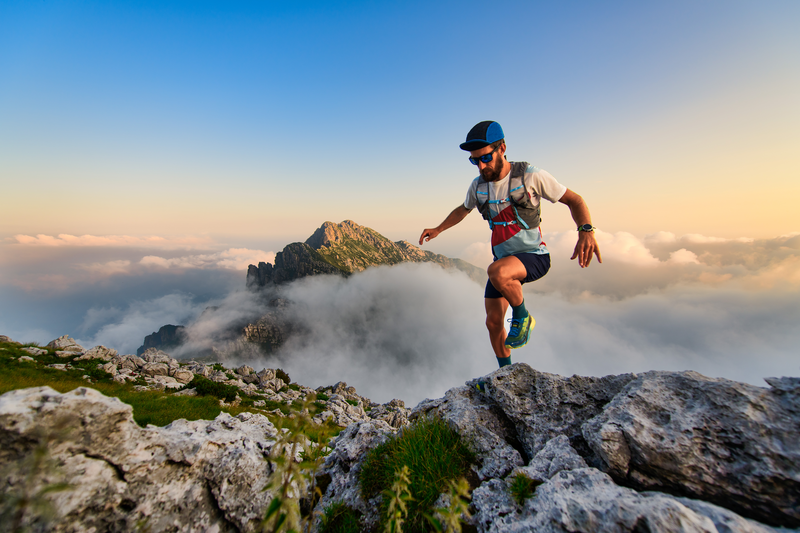
95% of researchers rate our articles as excellent or good
Learn more about the work of our research integrity team to safeguard the quality of each article we publish.
Find out more
ORIGINAL RESEARCH article
Front. Vet. Sci. , 27 September 2022
Sec. Parasitology
Volume 9 - 2022 | https://doi.org/10.3389/fvets.2022.996422
This article is part of the Research Topic Alternatives for the Control of Parasites to Promote Sustainable Livestock View all 9 articles
The poultry red mite Dermanyssus gallinae is an economically important pest in poultry farms worldwide, but an effective treatment option is lacking. The current study determined the effectiveness of six Chinese herbal medicines [Syzygium aromaticum (clove), Hibiscus syriacus (Hibiscus), Illicium verum (star anise), Leonurus artemisia (motherwort), Cinnamomum cassia (cinnamon), and Taraxacum sp. (dandelion)] against D. gallinae. Alcohol extracts were prepared via the solvent extraction method and the phenol, flavonoid, and tannin contents were determined. These active components were highest in S. aromaticum and lowest in H. syriacus, I. verum. No tannin content was detected in L. artemisia. All extracts showed contact toxicity against D. gallinae at a test concentration of 1 g/mL, with S. aromaticum and L. artemisia resulting in 100% mortality. S. aromaticum, L. artemisia, and I. verum showed the best efficacy (LC50 0.159, 0.200, and 0.292 g/mL, respectively). Different combinations of extracts showed an additive effect of I. verum LC90 + L. artemisia LC90. The acaricidal efficacy of this combination was tested against different developmental stages of D. gallinae, being most efficacious against nymphal and larval D. gallinae, with a corrected mortality rate of 100%. However, inhibition of egg hatching was only 53.69%. Taken together, these results highlight I. verum LC90 + L. artemisia LC90 as a promising compound with severe contact toxicity against D. gallinae. Given the wide cultivation of these species and their extensive use in foodstuffs and cosmetics as flavors and fragrances, they could be a cheap, readily available ecofriendly alternative to pesticides currently used in poultry farms.
