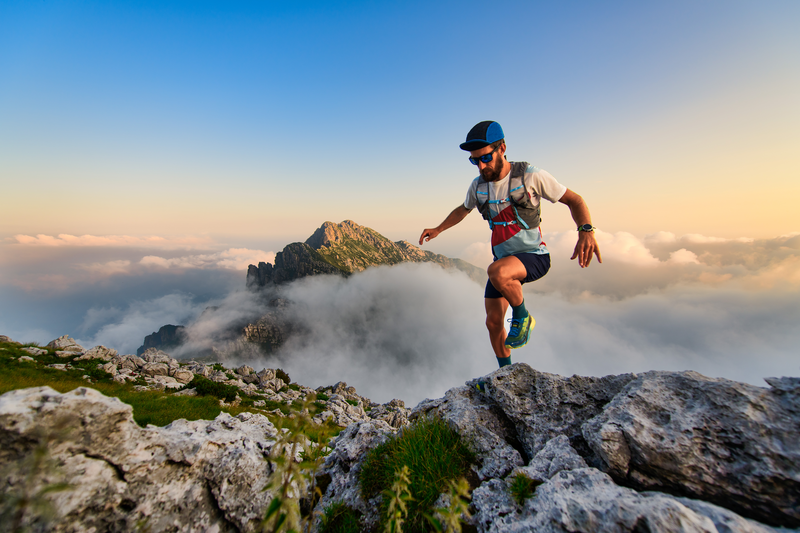
94% of researchers rate our articles as excellent or good
Learn more about the work of our research integrity team to safeguard the quality of each article we publish.
Find out more
REVIEW article
Front. Vet. Sci. , 02 February 2023
Sec. Animal Behavior and Welfare
Volume 10 - 2023 | https://doi.org/10.3389/fvets.2023.1086985
This article is part of the Research Topic Farm Animal Welfare and Climate Change: Methods of Assessment and Mitigation View all 6 articles
Yaks play an important role in the livelihood of the people of the Qinghai-Tibet Plateau (QTP) and contribute significantly to the economy of the different countries in the region. Yaks are commonly raised at high altitudes of ~ 3,000–5,400 m above sea level. They provide many important products, namely, milk, meat, fur, and manure, as well as social status, etc. Yaks were domesticated from wild yaks and are present in the remote mountains of the QTP region. In the summer season, when a higher quantity of pasture is available in the mountain region, yaks use their long tongues to graze the pasture and spend ~ 30–80% of their daytime grazing. The remaining time is spent walking, resting, and doing other activities. In the winter season, due to heavy snowfall in the mountains, pasture is scarce, and yaks face feeding issues due to pasture scarcity. Hence, the normal body weight of yaks is affected and growth retardation occurs, which consequently affects their production performance. In this review article, we have discussed the domestication of yaks, the feeding pattern of yaks, the difference between the normal and growth-retarded yaks, and also their microbial community and their influences. In addition, blood biochemistry, the compositions of the yaks' milk and meat, and reproduction are reported herein. Evidence suggested that yaks play an important role in the daily life of the people living on the QTP, who consume milk, meat, fur, use manure for fuel and land fertilizer purposes, and use the animals for transportation. Yaks' close association with the people's well-being and livelihood has been significant.
The QTP is a region where the majority of yaks (Bos grunniens) are concentrated. Yaks are very old bovines that live at an altitude ranging from 3,000 to 5,400m with low atmospheric oxygen pressure. Yaks play a critical role in the lives of the local population and also the ecological niche in the ecosystem of the Plateau (1). People living in the QTP obtain milk, meat, fuel, and fur from yaks. Yaks are endothermic animals that can tolerate impaired oxygen supply. Normally, these yaks are semi-domesticated grazing animals, while natural breeding takes place and no formulated feed is commonly provided for them (2). Besides production, including meat and milk, yaks are also used for transportation purposes. The meat of yaks contribute significantly to the economy of the local people and the meat is popular among the people living in the QTP (3, 4).
The environment of the QTP is very harsh, particularly in the cold season, with a long snowfall season from October to May (Temperature −15 to −05). In the QTP, these months of long snowfall and cold weather cause a shortage of grass, and the grass is covered with a thick layer of snow, which affects the survival of these yaks (5). In addition, this shortage of grass during the winter season also causes malnutrition and retarded growth in yaks. In the cold season, the birth rate of calves is at its peak. Yaks normally conceive during late summer and give birth in the early summer (6). Due to a shortage of green fodder in harsh weather, calves face growth retardation (5). Hence, in the QTP, growth-retarded yaks are a common issue each year. Yaks are the world's most notable animals living and reproducing freely in such harsh plateau conditions. In addition, early nomadic people domesticated yaks more than 7,300 years ago and yaks are the only major species of livestock that coexist in the same ecosystem as their wild forebears (7). Among all livestock animals, yaks are the only domesticated animals that can survive and adjust to the cold and harsh environment of the QTP and face high food scarcity (8).
Earlier studies showed that malnutrition during the early life of animals damages the gastrointestinal tract function and structure, affecting the normal growth of the animals (2, 9). The use of different feed additives in the fodder of growth-retarded yaks can improve the structure and function of the gastrointestinal tract of the animals because the health of the gastrointestinal tract is important for the proper absorption of nutrients from the tract and to increase the growth of the animals (10). The bacterial community of the gastrointestinal tract also plays a crucial role in the growth and production performance of the animals (11). In addition, a stable bacterial community is crucial for the host to maintain the health and internal environment of the gastrointestinal tract (12).
Yaks play a crucial role in the life of the people of Tibet, as they not only provide milk and meat but also hide, fur, and manure for land fertilization. In addition, the fur of yaks is used in the preparation of the local black tents that retain moisture when it rains, preventing tents from leaking. The long horns of yaks are used for the preparation of milk pails and other materials (13). The manure of yaks is not only used as a fertilizer but is also used as a construction material in walls, enclosures, and other different types of houses, as well as in the preparation of toys. It is observed that yaks are commonly crossbred with cattle to produce F1 hybrid dzo, which is a higher producer of milk compared to yaks and can survive in high altitudes (6). Yaks have the ability to adapt to the harsh environment of the winter season and live at high altitudes on the Plateau (Figure 1). The hide of yaks is very thick and helps the yak to protect its body from the cold and to keep it warm. Anatomically, its greater heart and lung size and the higher foraging ability of its adapted tongue allows the yak to eat the low-lying grasses easily in the area of the Plateau (14). The yak's genetic makeup allows for a unique ability to adapt the low-oxygen conditions of the QTP as compared to cattle (14).
Figure 1. A brief diagram of the domestication, feeding, microbiota, and harsh environment of the QTP.
Yaks are domesticated in different countries, such as Pakistan, Nepal, China, India, Kyrgyzstan, Russia, Tajikistan, Afghanistan, Bhutan, and Mongolia (Joshi), consisting of northern and southern zones connected in the west by the Pamir mountains. The entire QTP, east of Sichuan until Baltistan in the west, the Himalayas in Nepal, Bhutan, and India fall in the southern zone (15). This region shows the maximum range of the Tibetosphere, mostly occupied by speakers of Tibetic languages (16), but also speakers of Indo-Iranian (Indo-European), Burushaski (an isolated language), Mongolic, Turkic, and Sino-Tibetan languages that are culturally and linguistically influenced by Tibetan languages. Inconveniently, there is little research and literature available on the domestication of yaks in the archaeological department. In the Nuomohong sites on the northeastern Plateau, such as Xiariyamakebu and Tawendaliha (3,300–2,700 BP), physical evidence of yak skeletal remains was discovered; nevertheless, only their presence was reported, and it was unclear if they showed signs of domestication or even what domestication signs might look like in yaks. In Talitaliha (3,300–2,700 BP), a clay sculpture of a yak demonstrated the importance this animal may have held for the inhabitants of the site (13). In Qugong, a yak skull that dates to roughly 3,650 BC was also unearthed in an ashpit (17). The researchers contended that due to the horn's small size, it was probably from a domesticated yak or one hybridized with cattle. Yak skulls dating to 450 CE were also present in Samdzong 5 (18).
