- 1EquiCord S. L., Alcorcón, Madrid, Spain
- 2LEITAT Technological Center, Barcelona, Spain
Mesenchymal stem cells are multipotent cells with a wide range of therapeutic applications, including, among others, tissue regeneration. This work aims to test the safety (EUC-MSC) of intra-articular administration of equine umbilical cord mesenchymal stem cells in young healthy dogs under field conditions following single and repeated administration. This was compared with the safety profile of allogenic canine adipose derived mesenchymal stem cells (CAD-MSC) and placebo in order to define the safety of xenogeneic use of mesenchymal stem cells when administered intra-articular. Twenty-four police working dogs were randomized in three groups in a proportion 1:1:1. EUC-MSCs and CAD-MSCs were obtained from healthy donors and were manufactured following company SOPs and under GMP and GMP-like conditions, respectively, and compliant all necessary controls to ensure the quality of the treatment. The safety of the treatment was evaluated locally, systemically and immunologically. For this purpose, an orthopedic examination and Glasgow test for the assessment of pain in the infiltrated joint, blood tests, clinical examination and analysis of the humoral and cellular response to treatment were performed. No adverse events were detected following single and repeated MSC administration despite both equine and canine MSC generate antibody titres in the dogs. The intra-articular administration of equine umbilical cord mesenchymal stem cells in dogs has demonstrated to be safe.
1. Introduction
Mesenchymal stem cells (MSCs) are multipotent cells that can be isolated from many different adult and neonatal tissues. They are defined by their phenotype and functional properties, such as: spindle-shaped morphology, adherence to plastic, immune response modulation capacity, and multilineage differentiation potential (1). Accordingly, MSCs have a wide range of promising applications in the treatment of inflammatory diseases, autoimmune disorders, tissue repair and regeneration (2).
Due to their immunomodulatory properties (3) MSCs are being widely investigated in veterinary medicine for the treatment of inflammatory and autoimmune diseases (4). Also, it is known that through their immunomodulatory capacity they possess tissue regenerative properties (5–7), which is why they are also being investigated for their use in wound healing, tendinopathies, nerve injuries, etc. (4).
Among the pathologies for which MSCs are attracting interest is osteoarthritis (OA). Since current OA treatments treat the symptoms but not the underlying pathology (therefore cartilage deterioration continues) biological therapies based on MSCs have become of great interest in both human and veterinary osteoarthritis research (8). MSCs have demonstrated to be able to reduce local and systemic inflammation due to their immunomodulatory capabilities. In addition, MSCs seem to contribute to cartilage healing through their paracrine signalling which stimulate local repair (9–11).
To date, it has been widely considered that MSCs were immune-privileged, even between different species (12–14). However, recently it has been shown that after single and repeated allogeneic or xenogeneic MSC application the recipient may develop mild antibody titters to donor cells (15). This finding would suggest MSCs are immune-tolerant rather than immune-privileged (16, 17). Despite the induction of serologic response, it has not been involved in any case with systemic immunologic reactions following the application of allogeneic or xenogeneic cells. The lack of association between the presence of antibodies and the onset of clinical symptoms may be due to antibodies generated in the recipient were at very low titters, thus insufficient to provoke a clinically relevant immune response (15, 16). This may allow a patient to receive MSCs from different sources: the patient can receive his own MSCs (autologous MSCs), MSCs from a donor (allogeneic MSCs) or MSCs from a donor of a different species (xenogeneic MSCs) (8).
The intra-articular safety of autologous (18, 19) and allogeneic (20) canine MSCs is well reported in the literature.
The treatment with autologous MSCs has the advantage of not involving donor animal harvesting, so it does not imply ethical issues (21). However, it is a time-consuming task that requires a surgical procedure to harvest tissue containing MSCs (e.g., adipose, bone marrow) from the animal, which is used to grow MSCs. Autologous treatments have a big variability in terms of viability, population doublings and other manufacturing characteristics that have impact in the efficacy of the product and this can be influenced by the manufacturing process and the donor themselves, as the quality of the MSCs declines with increasing donor age (22). Allogeneic and xenogeneic MSC offers the possibility of producing homogenous off-the-shelf treatments so there is not waiting time for growing the MSCs and the animal do not need to have surgery in order to remove its own tissue (23).
Allogeneic cells might be thought to have advantages over xenogeneic cells as they are expected to have higher donor-host compatibility (23). However, there is evidence pointing at xenogeneic MSCs having a comparable effectiveness and safety profile to allogeneic MSCs (24–26), with the additional advantage, among others, of being absent of species-specific transferable pathogens (8). Another advantage of xenogeneic stem cells is the utilisation of a donor species with a higher culture capacity than the recipient (10).
For these reasons, the use of xenogeneic MSCs has been explored by some authors, demonstrating their safety and efficacy after single and repeated administrations (15, 27). Moreover, our group has demonstrated the safety and efficacy of EUC-MSC in natural occurring canine OA (28).
In this work the treated species is the dog and the tissue of choice for the extraction and culture of MSCs is the equine umbilical cord (EUC).
Umbilical cord (UC) presents important advantages as source of MSCs: non-invasive sourcing, higher proliferation capacity (29), greater immune-modulatory capacity (30), less immunogenic (31) and a more secure profile with less risks derived from possible cell mutations, viral agents, parasites agents or other contaminants (32).
Allogeneic MSCs from canine UC are not easy to obtain. It is almost impossible to obtain the canine UC as the dam’s ingests the placenta and UC after birth, therefore the only way to obtain the tissue would be after C-section surgery. Conducting a surgical procedure or C-section in order to obtain UC for pharmaceutical development purposes gives rise to the question of legitimacy and is definitely not aligned with animal welfare. For these reasons, the allogeneic use of canine MSCs from UC is not a good or viable option (33). In addition to being difficult and expensive to obtain (surgery or any other complex process), due to the small size of the tissue, cells require more expansion, passes and population doublings, which are known to have negative effects in terms of efficacy and safety of the product (34).
EUC provides a good alternative, because it is an easy tissue to obtain, virtually limitless that is discarded after birth and the mare does not instinctively ingest it. This makes it perfect from an ethical and animal welfare point of view. In addition, it is a large tissue (~1 kg), rich in MSCs (172,000 cell/g), therefore a large number of cells, requiring minor cell expansion, can be obtained (35).
The aim of this study is to demonstrate that the xenogeneic use of MSC is as safe as the allogeneic use. To this end the safety of intra-articular administration of EUC-MSC in young healthy dogs under field conditions in single and repeated administration was compared with the allogeneic use of canine adipose mesenchymal stem cells (CAD-MSCs) and placebo in order to define their safety profile in the treatment of osteoarthritis.
The study had a total duration of 9 weeks where patients were monitored for clinical signs on a regular basis, as well as orthopaedic signs of the infiltrated joint. Antibodies generated by the dogs against EUC-MSCs, CAD-MSCs and Placebo have also been monitored regularly. Finally, a possible cellular memory response (mediated by CD8 lymphocytes) has been studied, which, if present, could generate an exacerbated immune response after re-infiltration.
2. Materials and methods
2.1. Design
The present study is a double-blinded (owner and researcher), parallel group, randomized and placebo- controlled trial. It was carried out following the International Cooperation on Harmonisation of Technical Requirements for Registration of Veterinary Medicinal Products for Good Clinical Practice (VICH guidelines) and satisfied national regulatory and animal welfare standards and requirements. Informed consent was obtained from dog owner prior to inclusion.
The study was conducted according to the schedule on Table 1.
On day 0 and day 28 (administration days), before product administration, a blood sample was extracted for haematology and biochemistry. On these visits it was also performed a clinical exploration and an orthopaedic evaluation of the joint to ensure that it was not affected prior to infiltration.
After this, all the animals received one intra-articular dose of EUC-MSCs, CAD-MSC or placebo in the right knee.
As disclosed in Table 1, for the first 6 days after the two administrations, the dogs received daily safety visits and weekly visits on days 7, 14, 21, 28, 35, 42, 49, 56 and 63 with a margin of ±2 days for the weekly review. Caregivers were trained to detect any potential adverse effect (AE) and dogs were monitored daily by them. The expected AE were local heat, inflammation and lameness after administration.
Each visit was conducted according to the schedule on Table 1. Any abnormal health observation irrespective of their nature and severity made by either owner or veterinarian was recorded according to VICH guidelines.
2.2. Animal selection
The animals were active police dogs belonging to the Centro Cinológico de la Guardia Civil. It was a uniform population in terms of size, feeding and lifestyle. They were German Shepherds, Malinois and Labrador dogs.
The inclusion criteria were: healthy young animals (older than 1 year), between 20 and 40 kg body weight, with normal haematology and biochemistry. Dogs showed no orthopaedic discomfort at exploration.
Table 2 describes the main characteristics of the population.
2.3. Treatments
Twenty-four dogs that met the inclusion criteria were randomized in three groups of treatment (1:1:1) with: equine cells EUC-MSCs (8), canine cells CAD-MSCs (8) or placebo (8).
Dogs in the EUC-MSC group received 7.5 × 106 EUC-MSCs (DogStem®) intra-articular. Dogs in the CAD-MSC group received 7.5 × 106 CAD-MSCs, at day 0 and 28 of the study.
Both MSCs types were thawed and placed in culture for recovery. They were then harvested and packed in 1 ml of vehicle consisting on a DMSO-free and protein-free solution of salts, sugars and antioxidants. Dogs in the placebo group received 1 ml of saline.
