- 1Institute of Nutrition Metabolic Disease and Poisoning Disease in Animals, Qingdao Agricultural University, Qingdao, China
- 2Institute of Nutrition Metabolic Disease in Animals, Haidu College, Qingdao Agricultural, University, Laiyang, China
- 3Key Laboratory of Zoonosis, Ministry of Education, College of Veterinary Medicine, Jilin University, Changchun, China
The research evaluated the effects of Aflatoxin B1 on growth performance, antioxidant status, immune response, and pro-inflammatory cytokine mRNA expression in ISA chicks. In total, 240 7-day-old ISA chicks were randomly assigned to four treatment groups. The control group comprised chicks fed a basal diet. The aflatoxin (AFB1)-treatment groups (T1, T2, and T3) comprised chicks fed the basal diet supplemented with AFB1 at concentrations of 5, 8, and 10 μg/kg, respectively. The growth performance, antioxidant status, immune responses, and pro-inflammatory cytokine mRNA expression in all groups were measured. In the T1 treatment group (receiving the lowest AFB1 dose), a reduction in the Newcastle disease virus antibody (NDV-Ab) titer, and increases in interleukin 2 (IL-2), IL-6, and interferon γ (IFN-γ) mRNA levels were observed on days 21 and 42 (P < 0.05). Treatment with the higher AFB1 doses (groups T2 and T3) reduced the chicks' growth performance on days 21 and 42, measured as reductions in body weight (BW) and average daily gain (ADG) compared with the control group. In the T2 and T3 groups, the total antioxidant capacity (T-AOC), glutathione peroxidase (GPX) and superoxide dismutase (SOD) activities, serum immunoglobulin A (IgA) and IgG levels, and IL-2, IL-6, and IFN-γ levels were also lower than in the control group. On days 21 and 42, these two groups also showed increased malondialdehyde (MDA) content, higher feed to gain ratio (F/G), and higher IL-2, IL-6, and IFN-γ mRNA levels than the control group (P < 0.05). The T2 and T3 groups also showed reduced T-AOC, NDV-Ab titer, IL-2 content, and GPx-1 mRNA levels on days 21 and 42 (P < 0.05), increased IL-6 and IFN-γ mRNA levels on day 21, and increased F/G and MDA content on day 42 (P < 0.05) compared with group (T1). Increased MDA content and IL-6 mRNA levels in the liver and ileum were observed in group T3 compared with group T2 on day 21, and lower IgM and IL-6 levels were observed on days 21 and 42 (P < 0.05). In conclusion, our data showed that AFB1 exposure resulted in dose-dependent oxidative and inflammatory damage, immunosuppression, and a decline in the growth performance of chicks.
Introduction
Aflatoxins are toxic secondary metabolites produced by certain filamentous fungi, that occur widely in various foods and feeds (1, 2). Aflatoxin contamination can occur at every point along the food chain, from field to storage, including the feed-processing stage (3, 4). Aflatoxin B1 (AFB1) is the most toxic mycotoxin, with cytotoxic, genotoxic, and immunotoxic properties, and causes teratogenicity, mutagenesis and carcinogenesis (5, 6). AFB1 contamination can also cause the destruction of nutrients in raw materials or feed, reduce the palatability and nutritional value of feed, cause acute and chronic poisoning in animals, and lead to acute death (6, 7) and then cause significant economic losses.
Many studies have reported that poultry production is susceptible to AFB1 and that AFB1 contamination in feedstuffs poses a considerable threat to the growth and health of broiler chickens including weight gain, feed intake, and feed conversion ratio (8–11), and the growth and egg production of laying hens including egg production, egg size, and egg quality (12, 13) through toxic of the liver, kidneys, gastrointestinal tract and immune system. The immunosuppressive effect produced by aflatoxin AFB1 can be directly reduced effectiveness of vaccination programs, increased risk of infectious diseases, and high mortality (9).
However, most of the studies about negative effect of AFB1 were carried out on broilers and adult layers, the present study evaluated the toxic effects of AFB1 on growth performance, antioxidant status, immune response, and pro-inflammatory cytokine mRNA expression of pre-42-day-old ISA Chicks in order to improve the further understanding of AFB1 on the growth, health and immune suppression on egg production early of laying chicks, and provide theoretical basis for safe and healthy breeding of laying hens.
Materials and methods
Animals and treatment
In total, 240 1-day-old male chicks (ISA) were purchased from Institute of Animal Husbandry and Veterinary Medicine of Shandong Academy of Agricultural Sciences on day 0 after-hatching and randomly assigned to environmentally controlled brooder cages. Before the start of the experiment, all chicks were fed a basal diet for 7 days. The basal diet was a corn soybean- meal-based diet (Table 1), formulated to meet the nutritional requirements of ISA chicks aged 1–60 days. On day 7, the chicks were AFB1 assigned to four experimental treatment groups, each with six replicate pens, containing 10 chicks. The AFB1 content in the basal diet was confirmed with high-performance liquid chromatography tandem mass spectrometry (HPLC-MS; 1290 Infinity, Agilent, USA). The AFB1 content in the basal diet was 0, 5, 8, and 10 μg/kg according to the EU Commission Recommendation (14) and the Chinese Hygienic Standard for Feeds (15) guideline for the feed of young birds. The control group was fed the basal diet without AFB1 supplementation. The three AFB1 treatment groups, T1, T2 and T3, were fed the basal diet supplemented with AFB1 at concentrations of 2.70, 5.70 and 7.70 μg/kg, respectively. Based on the HPLC-MS analysis, the final AFB1 concentrations in the control, T1, T2, and T3 treatment groups feed were 2.30, 5.29, 8.43, and 10.90 μg/kg, respectively.