Yaks were domesticated from wild yaks (Bos mutus) and are present in the remote mountains of the QTP (19). Very little research was conducted regarding the feeding system of yaks (20, 21). Particularly in the summer season, when pasture covered with long grass is available in the mountain region, yaks use their long tongues for ingestion, while in short grass, they use lips and incisor teeth for ingestion (22) (Figure 1). In the winter season, when the pasture is fully covered with a big coat of snow, yaks use their hooves, horns, heads, and muzzles to remove the thick snow layers (23). This process helps the development of their sensory olfactory organs. However, in the scarcity period, yaks use the stem and leaves from residual wilted grasses and plants. In the winter season, yaks utilize more energy in grazing compared to the summer season. In summer, with the growth of new green herbage, the grazing time increases and even extends to midnight. Yaks spend more time idling in winter and early spring (March and April) since less herbage is available, and they spend more time searching or lying down to avoid the cold and strong winds (21). Growing and lactating yaks spend more time grazing because of their higher nutrient requirements. During snowfall and hail storms, yaks run over the pasture at very high speeds, of ~3,400 m/h (24). Yaks graze on alpine pastures, such as alfalfa, oak, and other herbages that are commonly available in mountain regions. Normally, yaks avoid grazing onpoisonous plants but in scarcity periods due to a shortage of pasture, they graze on poisonous weeds and suffer from poisoning. The use of different concentrates and urea molasses blocks is effective in improving the production of grazing yaks and maintaining body weight in winter. Yaks consume less feed compared to cattle due to their small rumen size and capacity (25). Yaks prefer fresh grass and high temperature affects their feed intakes. Yaks normally drink water from rivers and in the absence of water, particularly in the winter season, they lick ice to meet their water requirement (26). Ma et al. (27) concluded that the addition of glutamine in the diet of yaks improved the growth of slow-growing yaks, as wel l as gastrointestinal morphology and barrier function. In another study, study authors reported that the supplementation of the diet of grazing yaks with multi-nutrient blocks improved body weight gain and improved the health of yaks (28). Zhao et al. (29) reported that rumen-protected methionine (50 g/day) supplementation in the diet of lactating yaks increased the dry matter intake, milk production, milk protein content, and lactoferrin concentration. In addition, rumen protect methionine also improved the rumen fermentation characteristics in lactating yaks. The concentrate supplementation (CP 18%, 12.49 MJ ME/Kg) of grazing yaks during the warm season improves body weight gain and ruminal fermentation efficiency, mainly by an increase in MCP and VFA productions and by lowering acetate to propionate ratio. In addition, supplementation of concentrates reduced bacterial richness, diversity indices, and relative abundance of succiniclasticum, Prevotellaceae_UCG_003, Prevotel-laceae_UCG_005, and Ruminococcus_1, while they enhanced the relative abundance of the Christensenellaceae_R-7_group and the Ruminococca-ceae_NK4A214_group (30). In addition we have mentioned the effects of different feed supplements on the growth and production performance of yaks in Table 1.
The QTP environment is very harsh, particularly during the cold season due to high snowfall from October to May (Temperature −15 to −05). Due to this high snowfall and cold season, the shortage of grass occurs during these months, which affects the survival of yaks (38). In addition, this shortage of grass during the winter season also causes malnutrition and retarded growth in yaks (Figure 1). During the cold season, the birth rate of calves is at its peak, and due to a shortage of green fodder, calves face growth retardation (5). Therefore, at the QTP, growth-retarded yaks are a common issue each year. Usually, yak growth retardation is defined as the yak's body weight being twice the standard deviation below the average weight of a yak population of the same breed and age (39). During the early life span, severe malnutrition (pregnancy and neonatal periods) affects the growth of the animals and causes growth-retarded yaks. These growth-retarded yaks are commonly found in the QTP with lower body weight and high morbidity and mortality rates (38). Hu et al. (39) stated that the serum somatotropic hormone axis concentration is significantly reduced in growth-retarded yaks in comparison to normal yaks. Hu et al. (1) showed that the initial body weight of growth-retarded yaks was significantly lower than that of healthy yaks. Ma et al. (8) concluded that growth performance of the growth-retarded yaks was poor in contrast to normal yaks, and the average daily gain and final body weight of growth-retarded yaks were differently lower than the control group. Dai et al. (30) demonstrated that supplementation improved the body weight of yaks compared to grazing-only yaks and that supplementation also increased the average daily gain of these yaks when compared to grazing-only yaks.
The microbiota in the gut plays an important role in the digestion of food and absorption of nutrients from the diet, in regulating the storage of fat, in stimulating intestinal epithelial renewal, and in directing immune system maturation (40). Ma et al. (2) reported that in the rumen and colon of normal yaks and growth-retarded yaks the alpha-diversity analysis showed that the observed species, Chao1, ACE, and Shannon indexes were similar between groups. The Shannon index in the duodenum and ileum of the growth-normal yak group was higher when compared to that of the growth-retarded yak group. However, in the jejunum and cecum, an opposite trend was observed. In the duodenum and ileum, no significant difference was found in species Chao1, and in ACE indexes in either group. Nonetheless, in the jejunum and cecum of growth-normal yaks, the observed species Chao1, and ACE indexes were lower compared to the growth-retarded yaks. In addition, the Bray–Curtis dissimilarity matrices, when using the PCoA to examine the structure of microflora in the GIT between the growth-normal yak and growth-retarded yak groups, and the bacterial communities in the rumen, duodenum, jejunum, ileum, and cecum were separated from each other (2).
It was noted that out of 32 phyla from 12 rumen samples, the number of bacteria phyla found in normal-growth and retarded-growth yaks was 29 and 31, respectively. In the rumen of the yaks, Firmicutes and Bacteroidetes were the predominant bacteria, accounts for at 75% of the total relative abundance of all bacteria. The relative abundance of the Spirochetes was higher in normal-growth yaks when compared to retarded-growth yaks. However, Actinobacteria and Patescibacteria relative abundance was found to be higher in the retarded-growth yaks than in the normal-growth yaks. In addition, Firmicutes and Tenericutes relative abundance were found to be higher in normal-growth yaks than in retarded-growth yaks. However, Chloroflexi relative abundance showed an opposite trend between the two groups. No significant difference in the Firmicutes-to-Bacteroidetes ratio was found in the normal-growth and retarded-growth yaks. The level of genus of the Rikenellaceae RC9 gut group was found to be the most dominant bacterium in the rumen digesta of the normal-growth and retarded-growth yaks, followed by Bacteroidales unclassified F082, Christensenellaceae R-7 group, and Prevotella 1. The relative abundance of Treponema 2, Ruminococcaceae UCG-014, and Ruminococcaceae NK4A214 were found to be higher in normal-growth yaks than in retarded-growth yaks. The relative abundance of the group of Christensenellaceae R-7 was found to be higher in retarded-growth yaks than in normal-growth yaks. Furthermore, the relative abundance of the group of Bacteroidales UCG-001, was found to be slightly higher in normal-growth yaks than in retarded-growth yaks. LEfSe analysis further classified different genera according to the relative abundance of different bacteria. In the rumen of normal-growth and retarded-growth yaks, a group of 13 bacteria was found in Ruminococcaceae UCG-014, Christensenellaceae R-7 group, and Treponema 2, respectively. Du et al. (41) conducted research on growth-retarded calves and supplemented their diet with the probiotic Bacillus amyloliquefaciens C-1 and found that body weight, feed intake, and efficiency of feed conversion ratio of the growth-retarded calves were increased compared to the control group. Moreover, the abundance of bacteria contributing to the production of energy and saturated fatty acids (short-chain fatty acids), including Proteobacteria, Rhodospiril-laceae, Campylobacterales, and Butyricimonas, were increased in growth-retarded calves supplemented with probiotics compared with the control group, and the suspected pathogens, which included Anaeroplasma and Acholeplasma were decreased with probiotic supplementation.
In extremely harsh environments, feed selection and feed intake influence the survival and health of yaks. The microbiota plays a crucial role in the digestion of nutrients, particularly in fiber digestion and synthesis of microbial protein, and helps the host to adapt to the environment (42). Different researchers suggested that feed composition is the main driver altering the microbial population in the gut. In all mammals, gut microbiota constitutes a complex and crucial ecosystem. Recent studies showed that the gut microbiota in yaks plays an important role in adaptation to the harsh environment, namely the cold season, though the composition of the microbiota of the yak is poorly categorized (43). The rumen of the yak is the largest section among all the sections of the gastrointestinal tract and the microbiota in this section plays a crucial role in the digestion of fiber and non-fiber carbohydrates and characterizes the major site of fermentation in yaks.
