- 1Key Laboratory of Medical Cell Biology, Clinical Medicine Research Center, Affiliated Hospital of Inner Mongolia Medical University, Hohhot, Inner Mongolia, China
- 2College of Life Science, Inner Mongolia University, Hohhot, Inner Mongolia, China
- 3Animal Husbandry Institute, Inner Mongolia Academy of Agricultural & Animal Husbandry Sciences, Hohhot, Inner Mongolia, China
- 4Center of Reproductive Medicine, Inner Mongolia People's Hospital, Hohhot, Inner Mongolia, China
Introduction: In the present study, the synergistic protective effect of co-supplementation of glutathione (GSH) with selenium nanoparticles (SeNPs) on the cryopreservation efficiency of bull semen was analyzed.
Methods: After collection, the ejaculates of Holstein bulls were subsequently diluted with a Tris extender buffer supplemented with different concentrations of SeNPs (0, 1, 2, and 4 μg/ml), followed by semen equilibration at 4°C and assessment of sperm viability and motility. Subsequently, the ejaculates of Holstein bulls were pooled, split into four equal groups, and diluted with a Tris extender buffer supplemented with basic extender (negative control group, NC group), 2 μg/ml SeNPs (SeNPs group), 4 mM GSH (GSH group), and 4 mM GSH plus 2 μg/ml SeNPs (GSH + SeNPs group). After cryopreservation, motility, viability, mitochondrial activity, plasma membrane integrity, acrosome integrity, concentration of malondialdehyde (MDA), superoxide dismutase (SOD), and catalase (CAT), and ability of frozen-thawed sperm cells to support in vitro embryonic development were evaluated.
Results and discussion: No side effect of SeNPs concentrations applied in the current study on the motility and viability of equilibrated bull spermatozoa was found. Meanwhile, supplementation of SeNPs significantly promoted the motility and viability of equilibrated bull spermatozoa. Furthermore, the co-supplementation of GSH with SeNPs effectively protected bull spermatozoa from cryoinjury as expressed by promoting semen motility, viability, mitochondrial activity, plasma membrane integrity, and acrosome integrity. Finally, the enhanced antioxidant capacity and embryonic development potential in the frozen-thawed bull spermatozoa cryopreserved by co-supplementation of GSH with SeNPs further confirmed the synergistic protective effect of co-supplementation of GSH with SeNPs on the cryopreservation of bull semen.
Highlights
- 1, 2 and 4 μg/ml SeNPs have no toxic effects on bull spermatozoa.
- Co-supplementation of GSH with SeNPs protects against the cryoinjury of cryopreserved bull spermatozoa.
- Co-supplementation of GSH with SeNPs enhance the ability of frozen-thawed spermatozoa to support in vitro development.
Introduction
The technology of semen cryopreservation effectively promotes the application of technologies including genetic resource conservation, zootechnical management, and artificial insemination (1, 2). In addition, the widely practiced applications of semen cryopreservation significantly enhance the reproductive efficiency and commercial value of livestock breeding (3, 4).
However, the cold shock and alterations in atmospheric oxygen seriously led to biochemical, biophysical, ultrastructural, molecular, and functional alterations (5–7). These alterations in turn trigger excessive production and accumulation of reactive oxygen species (ROS) in mammalian semen (8–11). The imbalance between free radicals and the antioxidant defense system in semen caused by altered ROS production levels led to oxidative stress and osmotic stressors with subsequent acrosomal damage, mitochondrial membrane depolarization, and disruption of plasma membrane permeability (12–16). In addition, oxidative stress-related damage is positively related to genome instability, loss of sperm quality, and fertilization failure (2, 17).
To ameliorate oxidative stress-related damage, the supplementations of antioxidants, including vitamins, coenzymes, exosomes, liposomes, nanomaterials and trace elements such as selenium (Se), copper, and zinc to semen extender have been widely conducted (18–21). As an immune-enhancing micronutrient with excellent antioxidant and anticancer properties, Se plays important role in regulating the mammalian antioxidant system and participates in mammalian spermatogenesis (22). Sperm production and testicular size in Se-deficient rats and mice are seriously disturbed and the stability of sperm mitochondrial sheath is also significantly affected (22). In addition, dietary deficiency of Se inhibits sperm motility and fertility potentials of broiler breeder males (23). These earlier studies found that Se supplementations in the form of selenium nanoparticles (SeNPs) effectively inhibit spermatotoxicity caused by exposure to aflatoxin B1 (24) or cisplatin (25) by reducing spermatic DNA fragmentation and damage. Furthermore, Se supplementations significantly promote the frozen-thawed sperm quality of roosters (21), camels (19), and ram (26). Khalil et al. (27) also found that Se supplementations significantly reduced the chromatin damage in bovine spermatozoa, further convincing the potential applications of SeNPs supplementations in the cryopreservation of mammalian semen. However, these previous studies revealed the low toxicity threshold of Se (>4 μg Se/gm dietary diet), making the application of SeNPs supplementation to the cryopreservation of mammalian semen still needed to be optimized (21, 28, 29).
According to a previous study, the co-supplementation of vitamin E effectively ameliorates the low toxicity threshold of Se (21). However, the potential effect of co-supplementation of glutathione (GSH) with SeNPs on the cryopreservation efficiency of bull spermatozoa has not been reported to date.
GSH, an endogenous antioxidant synthesized from different amino acids including glycine, cysteine, and glutamate, effectively inhibits oxidative stress-related damage and altered ROS production level (30, 31). Our past studies have confirmed the free radicals scavenging potentials of exogenous GSH supplementation on mammalian reproduction potentials (32, 33). Excitingly, exogenous GSH supplementation provides an excellent cryoprotective effect on humans (34), mice (35), jacks (36), canines (37, 38), bulls (39–42), roosters (43–45), ram (46, 47), red deer (48), and boar (49–52) spermatozoa by reducing oxidative stress-related damage. Therefore, it's reasonable to hypothesize that the co-supplementation of GSH with SeNPs would have a synergistic protective effect on the cryopreservation efficiency of bull spermatozoa. This study was designed to investigate the possible synergistic effect of GSH and SeNPs on the post-thawing laboratory indicators of sperm quality, oxidative-antioxidant status, and ability of spermatozoa to support in vitro development.
Materials and methods
Animals
In this study, five healthy Holstein bulls from Furuijin Animal Husbandry Biotechnology Co., Ltd. (Hohhot, Inner Mongolia) with known fertility were randomly selected for the following experiments (53, 54). The bulls were housed individually in pens and fed under uniform feeding conditions throughout the experiments.
Chemicals
Unless otherwise indicated, the chemicals, culture medium, and supplements used in this study were purchased from Sigma-Aldrich (Shanghai, China) and Thermo Fisher (Beijing, China). SeNPs with a particle diameter of 40–60 nm were kindly gifted by Mrs. Mengqi Wang from the College of Chemistry and Chemical Engineering at Weifang University and dissolved with the basic semen extender as the working solution.
Semen collection
For semen collection, bull ejaculates were collected twice a week for 6 weeks with an artificial vagina pre-warmed to 42°C according to the department's protocols (55). Once collected, semen quality parameters in each sample (n = 12) were assessed under a phase contrast microscope (CI-L, Nikon, Tokyo, Japan). After the assessment, each bull ejaculate (n = 9) with semen volume ≥4 ml, sperm cell concentration ≥1.0 × 109/ml, progressive motility ≥75%, and sperm viability ≥80% were split into aliquots according to the experimental design and applied for the following experiments (27).
Optimization of SeNPs concentration for semen cryopreservation
The basic semen extender used in this study was a Tris-based extender as distilled water supplemented with 28 mM fructose, 104 mM citric acid, 297 mM Tris buffer, 5.0% (v/v) glycerol, and 20% (v/v) egg yolk.
To optimize SeNPs concentration for subsequent semen cryopreservation, three semen samples were individually split into four equals and diluted to a final concentration of 6.0 × 107 spermatozoa/ml with the basic extender supplemented with 0 μg/ml SeNPs, 1 μg/ml SeNPs, 2 μg/ml SeNPs, and 4 μg/ml SeNPs. Dose selections for SeNPs were conducted according to previous publications with minor modifications (26, 27). Subsequently, diluted semen samples were loaded into 0.25 ml polyvinyl chloride straws, cooled slowly to 4°C in 2 h, and equilibrated at 4°C for a subsequent 2 h. After equilibration, semen samples were collected for the viability and motility assay.