All animal experiments were approved by the Qingdao Agricultural University Animal Care and Use Committee (Qingdao, China) in accordance with Laboratory Animal-Guidelines for the Ethical Review of Animal Welfare (GB/T35892-2018, National Standards of the People's Republic of China). During the experiment, the chicks were housed in a closed and ventilated building under continuous light. The room temperature was maintained at 32–34°C for the first 3 days, and then gradually reduced by 3°C/week until a temperature of 24°C was reached. The room was maintained at 24°C for the remainder of the experiment. Over the entire experimental period of 42 days, water and feed were provided ad libitum. All the chicks were inoculated with the Newcastle disease virus (NDV) vaccine (La Sota strain) on day 7, the attenuated infectious bursal disease virus (IBDV) vaccine on day 12, NDV on day 21, and IBDV on day 27.
Growth performance
On day 42, body-weight (BW) and feed consumption (FC) were recorded, and the average daily gain (ADG) and feed conversion ratio (FCR) were calculated with the following formulaes:
Serum Sampling and analysis
Following the euthanization of the chicks on day 21 or 42, blood samples were drawn from the hearts of five randomly selected chicks in each treatment group. The blood samples were centrifuged at 3,000 × g for 15 min, and the serum was collected and stored at −20°C for further analysis. The glutathione peroxidase (GPX) activity, superoxide dismutase (SOD) activity, catalase (CAT) activity, total antioxidant capacity (T-AOC), and malondialdehyde (MDA) content in the sera were determined with assay kits (Nanjing Jiancheng Bioengineering Institute, Nanjing, China), according to the manufacturer's instructions. Serum immunoglobulin G (IgG), IgM, IgA, IL-2, IL-6, IFN-γ, anti-NDV antibody (NDV-Ab) titer, and anti-IBDV antibody (IBDV-Ab) titer were analyzed with enzyme-linked immunosorbent assay (ELISA) kits (Lair Biotechnology Co., Ltd., Hefei, China) according to the manufacturer's instructions.
Tissue sampling and analysis
Following the euthanization of chicks on day 21 or 42, the liver, glandular stomach, ileum and spleen were collected from three randomly selected chicks and immediately processed in liquid nitrogen before further analysis of the mRNA expression of IL-2, IL-6, IFN-γ, and cellular GPX (GPx-1). Briefly, the total RNA was extracted from the tissues with TRIZOL Reagent (Sangon Biotech Shanghai Co., Ltd., China). The RNA was then reverse transcribed in 40 μl of reaction mixture according to the manufacturer's instructions (Sangon Biotech Shanghai Co., Ltd., China) and stored in liquid nitrogen. The Primer Premier 5.0 software (PREMIER Biosoft International, USA) was used to design specific PCR primers (Table 2).
Quantitative real-time polymerase chain reaction (qPCR) was performed with SYBR® Premix Ex Taq™ II (Sangon Biotech Shanghai Co., Ltd., China) on the Applied Biosystems® 7500 Fast Real-time PCR System (Applied Biosystems, Foster, USA) according to the manufacturer's instructions. The experiment was repeated in triplicate. The ratios of mRNA levels to that of the β-actin mRNA internal control were used to statistically compare the different treatments by 2−ΔΔCt method.
Statistical analysis
All data are expressed as means ± standard deviations (SD), and were analyzed with one-way ANOVA to compare the means and with a multivariate general linear model (GLM) procedure in IBM SPSS for Windows version 22.0 (SPSS, Chicago, USA). The least significant difference (LSD) and Dunnett's T3 test were used to evaluate the differences between means. “Pen” was defined as the experimental unit for statistical purposes, and all calculations were generated based on pen averages. Differences were considered statistically significant at P < 0.05 for all tests.
Results
Exposure to AFB1 dose-dependently reduced growth performance
A significant reduction in FC and ADG were observed in the chicks exposed to the lowest dose of AFB1 (T1) compared with the control group (both P < 0.05). Further reductions in FC and ADG were observed in chicks given feed containing higher doses of AFB1 (T2 and T3) compared with the control and groups T1 (P < 0.05). Significantly higher FCR was observed in the T2 and T3 group than in the control and T1 groups (P < 0.05; Figure 1).
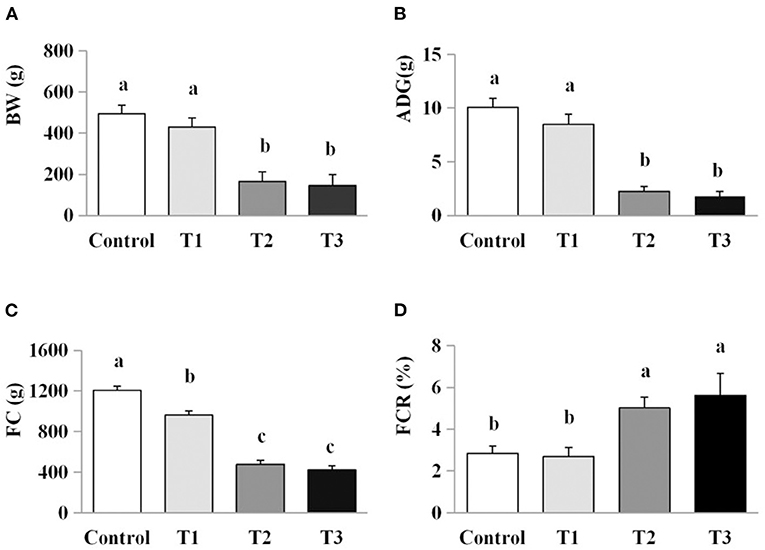
Figure 1. Effects of AFB1 on the growth performance of chicks. (A) BW, body weight. (B) ADG, average daily gain. (C) FC, feed consumption. (D) FCR, feed conversion ratio. Control: control group. T1: low doses of AFB1 group. T2: medium doses of AFB1 group. T3: high doses of AFB1 group. Data represent the means ± SD values of six replicate cages and were compared using one-way analysis of variance (ANOVA) followed by Duncan's multiple comparison tests. Different letters on the same row indicate significant differences (P < 0.05).