Su et al. (44) stated that in the gastrointestinal tract of grazing yaks more than three taxa were identified at the Phylum level during the summer season. These phyla were Firmicutes, Bacteroidetes, and Proteobacteria. At the genus level, eight genera were identified in the gastrointestinal tract of the yaks, such as Succiniclasticum (45), Prevotella (46), and Fibrobacter (47). It has been frequently reported from fecal samples that the gut microbiota in yaks are Christensenellaceae_R-7, Ruminococcaceae_UCG-005, Ruminococcaceae_NK4A-214, Rikenellaceae_RC9, Prevotellaceae_UCG-003, and Prevotellaceae_UCG-001, which are implicated in fiber digestion. Moreover, Akkermansia and Bacteroides were associated with enhancing the host immune responses and metabolic functions (48), and Ruminococcaceae_UCG-010 was reported as well. In this experiment, the subsequent downstream analysis selected these taxa as they appear to be the main representatives of gut microbiota in yaks. The rumen is the largest compartment in the yak digestive tract and it is in the rumen thatbacterial fermentation takes place and volatile fatty acids are produced. These volatile fatty acids are the main energy source for yaks. The rumen dominates the physiology of the animal, as well as the microbiota, and has a high concentration of Firmicutes and Bacteriodetes. In the four stomachs compartment, the genera Rikenellaceae_RC9, Prevotella, Christensenellaceae_R-7, and Prevotellaceae_UCG- 001 were found to be most dominant. In the rumen, Prevotella was highly prevalent but its levels displayed a reducing trend toward the reticulum and abomasum (44).
Global warming is caused by greenhouse gases, especially methane gas, which is the main component that directly contributes to global warming. The yak is a local and indigenous animal that produces low methane in the environment when compared to other ruminants living at low altitudes, such as cattle and sheep (3). Xue et al. (49) reported that yak indoor feeding is a newly developing factor that can increase the production of bacteria that are involved in methane production in the gut. Hence, in this respect, using advanced feeding treatments to decrease the production of bacteria that are involved in methane production should receive more attention (44). In the intestine of ruminants, trillions of microbes are present that play an important role in the host's health, as well as in the metabolism, digestion, and absorption of nutrients in the body and homeostasis of the intestine in yaks (50). Furthermore, microbiota in the gut also plays an important function in the maturation of the immune system, permeability, and differentiation of the epithelium of the intestine (51). Statistically, in the healthy intestine, more than 1,014 microbes are available, about tenfold the total quantity of host cells (52). Amid them, bacteria in the intestine account for about 98% of the total population of the microbiota, while fungi (0.1%), viruses, and protozoa (53) comprise the rest. Gut microbiota in stable conditions is needed for numerous physiological and metabolic processes, while the destabilization in the microbiota community may result in different gastrointestinal problems, such as enteritis, diarrhea, and irritable bowel syndrome. Guo et al. (54) researched the microbiota of yaks and found that on 97% of the similarity level, a total of 7,200 OTUs were recognized, and among them, 6,642 OTUs were related to bacteria and only 40 OTUs belonged to archaea. At OTUs levels, a rarefaction test was subsequently conducted.
In addition, Guo et al. (54) reported that in the taxonomy summary of the rumen bacterial community, a total of 23 phyla were noted in the rumen. Among these phyla, the six most abundant phyla were Firmicutes, Bacteroidetes, Proteobacteria, Spirochaetes, Tenericutes, and Verrucomicrobia and the remaining phyla were in lower abundance. At the taxonomic level, a more detailed analysis was done and found that the richest abundance of Proteobacteria contained the six classes, whereas Firmicutes and Bacteroidetes only had three and four classes, respectively, though Bacteroidia and Clostridia were the two predominant classes. At the family level, the most abundant families were Unclassified Bacteroidales, Ruminococcaceae, Unclassified Clostridiales, Lachnospiraceae, BS11, and Prevotellaceae. Other families were also found but at lower percentages. Although these yaks did not have major clinical problems, the growth-retarded yaks faced greater mortality and eccentricity rates and poor feed utilization, which results in greater feed costs. The proper health of the gastrointestinal tract plays a crucial role in the growth performance of animals (55, 56).
Ma et al. (2) researched the microbiota of normal yaks and growth-retarded yaks and concluded that the yak gastrointestinal tract displayed diverse microbiota communities among the groups. In the gastrointestinal tract of the growth-normal yaks the relative abundance of bacteria, such as Ruminococcaceae, Treponema, Clostridiaceae, Prevotellaceae, and Lachnospiraceae, were higher compared to the growth-retarded yaks and these bacteria played a crucial role in the degradation of the oligosaccharide, starch, and cellulose. Furthermore, in the gastrointestinal tract of the growth-retarded yaks the relative abundance of bacteria, such as (Eubacterium) tenue, Christensenellaceae R-7 group, and unclassified Chitinophagaceae, were higher compared to normal yaks. Ma et al. (2) stated that in the gastrointestinal tract of growth-retarded yaks the bacterial community was spared and that regulating the microbial population might be an effective solution to compensate growth of growth-retarded yaks. Xue et al. (57) reported that enteric methane is produced due to the degradation of the plant material by gastrointestinal microbiota in the rumen. In the yak, high throughput sequence data revealed that yak's rumen have highly diverse gastrointestinal microbial communities of unclassified microorganisms. The yak's prokaryotic community remains uncharacterized when compared with other livestock species. In addition, in yaks, the rumen's prokaryotic community consisted of 29 phyla, 40 classes, 63 orders, 77 families, and 79 genera. Bacteroidetes (59.1%) was the most abundant phylum, followed by Firmicutes, Proteobacteria, Fibrobacteres, and Euryarchaeota. Prevotella was the predominant genus, averaging 28.5% of all the rumen's prokaryotic genera. Archaea accounted for 2.26% of the total prokaryotic community, with their community dominated by Methanobacteriaceae (82%), followed by Methanomassiliicoccaceae, and Methanosarcinaceae. Huang et al. (58) investigated the methanogen diversity in yak and cattle by 16S rRNA gene sequences in the rumen and concluded that yaks have a unique ecosystem of microbiota in the rumen that is significantly changed from cattle. This helps explain why yaks produce less methane compared to cattle. In cattle's rumen, billions of microorganisms are present, such as bacteria, methanogenic archaea, protozoa, and fungi. The methanogen genera commonly found in the rumen of cattle are Methanobrevibacter, Methanomicrobium, Methanobacterium, and Methanosarcina (59, 60).
The level of glucose in serum reflects the utilization of energy in ruminants. When the level of serum glucose reduces, it indicates a lack of dietary energy or poor utilization of energy (30, 61). Mellado et al. (62) revealed that in goats the diet containing concentrate influenced the glucose concentration. This is because a diet containing a higher concentration of concentrate (350 g/day) contains more energy supply compared to a diet with a lower concentrate. In addition, in normal and healthy conditions, the animal's diet containing higher levels of protein increased the concentration of total protein in the blood (63). Unsurprisingly, diets containing supplements significantly enhanced the levels of total protein in yaks, mainly albumin and globulin (64). Chen et al. (65) showed that the aspartate aminotransferase level in serum improved with the provision of a diet with a high level of concentrate in the case of housing-feeding yaks. Dai et al. (30) stated that the use of different supplements in the diet of grazing yaks enhanced the levels of aspartate aminotransferase, glucose, and total protein as compared to grazing-only yaks. Though, no change was found in triglycerides, urea nitrogen, and alkaline phosphate concentrations between grazing-only and grazing yaks with supplementation. The higher altitude, cold stress, and forage scarcity changed the blood composition of the yaks compared to other bovine species, such as buffalo, cow, goat, and sheep (66). Due to high altitude, yak face chronic hypoxia and this stress affects the performance of the respiratory and cardiovascular systems in yaks. Due to this reason, the yaks have a larger pulmonary alveolar area per unit of time, thinner alveolar septum, and thinner blood-air barrier. In addition, yaks have larger lungs and hearts and greater levels of erythrocytes and hemoglobin compared to other bovine species (Figure 1) (66). Yang et al. (67) concluded that the provision of a higher energy diet for yaks increased the IGF-1 level and relative expressions of IGF-1 and IGFBP-3 and reduced the growth hormone levels and GHR expressions. In addition, seven days of starvation reduced the glucose, triglycerides, and blood urea nitrogen levels in yaks. However, NEFA and BHBA concentrations were fivefold higher in the starvation group compared to control groups, and after seven days of feeding, all of these parameters were found to be normal in the control group (46). Zhou et al. (68) reported that different concentrations of supplements for grazing yak enhanced the levels of albumin, blood urea nitrogen, and total protein compared to the control group. Gao et al. (69) stated that nutritional deficiency in the diet of grazing yaks had a bigger effect than the cold stress during the cold season. Meanwhile, an analysis of the random forest method showed that in the serum of grazing yaks, the metabolic profile related to the metabolism of amino acid and protein was altered and it was affected by nutrient deficiency than by the energy and minerals metabolism in cold conditions. Diet-containing supplements would be beneficial for grazing yaks in cold conditions.
