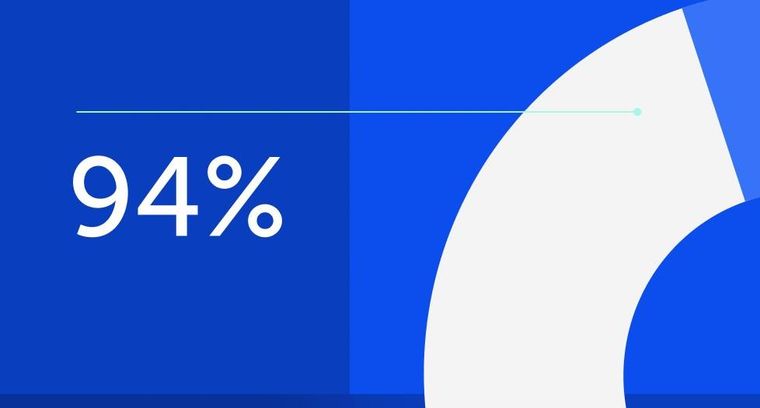
94% of researchers rate our articles as excellent or good
Learn more about the work of our research integrity team to safeguard the quality of each article we publish.
Find out more
ORIGINAL RESEARCH article
Front. Vet. Sci., 23 August 2022
Sec. Veterinary Infectious Diseases
Volume 9 - 2022 | https://doi.org/10.3389/fvets.2022.970327
Background: Trichinellosis is a helminthic disease caused by Trichinella spiralis via the ingestion of raw or undercooked meat of infected animals. Current estimates indicate that 11 million humans have trichinellosis, worldwide. The effective use of anti-trichinella medications is limited by side effects and resistance which highlight the critical need for safe and effective drugs, particularly those derived from medicinal plants. Therefore, in the present study, we aimed to evaluate the efficacy of the ethanolic extract of Artemisia annua (A. annua) in treatment of experimentally induced trichinellosis.
Materials and methods: Trichinellosis was induced experimentally in male 6–8 weeks BALB/c mice. BALB/c mice were divided into four groups, 10 mice each. One group was left uninfected and untreated, whereas three groups were infected with T. spiralis. One infected group of mice was left untreated (negative control) while the remaining two infected groups received either 300 mg/kg of the ethanolic extract of A. annua or 50 mg/kg of albendazole (positive control). All treatments started from the third day post-infection (dpi) for 3 successive days. All animals were sacrificed on the 7th dpi for evaluation of treatment efficacy.
Results: Our findings showed that A. annua treatment reduced the T. spiralis adult-worm count in the intestine of infected animals. Moreover, treatment with A. annua restored the normal intestinal architecture, reduced edema, alleviated inflammation as demonstrated by reduced inflammatory infiltrate and expression of TGF-β in intestinal tissues of A. annua-treated animals compared to infected untreated animals.
Conclusions: Our findings show that A. annua extract is effective in treating experimentally induced trichinellosis which highlight the therapeutic potential of A. annua for intestinal trichinellosis.
Trichinellosis is a helminth disease caused by Trichinella spiralis nematode (T. spiralis). Ingestion of the raw or undercooked meat of infected animals is the major source for the transmission of T. spiralis that have infected around 11 million humans globally (1). In 2014, the Food and Agriculture Organization of the United Nations (FAO), and the World Health Organization (WHO) had listed T. spiralis among the top 10 most common foodborne parasites that eventually lead to serious health problems. Trichinellosis have become a public health concern and is considered a re-emerging disease with recent outbreaks reported in 55 countries (2–6).
The early clinical signs of trichinellosis are gastrointestinal problems, such as abdominal pain, diarrhea, nausea, and vomiting, which commonly start 2–7 days following consumption of raw or undercooked meat (7). These manifestations are due to invasion of intestinal epithelial cells by T. spiralis larvae where they grow to adults, mate and produce neonatal larvae 3–7 days post infection (dpi) (7). Studies have shown that adult T. spiralis were expelled from the intestine within 10–17 days following infection which indicates that the intestinal phase (the first 17 dpi) is considered the critical trichinellosis stage which determines the progression and outcome of the disease (8, 9).
