0.006) in jejunum (B), the activities of maltase (p = 0.002), lactase (p = 0.922), sucrase (p = 0.005) and alkaline phosphatase (p = 0.010) in ileum (C), and the activities of maltase (p = 0.741), lactase (p = 0.418), sucrase (p = 0.099) and alkaline phosphatase (p = 0.417) in colon (D) in LPS-challenged piglets. Data are means ± SD, n = 6, CS, colistin sulfate; CCT, a complex of cinnamaldehyde, carvacrol and thymol. Differences among treatment means were determined using Duncan's post-hoc tests. a − cMeans within rows with different superscripts different (P < 0.05).
Compared with the control group, LPS challenge significantly increased the concentration of malondialdehyde (MDA) in the duodenum (Figure 2A) and the activities of total nitric oxide synthase (tNOS) in the ileum (Figure 2C), inducible nitric oxide synthase (iNOS) in the duodenum, jejunum and ileum (Figure 2D) and catalase (CAT) in the ileum (Figure 2E). LPS challenge obviously reduced the concentration of malondialdehyde (MDA) in the colon (Figure 2A) and the activity of myeloperoxidase (MPO) in the jejunum (Figure 2B) compared with those of the control group. Dietary supplementation with CS significantly reduced the concentration of malondialdehyde (MDA) in the duodenum (Figure 2A) and the activities of tNOS in the ileum (Figure 2C) and iNOS in the duodenum and ileum (Figure 2D) and CAT in the ileum (Figure 2E) in LPS-challenged weaned piglets. Dietary supplementation with CS obviously increased the activities of MPO in the jejunum (Figure 2B) and CAT in the colon (Figure 2E) in LPS-challenged weaned piglets. Dietary supplementation with CCT significantly reduced the concentration of MDA in the duodenum (Figure 2A) and the activities of iNOS in the duodenum (Figure 2D) and CAT in the colon (Figure 2E) in LPS-challenged weaned piglets. However, dietary supplementation with CCT significantly increased the concentration of MDA in the jejunum (Figure 2A) and the activities of MPO in the duodenum and jejunum (Figure 2B).
Figure 2. Effects of CCT and CS supplementation on intestinal redox status in LPS-challenged piglets. Data are means ± SD, n = 6, CS, colistin sulfate; CCT, a complex of cinnamaldehyde, carvacrol and thymol; malondialdehyde (MDA) in duodenum (p = 0.005), jejunum (p = 0.042), ileum (p = 0.077) and colon (p = 0.046) (A); myeloperoxidase (MPO) in duodenum (p = 0.055), jejunum (p = 0.001), ileum (p = 0.649) and colon (p = 0.192) (B); total nitric oxide synthase (tNOS) in duodenum (p = 0.914), jejunum (p = 0.099), ileum (p = 0.016) and colon (p = 0.965) (C); inducible nitric oxide synthase (iNOS) in duodenum (p < 0.001), jejunum (p = 0.037), ileum (p = 0.016) and colon (p = 0.015) (D); catalase (CAT) in duodenum (p < 0.048), jejunum (p = 0.049), ileum (p = 0.006) and colon (p = 0.001) (E); superoxide dismutase (SOD) in duodenum (p < 0.860), jejunum (p = 0.635), ileum (p = 0.640) and colon (p = 0.120) (F). Differences among treatment means were determined using Duncan's post-hoc tests. a, bMeans within rows with different superscripts differ (P < 0.05).
Compared with the control group, LPS challenge significantly decreased the mRNA levels of mucosal growth-related genes [mammalian target of rapamycin (mTOR) and alkaline phosphatase (ALP)], immune-related genes (IL-4, IL-6, IL-8, IL-10 and TNF-α) in the jejunum. Dietary supplementation with CS significantly increased the mRNA levels of mucosal growth-related genes (insulin-like growth factors-1 (IGF-1), mTOR and ALP), immune-related genes (IL-6 and IL-8) in LPS-challenged weaned piglets. Dietary supplementation with CS obviously reduced the mRNA levels of immune-related gene (IL-4) in the jejunum in LPS-challenged weaned piglets. Dietary supplementation with CCT significantly increased the immune-related genes (IL-6 and IL-8) in LPS-challenged weaned piglets. Dietary supplementation with CCT significantly reduced the immune-related gene (IL-4) in the jejunum in LPS-challenged weaned piglets. However, compared with the control group, dietary supplementation with CCT increased immune-related gene (IL-6) more than twice in the jejunum in LPS-challenged weaned piglets (Table 6).
Table 6. Effects of CCT supplementation on the mRNA levels of mucosal growth-related genes, immune-related genes and mucosal energy metabolism-related genes in the jejunum in piglets after LPS challenge.