The poultry red mite (PRM), Dermanyssus gallinae (De Geer, 1778) (Acari, Dermanyssidae), is a common ectoparasitoid that feeds on poultry and human blood, and is highly prevalent in poultry farms worldwide, causing annual losses of millions of Euros (1). PRM are tiny, hiding in cracks in the walls of poultry houses and chicken coops during the day, and sucking blood from the host at night. PRM infection can lead to restlessness, pecking of feathers, skin inflammation, anemia, and slow growth, and can also affect egg production and egg quality (2, 3). PRM infection outbreaks can not only cause serious harm to the poultry industry, but also pose a threat to human health. In recent years, there has been a series of reports of infections in humans caused by PRM bites (4, 5), such as rash, dermatitis, urticaria, and other skin diseases. In addition, PRM have been reported to bite cats and dogs (6, 7). Moreover, PRM are also a transmitter of more than 20 species of pathogen (8–10), including Escherichia coli, Streptomyces spp., Staphylococcus spp., Yersinia, Listeria, and Pasteurella spp., as well as eastern equine encephalitis virus, Venezuelan equine encephalitis virus, and alphavirus (11, 12). At present, the prevention and control of PRM mainly rely on chemical drugs. However, the long-term use of chemical drugs, such as organophosphorus and ivermectin, not only leads to PRM resistance, but also has a significant impact on the environment and egg production (13–16). Therefore, there is a need to develop new compounds that are not harmful to humans or the environment, that do not negatively impact the quality of eggs and have good acaricidal effects. The use of Chinese herbal medicines is becoming more popular in the poultry industry because of their natural safety, little if any development of drug resistance, and residue characteristics. Chinese herbal medicines have antibacterial and antiviral effects, as well as enhancing immunity and other functions, and have been developed and applied in the prevention and treatment of modern animal parasitic diseases (17). Although botanical pesticide research has mainly focused on mosquito and ticks (18), studies on other arthropods of medical and veterinary importance are still in the preliminary phase. Several botanical pesticides are currently used in arthropod pest management, such as products based on the neem tree (Azadirachta indica). Neem extracts are reported to have toxic effects against 200 species of arthropod pests (19). Herbs can produce a broad range of secondary metabolites, such as terpenoids, polyacetylenes, sugars, flavonoids, and alkaloids, which can act as antifeedants and repellents. In addition, they can also suppress acetylcholinesterase activity, negatively impacting the nervous system (20–23). They can also act on other targets in the nervous system, such as nicotinic acetylcholine receptors (nAChR), octopamine receptors, tyramine receptors, sodium channels, and γ-aminobutyric acid (GABA)-gated chloride channels (24, 25).
Thus, the current study evaluated the toxic effects of Chinese herbal medicines on D. gallinae, namely clove, Hibiscus, star anise, motherwort, cinnamon, and dandelion, which were selected based on previous studies (26–28). The toxicity of these Chinese herbal medicines was evaluated through contact assays on adult mites with compound synergy assays against different stages. The herbs with best acaricidal activity were screened out through contact assays, and the compound synergistic effect of the selected herbal medicine was tested. Finally, the best herbal was screened out according to the acaricidal effect of different developmental stages.
The experimental plants [clove (flower bud), cinnamon (bark), hibiscus (flowers), dandelion (whole plant), and star anise (ripe fruit), motherwort (whole plant)] were all purchased from Tongrentang Chinese Herbal Medicine Wholesale Store (Beijing, China). A positive control (100 mg/mL ivermectin) was purchased from Henan Anjin Biotechnology (Zhengzhou, Henan, China). Anhydrous ethanol (analytical grade) was purchased from Fuyu Fine Chemical (Tianjin, China). A negative control (0.9% sodium chloride) was purchased from Henan Kelun Pharmaceutical (Anyang, Henan, China). Blank control distilled water was produced by the Parasitology Laboratory, Henan Agricultural University.
For each herb (see above), 50 g of the dried tissues was crushed and passed through 30-mesh screens. Next, 200 mL of a 90% ethanol solution was added; the samples were soaked for 1 week and then filtered through six layers of gauze. The residue was then added to 100 mL of a 90% ethanol solution and soaked again for 24 h. This solution was filtered through six layers of gauze, and the two filtrates were then combined. The filtrate was centrifuged at 3,000 rpm for 10 min, and the ethanol was evaporated from the supernatant in a boiling water bath to concentrate it into a paste. This paste was diluted to 50 mL with 0.9% sodium chloride; thus, 50 g of each herb was used to make 50 mL of experimental insecticide. Each insecticide was stored at 4°C for later use.
The total phenolic compound content of the six herbal alcohol extracts was measured using the Folin-Ciocalteu method (29). First, a standard solution of gallic acid (0, 0.05, 0.1, 0.15, 0.2, 0.25, and 0.3 mg/mL) was prepared. Then, 1 mL of each extract, 3 mL of distilled water, 1 mL of Folin-Ciocalteu phenol reagent and 4 mL of 7.5% sodium carbonate solution were combined and diluted to 10 mL with distilled water in a volumetric flask (10 mL). The mixture was shaken well and allowed to stand for 1.5 min at 30°C in darkness. Absorbance for the test and standard solutions was read at 760 nm using distilled water for zero adjustment. All samples were replicated six times. Gallic acid solutions (0–0.3 mg/mL) were used to generate a standard curve (r2 = 0.9998). The gallic acid content was presented in terms of mg/mL of gallic acid in the sample.