Semen cryopreservation, drug treatments, and experimental group settings
Before semen cryopreservation, six semen samples were individually split into 4 equals and diluted to a final concentration of 6.0 × 107/ml spermatozoa with the basic extender supplemented with 0 μg/ml SeNPs and 0 mM GSH (negative control group, NC), 2 μg/ml SeNPs (SeNPs group) (27), 4 mM GSH (GSH group) (50) and 4 mM GSH plus 2 μg/ml SeNPs (GSH + SeNPs group). Dose selections for GSH were conducted according to previous publications (5, 56).
For semen cryopreservation, semen samples from each group after dilution were loaded onto semen straws and labeled. After equilibration, the labeled semen straws were inserted into a programmable freezer (IVM Technologies, France). The temperature applied in this study was initially reduced at a programmed rate of −5°C/min from 4°C to −10°C, then at a programmed rate of −40°C/min from −10 to −100°C and finally at a programmed rate of −20°C/min from −100 to −140°C. After cryopreservation, semen straws from each group were immersed in liquid nitrogen for storage.
Thawing process and quality evaluations of frozen-thawed semen
Two weeks after cryopreservation, frozen straws of each group were picked up and thawed in a 37°C water bath for 30 s (27).
The frozen-thawed sperm motility was visually assessed. Frozen-thawed samples from each group (repeated in triplicated) were placed on glass slides covered with a cover. After the percentage record of linear motile spermatozoa at 200× magnification under a phase contrast microscope in three different microscopic fields for each sample, the final motility ratio of frozen-thawed sperm motility in each group was calculated based on the mean of three successive estimations (27, 55, 57).
The frozen-thawed sperm viability was analyzed by eosin-nigrosin staining (55, 58). Briefly, frozen-thawed samples from each group (repeated in triplicated) were collected and incubated with the eosin-nigrosin solution at 25°C for 2 min and smeared on warm glass slides. The slides were air-dried and examined at 400× magnification under a light microscope. For microscope examination, sperm with unstained sperm were counted as alive and pink-stained sperm were counted as dead. The sperm viability of each group was calculated as the proportion of live spermatozoa/five hundred spermatozoa.
Giemsa staining of frozen-thawed spermatozoa (repeated in triplicated) was performed for the acrosome integrity assay (36). After Giemsa staining at room temperature for 3 h (59, 60), the slides were thoroughly rinsed with distilled water, air-dried, and mounted. For microscope examination at 400× magnification under a phase contrast microscope, 500 spermatozoa per group were classified as viable spermatozoa with intact acrosome stained with pink and non-viable spermatozoa with damaged acrosome stained with dark blue or lavender. The acrosome integrity of different groups was further analyzed.
After incubation with hypo-osmotic swelling solution [HOS, containing 6.75 g/L fructose and 3.67 g/L sodium citrate] at 37°C for 1 h, plasma membrane integrity of each group (repeated in triplicated)was analyzed by evaluating the modifications of sperm tail to score swollen sperm according to previous publications (27, 61).
For mitochondrial function assay, methyl thiazolyl diphenyl-tetrazolium bromide (MTT) assay was performed. Briefly, frozen-thawed spermatozoa from each group (repeated in triplicated) were collected, centrifuged at 500× g for 10 min, and diluted with phosphate buffer saline (PBS) solution. Subsequently, 10 μl MTT solutions were added to 100 μl sperm suspension and incubated at 37°C for 1 h. After MTT incubation, the optical density (OD) value at 550 nm of each group was further recorded with a Multiskan GO spectrophotometer (Thermo Scientific, Beijing, China) (62–65).
Assessment of antioxidant enzyme activities and oxidative stress markers
To assess antioxidant enzyme activities and oxidative stress markers, frozen-thawed spermatozoa of each group (repeated in triplicated) were collected and centrifuged at 800×g for 20 min to separate spermatozoa from seminal plasma. The collected spermatozoa were washed with PBS solution and centrifuged at 800×g for 20 min. After centrifugation, spermatozoa suspension was sonicated for 10 s on ice repeating six times at intervals of 30 s (66). Subsequently, the homogenate was centrifuged at 800×g for 15 min and the levels of MDA, SOD, and CAT in each group were individually measured by commercial assay kits according to the manufacturer's instructions.
Oocyte collection and in vitro maturation
For in vitro maturation (IVM), bovine ovaries, collected from a local slaughterhouse, were kept in normal saline and transported to the laboratory within 2 h. Once in the laboratory, ovaries were thoroughly washed with 70% ethanol and PBS solution to avoid contamination. Subsequently, cumulus-oocyte complexes (COCs) with a diameter of about 100–130 μm and a central oocyte surrounded by at least three layers of cumulus cells were collected according to the department's protocols (32).
After collection, COCs were transferred to 500 μl of IVM droplets (40–50 COCs per droplet) covered by mineral oil and cultured for a subsequent 24 h in a CO2 incubator (38.5°C and 5% CO2). IVM medium applied in this study was TCM-199 medium supplemented with 0.3 mM sodium pyruvate, 1 μg/ml β-2-oestradiol, 2 mM GlutaMAX, 10 ng/ml EGF, 10% fetal bovine serum, 10 U/ml FSH, 10 U/ml LH and 100 μM cysteamine (67–69).
In vitro fertilization and embryonic development analyses
COCs after IVM were collected and transferred to HEPES-synthetic oviductal fluid (H-SOF) medium supplemented with 400 IU/ml hyaluronidase. After incubation at 38°C for 3 min, COCs were gently pipetted to remove surrounding cumulus cells. After microscopic examination, oocytes at metaphase II stages (MII, with the polar body extrusion) were collected and cultured in 500 μl of fertilization droplets as SOF medium supplemented with 5% fetal bovine serum, 2 mM sodium pyruvate, 10 μg/ml heparin sodium and 1 mM caffeine in a CO2 incubator (38.5°C, 90%N2, 5%CO2 and 5%O2) (32).
For in vitro fertilization (IVF), the frozen-thawed semen of each group was placed in the 15 ml tube containing the Percoll density gradient. After centrifugation at 500×g for 15 min (27, 70), semen samples from each group (n = 3) were collected with the number of frozen-thawed spermatozoa adjusted to a final concentration of 1 × 106 sperms/ml, added to the above fertilization droplet containing MII oocytes (repeated in triplicate, 205 oocytes for the NC group, 210 oocytes for the GSH group, 220 oocytes for the SeNPs group and 217 oocytes for the GSH + SeNPs group, repeated in triplicate) and cultured in a CO2 incubator (38.5°C, 5%CO2 and 5%O2) for 18 h. Subsequently, fertilized oocytes from each group were transferred individually to embryo culture droplets as SOF medium supplemented with 0.5 mM sodium citrate, 1 mM sodium pyruvate, 1% non-essential amino acids, 2% essential amino acids, 2 mM GlutaMAX, 2.8 mM myo-inositol and 4 mg/ml bovine serum albumin covered by mineral oil in a 35 mm sterile dish, followed by embryo culture in a CO2 incubator (38.5°C, 5%O2 and 5%CO2) (71).
After 48 h, cleaved two-cell embryos from each group were microscopically observed with cleavage rates calculated as the percentage of cleaved two-cell embryos/MII oocytes. Subsequently, embryos of each group were cultured in vitro for a further 7 days with the culture medium changed every other day. After embryo culture, the rates of blastocyst development from cleaved two-cell embryos in each group were microscopically observed and calculated as the percentage of blastocysts/cleaved two-cell embryos. Finally, blastocysts from each group were collected and incubated with 5 μg/ml Hoechst 33342 solution at 38°C for 5 min, followed by blastocysts mounting and microscope examination with cell number per blastocyst (n = 20 for each group) further recorded.
Statistical analysis
The experimental data were analyzed by the Statistical Package for the Social Sciences (SPSS, IBM, version 19.0) and determined by parametric (LSD test) and non-parametric (Wilcoxon) tests, based on residual normality (Gaussian distribution) and variance homogeneity. All results were presented as mean ± standard deviation and P < 0.05 was considered significant.
Results
Effect of SeNPs supplementation on progressive motility and sperm viability of equilibrated bull spermatozoa
After the equilibration period at 4°C, progressive motility (62.89 ± 2.85% for the 1 μg/ml SeNPs group, 68.44 ± 2.35% for the 2 μg/ml SeNPs and 61.78 ± 5.29% for the 4 μg/ml SeNPs group, Figure 1A) and viability (73.56 ± 3.47% for the 1 μg/ml SeNPs group, 78.22 ± 2.28% for the 2 μg/ml SeNPs and 74.56 ± 3.97% for the 4 μg/ml SeNPs group, Figure 1B) of equilibrated bull spermatozoa in SeNPs supplementation groups were significantly increased compared to those in the 0 μg/ml SeNPs group (57.56 ± 3.47% for progressive motility and 70.11 ± 3.22% for sperm viability; P < 0.05).