Compared with the control group, LPS challenge obviously decreased the mRNA of immune-related genes (IL-4, IL-6, IL-8, IL-10, and TNF-α) in mesenteric lymph nodes in LPS-challenged weaned piglets. Dietary supplementation with CS significantly increased the mRNA levels of immune-related genes (IL-4, IL-6, IL-8, and IL-10) in mesenteric lymph nodes in LPS-challenged weaned piglets. Dietary supplementation with CCT also significantly increased the mRNA levels of immune-related genes (IL-4, IL-6, IL-8, and IL-10) in mesenteric lymph nodes in LPS-challenged weaned piglets. However, compared with the control group, dietary supplementation with CCT increased the immune-related gene (IL-6) by more than 1.7 in the jejunum in LPS-challenged weaned piglets (Table 7).
Table 7. Effects of CCT supplementation on the mRNA levels of immune related gene in mesenteric lymph nodes in piglets after LPS challenge.
To solve various problems encountered in weaned piglet feeding, a variety of green, safe and efficient antibiotic substitutes that can reduce the DR and increase the growth performance in weaned piglets have been studied. Dietary supplementation with 50 mg/kg oleum cinnamomi could increase ADFI and reduce DR in piglets (34). Yang et al. (16) found that 400 mg/d or 800 mg/d cinnamic aldehyde could increase feed intake, but have no effect on the average daily gain in feedlot cattle. Dietary supplementation with Lactobacillus casei decreased the F/G and DR in weaned piglets (35). N-Acetylcysteine supplemented in diet did not reduce DR and affect the growth performance in weaned piglets, but N-acetylcysteine improved intestinal function in LPS-challenged piglets (33). Glutamate precursor α-ketoglutarate supplemented in deity improved LPS-induced liver injury by improving energy metabolism and increasing anti-oxidative capacity in LPS-challenged young pigs (13). These studies suggested that potential antibiotic substitutes supplementation may play different roles in improving the growth performance of weaned piglets. In this study, our results showed that dietary supplementation with CCT reduced the DR of weaned piglets. Meanwhile, dietary supplementation with CS (as positive control) also reduced the DR of weaned piglets (Table 2). Previous study reported that CS probably will be the 'last-line' therapeutic drug against multidrug-resistant Gram-negative pathogens in the 21st century (39). CS, as an antibiotic growth promoter, had been broadly used as a growth-promoting agent in feed to improve growth performance and attenuate gastrointestinal infections in weaned piglets (28, 29, 40). However, CS as a feed additive has been banned to use in animal diets in China since May 2017 (41). Therefore, it is feasible and meaningful to use CS as a positive control to evaluate the effectiveness of novel alternatives of antibiotic feed additives. Our results showed that CCT supplementation exerted the same effect as CS in reducing the DR in weaned piglets, suggesting that CCT may be a promising alternative to antibiotic feed additives in piglets.
Although dietary supplementation with CCT reduced the DR in weaned piglets, it is still unclear the effects of CCT supplementation on physiological function and the ability to respond to external stimuli in weaned piglets. It is an important role for blood biochemical and blood cell indicators to reflect the status of health, cell permeability, and the level of metabolism (2). The changes in blood biochemical and blood cell indicators can reflect the function of multiple tissues and organs in the body. Plasma ALT, AST, TBIL, ALP and GGT activities are considered to be non-specific and specific markers for hepatic injury (42). Plasma Crea, CL and BUN levels can be associated with renal function (43). CHOL is involved in the stability of many cell membranes in the body, including the synthesis of sex hormones and vitamin D (44). Serum glucose concentrations can reflect hormone secretion levels in the body (2). Blood MONO can respond to the body's immune levels (2). Blood MPV and PCT are indicators that respond to hematologic diseases. In this study, compared with the control group, CCT supplementation significantly changed multiple blood biochemical parameters (ALT, CHOL, TG, BUN, CL, GLU and ALP) and hematological parameters (MONO, MPV and PCT), however, CS supplementation only changed blood biochemical parameters (CL) and hematological parameters (PCT) (Table 3). These results suggested that CCT supplementation may not be as good as CS in improving physiological function in weaned piglets.
The effects of CCT supplementation on blood biochemical and hematological parameters in LPS-challenged piglets were further evaluated. Consistent with previously reported results (2, 33, 35, 45) that LPS challenge changed the blood biochemical and hematological parameters in piglet, our results showed that blood biochemical parameters (TBLL, TG, BUN, CL, Crea, GLU, and GGT) and hematological parameters (WBC, NEU, LYM, MONO, EOS, BASO, and PLT) were significantly changed in LPS-challenged weaned piglets. Previous studies showed that the porcine liver function may be impaired based on the elevations in the activities of plasma AST and GGT under LPS stimulation (33, 35). The increases in AST and GGT activity in plasma may indicate liver damage by LPS challenge. TG is an important source of energy for the body, and when the TG in the body is too high, it will continue to accumulate fat in the liver, resulting in fatty liver (46). Blood GLU is the most direct source of energy for various life activities, the level of blood GLU concentration directly reflects the vitality of animals in the normal blood GLU range (47). LPS stimulation can cause the liver to be suppressed in the gluconeogenesis pathway, resulting in persistent hypoglycemia in vivo. The main function of PLT is coagulation and hemostasis, and it play an important role in the process of hemorrhagic coagulation (48). Our results showed that CS supplementation did not significantly alter the blood biochemical indicators and hematological parameters in piglets challenged with LPS stimulation (Table 4). While CCT supplementation significantly reduced the increase in GGT activity in LPS-challenged weaned piglets, CCT supplementation exacerbated changes in multiple indicators in LPS-challenged weaned piglets, including the decrease in GLU and PLT and the increase in ALT activity and TBLL levels. These results suggested that CCT supplementation may increase the susceptibility of weaned piglets to LPS challenge.