The Ruslin method was used to determine the total flavonoid content (30). First, a standard solution of rutin (0, 0.2, 0.4, 0.6, 0.8, 1.0, and 2.0 mg/mL) was prepared. To each 0.1 mL of herbal alcohol extract, 0.3 mL sodium nitrite solution with a concentration of 5% was added; the mix was shaken well and left to stand for 6 min. Then, 0.3 mL aluminum nitrate solution with a concentration of 10% was added and the mix was again shaken well and left to react for 15 min away from light. Next, 4 mL 4% sodium hydroxide solution was added, followed by a constant volume of 80% ethanol solution to the scale line. After 15 min of mixing and incubation at room temperature, the absorbance of the reaction mixtures was determined at 510 nm against a blank. Rutin solutions (0–2 mg/mL) were used to generate a standard curve (r2 = 0.9995). The total flavonoid assay was repeated six times for each extract.
Use Tannin Acid Content Assay kit, Micromethod (Sangon Biotech, Shanghai, China) instructions for determination. All samples were carried out in 6 copies. Tannin content solutions (0–10 mg/mL) were used to generate a standard curve (r2 = 0.9994). The tannin content was presented in terms of mg/mL of tannic acid in each sample.
On June 6, 2021 a suspected case of chicken mite infestation occurred at an egg farm in Tongxu County, Kaifeng City, Henan Province, China, with dense, fast-moving mites appearing in the chicken coop and surrounding cracks in the wall. A small brush was used to collect mites from the structure of the chicken coops, feed and water troughs, chickens body surface, and so on, and there were transferred into a sealed bag, which was taken to the Parasitology Laboratory of Henan Agricultural University. All experiments were approved by the Animal Welfare and Research Ethics Committee of the College of Animal Medicine, Henan Agricultural University (Permit No: HNND2021060601). Under a stereomicroscope, using the identification method of Di Palma (31), the morphology of the mouth organs, horns, and vent region of the mites was examined, and the molecular method of Chu was used for further identification (32). The final identification confirmed the presence of D. gallinae. According to the sequence comparative analysis of homology, the samples collected showed 100% homology with the D. gallinae LC034951.1 gene sequence from Japan. The collected mites were then stored in a 4°C refrigerator until use.
For each Chinese herbal medicine extract, the bottom of a 60 × 15 mm petri dish was lined with filter paper and 1 g/mL of extract was evenly added to the filter paper. The dish was then left for 24 h to allow for volatilization of the extract to form a drug film. Ten similar-sized D. gallinae of good physiological status were selected with a small brush, and placed each petri dish and left for 1 h. The mites were then transferred to a clean petri dish without a drug film. There were five replicates per extract per set of experiments. A treatment group containing no agent was set as a blank control, whereas the negative control dish contained 0.9% NaCl, and positive control contained 100 mg/mL ivermectin.
After exposure to the Chinese herbal medicine extract for 1 h, the mites were incubated in a constant temperature (25–30°C) and humidity (relative humidity 60–80%) incubator in a light: dark 12:12 h photoperiod (33). Their mortality was observed and recorded 48 h later. If a mite remained immobile after being continuously stimulated with a needle for 1 min, it was considered to be dead.
The adopted a method to determine whether the mixed use of two plant sources has synergistic effects using Equation (1):
The synergistic virulence index (Equation 2) was used to evaluate the combined effect of two agents:
where c·f > 20 indicated a synergistic effect, c·f < −20 indicated antagonistic effects, and −20 <c·f < 20 indicated additive effects. Each pair of Chinese herbal medicine extracts was analyzed using both approaches.
The experimental data were sorted with Excel, and the percentage mortality of each test group was calculated using Equation (3):
The mortality in each treatment group was corrected to take into account control mortality using Abbott's formula (Equation 4) (34):
If the mortality rate was <5%, there was no adjustment for mortality.
The egg-hatching rate was calculated using Equation (5):
Data were expressed as the mean ± standard deviation. SPSS v.20.0 was used to analyze the date using one-way ANOVA. P < 0.05 indicated statistically significant differences between the treatment and control groups. Coefficient of determination (r2) and regression equations were calculated by linear regression. The median lethal concentration (LC50) and 95% confidence interval (95% CI) were calculated using the Probit algorithm. Prism v.8.0 software was used to draw the figures (GraphPad, San Diego, CA, USA).
Table 1 summarizes the results from the quantitative determination of the tannin and flavonoid content of each plant extract and their respective total phenol content. Clove contained the highest levels overall (70.78 mg/mL), and of total phenols (66.50 mg/mL), flavonoids (3.97 mg/mL), and tannins (0.31 mg/mL), followed by star anise (64.88 mg/mL), total phenols (64.00 mg/mL), flavonoids (0.76 mg/mL), tannins (0.12 mg/mL); cinnamon (45.45 mg/mL), total phenols (45.00 mg/mL), flavonoids (0.41 mg/mL), and tannins (0.04 mg/mL); dandelion (21.58 mg/mL), total phenols (21.50 mg/mL), flavonoids (0.04 mg/mL) and tannins (0.04 mg/mL). Hibiscus contained the lowest levels overall (7.31 mg/mL), with low total phenols (7.00 mg/mL), flavonoids (0.24 mg/mL), and tannins (0.07 mg/mL), whereas motherwort was slightly higher overall (16.90 mg/mL), including total phenols (16.70 mg/mL) and flavonoids (0.20 mg/mL), and but contained no tannins detectable by the methods used.