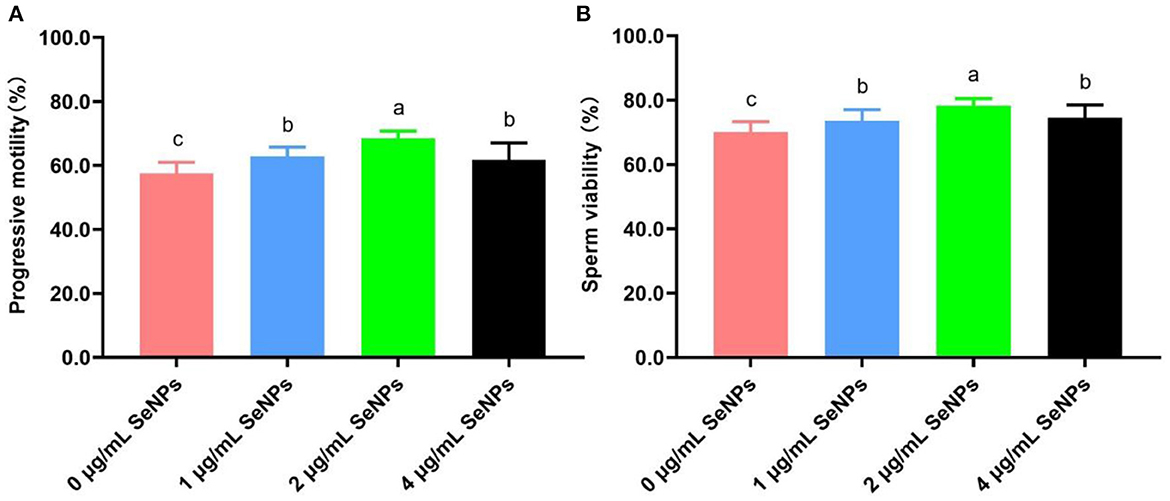
Figure 1. Effect of different concentrations of SeNPs supplementation on motility and viability of equilibrated bull spermatozoa. (A) Motility of equilibrated bull spermatozoa. (B) Viability of equilibrated bull spermatozoa. Labeling with a different lower-case letter in each column indicates significant differences among groups (n = 3, P < 0.05).
In addition, semen motility and viability in the 2 μg/ml SeNPs group were remarkably higher than those in the 1 μg/ml SeNPs and 4 μg/ml SeNPs groups (P < 0.05). At the same time, there was a non-statistical difference in progressive motility and sperm viability between the 1 μg/ml SeNPs and 4 μg/ml SeNPs groups (P > 0.05).
Effect of co-supplementation of GSH with SeNPs on quality of cryopreserved bull semen
As indicated in Figure 2A, the frozen-thawed sperm motility of the GSH + SeNPs group (53.98 ± 2.42%) was significantly higher than that in the NC (39.36 ± 1.86%), GSH (45.87 ± 2.52%) and SeNPs (45.62 ± 1.44%) groups (P < 0.05). In addition, there was a non-statistical difference in the post-thaw sperm motility between the GSH and SeNPs groups (P > 0.05).
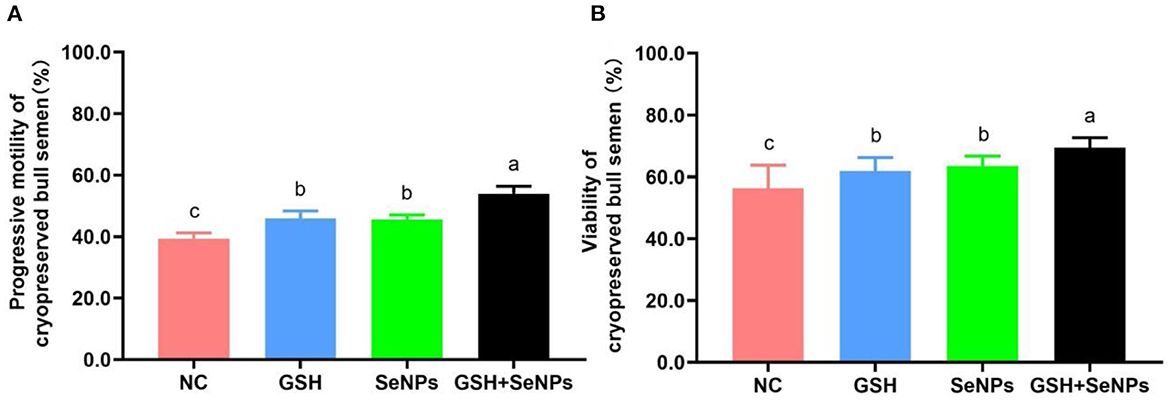
Figure 2. Effect of co-supplementation of GSH and SeNPs on motility and viability of cryopreserved bull spermatozoa. (A) Progressive motility of cryopreserved bull spermatozoa. (B) Viability of cryopreserved bull spermatozoa. Labeling with a different lowercase letter in each column indicates significant differences among groups (n = 6, P < 0.05).
The viability results of frozen-thawed spermatozoa, shown in Figure 2B, were partially consistent with the motility results of frozen-thawed spermatozoa as viability in the GSH + SeNPs group (69.44 ± 3.24%) was significantly higher than that in the NC (56.33 ± 7.43%), GSH (61.89 ± 4.35%) and SeNPs (63.49 ± 3.23%) groups (P < 0.05). At the same time, there was a non-significant difference in the motility of frozen-thawed spermatozoa between the GSH and SeNPs groups (P > 0.05).
Mitochondrial function assay in this study showed that OD550nm values were remarkably up-regulated from the NC (0.38 ± 0.04), GSH (0.43 ± 0.07), SeNPs (0.59 ± 0.04) groups to the GSH + SeNPs group (0.68 ± 0.05; Figure 3A, P < 0.05).
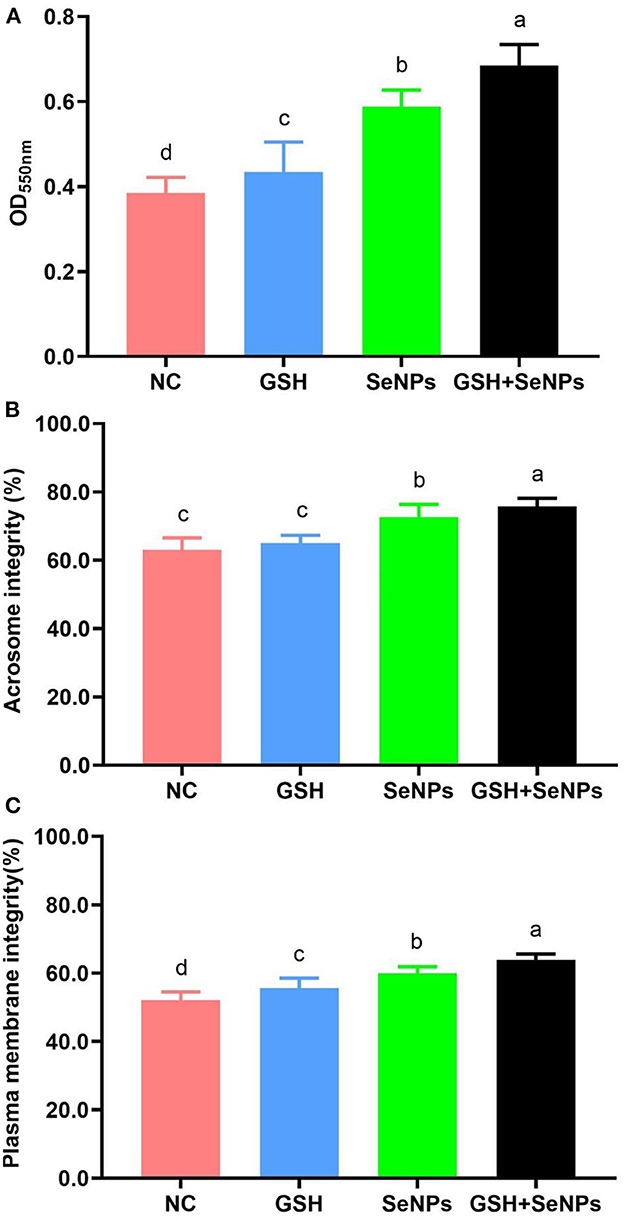
Figure 3. Effect of co-supplementation of GSH and SeNPs on mitochondrial activity, acrosome integrity, and plasma membrane integrity of cryopreserved bull spermatozoa. (A) Mitochondrial activity of cryopreserved bull spermatozoa. (B) Acrosome integrity of cryopreserved bull spermatozoa. (C) Plasma membrane integrity of cryopreserved bull spermatozoa. Labeling with a different lower-case letter in each column indicates significant differences among groups (n = 3, P < 0.05).