The above findings suggested that CCT supplementation may have a negative effect on body homeostasis in weaned piglets. However, how CCT supplementation reduced the DR in weaned piglets remains to be further studied. Consistent with previous reports that LPS challenge can induce intestinal oxidative stress in weaned piglets (2, 33, 35, 45), these results in this study showed that LPS challenge significantly increased the activities of tNOS and iNOS in the ileum and iNOS in the duodenum and jejunum and the concentration of MDA in the duodenum. MDA is usually used to assess the degree of damage to the cell membranes (2). MPO is an important iron-containing lysosome in neutrophils and monocytes, and plays an important role in the body's immune defense process (2). Inducible nitric oxide synthase (iNOS) delivers a great amount of NO compared to the constitutive isoforms of NOS (49). Physiological concentrations of NO play a crucial role in the maintenance of intestinal mucosal integrity, but pathological levels are detrimental to the gut (50, 51). CS supplementation ameliorated the adverse effects of LPS challenge on intestinal oxidative responses in weaned piglets (Figure 2). Furthermore, CCT supplementation also ameliorated the adverse effects of LPS challenge on intestinal oxidative responses in weaned piglets. These results suggested that CCT supplementation improved the antioxidant capacity in LPS-challenged weaned piglets. CCT supplementation may reduce the DR in piglets by increasing the antioxidant capacity.
Fifty to eighty percent of the ingredients in the animal diet are carbohydrates, and the energy required for animal growth mainly comes from carbohydrates (52–54). The carbohydrates in the diet are composed of polysaccharides, oligosaccharides, and monosaccharides (52). Only monosaccharides can be directly absorbed by the intestine (55). However, polysaccharides and oligosaccharides need to be decomposed into disaccharides by a series of enzymes, and then the disaccharides are then decomposed into monosaccharides by disaccharidases in the mucous membrane before they can be absorbed and utilized (33, 52–54, 56, 57). Therefore, disaccharidases play a key role in the absorption and utilization of carbohydrates. In this study, LPS challenge decreased the activities of sucrase in the jejunum and colon and the activities of alkaline phosphatase in the jejunum and ileum (Figure 1). These results suggested that LPS challenge reduced the intestinal function of piglets by reducing the activity of disaccharidases. CS supplementation increased the activities of sucrase in the jejunum and colon, suggesting that CS may improve intestinal function in LPS-challenged piglets. However, although CCT supplementation ameliorated the adverse effects of LPS challenge on sucrase activity in the colon, CCT supplementation exacerbated the LPS-induced decrease in the activity of maltase in the ileum. These results suggested that the effect of CCT supplementation on intestinal absorptive function needs to be further investigated.
The plasma D-xylose absorbed from the intestinal lumen is a useful indicator for intestinal absorption capacity and mucosal integrity (58, 59). D-xylose is readily absorbed by the jejunum in healthy piglets. However, under LPS stimulation or malabsorption, D-xylose is not absorbed by the intestine, thus reducing the D-xylose content in plasma (30). In this study, LPS challenge decreased the concentration of D-xylose in plasma, suggesting that LPS challenge reduced the intestinal absorption function (Table 5). Zhou et al. (60) found that cinnamaldehyde can improve absorption capacity in grass carp. Consistent with previously reported results, CS supplementation had a tendency to increase the content of D-xylose in plasma in LPS-challenged weaned piglets. However, CCT supplementation had a tendency to reduce the concentration of D-xylose in plasma in LPS-challenged weaned piglets. Meanwhile, CCT supplementation had no effect on the morphology and structure of the jejunum in LPS-challenged weaned piglets (data not shown). These results suggested that CCT supplementation exacerbated the decrease of intestinal absorption function in LPS-challenged weaned piglets, however, the mechanism remains unclear.