At a concentration of 1 mg/mL, the tested herbs showed different degrees of toxicity against adult D. gallinae (Table 2), with clove and motherwort resulting in 100% adjusted mortality, followed by hibiscus (98.82%), star anise (96.00%), and dandelion (90.00%). By contrast, cinnamon showed weak toxicity with an adjusted mortality of 60.00%. In terms of LC50 and LC90, star anise LC50 (0.159 g/mL) and motherwort LC50 (0.200 g/mL) had the strongest effects. By contrast, the LC50 of clove, hibiscus, and dandelion were 0.292 g/mL, 0.388 g/mL, and 0.410 g/mL, respectively. The strongest LC90 effect was seen with clove (0.521 g/mL) and motherwort (0.622 g/mL), compared with star anise (0.886 g/mL), hibiscus (1.119 g/mL), and dandelion (1.290 g/mL). The LC50 and LC90 of cinnamon were 0.812 mg/mL and 3.163 mg/mL, respectively (Table 3 and Figure 1). Thus, given these levels of biological activity and resource costs, star anise, clove, and motherwort were selected for further study.
Figure 1. Contact toxicity of alcohol extracts of Chinese herbal medicines against adult Dermanyssus gallinae.
The synergistic virulence index against D. gallinae adults of the six combinations of herbal extracts showed that star anise LC50 + motherwort LC50, star anise LC50 + motherwort LC90, and clove LC50 + motherwort LC90 had antagonistic effects, whereas star anise LC90 + motherwort LC50, clove LC90 + star anise LC90, and star anise LC90 + motherwort LC90 had additive effects. The mortality rate of D. gallinae adults treated with star anise LC90 + motherwort LC90 was 96.55%, whereas, with clove LC90 + star anise LC90, it was 87.64% (Table 4). Thus, based on the actual mortality rate, star anise LC90 + motherwort LC90 was selected for further study.
Table 4. Synergetic toxicity index (c·f value) of herbal alcohol extracts from Chinese herbal medicines against adult Dermanyssus gallinaeA.
Star anise LC90 + motherwort LC90 was most effective against Dermanyssus gallinae nymphs and larvae, with a mortality rate of 100.00% (Table 5 and Figure 2). By contrast, the corrected mortality of adult Dermanyssus gallinae in response to star anise LC90 + motherwort LC90 was 78.16%, and the rate of egg-hatching inhibition was 53.69%.
Table 5. Contact toxicity of compound herbal medicines against developmental stages of Dermanyssus gallinae.
Figure 2. Contact toxicity after 48 h of treatment with star anise LC90 + motherwort LC90 against different development stages of Dermanyssus gallinae.
Analysis of the composition of the six Chinese herbal medicine extracts showed total phenolics to be the main components, with flavonoids and tannins being present at low levels, if at all, similar to previous research (35). Thus, although the different herbals contained the same compounds, the relative amounts varied among species. This might be because climatic and edaphic conditions can have a strong influence on the composition of herbals (36–38). The total polyphenol, flavonoid, and condensed tannin contents of clove and star anise extracts were higher than in the other herbal extracts. Nevertheless, all plant extracts showed good acaricidal activity at 1 mg/mL, revealing for the first time the acaricidal effects of extracts of hibiscus and motherwort on adults of D. gallinae, with a mortality rate >98% (Table 2). In addition, star anise (LC50 = 0.159 g/mL) motherwort (LC50= 0.200 g/mL), and clove (LC50 = 0.292 g/mL) extracts showed significantly higher acaricidal activity compared with the other herbal extracts. Based on all parameters tested, the star anise and clove extracts exhibited the highest acaricidal activity, which could be due to the presence of a higher concentration of polar-soluble active molecule(s). Previous reports revealed that clove contains a volatile oil (15–20%) that mainly comprises eugenol (78–95%), acetyl eugenol (7.3%), and ß-caryophyllene (9%), star anise essential oil is characterized by phenylpropanoids (98.8 and 84.0%, respectively), with €-anethole (94.8 and 64.6%, respectively) as the predominant compound (27, 28). The authors investigated the efficacy of essential oil and fractions isolated from star anise and clove, respectively on D. gallinae, with in vitro test revealing a 100% mortality of clove at a concentration of 1.3 μg/m2; star anise was also found to have contact toxicity, with an LC50 of 59 μg/mL. There are also reports on the acaricidal and repellent properties of essential oils and extracts