T. spiralis adult worms have been shown to trigger acute inflammatory responses resulting in a series of intestinal pathological changes (10). Previous studies have shown that Th2, dendritic cells (DCs), eosinophils, and mast cells are the key cells involved in immune responses against T. spiralis. A strong Th2 response was demonstrated in T. spiralis infection which eventually lead to a transient inflammatory reaction and worm expulsion (11–14). Th2 immune response is mediated by soluble mediators such as IL-4, IL-5, IL-9, IL-13 which in addition to histamine, released from mast cells, and antibodies (IgE and IgG1) result in adult worm expulsion (15, 16). Although, the expulsion mechanisms depend mainly on Th2 cytokines; however, the pathways and mechanisms responsible for this process remain unclear (15, 16).
To date, albendazole and mebendazole are the main anthelmintic medications used for treatment of trichinellosis (17). However, their therapeutic utility is limited by emerging resistance and the reported side effects such as acute liver injury, anemia, leukopenia, hypercholesterolemia, proteinuria, hemolytic anemia, and, in rare occasions, kidney and brain injuries (18–22). Moreover, safety for pregnant women and children under the age of 3 years remains a concern (23). Therefore, the development of safe and effective anti-trichinellosis drugs is a necessity (24).
In traditional medicine, medicinal plants have long been used. Artemisia annua (A. annua), a plant known for its malarial properties, has been researched for a variety of biological activities, including anticancer, antidiabetic, and antibacterial properties (25–32). In addition to treatment of several parasitic diseases such as giardiasis (33), toxoplasmosis (34) and leishmaniasis (35). A. annua contains a wide range of chemical compounds (36, 37). Many bioactive compounds have been isolated from this plant; however, artemisinin is the most active of these compounds (38–40). Other important constituents in A. annua include specific sesquiterpene lactones, essential oils (41), tannins, saponins (42), polyalkenes (43), phytosterols, fatty acids (42), and proteins (42).
In the present study, we evaluated the efficacy of the ethanolic extract of A. annua in treatment of BALB/c mice, that were experimentally infected with T. spiralis. Based on parasitological and histopathological analysis, we report high efficacy of A. annua in treatment of intestinal trichinellosis. Our results indicate that A. annua ethanolic extract is a potential natural therapeutic for trichinellosis.
Leaves of A. annua were obtained during the flowering stage from a farmed field near South Valley University, Qena, Egypt. Plant was identified by Prof. Naglaa Lofty; an associate professor of Botany at the Faculty of Science, South Valley University, Qena, Egypt. The plant's voucher specimen (code: Aa.78) was kept in the herbarium of the Department of Pharmacognosy, Faculty of Pharmacy, South Valley University, Qena, Egypt following their taxonomic identification. The freshly collected plant was allowed to dry at room temperature before being grounded into fine powder. A. annua ethanolic extract was then prepared as previously described (44). Briefly, 200 g of powder was macerated in 1L of 95% ethanol at 25°C for 16h, filtered, and the filtrate was dried in a rotary evaporator at 40°C under reduced pressure (45). The dried extract was stored at 4°C till used in the preparation of 300 mg/kg dose for mice experiments (46, 47). The reference drug albendazole was obtained as a suspension (20 mg/mL) from the Egyptian International Pharmaceutical Industries Co, Cairo, Egypt.
BALB/c mice infected with T. spiralis were obtained from the Faculty of Medicine, Assiut University, Assiut, Egypt. Larvae were obtained from the infected muscles as previously described (48). Briefly, infected muscles were digested by immersion in a digestive solution (1,000 ml saline containing 20 mL of concentrated HCl and 20 g of Pepsin) for 12 h at 37°C on a mechanical stirrer. To release the larvae, the suspension was centrifuged at 1,000 rpm for 2 min. Material was rinsed with normal saline (0.9% NaCl) and was re-centrifuged. The larvae were counted by using hemocytometer to determine the inoculum size that required to infect mice. The sediment containing the larvae was then re-suspended in saline containing 1.5% gelatin for their use in the animal experiments.