Yaks are seasonal breeders like other bovine species and their mating and conception depend, annually, on the temperate season (70). Normally, yaks produce four to five calves during their entire life and they mature at the age of 3–4 years old (71). Various studies showed that different environmental conditions affect the reproductive cycle of yaks (72). The average length of the estrus cycle in yaks is 20 days, 1 day less than cattle. The signs of the estrus in yaks are not usually clear as in cattle (73). The duration of the gestation period of a yak is lower than that of a cow and lasts for 258 days. The stillbirth and abortion rates of yaks are between 5 and 10%. Normally, bulls mature at the age of 3–4 years old but they can breed properly at the age of 6–7 years old (72). Different authors suggested that maturity, conception rate, and other reproductive traits can be improved by using proper management techniques (5). The breeding season of yaks is affected by seasonal changes and other factors, such as environment, shortage of grass, latitude, and altitude (5, 74, 75). In the summer season, when the increase in temperature and humidity lead to a higher grass growth, yaks improve their body weight, particularly the females, and start their breeding season. Yaks' breeding season is at its peak from July to August as the temperature of the environment is higher compared to other months (72). In addition, this increase in temperature also improves the semen quality of yaks and the highest quality, quantity, and motility of the season observed from June to September and these semen parameters are also affected with time (72). Fertility depends on the number of services rather than the age of the bull. During the cold season, yaks increase their metabolism to maintain body temperature. During food scarcity and unavailability of forage due to cold harsh weather, yaks meet their nutritional requirement by using stored reserve and reducing body weight and body condition score by 17–25% (46).
There is a paucity of data on yak reproduction in the wild. Studies on domestic and wild yaks crossbreeding concentrated on male reproduction, particularly semen traits and hybrid productivity, and make up the majority of the data now available. For thawed and fresh semen, the motility of wild yaks is generally 63 and 39%, respectively (76). At 0 to 4°C, fresh semen can survive for 57 h when diluted with 7% glucose. Semen that has been frozen and then thawed at 37°C survives for 12 h (77). The resistance coefficients of the sperm from domestic yaks, domestic cattle, and wild yaks are 144.000, 12.750, and 6.000, respectively (77). This data demonstrates the exuberant motility of wild yak sperm. Spermatozoa from yaks typically have flagella. The head and centerpieces of yak sperm from wild and domestic animals are similar in length and width, but the main portion is noticeably longer in wild yaks (78). In regions where yaks procreate, the first estrus in females typically happens between the second and fourth warm season after birth, or between the ages of 13 and 36 months. Since yaks are seasonal breeders with a high natural conception rate, the fertility of a yak herd is primarily influenced by the percentage of yak cows that return to estrus in the year of calving. Research conducted at Sichuan revealed that 78% of female herds became pregnant following their first service, 15% following the second, and 7% following the third or more (79). Moreover, puberty onset is significantly influenced by nutritional conditions and the rate of live weight increase, with faster-growing yak heifers experiencing it earlier (79).
Yaks play an important role in the production of meat, milk, fur, and cheese for the people of the QTP (80). As mentioned earlier, the QTP has low temperatures, high altitudes, and low precipitation, and these aspects directly influence plant productivity and their nutritive values (81). Normally, the duration for growth of grass is ~100–150 days, and the dormant period lasts for ~7 months. Each year, grass begins to re-green in April, reaches its peak biomass in August, and withers in November. Yaks usually graze on natural grass all year without receiving additional feed. Due to the long cold season, QTP grassland is withered, and the most serious condition is a heavy snow disaster, in which all the green fodder is covered with heavy thick snow, impeding yaks from accessing any grazing fodder. This causes dramatic loss in the body weight of yaks and mortality. Earlier studies revealed that once food is accessible again in the short-term in the warm season, yaks enter a compensatory growth condition, with better growth rates and feed efficiency (82). With evolution, yaks developed unique physiological traits to acclimatize to extremely high-altitude environments.
It is important to comprehend the genetic makeup of yaks and how it can affect milk biosynthesis because yaks serve as the region's main supply of milk for human consumption. The milk of yaks is highly nutritive compared to dairy cow milk, particularly in protein and fat contents (83). The fat percentage in yak milk ranges from 5.5 to 7.5% as compared to 3.5% in bovine milk and the protein percentage ranges from 4.9 to 5.9% compared to 3.14% in bovine milk (Table 2) (87). The seasonal change in feed, particularly during the cold season, highly affected the traditional farming of yaks (87). Despite pastures' low protein content, using various supplements is not a usual practice (88). Consequently, determining yak milk composition, influenced by fodder grown at diverse phenological periods, is important to hypothetically increase milk quality and production.
When milked during the main lactating period, yak milk is a naturally concentrated milk due to the greater levels of fat (5.7–7.5%), protein (4.0–5.9%), and lactose (4.0–5.9%) (19). The milk and different milk products made from yak milk are the major ingredients in the everyday life of Tibetan herders, and in areas where yaks graze on alpine meadows and mountain pastures, especially for the weak, elderly, ill, and young people of the region (19). Yak milk and various products made from yak milk, such as butter and cheese, are important sources of various vitamins and are critical sources of food for the people of the Tibetan Plateau due to the lack of vegetables, fruits, and the few resources of food (19).
However, the low volume and seasonal cyclicity of individual milk production, of roughly 150–500 kg of fresh milk per lactation, limit the large-scale industrialization of yak milk production. These factors include yak breed, parity, age, body condition, raising areas, pasture quality, pasture growth, milking techniques, milking time, and other environmental factors (84).
Different milk products made from yak milk garner interest due to their special nutritional composition. Many companies in China provide fresh pasteurized and UHT-treated milk. The chemical composition and chemical properties of yak milk are important from an economical point of view, as is the successful establishment of an yak products industry and marketing. The chemical composition of yak milk is different than that of cow milk, as the milk of yak is mainly produced by seasonal breeding. The yak milk composition depends on the availability of seasonal grass and also environmental conditions (89). During the mid-lactation period in yaks, solids like lactose, protein, and amino acids was found to be higher, while the fat content in yak milk continuously increased during the late lactation period (89). In contrast, milk from cow herd differs little with the change of seasons due to cows' year-round breeding, meaning that cow milk composition displayed minimum changes throughout the year (90).
In China, the milk composition of the Maiwa yak breed was studied by Sheng et al. (90), and the researchers focused on the mineral content, nitrogen distribution, and chemical composition of milk in the QTP, China. Furthermore, the composition of yak milk in the cold and warm seasons was established by the researchers, as mentioned in Table 2 (91, 92).
In the QTP region, yaks play a vital role in the living standard of the people due to their production, which contributes to the economy. Yaks provide milk, milk products, meat, and meat products as food items; fur and hide for the textile industry for leather and cloths; and manure for use as soil fertilizer and fuel (93). Normally, in the traditional system, yaks are domesticated and grazed on natural pasture without any supplement feeding. Therefore, in the winter season, the nutritional value of the pasture is reduced, which alternatively affected the growth rate of yaks (94). Long et al. (24) reported that in the cold season, due to low nutritional value in grazing pasture, yaks lose ~25% of their body weight and decrease their growth performance, which harms their economic effectiveness. Due to this situation, the management of yak domestication has changed in recent years. It was decided that a portion of finishing yaks would progressively transition from traditional grazing to semi-housing and housing management, which improved productivity and increased profit. Even though earlier studies found that yaks' nutritional requirements and metabolism differed from those of lowland ruminants, no feeding strategy has been developed to date to help fatten yaks. Consequently, yak feedlots have different feeding plans, and the results of yak fattening are not as positive as they are for cattle steers.
Regarding the quality of yak meat, the fat percentage is lower but the protein percentage is higher and with abundant vital amino acids, fatty acids, and minerals than commercial beef meat from the lowland region (95, 96). In the Tibetan region, the main source of protein in people's diet, ~50%, originates from yak meat (97, 98). However, there is still a significant discrepancy between the high demand for yak meat consumption and the inefficient output in the yak sector. The average daily gain and meat redness were upsurged when yaks received rumen-protected amino acid in their diets, while lightness, yellowness, drip loss, and tenderness were decreased, and these auspicious effects were revealed by the rumen-protected lysine and methionine supplementation in the yak diet. The supplementation of the rumen-protected amino acid in the diet of yaks increased the growth performance and meat quality, and for each fattening yak, the recommended doses of rumen-bypassed methionine and lysine should be given at rates of 15.0 and 5.0 g per day, respectively.