2.4. MSCs manufacturing
For this work, EUC from one donor was collected from a concerted stud after the natural birth of a male foal and it was processed. EUC-MSCs were isolated from Wharton’s jelly, expanded in primary culture until passage 4 following company SOPs, European regulations and under Good Manufacturing Practices (GMP) conditions. Cells were passed when they reached 90% confluence using trypsin 1X (Life Thecnologies).
For the obtaining of CAD-MSCs, the adipose tissue sample was obtained during a surgical intervention for castration from a healthy young female donor. Cells were expanded in primary culture under GMP-like conditions up to passage 4.
Both tissues underwent enzymatic digestion with collagenase type I (Gibco) 1 mg/ml for 3 h for adipose tissue and 4 for UC MSCs were cultured in Dulbecco’s modified Eagle’s medium (DMEM; Gibco) with 10% foetal bovine serum (FBS; Gibco) and 1% Penicillin/streptomycin (Gibco).
After the expansion of the cells of both final products (EUC-MSCs and CAD-MSCs), consisting of 7.5 × 106 MSCs per vial, were tested for sterility, cell concentration, viability, morphology, accumulative population doublings, mycoplasma contamination and were characterised by flow cytometry following Eu.Ph 2.6.27 for sterility, Eu.Ph 2.7.29 for cell concentration and viability Eu.Ph 2.6.7 for mycoplasma and Eu. Ph 2.7.24 for flow cytometry. For morphology and accumulative population doublings a justification and validation of the method was provided to the EMA for approval. For characterisation MSCs must be positive for CD90 (BD Biosciences; 5E10) and CD44 (Bio-Rad; CVS18 for equine; R&D Systems; 69-S5 for canine) and negative for MHC-II (Bio-Rad; CVS20) and CD45 (Bio-Rad; F10-89-4 for equine and Bio-Rad; YKIX716.13 for canine) by using the CytoFLEX flow cytometer and CytExpert 2.0 Software for data analysis.
In addition, a potency test was performed consisting on the ability of MSCs to inherently secrete prostaglandin E-2 (R&D Systems Parameter Prostaglandin E2), as it has been shown that the immunomodulatory capacity of the cells depends on their ability to secrete this molecule (36). The specification for a batch of MSCs to be compliant is [PGE2] ≥2,366 pg./ml.
2.5. Orthopaedic exploration
Orthopaedic exploration was performed by the assessment of joint effusion, detection by palpation of patellar ligament through medial approach, lameness at walk, joint palpation and inspection of injection point. The same veterinary surgeon made the orthopaedic exploration for every dog and at all the time points. For the evaluation of joint effusion, lameness at walk, joint palpation and inspection of injection point the criteria was “yes/no.” A minimum of lameness would be considered lameness and the same for any sign of discomfort of the animal to manipulation of the joint: it would be considered pain.
On day 0 and 28 (before product administration) a drawer test was performed in order to discard rupture of cruciate ligament.
The orthopaedic exploration was assessed on every visit performed.
2.6. Clinical examination
The parameters assessed included: overall status (including hydration, lymph nodes and abdominal palpation) mucous membranes colour, pulmonary auscultation and breathing frequency, cardiac auscultation, heart rate, and rectal temperature. The same veterinary surgeon made the clinical examination for every dog and at all the time points.
The clinical examination was performed on every scheduled visit.
2.7. Glasgow scale determination
Glasgow Composite Measure Pain Scale short form (CMPS-SF) was developed by Glasgow University to measure acute pain in dogs (surgical, medical, inflammatory, or traumatic) and was designed as a clinical decision-making tool. It was constructed using psychometric methods and has been shown to be valid, reliable, and responsive to clinical change in a range of clinical settings (37). The CMPS-SF is used to establish when a patient needs analgesic treatment. The recommended analgesic intervention level was 6/24. Therefore, if an animal score was 6 or above out of 24, analgesic treatment (0.2 mg/kg body weight of meloxicam) was administered.
CMPS-SF comprises six behavioural categories with associated descriptive expressions (38): vocalisation, attention to wound, mobility, response to touch, demeanour, and posture/activity. Items are placed in increasing order of pain intensity and numbered accordingly.
The Glasgow scale was assessed on every visit and by the same veterinary surgeon.
2.8. Laboratory examinations
Blood samples were collected when indicated in Table 1 for haematology (red blood cells, haemoglobin, haematocrit, medium corpuscular volume, whole blood cells and blood smear formula of Eosinophils, Basophils, Lymphocytes, Monocytes, and platelet count) and serum biochemistry (Blood Urea Nitrogen (BUN), Creatinine, Aspartate Aminotransferase (AST), Alanine Aminotransferase (ALT), Total Proteins, Alkaline phosphatase, Glucose, Amylase, Total bilirubin, Cholesterol, Albumin and Globulins).
Blood samples were analysed by the same external laboratory.
2.9. Humoral response
For the analysis of the humoral response against the MSCs, a CELISA (Cellular enzyme-linked immunospecific assay) was performed (Figure 1). Sera samples of all dogs were taken at day 0 and day 42. For the CELISA, EUC-MSCs or CAD-MSCs were seeded onto cell culture treated flat bottom 96-well microplates (Nunc) at a density of 20,000 cells per well and 200 μl/well of Dulbecco’s Modified Eagle Medium (DMEM)-10% foetal bovine serum (FBS) and let to adhere at 37°C and 5% CO2 for 24 h. The day after, supernatant was removed, and primary antibodies (positive control) or sera from placebo, CAD-MSC and EUC-MSC treated dogs (test sample) were added diluted in 100 μl/well of DMEM-10% FBS and incubated for 90 min at 37°C and 5% CO2. Sera from placebo dogs were exposed to both CAD-MSCs and EUC-MSCs. As positive control, mouse monoclonal anti-equine CD44 (Clone 69-S5, R&D Systems) and mouse monoclonal anti-equine CD90 (Clone MRC OX-7, Abcam) were used at 2 μg/ml. Reactivity of control antibodies against equine antigens was predicted bibliographically (39, 40) and confirmed experimentally using several concentrations of antibody on equine MSCs and control cells (negative for CD44 and CD90) (data not shown). After the incubation, wells were washed with 300 μl/well of PBS. Then, the horseradish peroxidase (HRP) conjugated secondary antibody goat anti-mouse (Jackson Immunoresearch) at a dilution of 0.04 μg/ml or rabbit anti-dog (Jackson Immunoresearch) at a dilution of 0.8 μg/ml were incubated in 100 μl/well for 30 min at 37°C and 5% CO2. After the incubation, wells were washed 5 times with 300 μl/well of PBS. Finally, 100 μl/well of 3,3′,5,5’-Tetramethylbenzidine (TMB) substrate was added. After 15 min at room temperature (RT), reaction was stopped by adding 100 μl/well of 1 M HCl, and signal was read at 450 nm using a Thermo Labsystems Multiskan Ascent plate reader. Background correction (OD450nm 0.3UA) was not applied. All conditions were represented in duplicates and the dilution used was 1/1000.
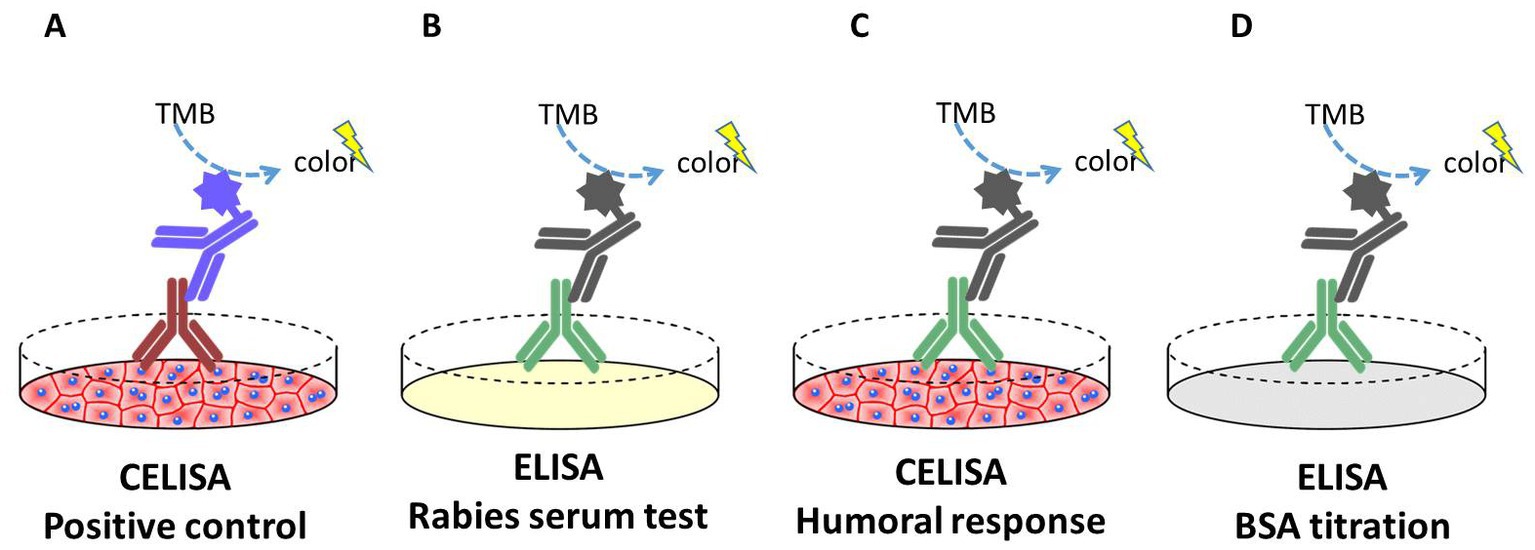
Figure 1. Schematic representation of the CELISA and ELISA assays performed in this study. (A) Positive control: CELISA where plates were coated with live EUC-MSCs or CAD-MSCs. After the incubation of mouse anti-CD44 or mouse anti-CD90 primary antibody (brown), signal was quantified. (B) Rabies serum test: ELISA where plates were coated with rabies vaccine antigen. After the incubation of the dog sera (green), signal was quantified. (C) Humoral response test: CELISA where plates were coated with live EUC-MSCs or CAD-MSCs. After the incubation of the dog sera (green), signal was quantified. (D) BSA titration: ELISA where plates were coated with bovine serum albumin. After the incubation of the dog sera (green), signal was quantified.