Exposure to AFB1 dose-dependently affected oxidative stress
The effect of AFB1 exposure on oxidative stress was examined by comparing the levels of oxidative stress markers (GPX, SOD, CAT, MDA and T-AOC) in the sera of the control and AFB1-treated chicks. The GPX and SOD activities in the AFB1 treatment groups (T1, T2, and T3) were reduced in a dose-dependent manner at days 21 and 42 compared with the control (P < 0.05; Figure 2). A dose-dependent reduced in T-AOC levels was also observed in the AFB1 treatment groups (T1, T2, and T3) on day 42 compared with that of the control group (P < 0.05). In contrast, the MDA levels in the AFB1 treatment groups (T1, T2, and T3) increased dose-dependently relative to those in control group on days 21 and 42 (P < 0.05; Figure 2). CAT activity was unaffected by any AFB1 treatment.
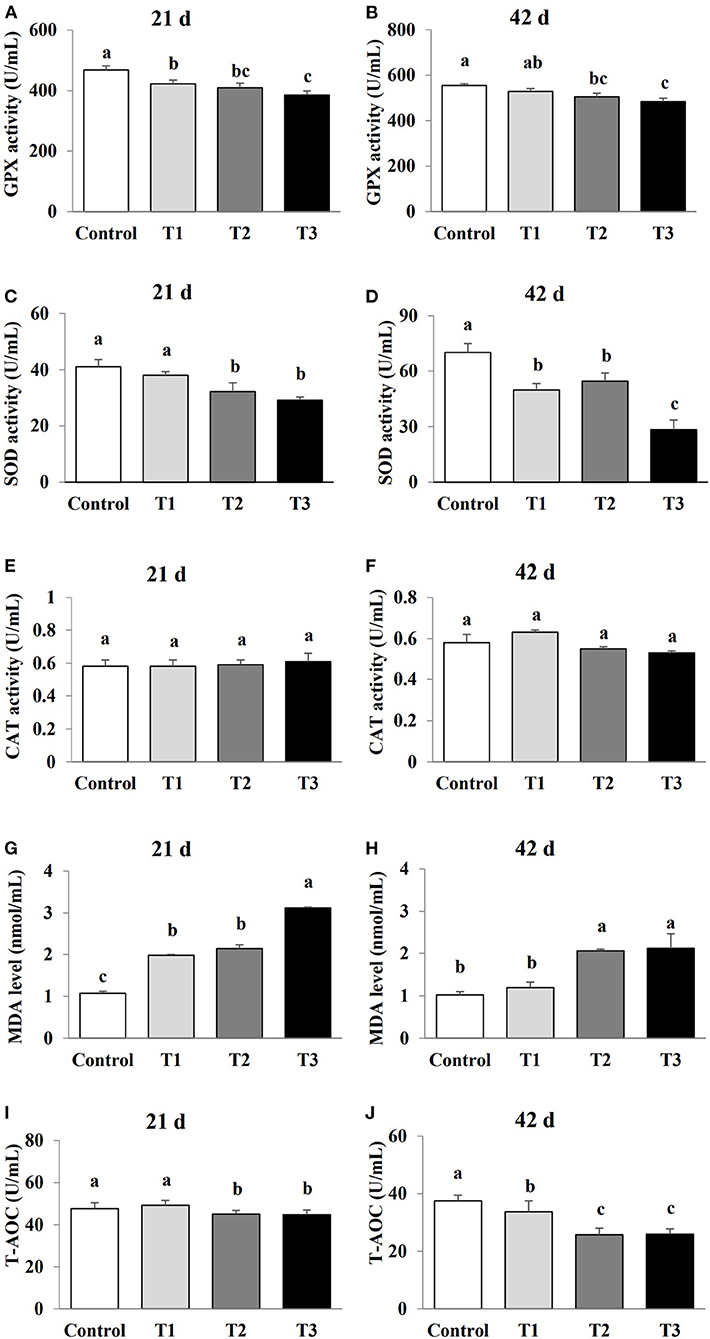
Figure 2. Effects of AFB1 on the antioxidant parameters in serum of chicks on day 21 and day 42. (A) GPX activity on day 21. (B) GPX activity on day 42. (C) SOD activity on day 21. (D) SOD activity on day 42. (E) CAT activity on day 21. (F) CAT activity on day 42. (G) MDA content on day 21. (H) MDA content on day 42. (I) T-AOC activity on day 21. (J) T-AOC activity on day 42. Control: control group. T1: low doses of AFB1group. T2: medium doses of AFB1 group. T3: high doses of AFB1 group. Data represent the means ± SD values of six replicate cages and were compared using one-way analysis of variance (ANOVA) followed by Duncan's multiple comparison tests. Different letters on the same row indicate significant differences (P < 0.05).
Exposure to AFB1 dose-dependently reduced serum immunoglobulin levels
The effects of AFB1 exposure on immunoglobulin levels (IgA, IgG, and IgM) in the sera of the control and AFB1-treated chicks were determined. The IgA and IgG levels in the AFB1-treatment groups (T1, T2, and T3) were significantly reduced in a dose-dependent manner at days 21 and 42 compared with those in the control (P < 0.05; Figure 3). A significant dose-dependent reduction in IgM was observed in the AFB1-treated chicks on day 42 compared with the controls (P < 0.05; Figure 3).