Combined with the above results, we speculated that CCT supplementation aggravated the decline in intestinal absorption function in LPS-challenged weaned piglets by altering the intestinal stress responses. Stress response, including activation of the sympathetic nervous system, glucocorticoid secretion, and changes in emotional behaviors, is a state of physiological or psychological stress caused by adverse stimuli (61). COR is a glucocorticoid that is widely used as a biomarker for the detection of stress responses in pigs (62). PGE2 is involved in these stress responses mentioned above and it is released from various cell types depending on the type of stressors present and acts in the vicinity of its synthesis (61). Previous studies reported that LPS challenge significantly increased the concentrations of COR and PGE2 in plasma (54, 63). Consistent with previous reports, our results showed that LPS challenge increased the concentrations of COR and PGE2 in plasma in pigs, suggesting that LPS challenge elicited a stress response in piglets (Table 5). CS supplementation reduced the concentration of COR in plasma of LPS-challenged weaned piglets. However, CCT supplementation increased the concentration of PGE2 in plasma of LPS-challenged weaned piglets. These results indicated that CS supplementation alleviated the stress response in LPS-challenged weaned piglet, whereas CCT supplementation exacerbated the stress response in LPS-challenged weaned piglet by increasing the concentration of PGE2.
PGE2 is involved in various aspects of inflammation and immunity stress (61). PGE2, as a mediator, functions in immune inflammation (64). PGE2 as a key molecule regulates the activation, maturation, migration, and cytokine secretion of innate immune cells including natural killer cells, dendritic cells, neutrophils, and macrophages (65). In this study, CCT supplementation significantly increased the levels of pro-inflammatory factor IL-6 in the jejunum and mesenteric lymph nodes in LPS-challenged weaned piglets, suggesting that CCT supplementation may exacerbate the intestinal dysfunction by altering the immune stress response in LPS-challenged weaned piglets.
In addition, ALP in the intestine tract is associated with the proliferation and differentiation of intestinal epithelial cell, so ALP is regarded as a critical biomarker enzyme for changes in the primary digestive and absorptive functions of the small intestine (66). The mTOR signaling pathway plays a vital role in enterocyte growth, proliferation, and regeneration (67, 68), and thereby being conducive to the recovery of the small intestinal mucosa after damage (69, 70). The IGF-I plays a prominent role in regulating cell metabolism, proliferation, and differentiation (71, 72). Consistent with the previous study by Yi et al. (23), gene expressions of ALP, mTOR, and IGF-I in the jejunum were dramatically decreased by LPS challenge. In the study, CS significantly increased the mRNA levels of ALP, mTOR, and IGF-I in the jejunum in LPS-challenged weaned piglets, whereas CCT didn't affect mRNA levels for ALP, mTOR, and IGF-I in LPS-challenged piglets. These results indicated that CS improved jejunal mucosal growth in LPS-challenged weaned piglets, whereas CCT did not.
In conclusion, dietary supplementation with CCT reduced the DR in weaned piglets and alleviated intestinal oxidative stress in LPS-challenged weaned piglets. However, CCT supplementation tended to aggravate the intestinal absorption dysfunction in LPS-challenged weaned piglets by altering the immune stress response. Furthermore, CCT was less effective than CS in improving intestinal function in LPS-challenged weaned piglets. Therefore, our results suggest whether CCT can be as an effective feed additive still needs further study for weaned piglets.
The original contributions presented in the study are included in the article/supplementary material, further inquiries can be directed to the corresponding author.
The animal study was reviewed and approved by the Animal Care and Use Committee at Wuhan Polytechnic University (2014-0514, May 10, 2014).
YH designed the study and revised the manuscript. YZ, QL, and YD wrote and revised the manuscript. ZW, LW, DZ, and QL collected and analyzed experimental results. QL, DY, TW, and ZW participated in the discussion of results and the revision of the paper. All authors contributed to the data interpretation and approved the final version of the manuscript.
This work was jointly supported by National Natural Science Foundation of China (32172763 and 32072762) and Hubei Provincial Key R&D Program (2021BBA083).
We thank our students and technicians for their contributions to this research.
The authors declare that the research was conducted in the absence of any commercial or financial relationships that could be construed as a potential conflict of interest.
All claims expressed in this article are solely those of the authors and do not necessarily represent those of their affiliated organizations, or those of the publisher, the editors and the reviewers. Any product that may be evaluated in this article, or claim that may be made by its manufacturer, is not guaranteed or endorsed by the publisher.