Rabies vaccination was used as positive control. In this assay, the antigen of the rabies vaccine vial (Etadex®, Ecuphar) was diluted 1/1,000 in PBS and coated onto the ELISA plates (MaxiSorp™, Nunc). Additionally, dogs’ sera were tested in ELISA plates coated with 5 mg/ml of bovine serum albumin (BSA fraction V, from Calbiochem) at Day 0. This control is included in order to test the presence of xenogeneic antibodies in dogs’ blood. In both assays, dog sera were analysed as previously explained in the CELISA assay.
The readout of the CELISA was qualitative (presence/absence). Quantification was not performed.
2.10. Cellular response
The secondary cellular response is measured in terms of an increase in the percentage of CD8+ lymphocytes within the PBMC population. For this purpose, Peripheral Blood Mononuclear Cells (PBMCs) obtained from blood samples of day 42 per protocol (after the second administration) of all the EUC-MSCs treated dogs and 3 placebo dogs were co-cultured with EUC-MSCs for 4 days. CAD-MSCs group was not included since sample was not available.
Fresh blood samples from recipient dogs were used for PBMCs isolation by Ficoll® and cryopreserved in liquid nitrogen. Viability and cell number were checked by dye-exclusion method before the assay was performed.
10Gy irradiated EUC-MSCs were seeded in 96-well plates at 20,000 cells/well in 200 μl DMEM and culture at 37°C 5% CO2. After 48 h, EUC-MSCs monolayer was washed once with PBS and 100,000 thawed PBMCs from dogs were seeded on top in 200 μl of Roswell Park Memorial Institute (RPMI) medium. Each condition was seeded in triplicate. The co-culture was continued for 4 days at 37°C and 5% CO2. As positive control PBMCs were activated with 10 μg/ml phytohemagglutinin (PHA; Sigma Aldrich). As negative control PBMCs were cultivated alone (basal condition).
On the fourth day of co-culture, PBMCs of all conditions were collected in order to determine, by flow cytometry, whether the percentage of memory lymphocyte CD8+ had increased due to the exposure with MSCs. In addition, at the end of the co-culture period, MSCs were assessed for cell number and viability to rule out the possibility that the PBMCs had reacted against them.
In order to deplete the immunomodulatory capacity of MSCs so they do not interfere with the potential memory response of the PBMCs against the MSCs a control is added. Indomethacin is used as a blocker of the production of PGE2 by the MSCs (41). It is known that the mechanism of action of MSCs is through PGE2 (36, 42). In this condition, EUC-MSCs were co-cultured with pre-treated dogs’ PBMCs in presence of indomethacin 10 μM as previously described by Carrade Holt et al. in MSCs from different sources (41). According to bibliography this concentration is enough to block completely the secretion of PGE2.
Approximately 300,000 PBMCs per condition (Figure 2) were distributed into eppendorf tubes and washed by centrifugation at 1,800 rpm for 10 min at RT. Subsequently, the supernatant was discarded by decantation and the resulting pellet was labelled with CD8-FITC monoclonal antibody (clone YCATE55.9; BioRad) specific for dog or with an FITC isotypic control Rat IgG2a antibody at a concentration of 10 μl/106 cells in a final volume of 100 μl of PBS.
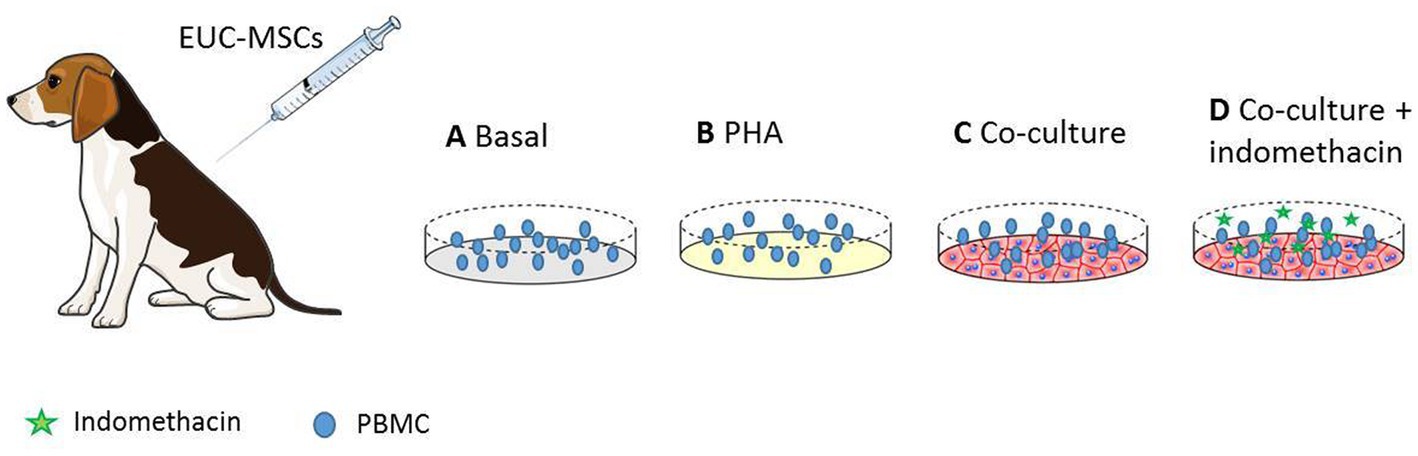
Figure 2. PBMCs from EUC-MSCs treated dogs were extracted and cultured in the four experimental conditions represented above. (A) Basal PBMCs are incubated in culture media. (B) Shows the positive control where PBMCs are stimulated with PHA. (C) The experimental condition, where the PBMCs are co-cultured with EUC-MSCs. (D) The control with indomethacin. In this condition the immunosupresive capacity of EUC-MSCs is blocked.
All cells were incubated for 20 min at 2–8°C. After incubation, about 900 μl of PBS was added to all tubes and they were washed by centrifugation at 1,800 rpm for 10 min at RT to remove excess antibody.
Subsequently, the supernatant was discarded by decantation and the resulting pellet was resuspended in 450 μl of PBS.
To discard dead cells in analysis, 2 μl of Propidium Iodide was added to each tube just prior to cell acquisition. A total of 100,000 viable cells per condition were acquired using the CytoFLEX flow cytometer and were analysed using CytExpert 2.0 Software. For the analysis of the CD8 population, 20.000 events in the lymphocytes region were gated according to their forward (FSC-H) and sideways (SSC-H) scatter. Over this population the CD8 positive cells were analysed (43).
2.11. Statistical methods
Statistical analysis was made using SAS System v9.4. All statistical decisions were performed considering two-sided tests and a significance level set at 0.05.
For quantitative variables, differences between groups were tested by means of t-tests or Mann–Whitney’s test when the normality assumption was not met. Data are expressed as mean ± SEM.
For qualitative variables, the association between variables was tested by means of the appropriate test (Chi-square test or Fisher’s exact when the Cochran’s rule was not satisfied).
3. Results
3.1. MSCs manufacturing
MSCs were compliant for all the quality controls performed: sterility, cell concentration, viability, morphology, potency test accumulative population doublings and mycoplasma contamination. The characterisation by flow cytometry was also compliant with the established specifications: positive markers were above 90% and negative markers below 3%.
3.2. Orthopaedic assessment
The screening visit determined that all the dogs included in the study had a normal orthopaedic evaluation: no joint effusion, no lameness, normal joint palpation, negative Drawer Sign and skin was intact in the injection point.
After the first administration no AE was reported in any group.
Only one AE was detected after the second administration, on day 29 per protocol, in one dog from the placebo group that presented effusion and mild lameness.
3.3. Clinical examination
Three dogs (1 EUC-MSC group and 2 placebos) had mildly increased temperature (~40°C) on days 15, 28 and 42, respectively. In all cases the animals were nervous and not presenting any other symptoms of disease. Therefore, it was considered as a casual finding, without being a sign of illness.
No sings of illness or abnormal findings occurred in any other dog during the trial in the clinical exams performed.
3.4. Laboratory examinations
No pathological alterations in blood test results were present in the laboratory analysis performed along the study.
Some causal findings (mildly increased haematocrit, haemoglobin levels, platelet count, MHC and HMCC values, glucose and AST levels) were observed equally in the three groups but they were not considered pathological given the population of dogs used during the study (high training police dogs), and considering the values found were not alarmingly out of range.
3.5. Glasgow scale evaluation
One of the dogs from the placebo group (same already mentioned in orthopaedic assessment), had a “Mobility” score of 1 on day 29. Also, a dog in the CAD-MSCs group was scored as 1 on day 31 (3 days after second administration).
All the other dogs and time points did not show abnormal scores.