The acrosome integrity of each group was also assessed and the results further confirmed that the acrosome integrity was effectively increased from 63.02 ± 3.52% for the NC group, 65.02 ± 2.25% for the GSH group, 72.58 ± 3.72% for the SeNPs group to 75.67 ± 2.47% for the GSH + SeNPs group (Figure 3B, P < 0.05).
Additionally, significant increases in plasma membrane integrity from the NC (52.04 ± 2.46%), GSH (55.67 ± 2.87%), SeNPs (59.89 ± 1.97%) groups to the GSH + SeNPs group (63.82 ± 1.78%) were found in this study (Figure 3C, P < 0.05).
Effect of co-supplementation of GSH with SeNPs on antioxidant potentials of cryopreserved bull spermatozoa
As shown in Figure 4A, co-supplementation of GSH with SeNPs effectively reduced the MDA levels of frozen-thawed spermatozoa compared to that in the NC group as the MDA levels were effectively reduced from 17.61 ± 1.26 nM/ml for the NC group, 14.23 ± 1.04 nM/ml for the SeNPs group, 12.64 ± 1.23 nM/ml for the GSH group to 11.03 ± 1.03 nM/ml for the GSH + SeNPs group (P < 0.05).
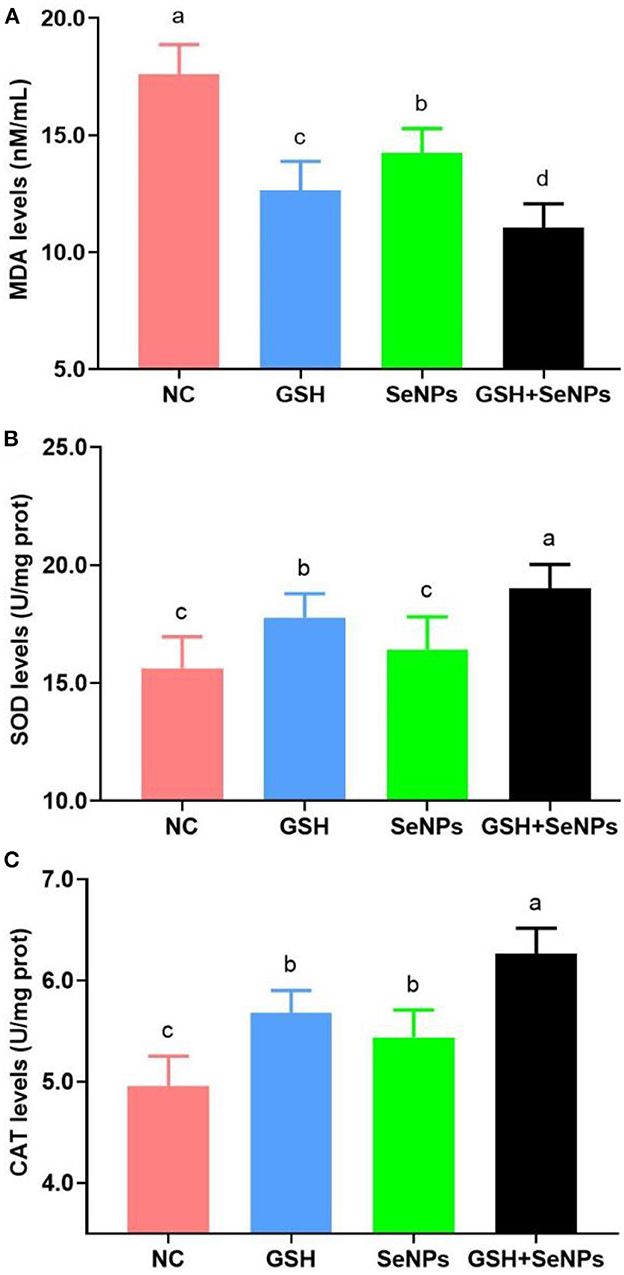
Figure 4. Effect of GSH and SeNPs co-supplementation on antioxidant potentials of cryopreserved bull spermatozoa. (A) MDA levels of cryopreserved bull spermatozoa. (B) SOD levels of cryopreserved bull spermatozoa. (C) CAT levels of cryopreserved bull spermatozoa. Labeling with a different lower-case letter in each column indicates significant differences among groups (n = 3, P < 0.05).
Meanwhile, SOD levels in the GSH + SeNPs (19.01 ± 1.01 U/mg prot) and GSH (17.77 ± 1.02 U/mg prot) groups were significantly elevated compared to those in the NC (15.63 ± 1.33 U/mg prot) and SeNPs (16.42 ± 1.39 U/mg prot) groups (Figure 4B, P < 0.05). Non-significant differences were found in SOD level between the SeNPs and NC groups (P > 0.05). In addition, the SOD level in the GSH + SeNPs group was significantly higher than that in the GSH group (P < 0.05).
Results of CAT levels, shown in Figure 4C, confirmed that CAT levels in the GSH + SeNPs (6.26 ± 0.25 U/mg prot), SeNPs (5.44 ± 0.27 U/mg prot), and GSH (5.68 ± 0.22 U/mg prot) groups were significantly increased compared to the NC group (4.96 ± 0.29 U/mg prot, P < 0.05). At the same time, a significant increase in CAT level was also found in the GSH + SeNPs compared to the SeNPs and GSH groups (P < 0.05).
Effect of co-supplementation of GSH with SeNPs on development potentials of cryopreserved bull spermatozoa
Embryonic development potentials of frozen-thawed spermatozoa were further analyzed to assess the effect of co-supplementation of GSH with SeNPs on the development potentials of cryopreserved bull spermatozoa with the results present in Figure 5.
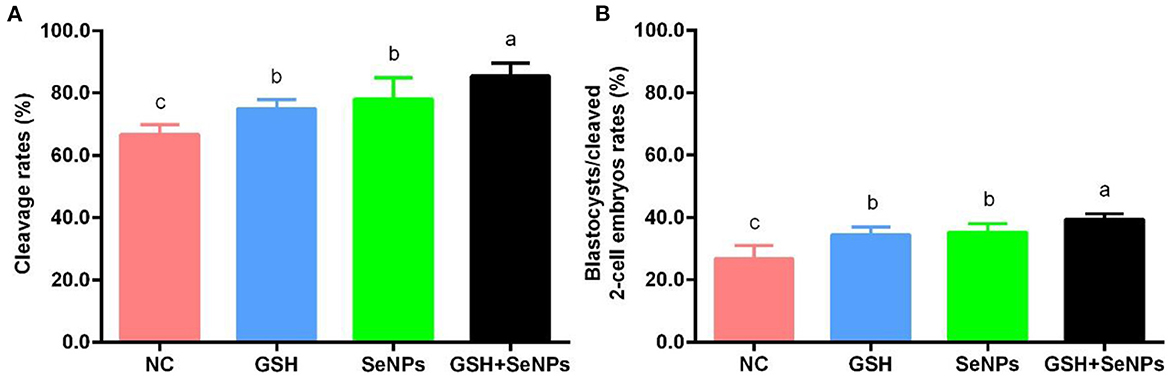
Figure 5. Effect of GSH and SeNPs co-supplementation on cleavage and blastocyst development of cryopreserved bull spermatozoa. (A) Cleavage rates of cryopreserved bull spermatozoa. (B) Percentage of blastocysts/cleaved 2-cell embryos. Labeling with a different lower-case letter in each column indicates significant differences among groups (n = 3, P < 0.05).
After IVF, cleavage rates in the GSH + SeNPs (85.43 ± 4.19%), SeNPs (77.96 ± 6.86%), and GSH (74.94 ± 2.96%) groups were effectively promoted compared to the NC group (66.70 ± 3.06%, Figure 5A, P < 0.05). Meanwhile, there was a non-significant difference in cleavage rates between the SeNPs and GSH groups (P > 0.05).