In meat quality, the fat and fatty acids profile plays a crucial role, and these parameters affect the appearance, texture, flavor, and hardness of the meat (99–101), as well as play a crucial part in preventing dark cutting, drip loss, and cold shortening (102). Optimum marbling in the meat is important in animal husbandry (103, 104). Demand, supply, and acceptability of unusual and exotic meats are rising globally along with the quickening pace of globalization and a shift in consumer philosophy (105, 106). Presently, the yak population is about 14 million worldwide. People living in the QTP region use the meat of yaks as a major source of animal protein (107, 108). Due to its lower fat content and higher protein content, devoid of anabolic steroids and pollutants, yak meat is gradually popular with consumers (109). The production of yak meat is more than 500 tons annually. The productions of carcass and dressing percentage of yak are 46.67–51.0 and 37.10–43.02%, respectively.
Yak meat, particularly the fatty acids in the meat, can be influenced by various factors, such as genetic variables (110), nutrition (111), gender (112), breeding environment (113), and etc. The metabolic responses are different in male and female animals (114), and their different hormones affect their fat composition (115).
In conclusion, domestication of yaks in the QTP plays an essential role to provide meat, milk, leather, fur, manure, etc. to local people and, due to these benefits, yaks are gaining importance worldwide. However, the high altitude, low temperature, low oxygen level, and harsh environment of the QTP subsequently affect the growth performance (growth-retarded yaks) and the survival of yaks. Yaks have a unique rumen ecology by hosting an essential gastrointestinal microbial community for pasture, fodders, and roughage degradation, yielding a high concentration of volatile fatty acids and microbial protein synthesis required for their maintenance and productivity. Information related to factors that influence the life of yaks and the microbial community demonstrated the novel rational avenues for designing strategies aimed at improving animal welfare and enhancing economic value. Furthermore, omics studies, particularly metabolomics and proteomics studies, should be carried out to provide additional information as to how these environmental factors influence yaks' survival and productive efficiency.
Conceptualization: AS and MW. Writing—original draft preparation: AS. Writing—review and editing: AS, IB, IQ, MM, and MW. Visualization and supervision: MW. All authors have read and agreed to the published version of the manuscript.
This research was funded by the Fundamental Fund (FF) (No. 66A103000057), Ministry of Higher Education, Science, Research, and Innovation (MHESI), and by Khon Kaen University, Thailand.
The authors declare that the research was conducted in the absence of any commercial or financial relationships that could be construed as a potential conflict of interest.
All claims expressed in this article are solely those of the authors and do not necessarily represent those of their affiliated organizations, or those of the publisher, the editors and the reviewers. Any product that may be evaluated in this article, or claim that may be made by its manufacturer, is not guaranteed or endorsed by the publisher.
1. Hu R, Zou H, Wang Z, Cao B, Peng Q, Jing X, et al. Nutritional interventions improved rumen functions and promoted compensatory growth of growth-retarded yaks as revealed by integrated transcripts and microbiome analyses. Front Microbiol. (2019) 10:318. doi: 10.3389/fmicb.2019.00318
2. Ma J, Zhu Y, Wang Z, Yu X, Hu R, Wang X, et al. Comparing the bacterial community in the gastrointestinal tracts between growth-retarded and normal Yaks on the Qinghai–Tibetan Plateau. Front Microbiol. (2020) 11:600516. doi: 10.3389/fmicb.2020.600516
3. Zhang Z, Xu D, Wang L, Hao J, Wang J, Zhou X, et al. Convergent evolution of rumen microbiomes in high-altitude mammals. Curr Biol. (2016) 26:1873–9. doi: 10.1016/j.cub.2016.05.012
4. Liu H, Li Z, Pei C, Degen A, Hao L, Cao X, et al. A comparison between yaks and Qaidam cattle in in vitro rumen fermentation, methane emission, and bacterial community composition with poor quality substrate. Anim Feed Sci Technol. (2022) 291:115395. doi: 10.1016/j.anifeedsci.2022.115395
5. Zi XD. Reproduction in female yaks (Bos grunniens) and opportunities for improvement. Theriogenology. (2003) 59:1303–12. doi: 10.1016/S0093-691X(02)01172-X
6. Rhode D, Madsen DB, Brantingham PJ, Dargye T. Yaks, yak dung, and prehistoric human habitation of the Tibetan Plateau. Dev Q Sci. (2007) 9:205–24. doi: 10.1016/S1571-0866(07)09013-6
7. Ren M, Song JK, Yang F, Zou M, Wang PX, Wang D, et al. First genotyping of Blastocystis in yaks from Qinghai Province, northwestern China. Parasit Vectors. (2019) 12:1–8. doi: 10.1186/s13071-019-3436-5
8. Ma J, Shah AM, Wang Z, Hu R, Zou H, Wang X, et al. Comparing the gastrointestinal barrier function between growth-retarded and normal yaks on the Qinghai-Tibetan Plateau. PeerJ. (2020) 8:e9851. doi: 10.7717/peerj.9851
9. Wu D, Vinitchaikul P, Deng M, Zhang G, Sun L, Wang H, et al. Exploration of the effects of altitude change on bacteria and fungi in the rumen of yak (Bos grunniens). Arch Microbiol. (2021) 203:835–46. doi: 10.1007/s00203-020-02072-x
10. Celi P, Verlhac V, Calvo EP, Schmeisser J, Kluenter AM. Biomarkers of gastrointestinal functionality in animal nutrition and health. Anim Feed Sci Technol. (2019) 250:9–31. doi: 10.1016/j.anifeedsci.2018.07.012
11. Yeoman CJ, White BA. Gastrointestinal tract microbiota and probiotics in production animals. Annu Rev Anim Biosci. (2014) 2:469–86. doi: 10.1146/annurev-animal-022513-114149
12. Parker A, Lawson MA, Vaux L, Pin C. Host-microbe interaction in the gastrointestinal tract. Environ Microbiol. (2018) 20:2337–53. doi: 10.1111/1462-2920.13926
13. Jacques G, Guedes JDA, Shuya Z. Yak Domestication. Ethnobiology Letters. (2021) 12:103–14. doi: 10.14237/ebl.12.1.2021.1755
14. Qiu Q, Wang L, Wang K, Yang Y, Ma T, Wang Z, et al. Yak whole-genome resequencing reveals domestication signatures and prehistoric population expansions. Nat Commun. (2015) 6:1–7. doi: 10.1038/ncomms10283
15. Joshi S, Shrestha L, Bisht N, Wu N, Ismail M, Dorji T, et al. Ethnic and cultural diversity amongst yak herding communities in the Asian highlands. Sustainability. (2020) 12:957. doi: 10.3390/su12030957
16. Tournadre N, Suzuki H. The Tibetic languages, an introduction to the family of languages derived from Old Tibetan. (2021). Paris: Lacito Publications.
17. Qi XB, Jianlin H, Blench R, Rege JEO. Understanding yak pastoralism in Central Asian Highlands: mitochondrial DNA evidence for origin, domestication and dispersal of domestic yak. In: Past Human Migrations in East Asia. (2008). England, UK: Routledge. p. 459–74.
18. Aldenderfer M, Eng JT. CHApTer 24 among two ancient high-altitude communities of Nepal. A companion to South Asia in the past. (2016) 16:374. doi: 10.1002/9781119055280.ch24
19. Shikui D, Ruijun L, Muyi K. Milking performance of China yak (Bos grunniens): A preliminary report. Afr J Agric Res. (2007) 2:052–7. doi: 10.5897/AJAR.9000491
20. Cui Z, Wu S, Li J, Yang QE, Chai S, Wang L, et al. Effect of alfalfa hay and starter feeding intervention on gastrointestinal microbial community, growth and immune performance of yak calves. Front Microbiol. (2020) 11:994. doi: 10.3389/fmicb.2020.00994
21. Jing X, Ding L, Zhou J, Huang X, Degen A, Long R, et al. The adaptive strategies of yaks to live in the Asian highlands. Animal Nutr. (2022) 9:249–58. doi: 10.1016/j.aninu.2022.02.002
22. Luming D, Ruijun L, Zhanhuan S, Changting W, Yuhai Y, Songhe X, et al. Feeding behaviour of yaks on spring, transitional, summer and winter pasture in the alpine region of the Qinghai–Tibetan plateau. Appl Anim Behav Sci. (2008) 111:373–90. doi: 10.1016/j.applanim.2007.06.008
23. Yang C, Millner JP, Sun Y, Yuan H, Liu Y, Zhang Y, et al. Supplementary feeding yak with oat hay improves nitrogen cycling in an alpine meadow on the Qinghai-Tibet Plateau, China. Agric Syst. (2021) 193:103216. doi: 10.1016/j.agsy.2021.103216
24. Long R, Ding L, Shang Z, Guo X. The yak grazing system on the Qinghai-Tibetan plateau and its status. Rangeland J. (2008) 30:241–6. doi: 10.1071/RJ08012
25. Chen Z, Ji Q, Dawa Y. Advances on research of yak production system in China. In: The international congress on yak, Chengdu, China. (2004). p. 47–56.