Parasite free 6–8-week-old male BALB/c mice (N = 40), weighing 25–30 g, were purchased from Theodor Biharz Research Institute, Giza, Egypt, and were kept in a sanitary condition in Faculty of Veterinary Medicine, South Valley University, Qena, Egypt during the whole experiment. Mice were housed under controlled temperature and light conditions with water and standard rodent diet, ad libitum.
Mice were divided into four groups, 10 mice each. Group A represent uninfected mice (negative control) while Groups B, C, and D were starved for 12 h then orally infected, each with 300 T. spiralis larvae, using a syringe (24). Group B did not receive any treatment (infected untreated mice), whereas Group C and D infected mice were treated with 50 mg/kg albendazole (49) and 300 mg/kg of the A. annua ethanolic extract, respectively (18, 33). All treatments started from the third day post-infection (3 dpi) for three consecutive days (18, 50). All animals were sacrificed on the 7th dpi for the evaluation of treatment efficacy (24, 49, 51).
T. spiralis adult worms were isolated from the small intestine of each mouse and counted as previously described by Denham (52). The small intestine of each animal was split longitudinally, cut into 5 cm pieces and incubated in 0.9% saline for 2h at 37°C (53). Subsequently, intestine was incubated in sodium hydroxide (0.05%) for 6h at 5°C. The worms were then retrieved by washing the intestines with water and passing the wash through via a 200-mesh sieve (52). The collected material was washed with distilled water and streaked across 2- × 3-inch slides by using a Pasteur pipette. Total number of worms in all intestinal 5 cm pieces/animal were counted under a dissecting microscope and the mean number of worms/group was calculated for comparison between different groups.
For detection of histopathological changes in different animal groups, we used 1 cm specimens from the junction of the proximal (1/3) and distal (2/3) parts of the intestine of mice sacrificed on the 7th dpi. The intestinal specimens were fixed in 10% formalin and embedded in paraffin (54). Sections, with a 3 mm thickness, were stained with hematoxylin and eosin (H&E) as previously described (54, 55) and evaluated by an independent blinded pathologist. Histopathological changes of the intestinal tissue were used to assess the intensity of inflammation and were scored from 0 to 4 (0, normal; 1, mucosal hyperplasia; 2, spotty infiltration by inflammatory cells not involving the entire mucosa and/or submucosal thickness; 3, marked increase of inflammatory cells involving the full thickness of the mucosa and/or submucosal thickness; 4, marked increase of inflammatory cells in both the mucosa and submucosa).
Villi length was measured from the tip of villi to their base at the mucosal surface. Crypt depth was measured from the base of villi up to the beginning of the muscularis mucosa. For the detection of eosinophils, at least three sections/animal were examined under light microscopy at 400X magnification.
We used fixed 2 cm sections of the duodenum, jejunum, and ileum with Carnoy's solution. The tissues were embedded in paraffin, cut with a microtome, and stained with Periodic Acid Schiff (PAS) stain. Hematoxylin was used as the counterstain. The number of goblet cells in mucosal linings in 3 high power fields (HPF; 400X) were counted, and the mean number ± S.D was calculated (56).