Results of blastocyst development rates, shown in Figure 5B, confirmed that the development rate of the blastocyst from cleaved two-cell embryos in the GSH + SeNPs group (39.44 ± 1.80%) was significantly higher than those in the NC (26.67 ± 4.26%), GSH (34.40 ± 2.46%), and SeNPs (35.12 ± 2.77%) groups (P < 0.05).
Hoechst 33342 staining of the bovine blastocyst (Figure 6A) was also applied to assess the developmental potentials of cryopreserved bull semen with the cell number/blastocyst of each group analyzed. As indicated in Figure 6B, the cell number/blastocyst in the NC group (84.35 ± 8.29) was significantly lower in comparison with those of the GSH (92.85 ± 7.92), SeNPs (110.30 ± 7.79), and GSH + SeNPs (118.25 ± 10.37) groups (P < 0.05). Meanwhile, the cell number/blastocyst in the GSH + SeNPs group was significantly higher than those of the SeNPs and GSH groups (P < 0.05).
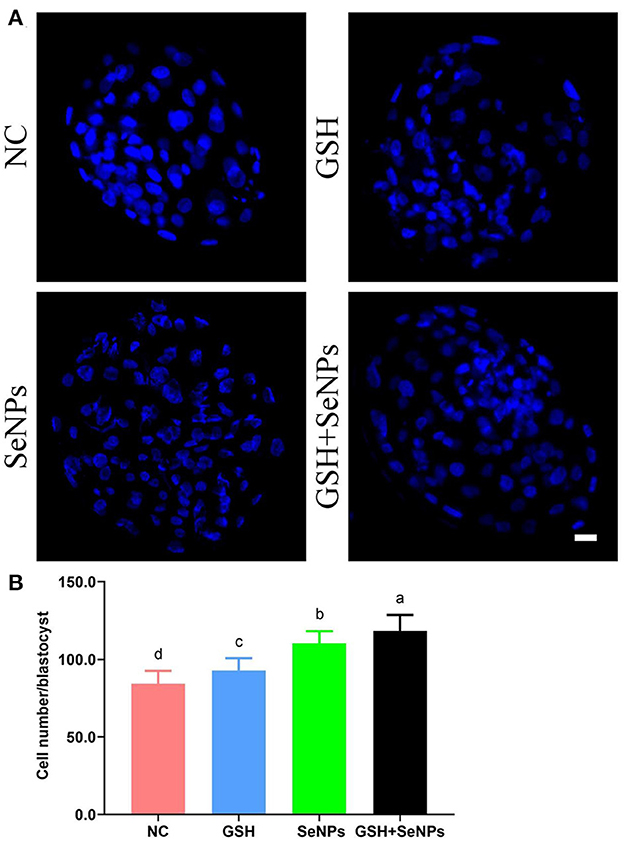
Figure 6. Effect of GSH and SeNPs co-supplementation on blastocyst development of cryopreserved bull spermatozoa. (A) Hoechst 33342 staining of the bovine blastocyst. Scale bar = 20 μm. (B) Cell number/blastocyst of each group. Labeling with a different lowercase letter in each column indicates significant differences among groups (n = 20, P < 0.05).
Discussion
In this study, we found that co-supplementation of GSH with SeNPs has a synergistic protective effect, which is manifested up-regulation of semen motility, viability, mitochondrial activity, plasma membrane integrity, acrosome integrity, antioxidant penitential, and embryonic development.
The promising effect of SeNPs supplementation on the cryopreservation efficiency of bull semen was confirmed in the present study, partially consistent with Khalil's findings (27). Khalil et al. (27) found that the supplementation of commercial SeNPs (with a particle diameter of 87.6 ± 5.81 nm) to the bull semen extender at a level of 0.5 or 1.0 μg/ml promoted motility, viability, and plasma membrane integrity of bull semen with the levels of sperm abnormality and chromatin damage reduced. They also confirmed that excessive supplementation with SeNPs (>1.5 μg/ml) decreased the viability of bull semen and increased the apoptotic rates of bull semen. Meanwhile, the toxicity of 1.5 μg/ml SeNPs supplementation on bull semen was correlated with decreased activity of glutathione peroxidase and increased level of lipid peroxidation, which enhanced the apoptosis of bull semen. To avoid the toxicity of overdose SeNPs supplementation, different concentrations of SeNPs supplementation to the bull semen extender were analyzed in this study. We found that after equilibration, motility and viability of bull spermatozoa in the 2 μg/ml SeNPs group were increased compared to the 0 μg/ml SeNPs, 1 μg/ml SeNPs, and 4 μg/ml SeNPs groups. Our study additionally confirmed the beneficial effect of 2 μg/ml SeNPs supplementation on the effectiveness of cryopreservation of bull semen. Combined with the embryonic developmental potential result of frozen-thawed bull spermatozoa, our study demonstrated the non-existent toxicity of 2 μg/ml SeNPs supplementation on cryopreservation of bull semen. We hypothesized that the reason for the different results compared with Khalil's study may be due to the particle size and component of SeNPs. Accumulating studies have pointed out that the modifications of pore size, particle size, surface charge, and mechanical strength of biomaterials influence their bioactivities and mechanical properties (72–74). However, the bioactivities of SeNPs need further investigations to confirm their bioactivities and application potentials in the cryopreservation of bull semen.
In addition, we found that the co-supplementation of 4 mM GSH with 2 μg/ml SeNPs to the bull semen extender promoted the cryopreservation efficiency of bull semen. El-Batal et al. (75) found that the synthesized incorporation of GSH and SeNPs promoted the antimicrobial activity, anticancer activity, and high DPPH scavenging ability of GSH or selenium dioxide, partly consistent with our findings. We assumed that the synergistic protective effect of co-supplementation of GSH with SeNPs on the cryopreservation efficiency of bull semen may be due to the particular characteristic of nanomaterials on enhancing the antioxidant potentials of GSH (75), which needs further detailed research.
Our former studies have confirmed that GSH supplementation promoted mitochondrial functions of mammalian gametes (32, 33). These former publications have confirmed the beneficial effect of co-supplementation of GSH with BIOXcell extender, raffinose, sucrose, and low-density lipoproteins on the cryopreservation of bull semen (40, 54, 76–79). Our study also confirmed that GSH supplementation promoted motility, viability, plasma membrane integrity, mitochondrial activity, antioxidant ability, and embryonic development of cryopreserved bull spermatozoa. However, the acrosome integrity of frozen-thawed bull spermatozoa was not affected by the GSH supplementation alone. We hypothesize that controversial results about the effectiveness of GSH supplementation alone on the cryopreservation of mammalian semen may be due to the differences in species, extender composition, and GSH concentration (5, 53, 80–82), which still need further detailed investigations.
Moreover, the current study was limited by the lacking of DNA integrity and in vivo fertility assessment to confirm the synergistic protective effect of co-supplementation of GSH with SeNPs on the cryopreservation efficiency of bull semen. We hope that the limitations of the present study will warrant more research to determine the potential effect of co-supplementation of GSH with SeNPs on the cryopreservation efficiency of bull semen.
In conclusion, the co-supplementation of 4 mM GSH with 2 μg/ml SeNPs significantly promoted motility, viability, mitochondrial activity, plasma membrane integrity, acrosome integrity, antioxidant ability, and embryonic development potentials of cryopreserved bull spermatozoa. We sincerely hope that the finding in the present study would benefit the promising applications of co-supplementation of GSH with SeNPs in the future applications of bull semen cryopreservation.
Data availability statement
The original contributions presented in the study are included in the article/supplementary material, further inquiries can be directed to the corresponding author.
Ethics statement
The animal study was reviewed and approved by the Animal Care Committee of Inner Mongolia University. Written informed consent was obtained from the owners for the participation of their animals in this study.
Author contributions
Data curation, formal analysis, project administration, supervision, writing—original draft, and writing—review and editing: GL. Funding acquisition: BW, YM, LS, and YD. Investigation: SL, JR, WZ, BW, YM, LS, YD, and GL. Methodology: GL and YD. Resources: YD. All authors have read and approved the final manuscript.
Funding
This work was supported by the National Natural Science Foundation of China (32002184 to BW), the ZhiYuan project of Inner Mongolia Medical University (ZY0120025 to LS), and the Key Technology Research, Development and Transformation Projects of Inner Mongolia (2021GG0402 to YD and 2022YFSH0102 to YM).
Acknowledgments
We would like to thank all staff from the Clinical Medicine Research Center, Affiliated Hospital of Inner Mongolia Medical University for their professional help.