from other plant species against D. gallinae. for example, George et al. showed that thyme and cade oil are effective acaricides against D. gallinae when tested over a 24-h period (39). Nechita et al. tested ten essential oils (basil, thyme, coriander, eucalyptus, lavender, lemon, fir tree, oregano, mint, and juniper) against D. gallinae, with the best results observed for lavender (>97% mortality after 48 and 72 h) and thyme (84% at 72 h) at a dose of 0.12 mg/cm2 (40). Furthermore, Ghrabi-Gammar et al. reported that essential oil from Pelargonium graveolens killed 100% of D. gallinae exposed to it for 24 h at a concentration of 0.21 mg/cm2 (41). Studies on plant extracts have shown that the acaricidal effect of plant oils with more chemical components is more significant than that of essential oils with fewer chemical components, possibly because of the synergistic effect between chemical components (42). Our study showed that star anise LC90+ motherwort LC90 had a significant acaricidal effect on nymphal and larval D. gallinae, with a mortality rate of 100.00%. Star anise contains total phenolics (64.00 mg/mL), total flavonoids (0.76 mg/mL) and tannins (0.12 mg/mL), all of which have well-known acaricide and insecticide activities (43, 44). For example, tannin has a strong protein precipitation capacity and a high oxidation activity, which can interfere with egg hatching and larval development and larval movement, as indicated by its acaricidal activity against larvae and eggs reported that phenolic compounds were very effective in killing adult Rhipicephalus microplus and inhibiting their oviposition and hatching, with methyl eugenol being more active in terms of disrupting egg hatching (45, 46). At present, the author has not found any information about motherwort with mite prevention. However, total phenols (16.70 mg/mL) and total flavonoids (0.20 mg/mL) were detected in the alcohol extract of motherwort in this study. Thus, the acaricidal properties of star anise LC90+ motherwort LC90 might result from the combined involvement of phenolics, tannins, and flavonoids. However, further studies are required to determine the mechanism of action behind these effects.
In conclusion, star anise LC90+ motherwort LC90, rich in tannins, flavonoids, and polyphenol compounds, can be considered as a potential alternative to chemical insecticides because of their appreciable acaricidal properties against D. gallinae, an ectoparasite of veterinary importance in poultry and other livestock. As a continuation of this work and to confirm the current findings, the mechanism(s) of the synergistic and antagonistic effects between the herbs used in this study should be analyzed. In addition, examination of their acaricidal activities against ectoparasites should also be performed in field trials.
The original contributions presented in the study are included in the article/supplementary material, further inquiries can be directed to the corresponding author.
This study involved in vitro experiments and the Ethics governing the use and conduct of experiments on animals were strictly observed. Proper permits and consent were obtained from the Tongxu Poultry Farm management team before the Dermanyssus gallinae samples from poultry were used for this experiment.
YJ: methodology, formal analysis, validation, and writing—original draft preparation. FJ: conceptualization, supervision, project administration, and writing—review and editing. HY, DL, and QG: investigation. XL, SZ, CN, and LZ: theoretical guidance, supervision, and analysis of the experimental results. All authors contributed to the article and approved the submitted version.
This work was supported by the China Agriculture Research System of MOF and MARA (CARS-38).
We thank Associate Professor Erqin Zhang for help with using the equipment in this study.
Author QG was employed by Hennan Hemu Animal Pharmaceutical Co., Ltd.
The remaining authors declare that the research was conducted in the absence of any commercial or financial relationships that could be construed as a potential conflict of interest.
All claims expressed in this article are solely those of the authors and do not necessarily represent those of their affiliated organizations, or those of the publisher, the editors and the reviewers. Any product that may be evaluated in this article, or claim that may be made by its manufacturer, is not guaranteed or endorsed by the publisher.