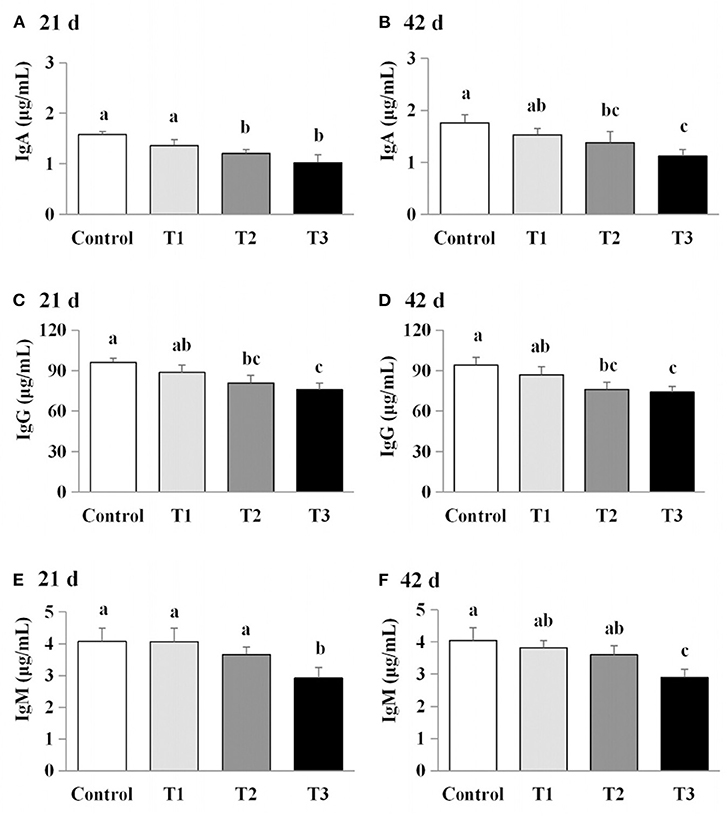
Figure 3. Effects of AFB1 on the immunoglobulin content in serum of chicks on day 21 and day 42 (A) IgA content on day 21. (B) IgA content on day 42. (C) IgG content on day 21. (D) IgG content on day 42. (E) IgM content on day 21. (F) IgM content on day 42. Control: control group. T1: low doses of AFB1group. T2: medium doses of AFB1 group. T3: high doses of AFB1 group. Data represent the means ± SD values of six replicate cages and were compared using one-way analysis of variance (ANOVA) followed by Duncan's multiple comparison tests. Different letters on the same row indicate significant differences (P < 0.05).
Exposure to AFB1 dose-dependently reduced serum cytokine levels
The effects of AFB1 exposure on cytokine levels (IL-2, IL-6, and IFN-γ) in the sera of the control and AFB1-treated chicks were determined. The IL-2, IL-6, and IFN-γ levels in the AFB1-treatment groups (T1, T2, and T3) were significantly and dose-dependently lower than those in the control group on days 21 and 42 (P < 0.05) (Figure 4).
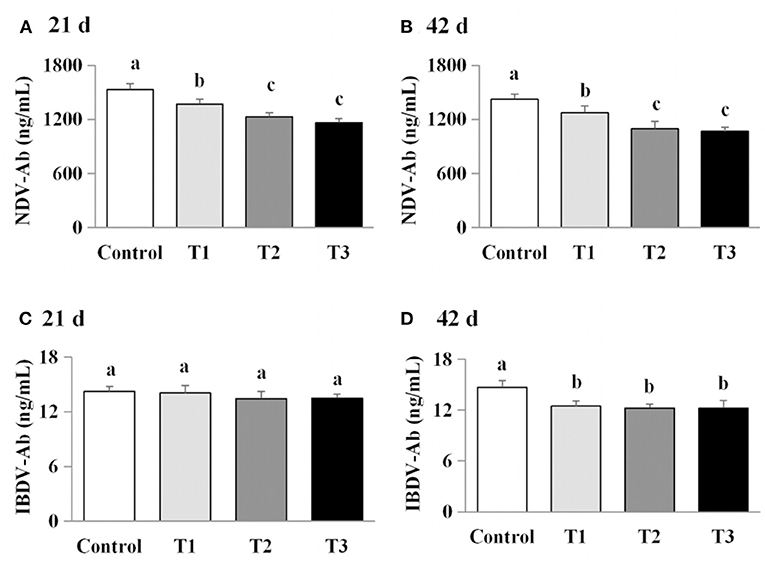
Figure 4. Effects of AFB1 on the antibody content in serum of chicks on day 21 and day 42. (A) IBDV-Ab content on day 21. (B) IBDV-Ab content on day 42. (C) NDV-Ab content on day 21. (D) NDV-Ab content on day 42. Control: control group. T1: low doses of AFB1group. T2: medium doses of AFB1 group. T3: high doses of AFB1 group. Data represent the means ± SD values of six replicate cages and were compared using one-way analysis of variance (ANOVA) followed by Duncan's multiple comparison tests. Different letters on the same row indicate significant differences (P < 0.05).
Exposure to AFB1 dose-dependently reduced serum antibody titers
We next examined the effects of AFB1 on the titers of IBDV-Ab and NDV-Ab. Compared with the control group, the AFB1-treated chicks (T1, T2, and T3) had a lower IBDV-Ab on day 21, and lower NDV-Ab and IBDV-Ab on days 42 (P < 0.05) (Figure 5). Furthermore, the NDV-Ab levels were lower on days 21 and 42 in the chicks exposed to the higher AFB1 concentrations (T2 and T3) than in the lowest-dose AFB1-treatment group (T1; P < 0.05; Figure 5).
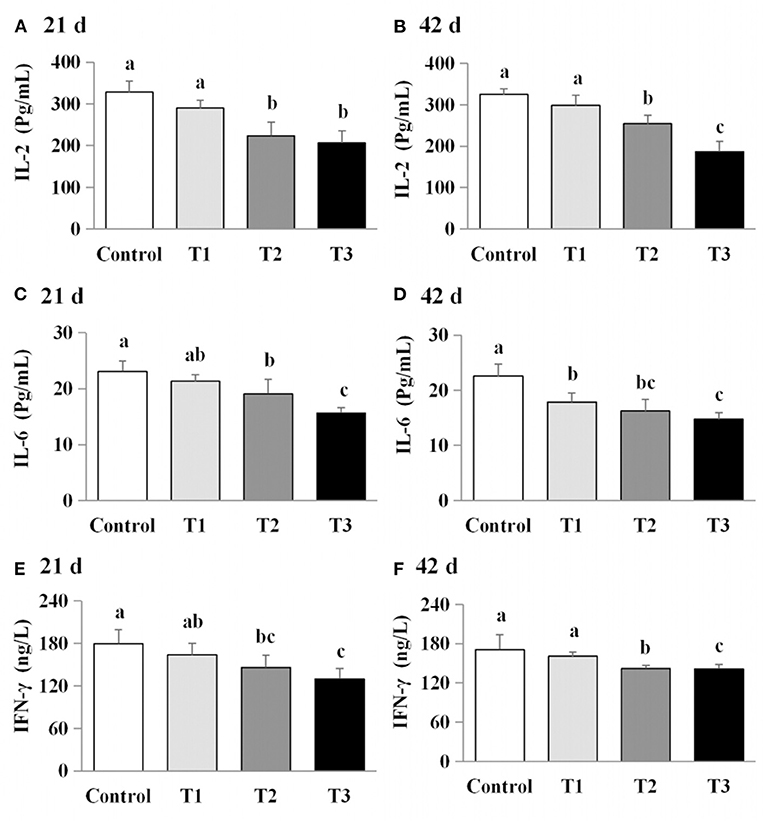
Figure 5. Effects of AFB1 on the immune factors content in serum of chicks on day 21 and day 42. (A) IL-2 content on day 21. (B) IL-2 content on day 42. (C) IL-6 content on day 21. (D) IL-6 content on day 42. (E) IFN-γ content on day 21. (F) IFN-γ content on day 42. Control: control group. T1: low doses of AFB1group. T2: medium doses of AFB1 group. T3: high doses of AFB1 group. Data represent the means ± SD values of six replicate cages and were compared using one-way analysis of variance (ANOVA) followed by Duncan's multiple comparison tests. Different letters on the same row indicate significant differences (P < 0.05).