Conflict of interest
The authors declare that the research was conducted in the absence of any commercial or financial relationships that could be construed as a potential conflict of interest.
Publisher's note
All claims expressed in this article are solely those of the authors and do not necessarily represent those of their affiliated organizations, or those of the publisher, the editors and the reviewers. Any product that may be evaluated in this article, or claim that may be made by its manufacturer, is not guaranteed or endorsed by the publisher.
References
1. Saadeldin IM, Khalil WA, Alharbi MG, Lee SH. The current trends in using nanoparticles, liposomes, and exosomes for semen cryopreservation. Animals. (2020) 10:2281. doi: 10.3390/ani10122281
2. Upadhyay VR, Ramesh V, Dewry RK, Kumar G, Raval K, Patoliya P. Implications of cryopreservation on structural and functional attributes of bovine spermatozoa: an overview. Andrologia. (2021) 53:e14154. doi: 10.1111/and.14154
3. Akhtar MF, Ma Q, Li Y, Chai W, Zhang Z, Li L, et al. Effect of sperm cryopreservation in farm animals using nanotechnology. Animals. (2022) 12:2277. doi: 10.3390/ani12172277
4. Hu E, Yang H, Tiersch TR. High-throughput cryopreservation of spermatozoa of blue catfish (Ictalurus furcatus): establishment of an approach for commercial-scale processing. Cryobiology. (2011) 62:74–82. doi: 10.1016/j.cryobiol.2010.12.006
5. Tuncer P, Bucak MN, Büyükleblebici S, Sariözkan S, Yeni D, Eken A, et al. The effect of cysteine and glutathione on sperm and oxidative stress parameters of post-thawed bull semen. Cryobiology. (2010) 61:303–7. doi: 10.1016/j.cryobiol.2010.09.009
6. Öztürk A, Bodu M, Bucak MN, Agir V, Özcan Sari A, Keskin N, et al. The synergistic effect of trehalose and low concentrations of cryoprotectants can improve post-thaw ram sperm parameters. Cryobiology. (2020) 95:157–63. doi: 10.1016/j.cryobiol.2020.03.008
7. Keskin N, Erdogan C, Bucak MN, Ozturk AE, Bodu M, Ili P, et al. Cryopreservation effects on ram sperm ultrastructure. Biopreserv Biobank. (2020) 18:441–8. doi: 10.1089/bio.2020.0056
8. Khosravizadeh Z, Khodamoradi K, Rashidi Z, Jahromi M, Shiri E, Salehi E, et al. Sperm cryopreservation and DNA methylation: possible implications for ART success and the health of offspring. J Assist Reprod Genet. (2022) 39:1815–24. doi: 10.1007/s10815-022-02545-6
9. Liman MS, Hassen A, Mcgaw LJ, Sutovsky P, Holm DE. Potential use of tannin extracts as additives in semen destined for cryopreservation: a review. Animals. (2022) 12:1130. doi: 10.3390/ani12091130
10. Lone SA, Mohanty TK, Baithalu RK, Yadav HP. Sperm protein carbonylation. Andrologia. (2019) 51:e13233. doi: 10.1111/and.13233
11. Bucak MN, Necmettin T. Kryoprotektanlar ve gamet hücrelerinin dondurulmasinda kryoprotektif etki. Ankara Üniversitesi Vet Fak Derg. (2007) 54:67–72. doi: 10.1501/Vetfak_0000000255
12. Tiwari S, Dewry RK, Srivastava R, Nath S, Mohanty TK. Targeted antioxidant delivery modulates mitochondrial functions, ameliorates oxidative stress and preserve sperm quality during cryopreservation. Theriogenology. (2022) 179:22–31. doi: 10.1016/j.theriogenology.2021.11.013
13. Treulen F, Arias ME, Aguila L, Uribe P, Felmer R. Cryopreservation induces mitochondrial permeability transition in a bovine sperm model. Cryobiology. (2018) 83:65–74. doi: 10.1016/j.cryobiol.2018.06.001
14. Treulen F, Uribe P, Boguen R, Villegas JV. Mitochondrial outer membrane permeabilization increases reactive oxygen species production and decreases mean sperm velocity but is not associated with DNA fragmentation in human sperm. Mol Hum Reprod. (2016) 22:83–92. doi: 10.1093/molehr/gav067
15. Yeste M, Estrada E, Rocha LG, Marín H, Rodríguez-Gil JE, Miró J. Cryotolerance of stallion spermatozoa is related to ROS production and mitochondrial membrane potential rather than to the integrity of sperm nucleus. Andrology. (2015) 3:395–407. doi: 10.1111/andr.291
16. Öztürk A, Bucak MN, Bodu M, Başpin N, Celik I, Shu Z, et al. Cryobiology and cryopreservation of sperm. In: Quain M, editor. Cryobiology. London: IntechOpen (2019), p. 75–116.
17. Len JS, Koh WSD, Tan SX. The roles of reactive oxygen species and antioxidants in cryopreservation. Biosci Rep. (2019) 39:BSR20191601. doi: 10.1042/BSR20191601
18. Cengiz M, Sahinturk V, Yildiz SC, Sahin I K, Bilici N, Yaman SO, et al. Cyclophosphamide induced oxidative stress, lipid per oxidation, apoptosis and histopathological changes in rats: protective role of boron. J Trace Elem Med Biol. (2020) 62:126574. doi: 10.1016/j.jtemb.2020.126574
19. Shahin MA, Khalil WA, Saadeldin IM, Swelum AA, El-Harairy MA. Comparison between the effects of adding vitamins, trace elements, and nanoparticles to SHOTOR extender on the cryopreservation of dromedary camel epididymal spermatozoa. Animals. (2020) 10:78. doi: 10.3390/ani10010078
20. Arangasamy A, Krishnaiah MV, Manohar N, Selvaraju S, Rani GP, Soren NM, et al. Cryoprotective role of organic Zn and Cu supplementation in goats (Capra hircus) diet. Cryobiology. (2018) 81:117–24. doi: 10.1016/j.cryobiol.2018.02.001
21. Safa S, Moghaddam G, Jozani RJ, Daghigh Kia H, Janmohammadi H. Effect of vitamin E and selenium nanoparticles on post-thaw variables and oxidative status of rooster semen. Anim Reprod Sci. (2016) 174:100–6. doi: 10.1016/j.anireprosci.2016.09.011
22. Saaranen M, Suistomaa U, Vanha-Perttula T. Semen selenium content and sperm mitochondrial volume in human and some animal species. Hum Reprod. (1989) 4:304–8. doi: 10.1093/oxfordjournals.humrep.a136893
23. Edens F, Sefton AE. Sel-Plex® Improves spermatozoa morphology in broiler breeder males Int J Poultry Sci. (2009) 8:853–61. doi: 10.3923/ijps.2009.853.861
24. Asadpour R, Aliyoldashi MH, Saberivand A, Hamidian G, Hejazi M. Ameliorative effect of selenium nanoparticles on the structure and function of testis and in vitro embryo development in Aflatoxin B1-exposed male mice. Andrologia. (2020) 52:e13824. doi: 10.1111/and.13824
25. Rezvanfar MA, Rezvanfar MA, Shahverdi AR, Ahmadi A, Baeeri M, Mohammadirad A, et al. Protection of cisplatin-induced spermatotoxicity, DNA damage and chromatin abnormality by selenium nano-particles. Toxicol Appl Pharmacol. (2013) 266:356–65. doi: 10.1016/j.taap.2012.11.025
26. Nateq S, Moghaddam G, Alijani S. The effects of different levels of Nano selenium on the quality of frozen-thawed sperm in ram. J Appl Anim Res. (2020) 48:434–9. doi: 10.1080/09712119.2020.1816549
27. Khalil WA, El-Harairy MA, Zeidan AEB, Hassan MaE. Impact of selenium nano-particles in semen extender on bull sperm quality after cryopreservation. Theriogenology. (2019) 126:121–7. doi: 10.1016/j.theriogenology.2018.12.017
28. Nancharaiah YV, Lens PN. Selenium biomineralization for biotechnological applications. Trends Biotechnol. (2015) 33:323–30. doi: 10.1016/j.tibtech.2015.03.004
29. Forceville X, Van Antwerpen P, Annane D, Vincent JL. Selenocompounds and sepsis-redox bypass hypothesis: part B-selenocompounds in the management of early sepsis. Antioxid Redox Signal. (2022) 37:998–1029. doi: 10.1089/ars.2020.8062
30. Forman HJ, Zhang H, Rinna A. Glutathione: overview of its protective roles, measurement, and biosynthesis. Mol Aspects Med. (2009) 30:1–12. doi: 10.1016/j.mam.2008.08.006
31. Calabrese G, Morgan B, Riemer J. Mitochondrial glutathione: regulation and functions. Antioxid Redox Signal. (2017) 27:1162–77. doi: 10.1089/ars.2017.7121
32. Ren J, Hao Y, Liu Z, Li S, Wang C, Wang B, et al. Effect of exogenous glutathione supplementation on the in vitro developmental competence of ovine oocytes. Theriogenology. (2021) 173:144–55. doi: 10.1016/j.theriogenology.2021.07.025
33. Ren J, Li S, Wang C, Hao Y, Liu Z, Ma Y, et al. Glutathione protects against the meiotic defects of ovine oocytes induced by arsenic exposure via the inhibition of mitochondrial dysfunctions. Ecotoxicol Environ Saf. (2021) 230:113135. doi: 10.1016/j.ecoenv.2021.113135
34. Ghorbani M, Vatannejad A, Khodadadi I, Amiri I, Tavilani H. Protective effects of glutathione supplementation against oxidative stress during cryopreservation of human. Spermatozoa. Cryo Letters. (2016) 37:34–40.