3.6. Humoral response
Before product administration none of the dogs showed antibodies against CAD-MSC nor EUC-MSCs. Fourteen days after second administration (day 42) 6/8 dogs (75%) EUC-MSC treated dogs and 5/8 dogs (63%) CAD-MSC treated dogs generated antibodies against EUC-MSC or CAD-MSC, respectively. One dog (13%) in the placebo group also showed a humoral response against EUC-MSCs but not against CAD-MSC (Figure 3).
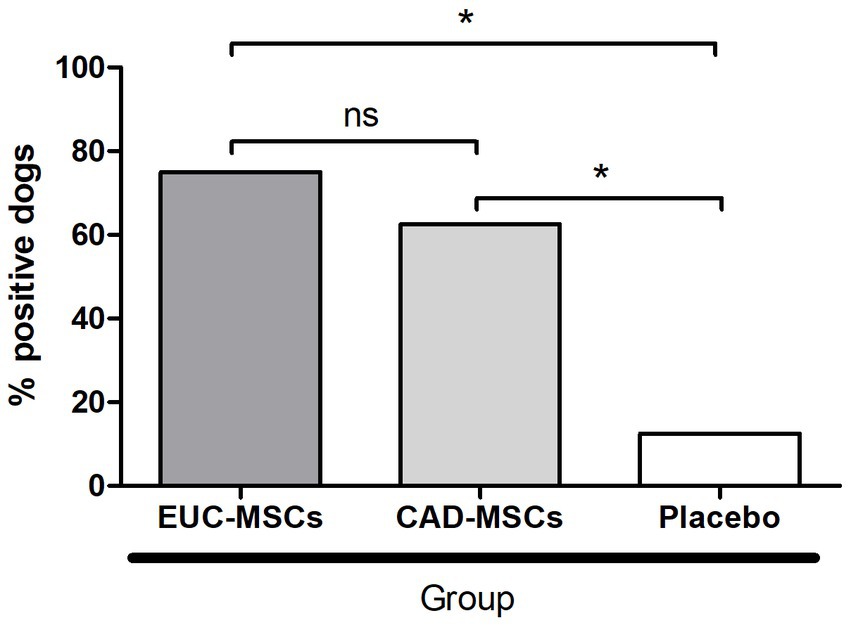
Figure 3. Percentage of dogs generating a humoral response after reinfiltration (day 42 per protocol) against the MSC type they received. Placebo sera were examined against both (EUC-MSCs and CAD-MSCs) and the result was negative for all of them against CAD-MSCs and one dog was positive against EUC-MSCs. *p < 0.05.
All dogs showed antibodies against rabies (positive control) and 88% of them showed antibodies against BSA on day zero, before the treatment injection (Figure 3).
3.7. Cellular response
For EUC-MSC treated animals, basal PBMCs showed a mean percentage of lymphocytes CD8+ of 18.1% of the total lymphocyte population. In co-culture the mean % of CD8+ was 19.6% not being this difference statistically significant (Figure 4).
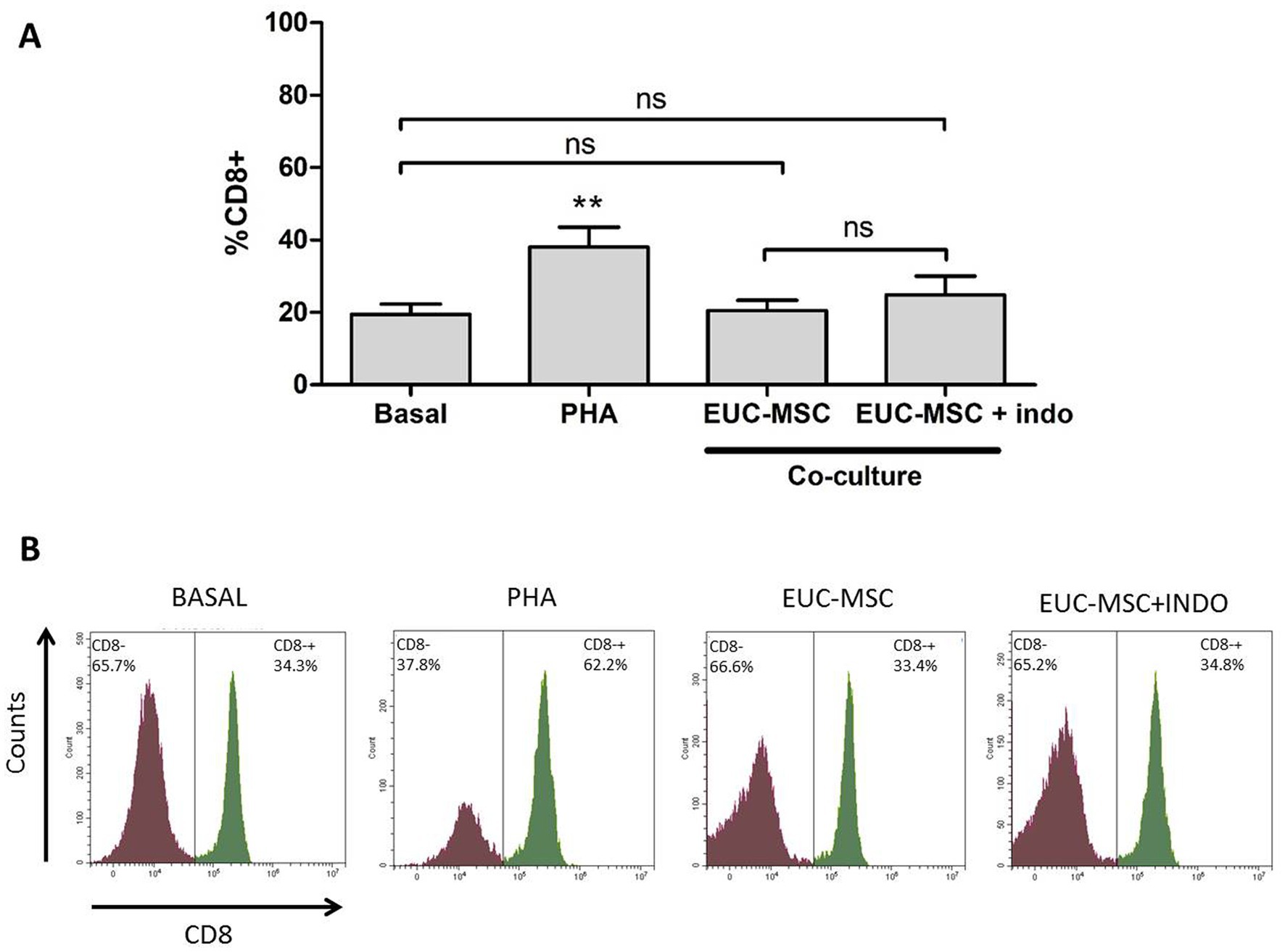
Figure 4. Percentage of memory lymphocytes CD8+ under each condition. (A) Mean percentage of memory lymphocytes CD8+ over the total lymphocyte population for each condition. Basal condition is compared with the rest of conditions. No differences were found in the co-culture compared neither to Basal nor between the two conditions of co-culture. (B) Example of an histogram of a donor under the different conditions. **p < 0.01 compared to Basal.
The co-culture with indomethacin did not show any difference with the one without it, demonstrating that MSCs are not inhibiting the activation of lymphocytes, but on the contrary, there was no activation.
In the lymphocyte population incubated with PHA the % of CD8+ was around 40% of the total lymphocyte population, confirming the ability of lymphocytes to be activated in the presence of a nonspecific stimulus. Activation of lymphocytes population is also observed by the increase in size and complexity which translates into a displacement of the population in the histogram upwards and to the right. This activation was not seen in the co-cultures (Figure 5).