Small intestine sections embedded in paraffin were cut into 4 μm thick sections for immunohistochemical (IHC) staining. Sections were de-paraffinized and rehydrated with descending ethanol grades. Sections were placed in citrate buffer (pH 6.0) and heated in microwave for epitope retrieval. Endogenous peroxidases were then blocked by using 3% H2O2 in ethanol (for 10 min) and incubated with the primary anti-TGF-β rabbit polyclonal antibody (ABclonal USA, Catalog No.: A16640) for 1h at room temperature. Sections were washed with TBS containing 0.05% Tween-20 (TBS-T) and incubated with HRP-conjugated goat anti-rabbit secondary antibody for 1h at room temperature (Abcam, Cambridge, MA, USA. Catalog No.: HRP ab6728). Finally, slides were washed with TBS-T and the color was developed by incubating the tissue sections with 0.05% diaminobenzidine (DAB) and 0.01% H2O2 for 3 min. The sections were finally counterstained with hematoxylin for 1 min prior to dehydration and mounting. Sections with no added primary antibody were used as negative control. Images were captured using an Olympus light microscope equipped with a digital camera (Olympus, Japan, BX53). The number of TGF-β-positive mucosal glandular and stromal cells, and submucosal stromal cells were counted in 10 images captured at 400x and their percentage were calculated (57).
The results were analyzed using the Statistical Package for Social Sciences (SPSS) version 20 for Windows. All values are expressed as mean ± Standard deviation (SD). ANOVA test was used for the comparison of mean number of adult worms, goblet cells and eosinophils in the intestine wall between all groups of animals. P-values < 0.05 were considered statistically significant.
We aimed to evaluate the anthelmintic efficacy of A. annua extract against T. spiralis adult worms in the intestine of infected mice. Examination of the small intestines of A. annua and albendazole-treated animals (positive control) revealed a significant reduction in the number of T. spiralis adult worms compared to infected untreated animals (P = 0.004) (Figure 1). However, there was no significant difference between the number of adult worms in A. annua and albendazole-treated mice (P = 0.19). Our results indicated that A. annua extract is effective in reducing the number of T. spiralis adult worms in the small intestine of infected mice.
Figure 1. Effect of A. annua treatment on the number of adult T. spiralis worm in the small intestine of infected mice. Treatment with A. annua extract significantly reduced worm count in the intestine of infected mice, an effect that was comparable to albendazole (positive control). Data are expressed as mean ± SD (N = 10) and analyzed by using ANOVA followed by LSD as a post hoc test. Asterisk (*) indicate significant difference; p < 0.05.
Next, we examined the intestinal sections of different mice groups to evaluate the therapeutic effect of A. annua in reducing the pathological changes due to T. spiralis infection. H&E staining of intestinal sections of uninfected untreated mice showed long regular, finger-like intestinal villi (black arrows), shallow intestinal crypts, and sparse goblet cells (red arrows) (Figure 2A). However, intestinal sections of infected untreated mice showed shortened, leaf-shaped, degenerated, distorted and edematous intestinal villi with some areas showing complete villous atrophy (black arrows) and significant mucosal inflammation (red arrows) (Figure 2B). Higher magnification (400x) showed edema in villi cores and lamina propria (blue arrows) and increase in goblet cell numbers (black arrows) (Figure 2C). Moreover, inflammatory cellular infiltrate was detected in the lamina propria composed mainly of eosinophils (red arrows). T. spiralis adult worms were also observed (red arrows) lying on surface of distorted intestinal villi (black arrows) (Figure 2D). Conversely, the intestinal sections of mice treated with A. annua extract showed marked improvement of the villi outline along with reduced edema (black arrows) and absence of worms (Figure 2E). Higher magnification (400x) demonstrated similar frequency of goblet cells (black arrows) but reduced inflammatory infiltrate (red arrows) (Figure 2F) compared to infected untreated mice. Similarly, albendazole treatment preserved villi structure and length with mild edematous cores (black arrows) (Figure 2G), while reducing inflammatory cellular infiltrates (red arrows) with no significant change in numbers of goblet cells compared to infected untreated mice (black arrows) (Figure 2H).