1. Blavi L, Solà-Oriol D, Llonch P, López-Vergé S, Martín-Orúe SM, Pérez JF, et al. Management and feeding strategies in early life to increase piglet performance and welfare around weaning: a review. Animals. (2021) 11:302. doi: 10.3390/ani11020302
2. Zhang YY Yi D, Xu HW, Tan ZH, Meng YX, Wu T, et al. Dietary supplementation with sodium gluconate improves the growth performance and intestinal function in weaned pigs challenged with a recombinant Escherichia coli strain. BMC Vet Res. (2022) 18:303. doi: 10.1186/s12917-022-03410-5
3. Middelkoop A, van Marwijk MA, Kemp B, Bolhuis JE. Pigs like it varied; feeding behavior and pre- and post-weaning performance of piglets exposed to dietary diversity and feed hidden in substrate during lactation. Front Vet Sci. (2019) 6:408. doi: 10.3389/fvets.2019.00408
4. Zhao J, Zhang Z, Zhang S, Page G, Jaworski NW. The role of lactose in weanling pig nutrition: a literature and meta-analysis review. J Anim Sci Biotechnol. (2021) 12:10. doi: 10.1186/s40104-020-00522-6
5. Gallois M, Rothkötter, HJ, Bailey M, Stokes, CR, Oswald IP. Natural alternatives to in-feed antibiotics in pig production: can immunomodulators play a role? Animal. (2009) 3:1644–61. doi: 10.1017/S1751731109004236
6. Wang X, Wu D, Xuan Z, Wang W, Zhou X. The influence of a ban on outpatient intravenous antibiotic therapy among the secondary and tertiary hospitals in China. BMC Public Health. (2020) 20:1794. doi: 10.1186/s12889-020-09948-z
7. Durmic Z, Moate PJ, Eckard R, Revell DK, Williams R, Vercoe PE, et al. In vitro screening of selected feed additives, plant essential oils and plant extracts for rumen methane mitigation. J Sci Food Agric. (2014) 94:1191–6. doi: 10.1002/jsfa.6396
8. Akram M, Mu A, Jalal H. Essential oils as alternatives to chemical feed additives for maximizing livestock production. J Hellenic Vet Med Soc. (2021) 3:26741. doi: 10.12681./JHVMS.26741
9. Vigan M. Essential oils: renewal of interest and toxicity. Eur J Dermatol. (2010) 20:685–92. doi: 10.1684/ejd.2010.1066
10. Chen F, Liu Y, Zhu H, Hong Y, Wu Z, Hou Y, et al. Fish oil attenuates liver injury caused by, LPS, in weaned pigs associated with inhibition of TLR4 and nucleotide-binding oligomerization domain protein signaling pathways. Innate Immun. (2013) 19:504–15. doi: 10.1177/1753425912472003
11. Chang ST, Chen PF, Chang SC. Antibacterial activity of leaf essential oils and their constituents from Cinnamomum osmophloeum. J Ethnopharmacol. (2001) 77:123–7. doi: 10.1016/s0378-8741(01)00273-2
12. Nuryastuti T, van der Mei HC, Busscher HJ, Iravati S, Aman AT, Krom BP, et al. Effect of cinnamon oil on ica A expression and biofilm formation by staphylococcus epidermidis. Appl Environ Microbiol. (2009) 75:6850–5. doi: 10.1128/AEM.00875-09
13. Wang L, Hou Y, Yi D, Ding B, Zhao D, Wang Z, et al. Beneficial roles of dietary oleum cinnamomi in alleviating intestinal injury. Front Biosci. (2015) 20:814–28. doi: 10.2741/4339
14. Shreaz S, Wani WA, Behbehani JM, Raja V, Irshad M, Karched M, et al. (2016). Cinnamaldehyde and its derivatives, a novel class of antifungal agents. Fitoterapia. (2016) 112:116–31. doi: 10.1016/j.fitote.05016
15. Gowder SJT, Devaraj H. Effect of the food flavour cinnamaldehyde on the antioxidant status of rat kidney. Basic Clin Pharmacol Toxicol. (2006) 99:379–82. doi: 10.1111/j.1742-7843.2006.pto_560.x
16. Yang WZ, Ametaj BN, Benchaar C, He ML, Beauchemin KA. Cinnamaldehyde in feedlot cattle diets: Intake, growth performance, carcass characteristics, and blood metabolites. J Anim Sci. (2010) 88:1082–92. doi: 10.2527/jas.2008-1608
17. Muhammad JS, Zaidi SF, Shaharyar S, Refaat A, Usmanghani K, Saiki I, et al. Anti-inflammatory effect of cinnamaldehyde in Helicobacter pylori induced gastric inflammation. Biol Pharm Bull. (2015) 38:109–15. doi: 10.1248/bpb.b14-00609
18. Sakkas H, Papadopoulou C. Antimicrobial activity of basil, oregano, and thyme essential oils. J Microbiol Biotechnol. (2017) 27:429–38. doi: 10.4014/jmb.1608.08024
19. Cicalǎu GIP, Babes PA, Calniceanu H, Popa A, Ciavoi G, Iova GM, et al. Anti-inflammatory and antioxidant properties of carvacrol and magnolol, in periodontal disease and diabetes mellitus. Molecules. (2021) 26:6899. doi: 10.3390/molecules26226899
20. Mohammadi Gheisar M, Kim IH. Phytobiotics in poultry and swine nutrition—a review. Italian J Animal Sci. (2018) 17:92–99.