26. Singh S, Mishra A, Singh J, Rai S, Baig M, Biradar N, et al. Water requirement estimates of feed and fodder production for Indian livestock vis a vis livestock water productivity. Indian J Anim Sci. (2014) 84:1090–4.
27. Ma J, Shah A, Wang Z, Hu R, Zou H, Wang X, et al. Dietary supplementation with glutamine improves gastrointestinal barrier function and promotes compensatory growth of growth-retarded yaks. Animal. (2021) 15:100108. doi: 10.1016/j.animal.2020.100108
28. Long R, Dong S, Wei X, Pu X. The effect of supplementary feeds on the bodyweight of yaks in cold season. Livestock Prod Sci. (2005) 93:197–204. doi: 10.1016/j.livprodsci.2004.08.016
29. Zhao Z, Ma Z, Wang H, Zhang C. Effects of rumen-protected methionine and lysine supplementation on milk yields and components, rumen fermentation, and the rumen microbiome in lactating yaks (Bos grunniens). Anim Feed Sci Technol. (2021) 277:114972. doi: 10.1016/j.anifeedsci.2021.114972
30. Dai D, Pang K, Liu S, Wang X, Yang Y, Chai S, et al. Effects of concentrate supplementation on growth performance, rumen fermentation, and bacterial community composition in grazing Yaks during the warm season. Animals. (2022) 12:1398. doi: 10.3390/ani12111398
31. Zhu M, Xie R, Chen L, You M, Gou W, Chen C, et al. Milk production and quality of lactating yak fed oat silage prepared with a low-temperature-tolerant lactic acid bacteria inoculant. Foods. (2021) 10:2437. doi: 10.3390/foods10102437
32. Zhao Z, Ma Z, Wang H, Zhang C. Effects of trace minerals supply from rumen sustained release boluses on milk yields and components, rumen fermentation and the rumen bacteria in lactating yaks (Bos grunniens). Anim Feed Sci Technol. (2022) 283:115184. doi: 10.1016/j.anifeedsci.2021.115184
33. Wangmo S, Dorji P. Effect of Feeding Dolichousnea longissima (Ach.) Articus as Supplement during Winter on Milk Yield and its Composition in Yaks. Bhutan J Nat Resour Dev. (2017) 4, 7–7.
34. Dong Q, Zhao X, Ma Y, Xu S, Li Q. Live-weight gain, apparent digestibility, and economic benefits of yaks fed different diets during winter on the Tibetan plateau. Livest Sci. (2006) 101:199–207. doi: 10.1016/j.livprodsci.2005.11.009
35. Wei H, Ding L, Wang X, Yan Q, Jiang C, Hu C, et al. Astragalus root extract improved average daily gain, immunity, antioxidant status and ruminal microbiota of early weaned yak calves. J Sci Food Agric. (2021) 101:82–90. doi: 10.1002/jsfa.10617
36. Jiang C, Ding L, Dong Q, Wang X, Wei H, Hu C, et al. Effects of root extracts of three traditional Chinese herbs as dietary supplements on dry matter intake, average daily gain, rumen fermentation and ruminal microbiota in early weaned yak calves. Anim Feed Sci Technol. (2021) 278:115002. doi: 10.1016/j.anifeedsci.2021.115002
37. Liu H, Jiang H, Hao L, Cao X, Degen A, Zhou J, et al. Rumen bacterial community of grazing lactating yaks (Poephagus grunniens) supplemented with concentrate feed and/or rumen-protected lysine and methionine. Animals. (2021) 11:2425. doi: 10.3390/ani11082425
38. Ma J, Zhu Y, Wang Z, Yu X, Hu R, Wang X, et al. Glutamine supplementation affected the gut bacterial community and fermentation leading to improved nutrient digestibility in growth-retarded yaks. FEMS Microbiol Ecol. (2021) 97:fiab084. doi: 10.1093/femsec/fiab084
39. Hu R, Wang Z, Peng Q, Zou H, Wang H, Yu X, et al. Effects of GHRP-2 and cysteamine administration on growth performance, somatotropic axis hormone and muscle protein deposition in yaks (Bos grunniens) with growth retardation. PLoS ONE. (2016) 11:e0149461. doi: 10.1371/journal.pone.0149461
40. Zhang C, Li S, Yang L, Huang P, Li W, Wang S, et al. Structural modulation of gut microbiota in life-long calorie-restricted mice. Nat Commun. (2013) 4:1–10. doi: 10.1038/ncomms3163
41. Du R, Jiao S, Dai Y, An J, Lv J, Yan X, et al. Probiotic Bacillus amyloliquefaciens C-1 improves growth performance, stimulates GH/IGF-1, and regulates the gut microbiota of growth-retarded beef calves. Front Microbiol. (2018) 9:2006. doi: 10.3389/fmicb.2018.02006
42. Bang C, Dagan T, Deines P, Dubilier N, Duschl WJ, Fraune S, et al. Metaorganisms in extreme environments: do microbes play a role in organismal adaptation? Zoology. (2018) 127:1–19. doi: 10.1016/j.zool.2018.02.004
43. Guo W, Zhou M, Ma T, Bi S, Wang W, Zhang Y, et al. Survey of rumen microbiota of domestic grazing yak during different growth stages revealed novel maturation patterns of four key microbial groups and their dynamic interactions. Animal Microb. (2020) 2:1–20. doi: 10.1186/s42523-020-00042-8
44. Su Y, Su J, Li F, Tian X, Liu Z, Ding G, et al. Yak Gut Microbiota: A Systematic Review and Meta-Analysis. Front Vet Sci. (2022) 9:889594. doi: 10.3389/fvets.2022.889594
45. Zhang L, Jiang X, Li A, Waqas M, Gao X, Li K, et al. Characterization of the microbial community structure in intestinal segments of yak (Bos grunniens). Anaerobe. (2020) 61:102115. doi: 10.1016/j.anaerobe.2019.102115
46. Zou H, Hu R, Wang Z, Shah AM, Zeng S, Peng Q, et al. Effects of nutritional deprivation and re-alimentation on the feed efficiency, blood biochemistry, and rumen microflora in yaks (Bos grunniens). Animals. (2019) 9:807. doi: 10.3390/ani9100807
47. Ransom-Jones E, Jones DL, Mccarthy AJ, Mcdonald JE. The Fibrobacteres: an important phylum of cellulose-degrading bacteria. Microb Ecol. (2012) 63:267–81. doi: 10.1007/s00248-011-9998-1
48. Li L, Su Y, Li F, Wang Y, Ma Z, Li Z, et al. The effects of daily fasting hours on shaping gut microbiota in mice. BMC Microbiol. (2020) 20:1–8. doi: 10.1186/s12866-020-01754-2
49. Xue D, Chen H, Luo X, Guan J, He Y, Zhao X, et al. Microbial diversity in the rumen, reticulum, omasum, and abomasum of yak on a rapid fattening regime in an agro-pastoral transition zone. J Microbiol. (2018) 56:734–43. doi: 10.1007/s12275-018-8133-0
50. Cortés A, Rooney J, Bartley DJ, Nisbet AJ, Cantacessi C. Helminths, hosts, and their microbiota: new avenues for managing gastrointestinal helminthiases in ruminants. Expert Rev Anti Infect Ther. (2020) 18:977–85. doi: 10.1080/14787210.2020.1782188
51. Dimmitt RA, Staley EM, Chuang G, Tanner SC, Soltau TD, Lorenz RG, et al. The role of postnatal acquisition of the intestinal microbiome in the early development of immune function. J Pediatr Gastroenterol Nutr. (2010) 51:262. doi: 10.1097/MPG.0b013e3181e1a114
52. Zhang YJ Li S, Gan RY, Zhou T, Xu DP Li HB, et al. Impacts of gut bacteria on human health and diseases. Int J Mol Sci. (2015) 16:7493–519. doi: 10.3390/ijms16047493
53. Isaacson R, Kim HB. The intestinal microbiome of the pig. Animal Health Res Rev. (2012) 13:100–9. doi: 10.1017/S1466252312000084
54. Guo W, Li Y, Wang L, Wang J, Xu Q, Yan T, et al. Evaluation of composition and individual variability of rumen microbiota in yaks by 16S rRNA high-throughput sequencing technology. Anaerobe. (2015) 34:74–9. doi: 10.1016/j.anaerobe.2015.04.010
55. Malmuthuge N, Griebel PJ, Guan LL. The gut microbiome and its potential role in the development and function of newborn calf gastrointestinal tract. Front Vet Sci. (2015) 2:36. doi: 10.3389/fvets.2015.00036
56. Xiao J, Alugongo G, Chung R, Dong S, Li S, Yoon I, et al. Effects of Saccharomyces cerevisiae fermentation products on dairy calves: Ruminal fermentation, gastrointestinal morphology, and microbial community. J Dairy Sci. (2016) 99:5401–12. doi: 10.3168/jds.2015-10563
57. Xue D, Chen H, Chen F, He Y, Zhao C, Zhu D, et al. Analysis of the rumen bacteria and methanogenic archaea of yak (Bos grunniens) steers grazing on the Qinghai-Tibetan Plateau. Livest Sci. (2016) 188:61–71. doi: 10.1016/j.livsci.2016.04.009
58. Huang XD, Tan HY, Long R, Liang JB, Wright ADG. Comparison of methanogen diversity of yak (Bos grunniens) and cattle (Bos taurus) from the Qinghai-Tibetan plateau, China. BMC Microbiol. (2012) 12:1–10. doi: 10.1186/1471-2180-12-237
59. Harfoot C, Hazlewood G, Hobson P, Stewart C. The rumen microbial ecosystem. eds. Hobson PN and Stewart CS (1997). London: Chapman and Hall, 382–426.