Figure 2. Treatment with A. annua restored villi architecture and alleviated inflammation in intestinal tissues of T. spiralis-infected mice. (A) Representative image of small intestinal tissue of uninfected mice stained with H&E showing uniform villi and goblet cells (100x). (B) Representative image of small intestinal tissue of T. spiralis-infected untreated mice showing distorted degenerated edematous villi (black arrows) with significant mucosal inflammation (red arrows) (100x). (C) Higher magnification of (B) (400x) demonstrating edema in villi and lamina propria (blue arrows) and also high goblet cell numbers (black arrows) and inflammatory infiltrate composed mainly of eosinophils (red arrows) (D) Higher magnification of (B) (400x) with parasite on surface (red arrows) of distorted villi (black arrows). (E) Representative image of small intestinal tissue of infected mice treated with A. annua extract showing restoration of villi outline and reduced edema (black arrows). (F) Higher magnification (400x) of (E) with similar number of goblet cells (black arrows) and lower inflammatory infiltrate than infected untreated mice (red arrows). (G). Representative image of small intestinal tissue of infected mice treated with albendazole showing protected villi and reduced edema (black arrows) compared to infected untreated mice. (H) Higher magnification (400x) of (G) showing reduced inflammatory infiltrate (red arrows) and similar frequency of goblet cell number (black arrows) compared to infected untreated mice.
Interestingly, we found that eosinophils were significantly elevated in all T. spiralis-infected mice compared with uninfected mice (P = 0.007) (Figure 3). We also detected increase in goblet cell number in all infected animal groups compared to uninfected animals (P = 0.003). To further valid our observation, we used periodic acid Schiff staining for the visualization of goblet cells. In uninfected mice, goblet cells were distributed among the epithelial cells covering the villi. A significantly higher number of goblet cells were observed in intestine sections from T. spiralis-infected animals compared to uninfected animals (P < 0.003). There was no significant difference in the number of goblet cells between A. annua or albendazole-treated mice and infected untreated mice (Figures 4, 5).
Figure 3. Eosinophil cell numbers in intestinal tissues of different groups of mice. Infection of mice with T. spiralis significantly increased eosinophil cell number in intestinal tissue/high power field (HPF) compared to uninfected mice. Treatment with either A. annua or albendazole (positive control) did not result in significant change (ns) in eosinophil cell numbers infiltrating the mucosa and submucosa of small intestine compared to infected untreated mice. Asterisk (*) indicate significant difference between uninfected and infected untreated mice (p < 0.007), whereas ns, non-significant.
Figure 4. Representative microphotographs of intestinal tissue of different groups of mice showing goblet cells. Periodic Acid Schiff's (PAS) staining was performed to demonstrate goblet cells in intestinal tissues. (A) Uninfected mice. (B) Infected untreated mice. (C) Intestinal tissue of mice treated with A. annua ethanolic extract. (D) Intestinal tissue of mice treated with albendazole (positive control). Black arrows point to goblet cells. Magnification, 400x.
Figure 5. Goblet cell numbers in intestinal tissues of different groups of mice. High magnification images (400x) of intestinal tissues stained with PAS and hematoxylin were used to count goblet cells. All infected mice groups (treated and untreated) showed significant high numbers of goblet cells/HPF in comparison to uninfected mice group. Asterisk (*) indicate significant difference, whereas ns, non-significant.
Immunohistochemical staining of the intestinal tissues showed weak expression of TGF-β in the intestinal tissues of uninfected mice (black arrows) (Figure 6A). In contrast, elevated expression of TGF-β was detected in mucosal stromal cells (arrow heads) extending to the submucosal inflammatory cells (black arrows) in infected untreated animals (Figure 6B). Interestingly, albendazole and A. annua-treated mice showed a significantly lower number of positive cells (black arrows) (35.70 and 20.75%, respectively) (Table 1; Figures 6C,D).
Figure 6. A. annua treatment markedly reduced TGF-β expression in intestinal tissues of T. spiralis-infected mice. (A–D) Immunohistochemical staining of TGF-β in intestinal tissues of different mice groups A. Representative image of intestinal tissue of uninfected mice showing weak expression of TGF-β (black arrows). (B) Representative image of intestinal tissue of infected untreated animals showing high expression of TGF-β in submucosal inflammatory cells (black arrows) and mucosal stromal cells (arrow heads). (C) Representative image of intestinal tissue of albendazole-treated animals showing reduction of TGF-β-positive mucosal stromal cells (black arrows). (D)Representative image of intestinal tissue of A. annua-treated mice showing marked reduction in TGF-β -positive mucosal stromal cells (black arrows). Magnification is 200x.