1. Roy L, Giangaspero A, Sleeckx N, Øines Ø. Who is Dermanyssus gallinae? Genetic structure of populations and critical synthesis of the current knowledge. Front Veter Sci. (2021) 8:650546. doi: 10.3389/fvets.2021.650546
2. Koziatek-Sadłowska S, Sokół R. Changes in the percentages of B- and T-Lymphocytes and antibody titres in laying hens infested with Dermanyssus gallinae-A preliminary study. Animals. (2020) 10:987. doi: 10.3390/ani10060987
3. Sleeckx N, Van Gorp S, Koopman R, Kempen I, Van Hoye K, De Baere K, et al. Production losses in laying hens during infestation with the poultry red mite Dermanyssus gallinae. Avian Pathol. (2019) 48:S17–21. doi: 10.1080/03079457.2019.1641179
4. Cafiero MA, Barlaam A, Camarda A, Radeski M, Mul M, Sparagano O, et al. Dermanysuss gallinae attacks humans. Mind the gap! Avian Pathol. (2019) 48:S22–34. doi: 10.1080/03079457.2019.1633010
5. Sioutas G, Minoudi S, Tiligada K, Chliva C, Triantafyllidis A, Papadopoulos E. Case of human infestation with Dermanyssus gallinae (poultry red mite) from Swallows (Hirundinidae). Pathogens. (2021) 10:299. doi: 10.3390/pathogens10030299
6. Baker KP. Parasitic skin diseases of dogs and cats. Vet Rec. (1970) 87:452–9. doi: 10.1136/vr.87.16.452
7. Moroni B, Barlaam A, Misia AL, Peano A, Rossi L, Giangaspero A. Dermanyssus gallinae in non-avian hosts: a case report in a dog and review of the literature. Parasitol Int. (2021) 84:102378. doi: 10.1016/j.parint.2021.102378
8. De Luna CJ, Arkle S, Harrington D, George DR, Guy JH, Sparagano OA. The poultry red mite Dermanyssus gallinae as a potential carrier of vector-borne diseases. Ann N Y Acad Sci. (2008) 1149:255–8. doi: 10.1196/annals.1428.085
9. Huong CT, Murano T, Uno Y, Usui T, Yamaguchi T. Molecular detection of avian pathogens in poultry red mite (Dermanyssus gallinae) collected in chicken farms. J Veter Med Sci. (2014) 76:1583–7. doi: 10.1292/jvms.14-0253
10. Valiente Moro C, De Luna CJ, Tod A, Guy JH, Sparagano OA, Zenner L. The poultry red mite (Dermanyssus gallinae): a potential vector of pathogenic agents. Exp Appl Acarol. (2009) 48:93–104. doi: 10.1007/s10493-009-9248-0
11. Raele DA, Galante D, Pugliese N, La Salandra G, Lomuto M, Cafiero MA. First report of Coxiella burnetii and Borrelia burgdorferi sensu lato in poultry red mites, Dermanyssus gallinae (Mesostigmata, Acari), related to urban outbreaks of dermatitis in Italy. New Micr. New Infec. (2018) 23:103–9. doi: 10.1016/j.nmni.2018.01.004
12. Valiente Moro C, Chauve C, Zenner L. Experimental infection of Salmonella Enteritidis by the poultry red mite, Dermanyssus gallinae. Vet Parasitol. (2007) 146:329–36. doi: 10.1016/j.vetpar.2007.02.024
13. Gokbulut C, Ozuicli M, Aslan B, Aydin L, Cirak VY. The residue levels of spinosad and abamectin in eggs and tissues of laying hens following spray application. Avian Pathol. (2019) 48:S44–51. doi: 10.1080/03079457.2019.1623380
14. Katsavou E, Vlogiannitis S, Karp-Tatham E, Blake DP, Ilias A, Strube C, et al. Identification and geographical distribution of pyrethroid resistance mutations in the poultry red mite Dermanyssus gallinae. Pest Manag Sci. (2020) 76:125–33. doi: 10.1002/ps.5582
15. Pugliese N, Circella E, Cocciolo G, Giangaspero A, Horvatek Tomic D, Kika TS, et al. Efficacy of λ-cyhalothrin, amitraz, and phoxim against the poultry red mite Dermanyssus gallinae De Geer, 1778 (Mesostigmata: Dermanyssidae): an eight-year survey. Avian Pathol. (2019) 48:S35–43. doi: 10.1080/03079457.2019.1645295
16. Wang C, Xu X, Huang Y, Yu H, Li H, Wan Q, et al. Transcription profiling and characterization of Dermanyssus gallinae cytochrome P450 genes involved in beta-cypermethrin resistance. Vet Parasitol. (2020) 283:109155. doi: 10.1016/j.vetpar.2020.109155