21. Kikusato, M. Phytobiotics to improve health and production of broiler chickens: functions beyond the antioxidant activity. Animal Biosci. (2021) 34:345. doi: 10.5713/ab.20.0842
22. Gholami-Ahangaran M, Ahmadi-Dastgerdi A, Azizi S, Basiratpour A, Zokaei M, Derakhshan M. Thymol and carvacrol supplementation in poultry health and performance. Vet Med Sci. (2022) 8:267–88. doi: 10.1002/vms3.663
23. Yi D, Hou Y, Wang L, Zhao D, Ding B, Wu T, et al. Gene expression profiles in the intestine of lipopolysaccharide-challenged piglets. Front Biosci. (2016) 21:487–501. doi: 10.2741/4404
24. Kang P, Xiao H, Hou Y, Ding B, Liu Y, Zhu H, et al. Effects of astragalus polysaccharides, achyranthes bidentata polysaccharides, and acantbepanax senticosus paponin on the performance and immunity in weaned pigs. Asian-Aust J Anim Sci. (2010) 23:750–56. doi: 10.5713/ajas.2010.90526
25. Sun KJ, Lei Y, Wang RJ, Wu ZL, Wu GY. Cinnamicaldehyde regulates the expression of tight junction proteins and amino acid transporters in intestinal porcine epithelial cells. J Anim Sci Biotechno. (2017) 8:66. doi: 10.1186/s40104-017-0186-0
26. Duan Y, Song B, Zheng C, Zhong Y, Guo Q, Zheng J, et al. Dietary beta-hydroxy beta-methyl butyrate supplementation alleviates liver injury in lipopolysaccharide-challenged piglets. Oxid Med Cell Longev. (2021) 2021:5546843. doi: 10.1155/2021/5546843
27. Zheng C, Song B, Duan Y, Zhong Y, Yan Z, Zhang S, et al. Dietary β-hydroxy-β-methylbutyrate improves intestinal function in weaned piglets after lipopolysaccharide challenge. Nutrition. (2020) 78:110839. doi: 10.1016/j.nut.2020.110839
28. Boyen F, Vangroenweghe F, Butaye P, De Graef E, Castryck F, Heylen P, et al. Disk prediffusion is a reliable method for testing colistin susceptibility in porcine E. coli strains. Vet Microbio. (2010) 144:359–62. doi: 10.1016/j.vetmic.01010
29. Pluske JR. Feed- and feed additives-related aspects of gut health and development in weanling pigs. J Anim Sci Biotechno. (2013) 4:1. doi: 10.1186/2049-1891-4-1
30. Hou Y, Wang L, Zhang W, Yang Z, Ding B, Zhu H, et al. Protective effects of, N-acetylcysteine on intestinal functions of piglets challenged with lipopolysaccharide. Amino Acids. (2012) 43:1233–42. doi: 10.1007/s00726-011-1191-9
32. Hou Y, Wang L, Ding B, Liu Y, Zhu H, Liu J, et al. Dietary α-ketoglutarate supplementation ameliorates intestinal injury in lipopolysaccharide-challenged piglets. Amino Acids. (2010) 39:555–64. doi: 10.1007/s00726-010-0473-y
33. Yi D, Hou Y, Xiao H, Wang L, Zhang Y, Chen H, et al. N-acetylcysteine improves intestinal function in lipopolysaccharides-challenged piglets through multiple signaling pathways. Amino Acids. (2017) 49:1915–29. doi: 10.1007/s00726-017-2389-2
34. Yi D, Fang Q, Hou Y, Wang L, Xu H, Wu T, et al. Dietary supplementation with oleum cinnamomi improves intestinal functions in piglets. Int J Mol Sci. (2018) 19:1284. doi: 10.3390/ijms19051284
35. Zhao D, Wu T, Yi D, Wang L, Li P, Zhang J, et al. Dietary supplementation with lactobacillus casei alleviates lipopolysaccharide-induced liver injury in a porcine model. Int J Mol Sci. (2017) 18:2535. doi: 10.3390/ijms18122535
36. Meurens F, Berri M, Auray G, Melo S, Levast B, Virlogeux-Payant I, et al. Early immune response following Salmonella enterica subspecies enterica serovar Typhimurium infection in porcine jejunal gut loops. Vet Res. (2009) 40:5. doi: 10.1051/vetres:2008043
37. Nygard A, Jørgensen CB, Cirera S, Fredholm M. Selection of reference genes for gene expression studies in pig tissues using SYBR green qPCR. BMC Mol Biol. (2007) 8:67. doi: 10.1186/1471-2199-8-67
38. Livak KJ, Schmittgen TD. Analysis of relative gene expression data using real-time quantitative, PCR, and the 2–ΔΔCt method. Methods. (2001) 25:402–8. doi: 10.1006/meth.2001.1262
39. Biswas S, Brunel JM, Dubus JC, Reynaud-Gaubert M, Rolain JM. Colistin: an update on the antibiotic of the 21st century. Exp Rev Anti Infect Ther. (2012) 10:917–34. doi: 10.1586/eri.12.78