Exposure to AFB1 dose-dependently altered in the expression of antioxidant enzymes and pro-inflammatory cytokines genes
The effects of AFB1 exposure on IL-2, IL-6, IFN-γ and GPx-1 mRNA levels in the livers, spleens, ileums and stomachs of control and AFB1-treated chicks were examined. IL-2, IL-6 and IFN-γ mRNA levels were significantly higher in all four organs of the AFB1-treated groups (T1, T2, and T3) than in those of the control group on days 21 and 42 (P < 0.05; Figure 6). On day 21, higher GPx-1 mRNA levels were observed in all four organs of the chicks exposed to the dose AFB1 treatment (T2) than in those of the control group. In contrast, reductions in the GPx-1 mRNA levels in all four organs were observed 21 days after treatment with the highest AFB1 dose (group T3) and in both the T2 and T3 groups at 42 days after treatment (P < 0.05) (Figure 6).
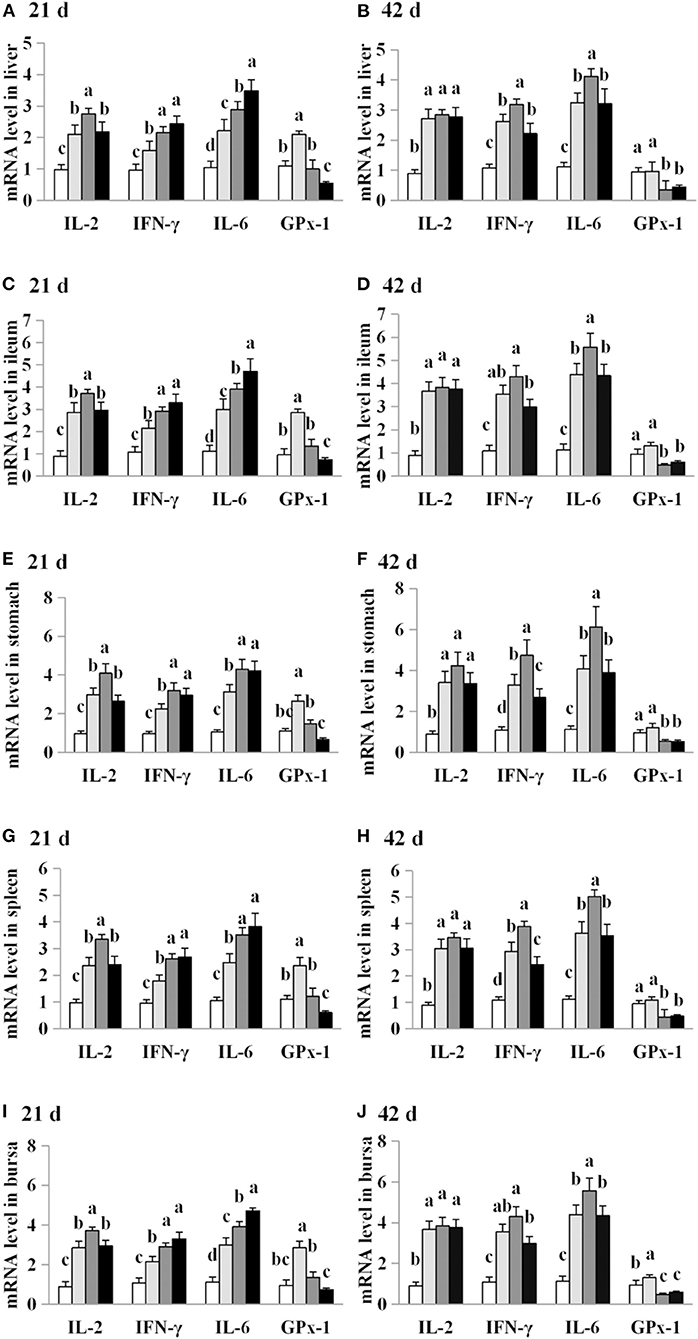
Figure 6. Effects of AFB1 on IL-2, IL-6, IFN-γ and GPx-1 mRNA levels in the liver, spleen, ileum, stomach and bursal of chicks on day 21 and day 42. (A) IL-2, IL-6, IFN-γ and GPx-1 mRNA levels in the liver on day 21. (B) IL-2, IL-6, IFN-γ and GPx-1 mRNA levels in the liver on day 42. (C) IL-2, IL-6, IFN-γ and GPx-1 mRNA levels in the spleen on day 21. (D) IL-2, IL-6, IFN-γ and GPx-1 mRNA levels in the spleen on day 42. (E) IL-2, IL-6, IFN-γ and GPx-1 mRNA levels in the ileum on day 21. (F) IL-2, IL-6, IFN-γ and GPx-1 mRNA levels in the ileum on day 42. (G) IL-2, IL-6, IFN-γ and GPx-1 mRNA levels in the stomach on day 21. (H) IL-2, IL-6, IFN-γ and GPx-1 mRNA levels in the stomach on day 42. (I) IL-2, IL-6, IFN-γ and GPx-1 mRNA levels in the bursal on day 21. (J) IL-2, IL-6, IFN-γ and GPx-1 mRNA levels in the bursal on day 42. Control: control group. T1: low doses of AFB1group. T2: medium doses of AFB1 group. T3: high doses of AFB1 group. Data represent the means ± SD values of six replicate cages and were compared using one-way analysis of variance (ANOVA) followed by Duncan's multiple comparison tests. Different letters on the same row indicate significant differences (P < 0.05).