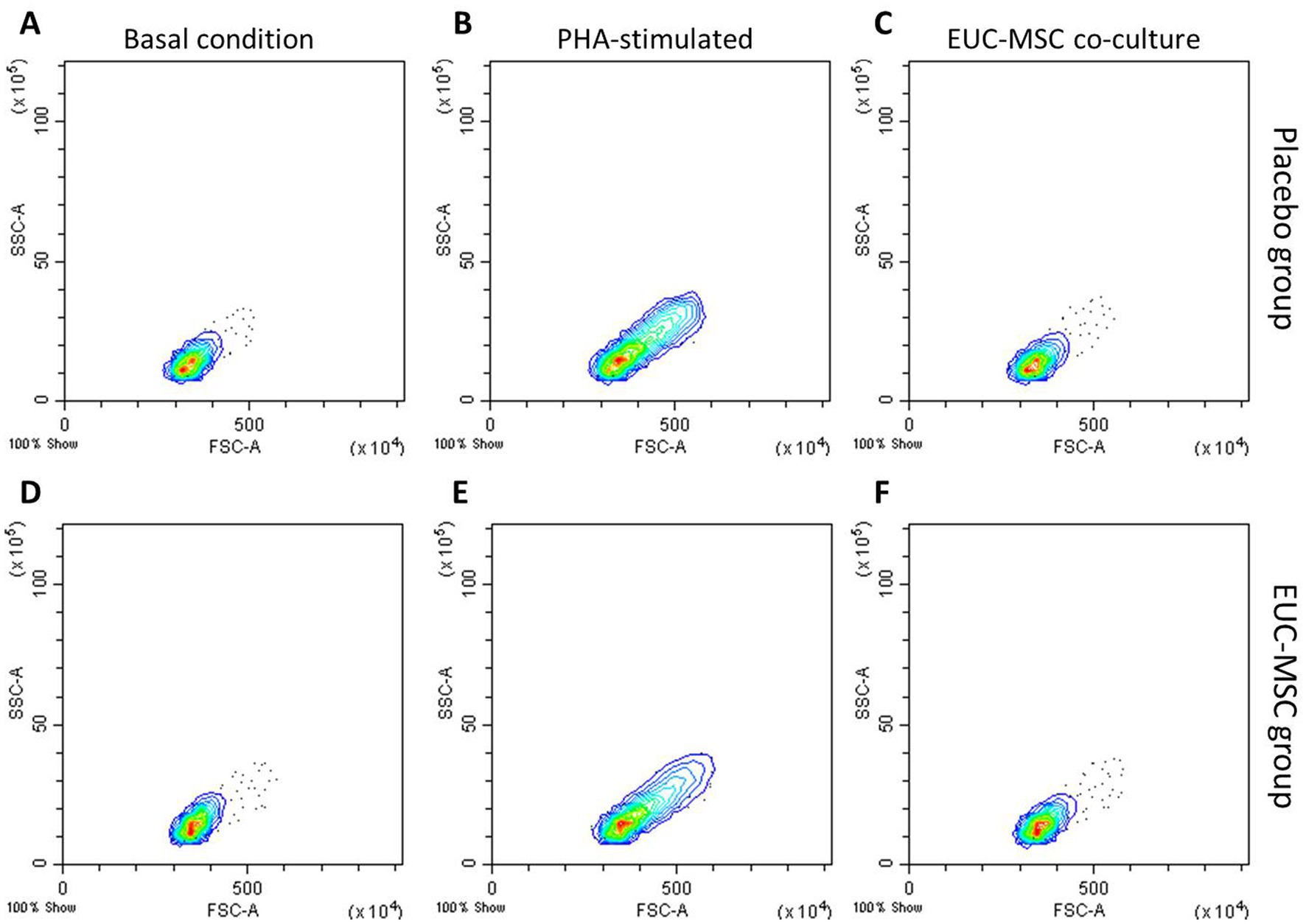
Figure 5. Flow cytometry analysis of lymphocyte populations from whole blood. (A–C) show basal condition, PHA-stimulated and co-cultured lymphocytes, respectively, from a placebo dog. (D–F) show the same from a EUC-MSCs treated dog.
In the placebo-treated dogs no differences were found compared with the EUC-MSCs treated dogs for any condition (data not shown).
MSCs showed a viability of more than 80% in all cases.
3.8. Adverse effects
Eleven non-product-related adverse events (AE) were detected in all groups indistinctly. These adverse events were: gastrointestinal symptoms, Dirofilaria repens, bite wound, epistasis and kennel cough. The AEs are reported individually in Table 3.
In all the cases the adverse effects were considered to not be related to product administration since they were seen in same prevalence in all the groups. The most common AE detected was diarrhoea but clinical exploration was considered normal (temperature, hydration and abdominal palpation) in all the cases. These events were not considered pathologic.
4. Discussion
The aim of this study was to demonstrate the safety of the xenogeneic use of EUC-MSC in dogs. To prove our hypothesis the safety of EUC-MSCs when administered intra-articular in young healthy dogs in single and repeated administration was compared to single and repeated administration of allogeneic CAD-MSCs and placebo.
MSCs do not respond to conventional dose/response studies (44), so selecting the appropriated dose is often challenging in stem cells therapies. The dose 7.5 × 106 cells/ml was calculated based on a multimodal approach. First, a comparison with the dose used in other species was done in order to determine the ideal dose comparing the joint size of the dog. For such comparison human and equine dose/size correlation was used (45). From this study it was determined that an intended dose for dogs should be between 5 and 10 million cell/joint. Then, a bibliographic research on the published intra-articular doses in dogs was done. According to the bibliography the most used dose for intra-articular administration in dogs is between 5 and 10 million (20, 21, 46–48). Last, the 7.5 × 106 cells/ml dose was tested in vivo in natural injured dogs, in a pre-clinical study (data pending publication).
When making a safety study for a conventional treatment the procedure is to administer 2, 3 and 5-fold the effective dose of choice (49, 50). However, according to EMA guidelines overdose does not provide significant added value, when MSC-based product is administered locally (44, 48, 51).
The selected administration route was intra-articular. Intra-articular administration in dogs practice is not as common as in equine practice, so an intravenous approach would be an advantage in small animal practice (8). However, intravenous application of MSC is not without its drawbacks: (1) On the one hand, the dose required in intravenous treatments depends on the weight of the animal, so in large dogs the number of cells to inject will be much higher in IV administration than in intra-articular administration, which would presumably increase the cost of treatment. (2) In osteoarthritis, where there is a local inflammatory environment mediated by cytokines and inflammatory cells, applying MSCs locally is much more direct than IV, generating a more powerful anti-inflammatory/immunomodulatory effect. (3) Efficacy is greater in intra-articular applications. Reliably derived from the two previous points, less need for cells and direct local effect, in canine OA, the efficacy of IA MSCs is much higher than the efficacy of IV MSCs. Shah reported that the efficacy in the treatment of canine OA after IA application of MSC was 62% while after IV application the efficacy was <40%. Results from Shah and her group (52) after IA application of MSC in canine OA are in line with the ones reported by our group (28) where the efficacy of EUC-MSC in naturally occurring OA is greater than 65%.
In this study, the analysis of the treatment safety was thoroughly investigated at both local and systemic levels.
The dogs chosen for this study were a uniform population of police working dogs. They were young, healthy and very active dogs. Owners were asked to keep the dogs at rest on the day of infiltration, but the following day they continued with their usual training routine without showing any effect due to the administration. Only one dog in the placebo group showed effusion and mild lameness after the second administration. Since the AE occurred in a placebo-treated dog, it can be attributable to the intra-articular administration process rather than the product itself.
As for the potential immune response generated by the MSCs, it was measured at both humoral and cellular levels.
In the EUC-MSC group, 75% of the dogs developed antibodies against EUC-MSCs after repeated administration compared to 63% in the CAD-MSC group, nevertheless the specific antibody titers were not investigated in the present study, which has prevented a potential comparison between the titration between xenogenic and allogenic. In the present study, there was not harmful in any group, since the dogs did not show any symptom or abnormality in any of the examinations or laboratory tests. Furthermore, this difference is based on only one more dog responding to EUC-MSCs than to CAD-MSCs. The fact that the dogs treated with CAD-MSCs showed almost the same percentage of animals developing a serological response (only one dog less) as the dogs treated with EUC-MSCs, shows that it is not relevant that the cells are from a different species to the development or not of antibodies. The process of injecting these cells itself is prone to generate a minimal serological response, regardless of the origin of the cells. In all cases it is subclinical and does not cause adverse effects on the patient (15). In a recent review (53) the generation of donor specific antibodies was investigated in 555 patients, despite 12% of the patients developed antibodies the safety of MSC was proved in all the studies concluding that the generation of alloantibodies had no clinical relevance. Interestingly, antibodies against EUC-MSCs were also found in one dog (13%) in the placebo group, suggesting that dogs’ population could have exposure to xenogeneic horse-like tissue/cells/antigens in their normal life (maybe through vaccination or other exposures) or a cross-reactive response to the study.
In recent years, some authors claim that MSCs cannot be used for retreatment because they generate a cytotoxic response in the host (54, 55) that results in cell death. This makes the efficacy of the repeated treatments to be questioned. In these studies the cytotoxicity of MSCs on re-exposures is observed after an in vitro model mediated by the exogenous application of rabbit complement.
However, the reality after in vivo applications is that efficacy of MSC in allogeneic or xenogeneic administration is not reduced after repeated application. There is a strong amount of evidence that reported the efficacy of repeated administration of allogeneic MSC (56, 57) but also xenogeneic (58–60).
The reasons for the differences in the results between Berglund (54) and Rosa (55) in vitro model mediated by the addition of exogenous rabbit complement and the results in clinical practice or the present study, are difficult to establish, however, the potent immunomodulatory effect of MSCs and their complex in vivo mechanism of action could explain the different results.
Although MSCs are not completely ignored by the immune system and it is clear that they are capable of triggering a humoral response, as seen in the present study they are not able to transform this humoral response in a CD8+ cytotoxic response.
In this regard, the cytotoxicity of EUC-MSCs elicited by dog PBMCs was investigated by Garcia-Pedraza (61). EUC-MSCs were co-cultured with PBMCs from dogs that had received single or repeated doses of EUC-MSCs or placebo and the cytotoxicity was evaluated by MTT (3-[4,5-dimethylthiazo1-2-y1]-2,5-diphenyltetrazolium bromide) at different times. No cytotoxic effect was seen at any time point after single and repeated treatments. These results are in line with those reported by Van Hecke and Deputhy (62, 63).
As it has been discussed, UC-MSCs are more suitable for allogeneic or xenogeneic use than cells from other sources where the immune response appears to be more enhanced (64). Whilst EUC-MSCs are not associated with cytotoxicity, a humoral response can be seen after allogeneic or xenogeneic treatments. The generation of antibodies against MSC is well described in the literature; however the safety after re-treatments is also well reported by other authors (62, 63) and in the present work.
The presence of xenogeneic antibodies is not something new; many authors have described the presence of antibodies against BSA in humans, horses and dogs (65, 66). The origin of these antibodies seems to be due the traces of BSA and foetal bovine serum (FBS) in vaccines and other medications. Specifically, Mogues (66) investigated the prevalence of antibodies against BSA in a healthy population (55%) and in a population with lung cancer (51%). Mogues also investigated the effect on these antibodies after BSA re-exposure due to cancer surgical resection. After re-exposure, 98% of the patients showed antibodies against BSA with an increase of titter of 200-fold compared to before of re-exposure. Nevertheless, no patient showed any adverse event or pathological effect after the re-exposure to BSA; moreover elevated levels of anti-BSA antibodies were not associated with any detectable clinical events in either the healthy blood donors or the cancer patients.