35. Shi X, Hu H, Ji G, Zhang J, Liu R, Zhang H, et al. Protective effect of sucrose and antioxidants on cryopreservation of sperm motility and DNA integrity in C57BL/6 mice. Biopreserv Biobank. (2018) 16:444–50. doi: 10.1089/bio.2018.0037
36. Kumar P, Kumar R, Mehta JS, Chaudhary AK, Ravi SK, Chandra Mehta S, et al. Ameliorative effect of ascorbic acid and glutathione in combating the cryoinjuries during cryopreservation of exotic Jack Semen. J Equine Vet Sci. (2019) 81:102796. doi: 10.1016/j.jevs.2019.102796
37. Lucio CF, Silva LC, Regazzi FM, Angrimani DS, Nichi M, Assumpção ME, et al. Effect of reduced glutathione (GSH) in canine sperm cryopreservation: in vitro and in vivo evaluation. Cryobiology. (2016) 72:135–40. doi: 10.1016/j.cryobiol.2016.02.001
38. Ogata K, Sasaki A, Kato Y, Takeda A, Wakabayashi M, Sarentonglaga B, et al. Glutathione supplementation to semen extender improves the quality of frozen-thawed canine spermatozoa for transcervical insemination. J Reprod Dev. (2015) 61:116–22. doi: 10.1262/jrd.2014-130
39. Shah N, Singh V, Yadav HP, Verma M, Chauhan DS, Saxena A, et al. Effect of reduced glutathione supplementation in semen extender on tyrosine phosphorylation and apoptosis like changes in frozen thawed Hariana bull spermatozoa. Anim Reprod Sci. (2017) 182:111–22. doi: 10.1016/j.anireprosci.2017.05.006
40. Salman A, Caamaño JN, Fernández-Alegre E, Hidalgo CO, Nadri T, Tamargo C, et al. Supplementation of the BIOXcell extender with the antioxidants crocin, curcumin and GSH for freezing bull semen. Res Vet Sci. (2021) 136:444–52. doi: 10.1016/j.rvsc.2021.03.025
41. Olfati Karaji R, Daghigh Kia H, Ashrafi I. Effects of in combination antioxidant supplementation on microscopic and oxidative parameters of freeze-thaw bull sperm. Cell Tissue Bank. (2014) 15:461–70. doi: 10.1007/s10561-013-9412-y
42. Ansari MS, Rakha BA, Andrabi SM, Ullah N, Iqbal R, Holt WV, et al. Glutathione-supplemented tris-citric acid extender improves the post-thaw quality and in vivo fertility of buffalo (Bubalus bubalis) bull spermatozoa. Reprod Biol. (2012) 12:271–6. doi: 10.1016/j.repbio.2012.10.001
43. Masoudi R, Sharafi M, Pourazadi L, Dadashpour Davachi N, Asadzadeh N, Esmaeilkhanian S, et al. Supplementation of chilling storage medium with glutathione protects rooster sperm quality. Cryobiology. (2020) 92:260–2. doi: 10.1016/j.cryobiol.2019.10.005
44. Masoudi R, Sharafi M, Shahneh AZ, Khodaei-Motlagh M. Effects of reduced glutathione on the quality of rooster sperm during cryopreservation. Theriogenology. (2019) 128:149–55. doi: 10.1016/j.theriogenology.2019.01.016
45. Zhandi M, Seifi-Ghajalo E, Shakeri M, Yousefi AR, Sharafi M, Seifi-Jamadi A. Effect of glutathione supplementation to semen extender on post-thawed rooster sperm quality indices frozen after different equilibration times. Cryo Letters. (2020) 41:92–9.
46. Mata-Campuzano M, Álvarez-Rodríguez M, Álvarez M, Tamayo-Canul J, Anel L, De Paz P, et al. Post-thawing quality and incubation resilience of cryopreserved ram spermatozoa are affected by antioxidant supplementation and choice of extender. Theriogenology. (2015) 83:520–8. doi: 10.1016/j.theriogenology.2014.10.018
47. Gadea J, Gumbao D, Gómez-Giménez B, Gardón JC. Supplementation of the thawing medium with reduced glutathione improves function of frozen-thawed goat spermatozoa. Reprod Biol. (2013) 13:24–33. doi: 10.1016/j.repbio.2013.01.174
48. Anel-López L, Garcia-Alvarez O, Maroto-Morales A, Iniesta-Cuerda M, Ramón M, Soler AJ, et al. Reduced glutathione addition improves both the kinematics and physiological quality of post-thawed red deer sperm. Anim Reprod Sci. (2015) 162:73–9. doi: 10.1016/j.anireprosci.2015.09.012
49. Gadea J, Gumbao D, Matás C, Romar R. Supplementation of the thawing media with reduced glutathione improves function and the in vitro fertilizing ability of boar spermatozoa after cryopreservation. J Androl. (2005) 26:749–56. doi: 10.2164/jandrol.05057
50. Giaretta E, Estrada E, Bucci D, Spinaci M, Rodríguez-Gil JE, Yeste M. Combining reduced glutathione and ascorbic acid has supplementary beneficial effects on boar sperm cryotolerance. Theriogenology. (2015) 83:399–407. doi: 10.1016/j.theriogenology.2014.10.002
51. Gadea J, García-Vazquez F, Matás C, Gardón JC, Cánovas S, Gumbao D. Cooling and freezing of boar spermatozoa: supplementation of the freezing media with reduced glutathione preserves sperm function. J Androl. (2005) 26:396–404. doi: 10.2164/jandrol.04155
52. Baishya SK, Biswas RK, Govindasamy K, Deka BC, Sinha S, Singh M. Effect of reduced glutathione, water soluble vitamin E analogue and butylated hydroxytoluene on the post thaw characteristics of boar spermatozoa. Cryo Letters. (2018) 39:227–34.