Discussion
Growth performance is an important economical factor in all livestock industries, and is influenced by the effects of toxins on an animal's digestion and metabolism. In the present study, the concentrations of dietary AFB1 used in the diets examined here were based on the EUCR (14) and CHSF (15) guidelines for AFB1 concentrations in the feeds of young birds. The EUCR (14) AFB1 guideline recommends 5 μg/kg in dairy animal feeds and 10 μg/kg in feed for young animals, whereas the CHSF (15) AFB1 guideline recommends 10 μg/kg for feed for young animals. Here, we found that AFB1 supplementation led to a dose-dependent reduced in growth performance in chicks, measured as reductions in BW, ADG, and FC, and increase in FCR. These results are consistent with those reported in broiler chickens (6), where a dose-dependent effect particularly in feed intake was observed. Other meta-analyses have confirmed that the magnitude of these effects varied with the concentration of mycotoxins present in poultry and growing pigs (5, 16).
Numerous studies have reported that multiple mycotoxins, including AFB1, deoxynivalenol (DON), zearalenone (ZEN), and T-2 could induce oxidative stress (17, 18). Consistent with these studies, our findings indicated that AFB1 supplementation caused oxidative stress, leading to a-dose-dependent reductions in GPx-1 mRNA levels, T-AOC, and SOD and GPX activities, and dose-dependent increases in MDA levels, and demonstrated that the degree of oxidative stress was gradually enhanced as the level of AFB1 supplementation increased. As key enzymatic antioxidants, SOD and GPX, play important roles in eliminating reactive oxygen species (ROS) from cells (19–21). As the end product of lipid peroxidation, MDA is widely used as a late biomarker of oxidative stress and cellular damage (22). Our results suggested that the oxidative damage induced by AFB1 occurred mainly through an increase in lipid peroxidation and oxygen free radicals, in response to reduced SOD and GPX activities, and a reduction in GPx-1 mRNA levels. In contrast, Li et al. (23) reported that the oxidative damage induced by AFB1 occurred mainly through increased lipid peroxidation, but that it had no effect on antioxidant enzymes when administered to broiler chickens at a high concentration (74 μg/kg). Therefore, we hypothesize that chronic low doses of AFB1 may play an important role in inducing oxidative damage through the sensitive antioxidant enzymes in layer chicks.
The concentrations of IgA, IgG, and IgM and the titers of IBDV-Ab and NDV-Ab were previously found to decrease dose-dependently as the concentration of mycotoxins increased (5, 16). In the present study, our results confirmed that AFB1 supplementation significantly modulated the humoral immune response. Similarly, numerous studies have reported that multiple mycotoxins resulted in lower antibody titers (24, 25) and immunoglobulin concentrations (26) after vaccination. In contrast, other studies have demonstrated that many mycotoxins do not alter the concentrations of immunoglobulin subsets (24, 27, 28) and therefore failed to cause a significantly impair the specific humoral response after vaccination or sensitization (29–31). Therefore, different doses of AFB1 can cause distinct humoral immune responses, resulting in changes in immunoglobulin concentrations and antibody titers.
Cytokines are essential mediators of the inflammatory response and immune function. Macrophages, T cells and B cells are the central targets of multiple mycotoxins, which can be immunostimulatory or immunosuppressive (31, 32). Previous studies have shown that mycotoxin treatment can increase (25, 28, 33) or reduce inflammatory cytokine mRNA levels (22, 30, 34, 35). In the present study, AFB1 supplementation inhibited IL-2, IL-6, and IFN-γ protein production while promoting the mRNA expressions of these cytokines. Our findings are consistent with previous studies, which demonstrated that AFB1 treatment reduced the concentrations or mRNA levels of IL-2 or IL-4 but increased the gene expression of IL-1α, IL-6, IFN-γ, or TNF-α (7, 23). We consider that the effects of AFB1 on the inflammatory cytokines are dependent upon the dose administered, the duration of exposure, the susceptibility of each tissue, and the animal species examined, as well as other experimental conditions. Therefore, the increase in pro-inflammatory cytokine mRNA expression observed here may be attributable to the suppression of pro-inflammatory cytokines by AFB1 exposure.
In summary, AFB1 exposure, even at low levels, causes dose-dependent oxidative and inflammatory damage and immunosuppression, which reduced the growth performance of chicks.
Data availability statement
The original contributions presented in the study are included in the article/supplementary material, further inquiries can be directed to the corresponding author.
Ethics statement
All animal experiments were approved by Qingdao Agricultural University Animal Care and Use Committee (Qingdao, China) in accordance with Laboratory Animal-Guideline for ethical review of animal welfare (GB/T35892-2018, National Standards of the People's Republic of China).
Author contributions
LH designed the study and discussed the results and wrote the paper. HQ contributed to the cell and animal experiment. AL contributed to interpretation of findings. JD contributed to the data analyses. LZ and GL contributed to reviewing of the manuscript. FC was the principal investigator and in charge of the whole trial. All authors read and approved the final version of the manuscript.
Funding
This study was supported by Shandong Natural Science Foundation (ZR2021MC150), Open Project of Shandong Provincial Key Laboratory of Poultry Diseases Diagnosis and Immunology, and Shandong Modern Agricultural Technology and Industry System, China (SDAIT-11-07). Doctoral Fund project of Qingdao Agricultural University (663/1120017). These fund projects are publicly licensed and free of any potential commercial conflicts.