As in the case of the present study with anti-MSC antibodies, it has been seen that anti-BSA antibodies have not been related to secondary cellular responses in humans (67), which may explain the innocuousness of re-exposure seen by Mogues (66). Therefore, it could be considered that in the absence of a cellular response, the presence of xenogeneic antibodies is not associated with safety or efficacy problems in re-exposures.
In the present study the presence of antibodies against BSA was also investigated at time 0 in all the dogs. As mentioned before, 88% of the dogs showed levels of xenogeneic antibodies against BSA before product administration. This demonstrates that the dogs are able to generate xenogeneic antibodies to manufacturing components of vaccines without relation to a negative immune response or safety problem in future canine vaccinations or re-exposure to BSA through diet or other sources. It would have been interesting to measure the anti-BSA titter after the administration of MSCs that may also contain traces of FBS in their composition, in order to determine if the administration of cell therapies is associated with an increase in anti-BSA antibodies. However, since anti-BSA antibodies were not associated with any adverse effects, it was not considered essential for the development of the study.
As discussed, it is more relevant the ability to generate a cellular response than the presence of antibodies per se, therefore the cellular response of canine PBMCs was investigated in the present work. For this work, CD8+ lymphocyte proliferation was expressed as an increase in the levels of CD8+ cells in peripheral blood of treated animals. A response mediated by cytotoxic CD8+ lymphocytes, would not allow re-infiltration with EUC-MSCs, which would prevent repeating the treatment. However, this study demonstrates the absence of a cytotoxic memory response indicating that re-infiltration with xenogeneic EUC-MSCs is safe and that the effectiveness would not be diminished. This memory response was evaluated by exposing the PBMCs from the dogs of the treatment group to EUC-MSCs in a co-culture, and measuring the differences in percentage of CD8+ memory lymphocytes (68). The generation of a memory response by the dog immune system would have resulted in an augment of CD8+ lymphocytes percentage upon re-contact with EUC-MSCs (69). PBMCs collected on day 42 of the protocol were chosen because it was considered the worst case scenario in which the cellular response, if any, would be the highest (14 days after the second administration). The co-culture conditions were followed as previously described in the literature (36). No significant increase in % CD8+ was seen in EUC-MSCs treated animals due to the re-exposition in the co-culture this means that the total percentage of CD8+ lymphocyte did not change along the study, nevertheless the proliferation was not addressed by other methods therefore could not be completely ruled out that proliferation could occur without changing the percentage of CD8+. On the other hand, no activation of PBMCs was observed since cells do not change in size and complexity, a typical variation when these cells are activated as shown in Figure 5, where PBMCs are stimulated with PHA (70). As observed, this absence of cellular response did not change in presence of indomethacin, showing that in the co-culture MSCs are not immunomodulating the memory response, but that the memory response does not exist.
Regarding the adverse events recorded in this study, the diarrheic episodes detected in both treatments and placebo group was considered to be a consequence of stress, confinement and training. It is well known that diarrheic episodes are common in working dogs and in highly nervous and excitable dogs (71).
Finally, no local or systemic adverse event has been identified after single or repeated administration in any of the animals.
In the present study it has been demonstrated that the single and repeated intra-articular administration of EUC-MSC is as safe as CAD-MSCs in young healthy dogs. As the main limitation of this study, it is worth noting the absence of dogs with natural disease or the evaluation of the efficacy of both single and repeated doses. Nevertheless, the same group recently conducted an efficacy and safety study (28) in dogs with naturally occurring OA treated with 7.5×106 EUC-MSC (DogStem®) demonstrating the efficacy and safety of equine umbilical MSC in dogs with OA.
Altogether, these findings support the safety of the xenogeneic use of EUC-MSCs in dogs.
Data availability statement
The raw data supporting the conclusions of this article will be made available by the authors, without undue reservation excepting that information considered confidential.
Ethics statement
The animal study was reviewed and approved by Spanish Medicines Agency (AEMPS). Written informed consent was obtained from the owners for the participation of their animals in this study.
Author contributions
AP designed the study and performed the data analysis. EP wrote the manuscript and performed the data analysis. MR conducted the cellular response test and the flow cytometry. LP conducted the humoral study. MG-C manufactured according GMP the MSCs injected in the animals. All authors contributed to the article and approved the submitted version.
Funding
This research was funded by EquiCord S.L.
Acknowledgments
We would like to thank to the Centro Cinológico de la Guardia Civil and their veterinary team for the good disposition in the conductance of the study and to the veterinarians that made the intra-articular administration. Also thanks to the care givers of the dogs for their cares and interest in the study.
Conflict of interest
EP, MG-C, MR, and AP are employed by EquiCord S.L. EquiCord owns DogStem’s European Marketing Authorisation.
The remaining authors declare that the research was conducted in the absence of any commercial or financial relationships that could be construed as a potential conflict of interest.
Publisher’s note
All claims expressed in this article are solely those of the authors and do not necessarily represent those of their affiliated organizations, or those of the publisher, the editors and the reviewers. Any product that may be evaluated in this article, or claim that may be made by its manufacturer, is not guaranteed or endorsed by the publisher.
References
1. Dominici, M, le Blanc, K, Mueller, I, Slaper-Cortenbach, I, Marini, FC, Krause, DS, et al. Minimal criteria for defining multipotent mesenchymal stromal cells. The International Society for Cellular Therapy Position Statement. Cytotherapy. (2006) 8:315–7. doi: 10.1080/14653240600855905
2. Huang, S, Leung, V, Peng, S, Li, L, Lu, FJ, Wang, T, et al. Developmental definition of MSCS: new insights into pending questions. Cell Reprogram. (2011) 13:465–72. doi: 10.1089/cell.2011.0045
3. Kim, HS, Kim, K-H, Kim, S-H, Kim, Y-S, Koo, K-T, Kim, T-I, et al. Immunomodulatory effect of canine periodontal ligament stem cells on allogenic and xenogenic peripheral blood mononuclear cells. J Periodontal Implant Sci. (2010) 40:265–70. doi: 10.5051/jpis.2010.40.6.265
4. Gugjoo, MB, Chandra, V, Wani, MY, Dhama, K, and Sharma, GT. Mesenchymal stem cell research in veterinary medicine. Curr Stem Cell Res Ther. (2018) 13:645–57. doi: 10.2174/1574888X13666180517074444
5. Toh, WS, Lai, RC, Hui, JHP, and Lim, SK. MSC exosome as a cell-free MSC therapy for cartilage regeneration: implications for osteoarthritis treatment. Semin Cell Dev Biol. (2017) 67:56–64. doi: 10.1016/j.semcdb.2016.11.008
6. Zhao, X, Zhao, Y, Sun, X, Xing, Y, Wang, X, and Yang, Q. Immunomodulation of MSCs and MSC-derived extracellular vesicles in osteoarthritis. Front Bioeng Biotechnol. (2020) 8:575057. doi: 10.3389/fbioe.2020.575057
7. Kriston-Pál, É, Czibula, Á, Gyuris, Z, Balka, G, Seregi, A, Sükösd, F, et al. Characterization and therapeutic application of canine adipose mesenchymal stem cells to treat elbow osteoarthritis. Can J Vet Res. (2017) 81:73–8.