60. Jarvis GN, Strömpl C, Burgess DM, Skillman LC, Moore ER, Joblin KN, et al. Isolation and identification of ruminal methanogens from grazing cattle. Curr Microbiol. (2000) 40:327–32. doi: 10.1007/s002849910065
61. Chai J, Diao Q, Wang H, Tu Y, Tao X, Zhang N, et al. Effects of weaning age on growth, nutrient digestibility and metabolism, and serum parameters in Hu lambs. Anim Nutr. (2015) 1:344–8. doi: 10.1016/j.aninu.2015.11.007
62. Mellado M, Rodríguez IJ, Alvarado-Espino A, Véliz FG, Mellado J, García JE, et al. Reproductive response to concentrate supplementation of mixed-breed goats on rangeland. Trop Anim Health Prod. (2020) 52:2737–41. doi: 10.1007/s11250-020-02264-z
63. Stanley C, Williams C, Jenny B, Fernandez J, Bateman Ii H, Nipper W, et al. Effects of feeding milk replacer once versus twice daily on glucose metabolism in Holstein and Jersey calves. J Dairy Sci. (2002) 85:2335–43. doi: 10.3168/jds.S0022-0302(02)74313-0
64. Jin Y, Zhou Y. Effects of concentrate level and chromium-methionine supplementation on the performance, nutrient digestibility, rumen fermentation, blood metabolites, and meat quality of Tan lambs. Anim Biosci. (2022) 35:677. doi: 10.5713/ab.20.0802
65. Chen G, Song S, Wang B, Zhang Z, Peng Z, Guo C, et al. Effects of forage: concentrate ratio on growth performance, ruminal fermentation and blood metabolites in housing-feeding yaks. Asian-Australas J Anim Sci. (2015) 28:1736. doi: 10.5713/ajas.15.0419
66. Guan J, Long K, Ma J, Zhang J, He D, Jin L, et al. Comparative analysis of the microRNA transcriptome between yak and cattle provides insight into high-altitude adaptation. PeerJ. (2017) 5:e3959. doi: 10.7717/peerj.3959
67. Yang C, Zhang J, Ahmad AA, Bao P, Guo X, Long R, et al. Dietary energy levels affect growth performance through growth hormone and insulin-like growth factor 1 in yak (Bos grunniens). Animals. (2019) 9:39. doi: 10.3390/ani9020039
68. Zhou J, Yue S, Peng Q, Wang L, Wang Z, Xue B, et al. Metabonomic responses of grazing yak to different concentrate supplementations in cold season. Animals. (2020) 10:1595. doi: 10.3390/ani10091595
69. Gao J, Yang D, Sun Z, Niu J, Bao Y, Liu S, et al. Changes in blood metabolic profiles reveal the dietary deficiencies of specific nutrients and physiological status of grazing Yaks during the cold season in Qinghai province of China. Metabolites. (2022) 12:738. doi: 10.3390/metabo12080738
70. Shoudong Y. A study on the improvement of yak reproductive performance by introducing wild yak blood. Yak production in central Asian highlands. (2002) 2002:324.
71. Fan J, Yu Y, Han X, He H, Luo Y, Yu S, et al. The expression of hypoxia-inducible factor-1 alpha in primary reproductive organs of the female yak (Bos grunniens) at different reproductive stages. Reprod Dom Anim. (2020) 55:1371–82. doi: 10.1111/rda.13783
72. Zhang R. Interspecies Hybridization between Yak, Bos taurus and Bos indicus and Reproduction of the Hybrids. (2000). Gaithersburg, MD: iVis.
73. Yu S, Huang Y, Chen B. Reproductive patterns of the yak. I Reproductive phenomena of the female yak. Br Vet J. (1993) 149:579–83. doi: 10.1016/S0007-1935(05)80042-9
74. Shikui D, Ruijun L, Muyi K, Xiaogeng P, Yanjun G. Effect of urea multinutritional molasses block supplementation on liveweight change of yak calves and productive and reproductive performances of yak cows. Can J Anim Sci. (2003) 83:141–5. doi: 10.4141/A01-097
75. Zhang R. Effects of environment and management on yak reproduction. Recent advances in yak reproduction. (2000). Ithaca, New York: International Veterinary Information Service.
76. Hecht D. Joel Berger: Review of Extreme Conservation: Life at the Edges of the World. Hum Ecol. (2022) 17:1–2. doi: 10.1007/s10745-022-00349-x
77. Zhang R, Guo X, Liang C, Pei J, Bao P, Yin M, et al. Identification and validation of Yak (Bos grunniens) frozen–thawed sperm proteins associated with capacitation and the acrosome reaction. J Proteome Res. (2022). doi: 10.1021/acs.jproteome.2c00528
78. Baldan T, Myagmardulam P, Jambatsadmid D, Khosbayar B. Research results on the use of frozen Yak sperm. Grand Altai Res Educ. (2022) 58–66. doi: 10.25712/ASTU.2410-485X.2022.01.008
79. Zhou J, Zhang J, Xue B, Yue S, Yang C, Xue B, et al. Effects of premating calcium and phosphorus supplementation on reproduction efficiency of grazing Yak heifers. Animals. (2021) 11:554. doi: 10.3390/ani11020554
80. Fan Q, Wanapat M, Hou F. Chemical composition of milk and rumen microbiome diversity of yak, impacting by herbage grown at different phenological periods on the Qinghai-Tibet Plateau. Animals. (2020) 10:1030. doi: 10.3390/ani10061030
81. Wang T, Guo Z, Liu Z, Feng Q, Wang X, Tian Q, et al. The aggregation behavior and interactions of yak milk protein under thermal treatment. J Dairy Sci. (2016) 99:6137–43. doi: 10.3168/jds.2016-11063
82. Sun W, Luo Y, Wang DH, Kothapalli KS, Brenna JT. Branched chain fatty acid composition of yak milk and manure during full-lactation and half-lactation. Prostaglandins Leukotrienes Essential Fatty Acids. (2019) 150:16–20. doi: 10.1016/j.plefa.2019.09.002
83. Shi B, Jiang Y, Chen Y, Zhao Z, Zhou H, Luo Y, et al. Variation in the fatty acid synthase gene (FASN) and its association with milk traits in Gannan yaks. Animals. (2019) 9:613. doi: 10.3390/ani9090613
84. Li H, Ma Y, Li Q, Wang J, Cheng J, Xue J, et al. The chemical composition and nitrogen distribution of Chinese yak (Maiwa) milk. Int J Mol Sci. (2011) 12:4885–95. doi: 10.3390/ijms12084885
85. Fox PF, Mcsweeney PL, Paul L. Dairy Chemistry and Biochemistry. (1998). Berlin, Germany: Springer.
86. Fox P. Milk proteins: general and historical aspects. In: Advanced Dairy Chemistry–1 Proteins. (2003). Berlin, Germany: Springer. p. 1–48.