53. Oliveira Resende C, Pedroso Betarelli R, Rabelo SS, Resende Chaves B, Rodriguez-Gil JE, Zangeronimo MG. Addition of insulin-like growth factor I (IGF-I) and reduced glutathione (GSH) to cryopreserved boar semen. Anim Reprod Sci. (2019) 208:106130. doi: 10.1016/j.anireprosci.2019.106130
54. Topraggaleh TR, Shahverdi A, Rastegarnia A, Ebrahimi B, Shafiepour V, Sharbatoghli M, et al. Effect of cysteine and glutamine added to extender on post-thaw sperm functional parameters of buffalo bull. Andrologia. (2014) 46:777–83. doi: 10.1111/and.12148
55. Liu G, Pan B, Li S, Ren J, Wang B, Wang C, et al. Effect of bioactive peptide on ram semen cryopreservation. Cryobiology. (2020) 97:153–8. doi: 10.1016/j.cryobiol.2020.08.007
56. Nadri T, Towhidi A, Zeinoaldini S, Riazi G, Sharafi M, Zhandi M, et al. Supplementation of freezing medium with encapsulated or free glutathione during cryopreservation of bull sperm. Reprod Domest Anim. (2022) 57:515–23. doi: 10.1111/rda.14088
57. Badr M, Rawash Z, Azab A, Dohreg R, Ghattas T, Fathi M. Spirulina platensis extract addition to semen extender enhances cryotolerance and fertilizing potentials of buffalo bull spermatozoa. Anim Reprod. (2021) 18:e20200520. doi: 10.1590/1984-3143-ar2020-0520
58. Mahiddine FY, You I, Park H, Kim MJ. Commensal Lactobacilli enhance sperm qualitative parameters in dogs. Front Vet Sci. (2022) 9:888023. doi: 10.3389/fvets.2022.888023
59. Ahmed H, Andrabi SMH, Jahan S. Semen quality parameters as fertility predictors of water buffalo bull spermatozoa during low-breeding season. Theriogenology. (2016) 86:1516–22. doi: 10.1016/j.theriogenology.2016.05.010
60. Shoae A, Zamiri MJ. Effect of butylated hydroxytoluene on bull spermatozoa frozen in egg yolk-citrate extender. Anim Reprod Sci. (2008) 104:414–8. doi: 10.1016/j.anireprosci.2007.07.009
61. Sánchez-Caycho K, Santolaria P, Soler C, Yániz J. Effect of hypoosmotic swelling test and water test on the distribution of sperm subpopulations in bull. Anim Reprod Sci. (2016) 169:101. doi: 10.1016/j.anireprosci.2016.03.024
62. Al Naib A, Hanrahan JP, Lonergan P, Fair S. In vitro assessment of sperm from bulls of high and low field fertility. Theriogenology. (2011) 76:161–7. doi: 10.1016/j.theriogenology.2010.10.038
63. Aziz DM. Assessment of bovine sperm viability by MTT reduction assay. Anim Reprod Sci. (2006) 92:1–8. doi: 10.1016/j.anireprosci.2005.05.029
64. Buranaamnuay K. The MTT assay application to measure the viability of spermatozoa: a variety of the assay protocols. Open Vet J. (2021) 11:251–69. doi: 10.5455/OVJ.2021.v11.i2.9
65. Aziz DM, Ahlswede L, Enbergs H. Application of MTT reduction assay to evaluate equine sperm viability. Theriogenology. (2005) 64:1350–6. doi: 10.1016/j.theriogenology.2005.02.009
66. Bucak MN, Tuncer PB, Sariözkan S, Başpinar N, Taşpinar M, Coyan K, et al. Effects of antioxidants on post-thawed bovine sperm and oxidative stress parameters: antioxidants protect DNA integrity against cryodamage. Cryobiology. (2010) 61:248–53. doi: 10.1016/j.cryobiol.2010.09.001
67. Mondal S, Mor A, Reddy IJ, Nandi S, Gupta PSP, Mishra A. In vitro embryo production in Sheep. Methods Mol Biol. (2019) 2006:131–40. doi: 10.1007/978-1-4939-9566-0_9
68. Shirazi A, Shams-Esfandabadi N, Ahmadi E, Heidari B. Effects of growth hormone on nuclear maturation of ovine oocytes and subsequent embryo development. Reprod Domest Anim. (2010) 45:530–6. doi: 10.1111/j.1439-0531.2008.01290.x
69. Fidanza A, Toschi P, Zacchini F, Czernik M, Palmieri C, Scapolo P, et al. Impaired placental vasculogenesis compromises the growth of sheep embryos developed in vitro. Biol Reprod. (2014) 91:21. doi: 10.1095/biolreprod.113.113902
70. Oliveira LZ, Hossepian De Lima VF, Levenhagen MA, Santos RM, Assumpção TI, Jacomini JO, et al. Transmission electron microscopy for characterization of acrosomal damage after Percoll gradient centrifugation of cryopreserved bovine spermatozoa. J Vet Sci. (2011) 12:267–72. doi: 10.4142/jvs.2011.12.3.267
71. Wang M, Ren J, Liu Z, Li S, Su L, Wang B, et al. Beneficial effect of selenium doped carbon quantum dots supplementation on the in vitro development competence of ovine oocytes. Int J Nanomedicine. (2022) 17:2907–24. doi: 10.2147/IJN.S360000
72. Bharadwaz A, Jayasuriya AC. Recent trends in the application of widely used natural and synthetic polymer nanocomposites in bone tissue regeneration. Mater Sci Eng C Mater Biol Appl. (2020) 110:110698. doi: 10.1016/j.msec.2020.110698
73. Najeeb S, Khurshid Z, Zafar MS, Khan AS, Zohaib S, Martí JM, et al. Modifications in glass ionomer cements: nano-sized fillers and bioactive nanoceramics. Int J Mol Sci. (2016) 17:1134. doi: 10.3390/ijms17071134
74. Ha HK, Rankin SA, Lee MR, Lee WJ. Development and characterization of whey protein-based nano-delivery systems: a review. Molecules. (2019) 24:3254. doi: 10.3390/molecules24183254
75. El-Batal AI, Ragab YM, Amin MA, El-Roubi GM, Mosallam FM. Investigating the antimicrobial, antioxidant and cytotoxic activities of the biological synthesized glutathione selenium nano-incorporation. Biometals. (2021) 34:815–29. doi: 10.1007/s10534-021-00309-w
76. Takahashi Y, Kanagawa H. Effects of glutamine, glycine and taurine on the development of in vitro fertilized bovine zygotes in a chemically defined medium. J Vet Med Sci. (1998) 60:433–7. doi: 10.1292/jvms.60.433
77. Tuncer PB, Sariözkan S, Bucak MN, Ulutaş PA, Akalin PP, Büyükleblebici S, et al. Effect of glutamine and sugars after bull spermatozoa cryopreservation. Theriogenology. (2011) 75:1459–65. doi: 10.1016/j.theriogenology.2010.12.006
78. Sariözkan S, Bucak MN, Tuncer PB, Büyükleblebici S, Cantürk F. Influence of various antioxidants added to TCM-199 on post-thaw bovine sperm parameters, DNA integrity and fertilizing ability. Cryobiology. (2014) 68:129–33. doi: 10.1016/j.cryobiol.2014.01.007
79. Amirat-Briand L, Bencharif D, Vera-Munoz O, Bel Hadj Ali H, Destrumelle S, Desherces S, et al. Effect of glutamine on post-thaw motility of bull spermatozoa after association with LDL (low density lipoproteins) extender: preliminary results. Theriogenology. (2009) 71:1209–14. doi: 10.1016/j.theriogenology.2008.10.002
80. Shah S, Qureshi IZ. Freezability of water buffalo bull (Bubalus bubalis) spermatozoa is improved with the addition of curcumin (diferuoyl methane) in semen extender. Andrologia. (2017) 49:1–10. doi: 10.1111/and.12713
81. Foote RH, Brockett CC, Kaproth MT. Motility and fertility of bull sperm in whole milk extender containing antioxidants. Anim Reprod Sci. (2002) 71:13–23. doi: 10.1016/S0378-4320(02)00018-0
Keywords: SeNPs, GSH, oxidative stress, bull, semen cryopreservation
Citation: Li S, Ren J, Zhang W, Wang B, Ma Y, Su L, Dai Y and Liu G (2023) Glutathione and selenium nanoparticles have a synergistic protective effect during cryopreservation of bull semen. Front. Vet. Sci. 10:1093274. doi: 10.3389/fvets.2023.1093274
Received: 08 November 2022; Accepted: 20 January 2023;
Published: 16 February 2023.
Edited by:
Ayman Abdel-Aziz Swelum, Zagazig University, EgyptReviewed by:
Amany Abdel-Rahman Mohamed, Zagazig University, EgyptHany Abdalla, Zagazig University, Egypt
Mahmoud Hussein Hadwan, University of Babylon, Iraq
Essam A. Almadaly, Kafrelsheikh University, Egypt
Copyright © 2023 Li, Ren, Zhang, Wang, Ma, Su, Dai and Liu. This is an open-access article distributed under the terms of the Creative Commons Attribution License (CC BY). The use, distribution or reproduction in other forums is permitted, provided the original author(s) and the copyright owner(s) are credited and that the original publication in this journal is cited, in accordance with accepted academic practice. No use, distribution or reproduction is permitted which does not comply with these terms.
*Correspondence: Gang Liu, 21408010@mail.imu.edu.cn
†These authors have contributed equally to this work
‡ORCID: Shubin Li orcid.org/0000-0002-6865-9667
Jingyu Ren orcid.org/0000-0002-0048-3052
Wenqi Zhang orcid.org/0000-0003-4891-500X
Yanfeng Dai orcid.org/0000-0001-7147-1748
Gang Liu orcid.org/0000-0002-5180-3765