Acknowledgments
We are grateful to our laboratory technicians for their assistance with the experimental animals. We thank International Science Editing (http://www.internationalscienceediting.com) for editing this manuscript.
Conflict of interest
The authors declare that the research was conducted in the absence of any commercial or financial relationships that could be construed as a potential conflict of interest.
Publisher's note
All claims expressed in this article are solely those of the authors and do not necessarily represent those of their affiliated organizations, or those of the publisher, the editors and the reviewers. Any product that may be evaluated in this article, or claim that may be made by its manufacturer, is not guaranteed or endorsed by the publisher.
References
1. Arroyo-Manzanares N, Rodríguez-Estévez V, Arenas-Fernández P, García-Campaña AM, Gámiz-Gracia L. Occurrence of mycotoxins in swine feeding from Spain. Toxins. (2019) 11:342. doi: 10.3390/toxins11060342
2. Abdallah MF, Girgin G, Baydar T. Mycotoxin detection in maize, commercial feed, and raw dairy milk samples from Assiut City, Egypt. Vet Sci. (2019) 6:57. doi: 10.3390/vetsci6020057
3. Franco LT, Petta T, Rottinghaus GE, Bordin K, Gomes GA, Oliveira CAF. Co-occurrence of mycotoxins in maize food and maize-based feed from small-scale farms in Brazil: a pilot study. Mycotoxin Res. (2019) 35:65–73. doi: 10.1007/s12550-018-0331-4
4. Gruber-Dorninger C, Jenkins T, Schatzmayr G. Global mycotoxin occurrence in feed: a ten-year survey. Toxins. (2019) 11:375. doi: 10.3390/toxins11070375
5. Andretta I, Kipper M, Lehnen CR, Hauschild L, Vale MM, Lovatto PA. Meta-analytical study of productive and nutritional interactions of mycotoxins in broilers. Poult Sci. (2011) 90:1934–40. doi: 10.3382/ps.2011-01470
6. Marchioro A, Mallmann AO, Diel A, Dilkin P, Rauber RH, Blazquez FJ, et al. Effects of aflatoxins on performance and exocrine pancreas of broiler chickens. Avian Dis. (2013) 57:280–4. doi: 10.1637/10426-101712-Reg.1
7. Qian G, Tang L, Guo X, Wang F, Massey ME, Su J, et al. Aflatoxin B1 modulates the expression of phenotypic markers and cytokines by splenic lymphocytes of male F344 rats. J Appl Toxicol. (2014) 34:241–9. doi: 10.1002/jat.2866
8. Rawal S, Kim JE, Coulombe R Jr. Aflatoxin B1 in poultry: toxicology, metabolism and prevention. Res Vet Sci. (2010) 89:325–31. doi: 10.1016/j.rvsc.2010.04.011
9. Mughal MJ, Peng X, Kamboh AA, Zhou Y, Fang J. Aflatoxin B1 induced systemic toxicity in poultry and rescue effects of selenium and Zinc. Biol Trace Elem Res. (2017) 178:292–300. doi: 10.1007/s12011-016-0923-9
10. Jiang M, Peng X, Fang J, Cui H, Yu Z, Chen Z. Effects of aflatoxin b1 on T-cell subsets and mRNA expression of cytokines in the intestine of broilers. Int J Mol Sci. (2015) 16:6945–59. doi: 10.3390/ijms16046945
11. Chen J, Chen K, Yuan S, Peng X, Fang J, Wang F, et al. Effects of aflatoxin B1 on oxidative stress markers and apoptosis of spleens in broilers. Toxicol Ind Health. (2016) 32:278–84. doi: 10.1177/0748233713500819
12. Peng X, Chen K, Chen J, Fang J, Cui H, Zuo Z, et al. Aflatoxin B1 affects apoptosis and expression of Bax, Bcl-2, and Caspase-3 in thymus and bursa of fabricius in broiler chickens. Environ Toxicol. (2016) 31:1113–20. doi: 10.1002/tox.22120
13. Erdélyi M, Balogh K, Pelyhe C, Kövesi B, Nakade M, Zándoki E, et al. Changes in the regulation and activity of glutathione redox system, and lipid peroxidation processes in short-term aflatoxin B1 exposure in liver of laying hens. J Anim Physiol Anim Nutr. (2018) 102:947–52. doi: 10.1111/jpn.12896
14. EUCR. (2006). Available online at: https://wenku.baidu.com/view/ed603eec9b89680202d82501.html
15. CHSF. (2017). Available online at: https://www.doc88.com/p-1042508151654.html
16. Andretta I, Kipper M, Lehnen CR, Hauschild L, Vale MM, Lovatto PA. Meta-analytical study of productive and nutritional interactions of mycotoxins in growing pigs. Animal. (2012) 6:1476–82. doi: 10.1017/S1751731111002278
17. Tao W, Li Z, Nabi F, Hu Y, Hu Z, Liu J. Penthorum chinense pursh compound ameliorates AFB1-induced oxidative stress and apoptosis via modulation of mitochondrial pathways in broiler chicken kidneys. Front Vet Sci. (2021) 8:750937. doi: 10.3389/fvets.2021.750937
18. Pelyhe C, Kövesi B, Zándoki E, Kovács B, Erdélyi M, Kulcsár S, et al. Multi-trichothecene mycotoxin exposure activates glutathione-redox system in broiler chicken. Toxicon. (2018) 153:53–7. doi: 10.1016/j.toxicon.2018.08.010
19. Chen F, Zhu L, Qiu H, Qin S. Selenium-enriched Saccharomyces cerevisiae improves growth, antioxidant status and selenoprotein gene expression in Arbor Acres broilers. J Anim Physiol Anim Nutr. (2017) 101:259–66. doi: 10.1111/jpn.12571
20. Rašić D, Micek V, Klarić MS, Peraica M. Oxidative stress as a mechanism of combined OTA and CTN toxicity in rat plasma, liver and kidney. Hum Exp Toxicol. (2019) 38:434–45. doi: 10.1177/0960327118819049