40. Verstegen MW, Williams BA. Alternatives to the use of antibiotics as growth promoters for monogastric animals. Animal Biotechnol. (2002) 13:113–27. doi: 10.1081/ABIO-120005774
41. Wang C, Zhang L, Su W, Ying Z, He J, Zhang L, et al. Zinc oxide nanoparticles as a substitute for zinc oxide or colistin sulfate: effects on growth, serum enzymes, zinc deposition, intestinal morphology and epithelial barrier in weaned piglets. PLoS ONE. (2017) 12:e0181136. doi: 10.1371/journal.pone.0181136
42. He P, Noda Y, Sugiyama K. Green tea suppresses lipopolysaccharide-induced liver injury in D-galactosamine sensitized rats. J Nutr. (2001) 131:1560–7. doi: 10.1093/jn/131.5.1560
43. Samuvel DJ, Shunmugavel A, Singh AK, Singh I, Khan M. S-,nitrosoglutathione ameliorates acute renal dysfunction in a rat model of lipopolysaccharide-induced sepsis. J Pharm, Pharmacol. (2016) 68:1310–9. doi: 10.1111/jphp.12608
44. Li P, Kim SW, Li XL, Datta S, Pond WG, Wu G. Dietary supplementation with cholesterol and docosahexaenoic acid affects concentrations of amino acids in tissues of young pigs. Amino Acids. (2009) 37:709–16. doi: 10.1007/s00726-008-0196-5
45. Liu Y, Chen F, Odle J, Lin X, Zhu H, Shi H, et al. Fish oil increases muscle protein mass and modulates Akt/FOXO, TLR4, and NOD signaling in weanling piglets after lipopolysaccharide challenge. J Nutr. (2013) 143:1331–9. doi: 10.3945/jn.113.176255
46. Zhang L, Wang X, Chen S, Wang S, Tu Z, Zhang G, et al. Medium-chain triglycerides attenuate liver injury in lipopolysaccharide-challenged pigs by inhibiting necroptotic and inflammatory signaling pathways. Int J Mol Sci. (2018) 19:3697. doi: 10.3390/ijms19113697
47. Gross JJ, van Dorland HA, Wellnitz O, Bruckmaier RM. Glucose transport and milk secretion during manipulated plasma insulin and glucose concentrations and during LPS-induced mastitis in dairy cows. J Anim Physiol Anim Nutr. (2015) 99:747–56. doi: 10.1111/jpn.12259
48. Olas B, Wachowicz B, Saluk-Juszczak J, Zieliński T. Effect of resveratrol, a natural polyphenolic compound, on platelet activation induced by endotoxin or thrombin. Thromb Res. (2002) 107:141–5. doi: 10.1016/s0049-3848(02)00273-6
49. Jones KL, Bryan TW, Jinkins PA, Simpson KL, Grisham MB, Owens MW, et al. Superoxide released from neutrophils causes a reductionin nitric oxide gas. Am J Physiol. (1998) 275:1120–26. doi: 10.1152/ajplung
50. Wu G, Bazer FW, Davis TA, Kim SW, Li P, Marc Rhoads J, et al. Arginine metabolism and nutrition in growth, health and disease. Amino Acids. (2009) 37:153–68. doi: 10.1007/s00726-008-0210-y
51. Flynn NE, Bird, JG, Guthrie, AS. Glucocorticoid regulation of amino acid and polyamine metabolism in the small intestine. Amino Acids. (2009) 37:123–9. doi: 10.1007/s00726-008-0206-7
52. Navarro DMDL, Abelilla JJ, Stein HH. Structures and characteristics of carbohydrates in diets fed to pigs: a review. J Anim Sci Biotechnol. (2019) 10:39. doi: 10.1186/s40104-019-0345-6
53. Jang KB, Kim SW. Role of milk carbohydrates in intestinal health of nursery pigs: a review. J Anim Sci Biotechnol. (2022) 13:6. doi: 10.1186/s40104-021-00650-7
54. Zhang YY, Wu T, Chen ZQ, Meng YX, Zhu ZK, Wang Q, et al. Dietary supplementation with Enterococcus faecium R1 attenuates intestinal and liver injury in piglets challenged by lipopolysaccharide. Animals. (2021) 11:1424. doi: 10.3390/ani11051424
55. Drozdowski LA, Thomson AB. Intestinal sugar transport. World J Gastroenterol. (2006) 12:1657–70. doi: 10.3748/wjg.v12.i11.1657
56. Wu T, Zhang Y, Lv Y, Li P, Yi D, Wang L, et al. Beneficial impact and molecular mechanism of Bacillus coagulans on piglets' intestine. Int J Mol Sci. (2018) 19:2084. doi: 10.3390/ijms19072084
57. Wu GD, Chen J, Hoffmann C, Bittinger K, Chen YY, Keilbaugh SA, et al. Linking long-term dietary patterns with gut microbial enterotypes. Science. (2011) 334:105–8. doi: 10.1126/science.1208344
58. Montagne L, Pluske JR, Hampson DJA. review of interactions between dietary fibre and the intestinal mucosa, and their consequences on digestive health in young non-ruminant animals. Anim Feed Sci Tech. (2003) 108:95–117.