8. Brondeel, C, Pauwelyn, G, de Bakker, E, Saunders, J, Samoy, Y, and Spaas, JH. Review: mesenchymal stem cell therapy in canine osteoarthritis research: “Experientia Docet” (experience will teach us). Front Vet Sci. (2021) 8:668881. doi: 10.3389/fvets.2021.668881
9. Gupta, PK, das, AK, Chullikana, A, and Majumdar, AS. Mesenchymal stem cells for cartilage repair in osteoarthritis. Stem Cell Res Ther. (2012) 3:116. doi: 10.1186/scrt116
10. Sasaki, A, Mizuno, M, Mochizuki, M, and Sekiya, I. Mesenchymal stem cells for cartilage regeneration in dogs. World J Stem Cells. (2019) 11:254–69. doi: 10.4252/wjsc.v11.i5.254
11. Lo Monaco, M, Merckx, G, Ratajczak, J, Gervois, P, Hilkens, P, Clegg, P, et al. Stem cells for cartilage repair: preclinical studies and insights in translational animal models and outcome measures. Stem Cells Int. (2018) 2018:1–22. doi: 10.1155/2018/9079538
12. Toupet, K, Maumus, M, Peyrafitte, JA, Bourin, P, van Lent, PL, Ferreira, R, et al. Long-term detection of human adipose-derived mesenchymal stem cells after intraarticular injection in SCID mice. Arthritis Rheum. (2013) 65:1786–94. doi: 10.1002/art.37960
13. Ra, JC, Shin, IS, Kim, SH, Kang, SK, Kang, BC, Lee, HY, et al. Safety of intravenous infusion of human adipose tissue-derived mesenchymal stem cells in animals and humans. Stem Cells Dev. (2011) 20:1297–308. doi: 10.1089/scd.2010.0466
14. Plotnikov, AN, Shlapakova, I, Szabolcs, MJ, Danilo, P Jr, Lorell, BH, Potapova, IA, et al. Xenografted adult human mesenchymal stem cells provide a platform for sustained biological pacemaker function in canine heart. Circulation. (2007) 116:706–13. doi: 10.1161/CIRCULATIONAHA.107.703231
15. Wang, Y, Han, ZB, Ma, J, Zuo, C, Geng, J, Gong, W, et al. A toxicity study of multiple-administration human umbilical cord mesenchymal stem cells in cynomolgus monkeys. Stem Cells Dev. (2012) 21:1401–8. doi: 10.1089/scd.2011.0441
16. Beggs, KJ, Lyubimov, A, Borneman, JN, Bartholomew, A, Moseley, A, Dodds, R, et al. Immunologic consequences of multiple, high-dose administration of allogeneic mesenchymal stem cells to baboons. Cell Transplant. (2006) 15:711–21. doi: 10.3727/000000006783981503
17. Kol, A, Wood, JA, Carrade Holt, DD, Gillette, JA, Bohannon-Worsley, LK, Puchalski, SM, et al. Multiple intravenous injections of allogeneic equine mesenchymal stem cells do not induce a systemic inflammatory response but do alter lymphocyte subsets in healthy horses. Stem Cell Res Ther. (2015) 6:73. doi: 10.1186/s13287-015-0050-0
18. Vilar, JM, Morales, M, Santana, A, Spinella, G, Rubio, M, Cuervo, B, et al. Controlled, blinded force platform analysis of the effect of intraarticular injection of autologous adipose-derived mesenchymal stem cells associated to PRGF-Endoret in osteoarthritic dogs. BMC Vet Res. (2013) 9:131. doi: 10.1186/1746-6148-9-131
19. Nicpoń, J, Marycz, K, Grzesiak, J, Śmieszek, A, and Toker, ZY. The advantages of autologus adipose derived mesenchymal stem cells (AdMSCs) over the non-steroidal anti-inflammatory drugs (NSAIDs) application for degenerative elbow joint disease treatment in dogs-twelve cases. Kafkas Univ Vet Fak Derg. (2014) 20:345–50. doi: 10.9775/kvfd.2013.10105
20. Cabon, Q, Febre, M, Gomez, N, Cachon, T, Pillard, P, Carozzo, C, et al. Long-term safety and efficacy of single or repeated intra-articular injection of allogeneic neonatal mesenchymal stromal cells for managing pain and lameness in moderate to severe canine osteoarthritis without anti-inflammatory pharmacological support: pilot clinical study. Front Vet Sci. (2019) 6:10. doi: 10.3389/fvets.2019.00010
21. Black, LL, Gaynor, J, Gahring, D, Adams, C, Aron, D, Harman, S, et al. Effect of adipose-derived mesenchymal stem and regenerative cells on lameness in dogs with chronic osteoarthritis of the coxofemoral joints: a randomized, double-blinded, multicenter controlled trial. Vet Ther. (2007) 8:272–84.
22. Nöth, U, Steinert, AF, and Tuan, RS. Technology insight: adult mesenchymal stem cells for osteoarthritis therapy. Nat Clin Pract Rheumatol. (2008) 4:371–80. doi: 10.1038/ncprheum0816
23. Lin, CS, Lin, G, and Lue, TF. Allogeneic and xenogeneic transplantation of adipose-derived stem cells in immunocompetent recipients without immunosuppressants. Stem Cells Dev. (2012) 21:2770–8. doi: 10.1089/scd.2012.0176
24. Ashour, RH, Saad, MA, Sobh, MA, Saad, MA, Sobh, MA, al-Husseiny, F, et al. Comparative study of allogenic and xenogeneic mesenchymal stem cells on cisplatin-induced acute kidney injury in Sprague-Dawley rats. Stem Cell Res Ther. (2016) 7:1–17. doi: 10.1186/s13287-016-0386-0
25. Amann, EM, Rojewski, MT, Rodi, S, Fürst, D, Fiedler, J, Palmer, A, et al. Systemic recovery and therapeutic effects of transplanted allogenic and xenogenic mesenchymal stromal cells in a rat blunt chest trauma model. Cytotherapy. (2018) 20:218–31. doi: 10.1016/j.jcyt.2017.11.005
26. Gutiérrez-Fernández, M, Rodríguez-Frutos, B, Ramos-Cejudo, J, Otero-Ortega, L, Fuentes, B, Vallejo-Cremades, MT, et al. Comparison between xenogeneic and allogeneic adipose mesenchymal stem cells in the treatment of acute cerebral infarct: proof of concept in rats. J Transl Med. (2015) 13:46–10. doi: 10.1186/s12967-015-0406-3
27. Tsai, SY, Huang, YC, Chueh, LL, Yeh, LS, and Lin, CS. Intra-articular transplantation of porcine adipose-derived stem cells for the treatment of canine osteoarthritis: a pilot study. World J Transplant. (2014) 4:196. doi: 10.5500/wjt.v4.i3.196
28. Punzón, E, Salgüero, R, Totusaus, X, Mesa-Sánchez, C, Badiella, L, García-Castillo, M, et al. Equine umbilical cord mesenchymal stem cells demonstrate safety and efficacy in the treatment of canine osteoarthritis: a randomized placebo-controlled trial. J Am Vet Med Assoc. (2022) 260:1947–55. doi: 10.2460/javma.22.06.0237
29. Kern, S, Eichler, H, Stoeve, J, Klüter, H, and Bieback, K. Comparative analysis of mesenchymal stem cells from bone marrow, umbilical cord blood, or adipose tissue. Stem Cells. (2006) 24:1294–301. doi: 10.1634/stemcells.2005-0342
30. Li, X, Bai, J, Ji, X, Li, R, Xuan, Y, and Wang, Y. Comprehensive characterization of four different populations of human mesenchymal stem cells as regards their immune properties, proliferation and differentiation. Int J Mol Med. (2014) 34:695–704. doi: 10.3892/ijmm.2014.1821
31. Schnabel, LV, Fortier, LA, McIlwraith, CW, and Nobert, KM. Therapeutic use of stem cells in horses: which type, how, and when? Vet J. (2013) 197:570–7. doi: 10.1016/j.tvjl.2013.04.018
32. Nagamura-Inoue, T, and He, H. Umbilical cord-derived mesenchymal stem cells: their advantages and potential clinical utility. World. J Stem Cells. (2014) 6:195. doi: 10.4252/wjsc.v6.i2.195
33. Sultana, T, Lee, S, Yoon, HY, and Lee, JI. Current status of canine umbilical cord blood-derived mesenchymal stem cells in veterinary medicine. Stem Cells Int. (2018) 2018:1–14. doi: 10.1155/2018/8329174
34. Crisostomo, PR, Wang, M, Wairiuko, GM, Morrell, ED, Terrell, AM, Seshadri, P, et al. High passage number of stem cells adversely affects stem cell activation and myocardial protection. Shock. (2006) 26:575–80. doi: 10.1097/01.shk.0000235087.45798.93
35. Smith, JR, Pfeifer, K, Petry, F, Powell, N, Delzeit, J, and Weiss, ML. Standardizing umbilical cord mesenchymal stromal cells for translation to clinical use: selection of GMP-compliant medium and a simplified isolation method. Stem Cells Int. (2016) 2016:1–14. doi: 10.1155/2016/6810980
36. Carrade, DD, and Borjesson, DL. Immunomodulation by mesenchymal stem cells in veterinary species. Comp Med. (2013) 63:207–17.
37. Morton, CM, Reid, J, Scott, EM, Holton, LL, and Nolan, AM. Application of a scaling model to establish and validate an interval level pain scale for assessment of acute pain in dogs. Am J Vet Res. (2005) 66:2154–66. doi: 10.2460/ajvr.2005.66.2154
38. Reid, J, Nolan, AM, Hughes, JML, Lascelles, D, Pawson, P, and Scott, EM. Development of the short-form Glasgow composite measure pain scale (CMPS-SF) and derivation of an analgesic intervention score. Anim Welf. (2007) 16:97–104. doi: 10.1017/S096272860003178X
39. Kornicka, K, Szłapka-Kosarzewska, J, Śmieszek, A, and Marycz, K. 5-Azacytydine and resveratrol reverse senescence and ageing of adipose stem cells via modulation of mitochondrial dynamics and autophagy. J Cell Mol Med. (2019) 23:237–59. doi: 10.1111/jcmm.13914
40. Bourebaba, L, Bedjou, F, Röcken, M, and Marycz, K. Nortropane alkaloids as pharmacological chaperones in the rescue of equine adipose-derived mesenchymal stromal stem cells affected by metabolic syndrome through mitochondrial potentiation, endoplasmic reticulum stress mitigation and insulin resistance alleviation. Stem Cell Res Ther. (2019) 10:178. doi: 10.1186/s13287-019-1292-z
41. Carrade Holt, DD, Wood, JA, Granick, JL, Walker, NJ, Clark, KC, and Borjesson, DL. Equine mesenchymal stem cells inhibit T cell proliferation through different mechanisms depending on tissue source. Stem Cells Dev. (2014) 23:1258–65. doi: 10.1089/scd.2013.0537
42. Van Pham, P, Bich, N, and Phan, N. Umbilical cord-derived stem cells (ModulatistTM) show strong immunomodulation capacity compared to adipose tissue-derived or bone marrow-derived mesenchymal stem cells. Biomed Res Ther. (2016) 3:29. doi: 10.7603/s40730-016-0029-1
43. Tuffs, SW, James, DB, Bestebroer, J, Richards, AC, Goncheva, MI, O’Shea, M, et al. The Staphylococcus aureus superantigen SElX is a bifunctional toxin that inhibits neutrophil function. PLoS Pathog. (2017) 13:e1006461. doi: 10.1371/journal.ppat.1006461
44. Maki, CB, Beck, A, Wallis, CCC, Choo, J, Ramos, T, Tong, R, et al. Intra-articular Administration of Allogeneic Adipose Derived MSCs reduces pain and lameness in dogs with hip osteoarthritis: a double blinded, randomized, placebo controlled pilot study. Front Vet Sci. (2020) 7:570. doi: 10.3389/fvets.2020.00570
45. Peeters, CM, Leijs, MJ, Reijman, M, van Osch, GJ, and Bos, PK. Safety of intra-articular cell-therapy with culture-expanded stem cells in humans: a systematic literature review. Osteoarthr Cartil. (2013) 21:1465–73. doi: 10.1016/j.joca.2013.06.025
46. El-Tookhy, O, AbouElkheir, W, Mokbel, A, and Osman, A. Intra-articular injection of autologous mesenchymal stem cells in experimental chondral defects in dogs. Egypt Rheumatol. (2008) 30:1–10.