17. Al-Snafi A E. Antiparasitic, antiprotozoal, molluscicidal and insecticidal activity of medicinal plants (part 2)—plant based review. Schol Acad J Pharm. (2016) 5:194–207. doi: 10.21276/sajp.2016.5.6.1
18. Pavela R, Canale A, Mehlhorn H, Benelli G. Application of ethnobotanical repellents and acaricides in prevention, control and management of livestock ticks: a review. Res Vet Sci. (2016) 109:1–9. doi: 10.1016/j.rvsc.2016.09.001
19. Choi WI, Lee SG, Park HM, Ahn YJ. Toxicity of plant essential oils to Tetranychus urticae (Acari: Tetranychidae) and Phytoseiulus persimilis (Acari: Phytoseiidae). J Econ Entomol. (2004) 97:553–8. doi: 10.1603/0022-0493-97.2.553
20. Isman MB. Botanical insecticides, deterrents, and repellents in modern agriculture and an increasingly regulated world. Ann Rev Entomol. (2006) 51:45–66. doi: 10.1146/annurev.ento.51.110104.151146
21. Marangi M, Cafiero MA, Capelli G, Camarda A, Sparagano OA, Giangaspero A. Evaluation of the poultry red mite, Dermanyssus gallinae (Acari: Dermanyssidae) susceptibility to some acaricides in field populations from Italy. Exp Appl Acarol. (2009) 48:11–8. doi: 10.1007/s10493-008-9224-0
22. Tabari MA, Youssefi MR, Barimani A, Araghi A. Carvacrol as a potent natural acaricide against Dermanyssus gallinae. Parasitol Res. (2015) 114:3801–6. doi: 10.1007/s00436-015-4610-0
24. Kostyukovsky M, Rafaeli A, Gileadi C, Demchenko N, Shaaya E. Activation of octopaminergic receptors by essential oil constituents isolated from aromatic plants: possible mode of action against insect pests. Pest Manag Sci. (2002) 58:1101–6. doi: 10.1002/ps.548
25. Pritchard J, Kuster T, Sparagano O, Tomley F. Understanding the biology and control of the poultry red mite Dermanyssus gallinae: a review. Avian Pathol. (2015) 44:143–53. doi: 10.1080/03079457.2015.1030589
26. Goleva I, Zebitz CP. Suitability of different pollen as alternative food for the predatory mite Amblyseius swirskii (Acari: Phytoseiidae). Exp Appl Acarol. (2013) 61:259–83. doi: 10.1007/s10493-013-9700-z
27. Lee SJ, Kim HK, Kim GH. Toxicity and effects of essential oils and their components on Dermanyssus gallinae (Acari: Dermanyssidae). Exp Appl Acarol. (2019) 78:65–78. doi: 10.1007/s10493-019-00363-7
28. Tabari MA, Rostami A, Khodashenas A, Maggi F, Petrelli R, Giordani C, et al. Acaricidal activity, mode of action, and persistent efficacy of selected essential oils on the poultry red mite (Dermanyssus gallinae). Food Chem Toxicol. (2020) 138:111207. doi: 10.1016/j.fct.2020.111207
29. Martins GR, Monteiro AF. do Amaral FRL, da Silva AS. A validated Folin-Ciocalteu method for total phenolics quantification of condensed tannin-rich açaí (Euterpe oleracea Mart). Food Sci Technol. (2021) 58:4693–702. doi: 10.1007/s13197-020-04959-5
30. Ruslin, Sahumena M H, Andriani R, Mamangkara M, Yamin. In vitro antioxidant activity test and determination of phenolic and flavonoid content of Moringa oleifera pulp and seeds. Food Res. (2021) 5:59–65. doi: 10.26656/fr.2017.5(4).033
31. Di Palma A, Giangaspero A, Cafiero MA, Germinara GS, A. gallery of the key characters to ease identification of Dermanyssus gallinae (Acari: Gamasida: Dermanyssidae) and allow differentiation from Ornithonyssus sylviarum (Acari: Gamasida: Macronyssidae). Parasit Vectors. (2012) 5:104. doi: 10.1186/1756-3305-5-104
32. Chu TT, Murano T, Uno Y, Usui T, Yamaguchi T. Molecular epidemiological characterization of poultry red mite, Dermanyssus gallinae, in Japan. J Veter Med Sci. (2015) 77:1397–403. doi: 10.1292/jvms.15-0203
33. Wang C, Ma Y, Huang Y, Su S, Wang L, Sun Y, et al. Darkness increases the population growth rate of the poultry red mite Dermanyssus gallinae. Parasit Vectors. (2019) 12:213. doi: 10.1186/s13071-019-3456-1