59. Hou Y, Wang L, Yi D, Ding B, Yang Z, Li J, et al. N-acetylcysteine reduces inflammation in the small intestine by regulating redox EGF and TLR4 signaling. Amino Acids. (2013) 45:513–22. doi: 10.1007/s00726-012-1295-x
60. Zhou Y, Jiang WD, Zhang JX, Feng L, Wu P, Liu Y, et al. Cinnamaldehyde improves the growth performance and digestion and absorption capacity in grass carp (Ctenopharyngodon idella). Fish Physiol Biochem. (2020) 46:1589–601. doi: 10.1007/s10695-020-00813-9
61. Furuyashiki T, Narumiya S. Stress responses: the contribution of prostaglandin E(2) and its receptors. Nat Rev Endocrinol. (2011) 7:163–75. doi: 10.1038/nrendo.2010.194
62. Bottoms GD, Roesel OF, Rausch FD, Akins EL. Circadian variation in plasma cortisol and corticosterone in pigs and mares. Am J Vet Res. (1972) 33: 785–90.
63. Yao M, Gao W, Tao H, Yang J, Huang T. The regulation effects of danofloxacin on pig immune stress induced by LPS. Res Vet Sci. (2017) 110:65–71. doi: 10.1016/j.rvsc.2016.11.005
64. Sakata D, Yao C, Narumiya S. Prostaglandin E2, an immunoactivator. J Pharmacol Sci. (2010) 112:1–5. doi: 10.1254/jphs.09r03cp
65. Agard M, Asakrah S, Morici LA. PGE(2) suppression of innate immunity during mucosal bacterial infection. Front Cell Infect Microbiol. (2013) 3:45. doi: 10.3389/fcimb.2013.00045
66. Lackeyram D, Yang C, Archbold T, Swanson KC, Fan MZ. Early weaning reduces small intestinal alkaline phosphatase expression in pigs. J Nutr. (2010) 140:461–8. doi: 10.3945/jn.109.117267
67. Thomson AB, Keelan M, Thiesen A, Clandinin MT, Ropeleski M, Wild GE, et al. Small bowel review: diseases of the small intestine. Dig Dis Sci. (2003) 48:1582–99. doi: 10.1023/a:1024776125966
68. Sarbassov DD, Ali SM, Sabatini DM. Growing roles for the mTOR pathway. Curr Opin Cell Bio. (2005) 17:596–603. doi: 10.101016/jceb09
69. Nair RR, Warner BB, Warner BW. Role of epidermal growth factor and other growth factors in the prevention of necrotizing enterocolitis. Semin Perinatol. (2008) 32:107–13. doi: 10.101053/jsemperi01
70. Boudreau F, Lussier CR, Mongrain S, Darsigny M, Drouin JL, Doyon G, et al. Loss of cathepsin L activity promotes claudin-1 overexpression and intestinal neoplasia. FASEB J. (2007) 21:3853–65. doi: 10.1096/fj.07-8113com
71. Florini JR, Ewton DZ, Magri KA. Hormones, growth factors, and myogenic differentiation. Annu Rev Physiol. (1991) 53:201–16. doi: 10.1146/annurev.ph.53.030191.001221
Keywords: piglet, a complex of cinnamaldehyde, carvacrol and thymol, lipopolysaccharide, intestinal function, immune stress response
Citation: Zhang Y, Li Q, Wang Z, Dong Y, Yi D, Wu T, Wang L, Zhao D and Hou Y (2023) Dietary supplementation with a complex of cinnamaldehyde, carvacrol, and thymol negatively affects the intestinal function in LPS-challenged piglets. Front. Vet. Sci. 10:1098579. doi: 10.3389/fvets.2023.1098579
Received: 15 November 2022; Accepted: 13 March 2023;
Published: 30 March 2023.
Edited by:
Takayuki Tohge, Nara Institute of Science and Technology (NAIST), JapanReviewed by:
Qingbiao Xu, Huazhong Agricultural University, ChinaCopyright © 2023 Zhang, Li, Wang, Dong, Yi, Wu, Wang, Zhao and Hou. This is an open-access article distributed under the terms of the Creative Commons Attribution License (CC BY). The use, distribution or reproduction in other forums is permitted, provided the original author(s) and the copyright owner(s) are credited and that the original publication in this journal is cited, in accordance with accepted academic practice. No use, distribution or reproduction is permitted which does not comply with these terms.
*Correspondence: Yongqing Hou, aG91eXFAYWxpeXVuLmNvbQ==
†These authors have contributed equally to this work and share first authorship
Disclaimer: All claims expressed in this article are solely those of the authors and do not necessarily represent those of their affiliated organizations, or those of the publisher, the editors and the reviewers. Any product that may be evaluated in this article or claim that may be made by its manufacturer is not guaranteed or endorsed by the publisher.
Research integrity at Frontiers
Learn more about the work of our research integrity team to safeguard the quality of each article we publish.