47. Mokbel, AN, El Tookhy, OS, Shamaa, AA, Rashed, LA, Sabry, D, and El Sayed, AM. Homing and reparative effect of intra-articular injection of autologus mesenchymal stem cells in osteoarthritic animal model. BMC Musculoskelet Disord. (2011) 12:259. doi: 10.1186/1471-2474-12-259
48. Kim, YS, Kim, YI, and Koh, YG. Intra-articular injection of human synovium-derived mesenchymal stem cells in beagles with surgery-induced osteoarthritis. Knee. (2021) 28:159–68. doi: 10.1016/j.knee.2020.11.021
49. Hahn, G. VICH GL43 Target Animal Safety: Pharmaceuticals. London, 22 September 2008 Doc. Ref. EMEA/CVMP/VICH/393388/2006 (2009)
50. European Medicines Agency. VICH GL44 Target Animal Safety for Veterinary Live and Inactivated Vaccines. London, 22 September 2008 Doc. Ref. EMEA/CVMP/VICH/359665/2005 (2008)
51. European Medicines Agency. Stem Cell-Based Products for Veterinary Use: Specific Question on Target Animal Safety Addressed by CVMP/ADVENT. London: European Medicines Agency (2020).
52. Shah, K, Drury, T, Roic, I, Hansen, P, Malin, M, Boyd, R, et al. Outcome of allogeneic adult stem cell therapy in dogs suffering from osteoarthritis and other joint defects. Stem Cells Int. (2018) 2018:1–7. doi: 10.1155/2018/7309201
53. Sanabria-de la Torre, R, Quiñones-Vico, MI, Fernández-González, A, Sánchez-Díaz, M, Montero-Vílchez, T, Sierra-Sánchez, Á, et al. Alloreactive immune response associated to human mesenchymal stromal cells treatment: a systematic review. J Clin Med. (2021) 10:2991. doi: 10.3390/jcm10132991
54. Berglund, AK, Fortier, LA, Antczak, DF, and Schnabel, LV. Immunoprivileged no more: measuring the immunogenicity of allogeneic adult mesenchymal stem cells. Stem Cell Res Ther. (2017) 8:288. doi: 10.1186/s13287-017-0742-8
55. Rosa, GDS, Krieck, AMT, Padula, ET, Stievani, FC, Rossi, MC, Pfeifer, JPH, et al. Production of cytotoxic antibodies after intra-articular injection of allogeneic synovial membrane mesenchymal stem cells with and without LPS administration. Front Immunol. (2022) 13:871216. doi: 10.3389/fimmu.2022.871216
56. Margiana, R, Markov, A, Zekiy, AO, Hamza, MU, Al-Dabbagh, KA, Al-Zubaidi, SH, et al. Clinical application of mesenchymal stem cell in regenerative medicine: a narrative review. Stem Cell Res Ther. (2022) 13:366. doi: 10.1186/s13287-022-03054-0
57. Matas, J, Orrego, M, Amenabar, D, Infante, C, Tapia-Limonchi, R, Cadiz, MI, et al. Umbilical cord-derived mesenchymal stromal cells (MSCs) for knee osteoarthritis: repeated MSC dosing is superior to a single MSC dose and to hyaluronic acid in a controlled randomized phase I/II trial. Stem Cells Transl Med. (2019) 8:215–24. doi: 10.1002/sctm.18-0053
58. Gonzalez-Rey, E, Anderson, P, González, MA, Rico, L, Büscher, D, and Delgado, M. Human adult stem cells derived from adipose tissue protect against experimental colitis and sepsis. Gut. (2009) 58:929–39. doi: 10.1136/gut.2008.168534
59. Zhou, B, Yuan, J, Zhou, Y, Ghawji, M Jr, Deng, YP, Lee, AJ, et al. Administering human adipose-derived mesenchymal stem cells to prevent and treat experimental arthritis. Clin Immunol. (2011) 141:328–37. doi: 10.1016/j.clim.2011.08.014
60. Choi, EW, Shin, IS, Park, SY, Park, JH, Kim, JS, Yoon, EJ, et al. Reversal of serologic, immunologic, and histologic dysfunction in mice with systemic lupus erythematosus by long-term serial adipose tissue-derived mesenchymal stem cell transplantation. Arthritis Rheum. (2012) 64:243–53. doi: 10.1002/art.33313
61. Garcia-Pedraza, E, de Miguel, AG, Gomez de Segura, IA, and Pérez, AP. Immunological safety assessment of a single and repeated intra-articular administration of xenogeneic equine umbilical cord mesenchymal stem cells under field conditions in young healthy dogs: a randomized double-blind placebo-controlled study. Res Vet Sci Med. (2022) 2:2. doi: 10.25259/RVSM_3_2021
62. Van Hecke, L, Magri, C, Duchateau, L, Beerts, C, Geburek, F, Suls, M, et al. Repeated intra-articular administration of equine allogeneic peripheral blood-derived mesenchymal stem cells does not induce a cellular and humoral immune response in horses. Vet Immunol Immunopathol. (2021) 239:110306. doi: 10.1016/j.vetimm.2021.110306
63. Depuydt, E, Broeckx, SY, Chiers, K, Patruno, M, da Dalt, L, Duchateau, L, et al. Cellular and humoral immunogenicity investigation of single and repeated allogeneic Tenogenic primed mesenchymal stem cell treatments in horses suffering from tendon injuries. Science. (2022) 8:8. doi: 10.3389/fvets.2021.789293
64. Kalaszczynska, I, and Ferdyn, K. Wharton’s jelly derived mesenchymal stem cells: Future of regenerative medicine? Recent findings and clinical significance. BioMed Res Int. (2015) 2015:1–11. doi: 10.1155/2015/430847
65. Gershwin, LJ, Netherwood, KA, Norris, MS, Behrens, NE, and Shao, MX. Equine IGE responses to non-viral vaccine components. Vaccine. (2012) 30:7615–20. doi: 10.1016/j.vaccine.2012.10.029
66. Mogues, T, Li, J, Coburn, J, and Kuter, DJ. IgG antibodies against bovine serum albumin in humans--their prevalence and response to exposure to bovine serum albumin. J Immunol Methods. (2005) 300:1–11. doi: 10.1016/j.jim.2005.01.022
67. Atkinson, MA, Bowman, MA, Kao, KJ, Campbell, L, Dush, PJ, Shah, SC, et al. Lack of immune responsiveness to bovine serum albumin in insulin-dependent diabetes. N Engl J Med. (1993) 329:1853–8. doi: 10.1056/NEJM199312163292505
68. Passero, LF, Carvalho, AK, Bordon, ML, Bonfim-Melo, A, Carvalho, K, Kallás, EG, et al. Proteins of Leishmania (Viannia) shawi confer protection associated with Th1 immune response and memory generation. Parasit Vectors. (2012) 5:64. doi: 10.1186/1756-3305-5-64
69. Martin, MD, and Badovinac, VP. Defining memory CD8 T cell. Front Immunol. (2018) 9:2692. doi: 10.3389/fimmu.2018.02692
70. Baran, J, Kowalczyk, D, Mariola, O, and Zembala, M. Three-color flow cytometry detection of intracellular cytokines in peripheral blood mononuclear cells: comparative analysis of Phorbol Myristate acetate-ionomycin and phytohemagglutinin stimulation. Clin Diagn Lab Immunol. (2001) 8:303–13. doi: 10.1128/CDLI.8.2.303-313.2001
Keywords: xenogeneic MSC transplantation, umbilical cord mesenchymal stem cell, xenogeneic, safety, intra-articular administration, dogs, mesenchymal stem cell, DogStem
Citation: Punzón E, García-Castillo M, Rico MA, Padilla L and Pradera A (2023) Local, systemic and immunologic safety comparison between xenogeneic equine umbilical cord mesenchymal stem cells, allogeneic canine adipose mesenchymal stem cells and placebo: a randomized controlled trial. Front. Vet. Sci. 10:1098029. doi: 10.3389/fvets.2023.1098029
Edited by:
Gustavo Rosa, São Paulo State University (UNESP), BrazilReviewed by:
Laura Barrachina, University of Galway, IrelandBruna De Vita, São Paulo State University, Brazil
Copyright © 2023 Punzón, García-Castillo, Rico, Padilla and Pradera. This is an open-access article distributed under the terms of the Creative Commons Attribution License (CC BY). The use, distribution or reproduction in other forums is permitted, provided the original author(s) and the copyright owner(s) are credited and that the original publication in this journal is cited, in accordance with accepted academic practice. No use, distribution or reproduction is permitted which does not comply with these terms.
*Correspondence: Almudena Pradera, apradera@equicord.com