34. Abbott, W. A method of computing the effectiveness of an insecticide. 1925. J Am Mosq Control Ass. (1987) 3:302–3.
35. Alimi D, Hajri A, Jallouli S, Sebai H. In vitro acaricidal activity of essential oil and crude extracts of Laurus nobilis, (Lauraceae) grown in Tunisia, against arthropod ectoparasites of livestock and poultry: Hyalomma scupense and Dermanyssus gallinae. Vet Parasitol. (2021) 298:109507. doi: 10.1016/j.vetpar.2021.109507
36. Fernandes SS, Tonato D, Mazutti MA, Abreu BD, Cabrera D, D'Oca CM, Prentice-Hernandez C, Myriam SM. Yield and quality of chia oil extracted via different methods. J Food Eng. (2019) 262:200–208. doi: 10.1016/j.jfoodeng.2019.06.019
37. Jemaa J, Tersim N, Toudert KT, Khouja ML. Insecticidal activities of essential oils from leaves of Laurus nobilis L. from Tunisia, Algeria and Morocco, and comparative chemical composition. J Stored Prod Res. (2012) 48:97–104. doi: 10.1016/j.jspr.2011.10.003
38. Jerković I, Mastelić J, Miloš M. The impact of both the season of collection and drying on the volatile constituents of Origanum vulgare L ssp hirtum grown wild in Croatia. Int J Food Sci Technol. (2001) 36:649–54. doi: 10.1046/j.1365-2621.2001.00502.x
39. George DR, Olatunji G, Guy JH, Sparagano OA. Effect of plant essential oils as acaricides against the poultry red mite, Dermanyssus gallinae, with special focus on exposure time. Vet Parasitol. (2010) 169:222–5. doi: 10.1016/j.vetpar.2009.12.038
40. Nechita IS, Poirel MT, Cozma V, Zenner L. The repellent and persistent toxic effects of essential oils against the poultry red mite, Dermanyssus gallinae. Vet Parasitol. (2015) 214:348–52. doi: 10.1016/j.vetpar.2015.10.014
41. Ghrabi-Gammar Z, George DR, Daoud-Bouattour A, Jilani IBH, Saad-Limam SB, Sparagano OAE. Screening of essential oils from wild-growing plants in Tunisia for their yield and toxicity to the poultry red mite, Dermanyssus gallinae. Indus Crops Prod. (2009) 30:441–3. doi: 10.1016/j.indcrop.2009.07.001
42. Bordin C, Alves DS, Alves LFA, Oliveira MS, Ascari J, Scharf DR. Fumigant activity of essential oils from Cinnamomum and Citrus spp. and pure compounds against Dermanyssus gallinae (De Geer) (Acari: Dermanyssidae) and toxicity toward the nontarget organism Beauveria bassiana (Vuill). Vet Parasitol. (2021) 290:109341. doi: 10.1016/j.vetpar.2021.109341
43. Du J, Gao R, Zhao J. The effect of volatile oil from Chinese mugwort leaf on human demodecid mites in vitro. Acta Parasitol. (2021) 66:615–22. doi: 10.1007/s11686-020-00314-y
44. Zhang WJ, You CX, Yang K, Chen R, Wang Y, Wu Y, et al. Bioactivity of essential oil of Artemisia argyi Lévl. et Van and its main compounds against Lasioderma serricorne. J Oleo Sci. (2014) 63:829–37. doi: 10.5650/jos.ess14057
45. Engström MT, Karonen M, Ahern JR, Baert N, Payré B, Hoste H, et al. Chemical Structures of Plant Hydrolyzable Tannins Reveal Their in vitro activity against egg hatching and motility of Haemonchus contortus nematodes. J Agric Food Chem. (2016) 64:840–51. doi: 10.1021/acs.jafc.5b05691
Keywords: Chinese herbal compound, ethanol extract, poultry red mite, content determination, toxic effects
Citation: Jian Y, Yuan H, Li D, Guo Q, Li X, Zhang S, Ning C, Zhang L and Jian F (2022) Evaluation of the in vitro acaricidal activity of Chinese herbal compounds on the poultry red mite (Dermanyssus gallinae). Front. Vet. Sci. 9:996422. doi: 10.3389/fvets.2022.996422
Received: 17 July 2022; Accepted: 16 August 2022;
Published: 27 September 2022.
Edited by:
Wesley Lyeverton Correia Ribeiro, Federal University of Ceará, BrazilReviewed by:
Nathalie Sleeckx, Experimental Poultry Center (EPC), BelgiumCopyright © 2022 Jian, Yuan, Li, Guo, Li, Zhang, Ning, Zhang and Jian. This is an open-access article distributed under the terms of the Creative Commons Attribution License (CC BY). The use, distribution or reproduction in other forums is permitted, provided the original author(s) and the copyright owner(s) are credited and that the original publication in this journal is cited, in accordance with accepted academic practice. No use, distribution or reproduction is permitted which does not comply with these terms.
*Correspondence: Fuchun Jian, amZjaHVuMjAwOEBoZW5hdS5lZHUuY24=; amZjaHVuMjAwOEAxNjMuY29t
Disclaimer: All claims expressed in this article are solely those of the authors and do not necessarily represent those of their affiliated organizations, or those of the publisher, the editors and the reviewers. Any product that may be evaluated in this article or claim that may be made by its manufacturer is not guaranteed or endorsed by the publisher.
Research integrity at Frontiers
Learn more about the work of our research integrity team to safeguard the quality of each article we publish.