87. Cui G, Yuan F, Degen A, Liu S, Zhou J, Shang Z, et al. Composition of the milk of yaks raised at different altitudes on the Qinghai–Tibetan Plateau. Int Dairy J. (2016) 59:29–35. doi: 10.1016/j.idairyj.2016.02.046
88. Fan Q, Wanapat M, Hou F. Mineral nutritional status of yaks (Bos grunniens) grazing on the Qinghai-Tibetan plateau. Animals. (2019) 9:468. doi: 10.3390/ani9070468
89. Ji Q. Analysis on the milk output and milk quality of three fine kinds of yaks of Tibet. China Herbivore. (2000) 5:3–6.
90. Sheng Q, Li J, Alam MS, Fang X, Guo M. Gross composition and nutrient profiles of Chinese yak (Maiwa) milk. Int J Food Sci Technol. (2008) 43:568–72. doi: 10.1111/j.1365-2621.2006.01463.x
91. Zhang J, Yang M, Cai D, Hao Y, Zhao X, Zhu Y, et al. Composition, coagulation characteristics, and cheese making capacity of yak milk. J Dairy Sci. (2020) 103:1276–88. doi: 10.3168/jds.2019-17231
92. Agyare AN, Liang Q. Nutrition of yak milk fat–Focusing on milk fat globule membrane and fatty acids. J Funct Foods. (2021) 83:104404. doi: 10.1016/j.jff.2021.104404
93. Bao G, Niu J, Li S, Zhang L, Luo Y. Effects of ultrasound pretreatment on the quality, nutrients and volatile compounds of dry-cured yak meat. Ultrason Sonochem. (2022) 82:105864. doi: 10.1016/j.ultsonch.2021.105864
94. Zhou J, Zhong C, Liu H, Degen A, Titgemeyer E, Ding L, et al. Comparison of nitrogen utilization and urea kinetics between yaks (Bos grunniens) and indigenous cattle (Bos taurus). J Anim Sci. (2017) 95:4600–12. doi: 10.2527/jas2017.1428
95. Yin R, Bai W, Wang J, Wu C, Dou Q, Yin R, et al. Development of an assay for rapid identification of meat from yak and cattle using polymerase chain reaction technique. Meat Sci. (2009) 83:38–44. doi: 10.1016/j.meatsci.2009.03.008
96. Wan H, Luo L, Wu J. Research advances in yak meat quality characteristics. J Anim Sci Vet Med. (2012) 31:36–40.
97. Honikel KO. Reference methods for the assessment of physical characteristics of meat. Meat Sci. (1998) 49:447–57. doi: 10.1016/S0309-1740(98)00034-5
98. Wu J, Ma B, Ren H, Zhang L, Xiang Y, Brown M, et al. Characterization of metallothioneins (MT-I and MT-II) in the yak. J Anim Sci. (2007) 85:1357–62. doi: 10.2527/jas.2006-291
99. Gagaoua M, Bonnet M, Picard B. Protein array-based approach to evaluate biomarkers of beef tenderness and marbling in cows: Understanding of the underlying mechanisms and prediction. Foods. (2020) 9:1180. doi: 10.3390/foods9091180
100. Wen Y, Liu H, Liu K, Cao H, Mao H, Dong X, et al. Analysis of the physical meat quality in partridge (Alectoris chukar) and its relationship with intramuscular fat. Poult Sci. (2020) 99:1225–31. doi: 10.1016/j.psj.2019.09.009
101. Xiong L, Pei J, Chu M, Wu X, Kalwar Q, Yan P, et al. Fat deposition in the muscle of female and male yak and the correlation of yak meat quality with fat. Animals. (2021) 11:2142. doi: 10.3390/ani11072142
102. Douny C, El Khoury R, Delmelle J, Brose F, Degand G, Moula N, et al. Effect of storage and cooking on the fatty acid profile of omega-3 enriched eggs and pork meat marketed in Belgium. Food Sci Nutr. (2015) 3:140–52. doi: 10.1002/fsn3.197
103. Guo Q, Kong X, Hu C, Zhou B, Wang C, Shen QW, et al. Fatty acid content, flavor compounds, and sensory quality of pork loin as affected by dietary supplementation with L-arginine and glutamic acid. J Food Sci. (2019) 84:3445–53. doi: 10.1111/1750-3841.14959
104. Liu S, Huang J, Wang X, Ma Y. Transcription factors regulate adipocyte differentiation in beef cattle. Anim Genet. (2020) 51:351–7. doi: 10.1111/age.12931
105. Cawthorn DM, Hoffman LC. Controversial cuisine: A global account of the demand, supply and acceptance of “unconventional” and “exotic” meats. Meat Sci. (2016) 120:19–36. doi: 10.1016/j.meatsci.2016.04.017
106. Popoola IO, Soladoye PO, Gaudette NJ, Wismer WV. A Review of sensory and consumer-related factors influencing the acceptance of red meats from alternative animal species. Food Rev Int. (2021) 2021:1–20. doi: 10.1080/87559129.2020.1860084
107. Guo X, Long R, Kreuzer M, Ding L, Shang Z, Zhang Y, et al. Importance of functional ingredients in yak milk-derived food on health of Tibetan nomads living under high-altitude stress: a review. Crit Rev Food Sci Nutr. (2014) 54:292–302. doi: 10.1080/10408398.2011.584134
108. Wen W, Luo X, Xia B, Guan J, Nie Y, Li L, et al. Post-mortem oxidative stability of three yak (Bos grunniens) muscles as influenced by animal age. Meat Sci. (2015) 105:121–5. doi: 10.1016/j.meatsci.2015.03.014
109. Wang LL, Han L, Ma XL, Yu QL, Zhao SN. Effect of mitochondrial apoptotic activation through the mitochondrial membrane permeability transition pore on yak meat tenderness during postmortem aging. Food Chem. (2017) 234:323–31. doi: 10.1016/j.foodchem.2017.04.185
110. Brzozowska AM, Lukaszewicz M, Oprzadek JM. Energy-protein supplementation and lactation affect fatty acid profile of liver and adipose tissue of dairy cows. Molecules. (2018) 23:618. doi: 10.3390/molecules23030618
111. Wang H, He Y, Li H, Wu F, Qiu Q, Niu W, et al. Rumen fermentation, intramuscular fat fatty acid profiles and related rumen bacterial populations of Holstein bulls fed diets with different energy levels. Appl Microbiol Biotechnol. (2019) 103:4931–42. doi: 10.1007/s00253-019-09839-3
112. Stergiadis S, Berlitz CB, Hunt B, Garg S, Givens DI, Kliem KE, et al. An update to the fatty acid profiles of bovine retail milk in the United Kingdom: Implications for nutrition in different age and gender groups. Food Chem. (2019) 276:218–30. doi: 10.1016/j.foodchem.2018.09.165
113. Bergamaschi M, Cipolat-Gotet C, Cecchinato A, Schiavon S, Bittante G. Chemometric authentication of farming systems of origin of food (milk and ripened cheese) using infrared spectra, fatty acid profiles, flavor fingerprints, and sensory descriptions. Food Chem. (2020) 305:125480. doi: 10.1016/j.foodchem.2019.125480
114. Aaslyng MD, Jensen H, Karlsson AH. The gender background of texture attributes of pork loin. Meat Sci. (2018) 136:79–84. doi: 10.1016/j.meatsci.2017.10.018
Keywords: yaks (Bos grunniens), environmental stress, growth retardation, climate change, domestication, feeding, microbiota, breeding
Citation: Shah AM, Bano I, Qazi IH, Matra M and Wanapat M (2023) “The Yak”—A remarkable animal living in a harsh environment: An overview of its feeding, growth, production performance, and contribution to food security. Front. Vet. Sci. 10:1086985. doi: 10.3389/fvets.2023.1086985
Received: 01 November 2022; Accepted: 05 January 2023;
Published: 02 February 2023.
Edited by:
Nesrein M. Hashem, Alexandria University, EgyptReviewed by:
Faizul Hassan, University of Agriculture, Faisalabad, PakistanCopyright © 2023 Shah, Bano, Qazi, Matra and Wanapat. This is an open-access article distributed under the terms of the Creative Commons Attribution License (CC BY). The use, distribution or reproduction in other forums is permitted, provided the original author(s) and the copyright owner(s) are credited and that the original publication in this journal is cited, in accordance with accepted academic practice. No use, distribution or reproduction is permitted which does not comply with these terms.
*Correspondence: Metha Wanapat, bWV0aGFAa2t1LmFjLnRo
Disclaimer: All claims expressed in this article are solely those of the authors and do not necessarily represent those of their affiliated organizations, or those of the publisher, the editors and the reviewers. Any product that may be evaluated in this article or claim that may be made by its manufacturer is not guaranteed or endorsed by the publisher.
Research integrity at Frontiers
Learn more about the work of our research integrity team to safeguard the quality of each article we publish.