21. Farzaei MH, Zobeiri M, Parvizi F, El-Senduny FF, Marmouzi I, Coy-Barrera E, et al. Curcumin in liver diseases: a systematic review of the cellular mechanisms of oxidative stress and clinical perspective. Nutrients. (2018) 10:855. doi: 10.3390/nu10070855
22. Ren Z, Wang Y, Deng H, Deng Y, Deng J, Zuo Z, et al. Deoxynivalenol-induced cytokines and related genes in concanavalin A-stimulated primary chicken splenic lymphocytes. Toxicol In Vitro. (2015) 29:558–63. doi: 10.1016/j.tiv.2014.12.006
23. Li Y, Ma QG, Zhao LH, Wei H, Duan GX, Zhang JY, et al. Effects of lipoic acid on immune function, the antioxidant defense system, and inflammation-related genes expression of broiler chickens fed aflatoxin contaminated diets. Int J Mol Sci. (2014) 15:5649–62. doi: 10.3390/ijms15045649
24. Taranu I, Marin DE, Bouhet S, Pascale F, Bailly JD, Miller JD, et al. Mycotoxin fumonisin B1 alters the cytokine profile and decreases the vaccinal antibody titer in pigs. Toxicol Sci. (2005) 84:301–7. doi: 10.1093/toxsci/kfi086
25. Choi BK, Jeong SH, Cho JH, Shin HS, Son SW, Yeo YK, et al. Effects of oral deoxynivalenol exposure on immune-related parameters in lymphoid organs and serum of mice vaccinated with porcine parvovirus vaccine. Mycotoxin Res. (2013) 29:185–92. doi: 10.1007/s12550-013-0161-3
26. Marin DE, Taranu I, Burlacu R, Manda G, Motiu M, Neagoe I, et al. Effects of zearalenone and its derivatives on porcine immune response. Toxicol In Vitro. (2011) 25:1981–8. doi: 10.1016/j.tiv.2011.06.022
27. Pinton P, Accensi F, Beauchamp E, Cossalter AM, Callu P, Grosjean F, et al. Ingestion of deoxynivalenol (DON) contaminated feed alters the pig vaccinal immune responses. Toxicol Lett. (2008) 177:215–22. doi: 10.1016/j.toxlet.2008.01.015
28. Meissonnier GM, Pinton P, Laffitte J, Cossalter AM, Gong YY, Wild CP, et al. Immunotoxicity of aflatoxin B1: impairment of the cell-mediated response to vaccine antigen and modulation of cytokine expression. Toxicol Appl Pharmacol. (2008) 231:142–9. doi: 10.1016/j.taap.2008.04.004
29. Watzl B, Neudecker C, Hänsch GM, Rechkemmer G, Pool-Zobel BL. Short-term moderate aflatoxin B1 exposure has only minor effects on the gut-associated lymphoid tissue of Brown Norway rats. Toxicology. (1999) 138:93–102. doi: 10.1016/S0300-483X(99)00088-8
30. Marin DE, Taranu I, Bunaciu RP, Pascale F, Tudor DS, Avram N, et al. Changes in performance, blood parameters, humoral and cellular immune responses in weanling piglets exposed to low doses of aflatoxin. J Anim Sci. (2002) 80:1250–7. doi: 10.2527/2002.8051250x
31. Goyarts T, Dänicke S, Tiemann U, Rothkötter HJ. Effect of the Fusarium toxin deoxynivalenol (DON) on IgA, IgM and IgG concentrations and proliferation of porcine blood lymphocytes. Toxicol In Vitro. (2006) 20:858–67. doi: 10.1016/j.tiv.2005.12.006
32. Reddy KE, Song J, Lee HJ, Kim M, Kim DW, Jung HJ, et al. Effects of high levels of deoxynivalenol and zearalenone on growth performance, and hematological and immunological parameters in pigs. Toxins. (2018) 10:114. doi: 10.3390/toxins10030114
33. Lessard M, Savard C, Deschene K, Lauzon K, Pinilla VA, Gagnon CA, et al. Impact of deoxynivalenol (DON) contaminated feed on intestinal integrity and immune response in swine. Food Chem Toxicol. (2015) 80:7–16. doi: 10.1016/j.fct.2015.02.013
34. Ghareeb K, Awad WA, Soodoi C, Sasgary S, Strasser A, Böhm J. Effects of feed contaminant deoxynivalenol on plasma cytokines and mRNA expression of immune genes in the intestine of broiler chickens. PLoS ONE. (2013) 8:e71492. doi: 10.1371/journal.pone.0071492
Keywords: aflatoxin B1, chick, growth, oxidant damage, pro-inflammatory cytokine
Citation: Hou L, Qiu H, Li A, Dong J, Zhu L, Liu G and Chen F (2022) Effects of aflatoxin B1 on growth performance, antioxidant status, immune response, and pro-inflammatory cytokine mRNA expression in ISA chicks. Front. Vet. Sci. 9:993039. doi: 10.3389/fvets.2022.993039
Received: 13 July 2022; Accepted: 05 August 2022;
Published: 13 September 2022.
Edited by:
Zhihua Ren, Sichuan Agricultural University, ChinaReviewed by:
Gang Liu, University of Copenhagen, DenmarkYong Wang, Tianjin Agricultural University, China
Shaojun He, Anhui Science and Technology University, China
Copyright © 2022 Hou, Qiu, Li, Dong, Zhu, Liu and Chen. This is an open-access article distributed under the terms of the Creative Commons Attribution License (CC BY). The use, distribution or reproduction in other forums is permitted, provided the original author(s) and the copyright owner(s) are credited and that the original publication in this journal is cited, in accordance with accepted academic practice. No use, distribution or reproduction is permitted which does not comply with these terms.
*Correspondence: Fu Chen, Y2Y1MDcmI3gwMDA0MDtzb2h1LmNvbQ==
†These authors have contributed equally to this work