Table 1. Percentage (%) of TGF-β positive cells in the intestinal tissue of different groups of mice.
Trichinellosis is a worldwide parasitic zoonotic disease that is commonly treated with benzimidazole derivatives, including albendazole. Toxicity, adverse effects, low bioavailability, and drug resistance limit the therapeutic utility of those anti-trichinella drugs. Therefore, the development of new and safe alternatives for treatment of trichinellosis is a necessity. Several recent studies have shown that medicinal plants may represent safe and effective alternative therapeutics for treatment of trichinellosis (23, 58–60). In our present study, we aimed to evaluate the efficacy of ethanolic extract of A. annua in treating intestinal trichinellosis in experimentally infected mice.
Our results demonstrated that treatment with A. annua extract significantly reduced the number of adult worms in the small intestine compared to infected untreated animals. Similarly, Abo Rayia et al. (61) reported 75% reduction in T. spiralis adult worm count after treatment of infected mice with artemisinin; the main active constituent of A. annua. Furthermore, Caner et al. (18) reported a good therapeutic effect of A. vulgaris and A. absinthium extracts against the T. spiralis larval stages in muscles.
Of note, several studies have shown that A. annua is also effective against many parasitic diseases such as trypanosomiasis (62), schistosomiasis (62), toxoplasmosis (34), leishmaniasis (34), giardiasis (33), and coccidiosis (63).
Our data also showed that treatment with A. annua ameliorated the T. spiralis induced histopathological changes in infected mice. In infected untreated animals, we observed prominent villous atrophy with marked chronic inflammatory cell infiltrates composed of neutrophils, lymphocytes, and macrophages. The administration of A. annua and albendazole to T. spiralis-infected mice restored the normal villi structure and reduced inflammatory cellular infiltration.
Although our treatments reduced inflammatory cellular infiltration, high eosinophilic infiltration was still recognized in all infected animal groups, including treated animals. We believe that high number of eosinophils in A. annua-treated mice was not caused by the treatment since high eosinophils count was noticed in all infected animals including the infected untreated group. Our speculation is supported by previous studies which showed that A. annua caused a decline in eosinophils count by 27% when used for treatment of schistosomiasis (64), and suppressed infiltration of mast cells and eosinophils into the epidermis (65), when used in treatment of atopic dermatitis. Moreover, infection with T. spiralis has been shown to trigger an increase in eosinophil counts (66). Eosinophils have been reported to play a major role in resistance to parasitic infections, including that of T. spiralis, and to kill newborn larvae (NBL) of this parasite (13). Additionally, IL5-dependent eosinophils promote worm expulsion during T. spiralis infection (67). The role of eosinophils was further confirmed by a study which detected a rich eosinophil infiltrate in the myocardial tissue of one patient who died of severe trichinellosis (68). In contrast, other studies suggested that eosinophils are essential for protection of NBL. Huang et al. suggested that recruitment of eosinophils to the site of infection may protect NBL by stimulating the production of IL-10 from DCs and CD4+ T cells which inhibit the activation of macrophages and neutrophils (the source of NO) and subsequently prevent NO production, thus promoting T. spiralis larval survival (69). This is further supported by the observation that eosinophil-ablated mice cleared T. spiralis adult worms normally (70, 71). Despite all of the above findings, the role of eosinophils in host defense against T. spiralis or promotion of parasite growth and survival remains to be determined.
Our histological data also demonstrated increased goblet cell counts in the intestinal sections of all infected mice groups compared to uninfected mice. It is well documented that goblet cells have a significant role in protection of the intestinal epithelium from intestinal pathogens by secretion of mucins which form a protective viscous barrier against invading organisms including intestinal nematodes (72, 73). Using PAS, we noticed that the infected untreated and treated animal groups have higher numbers of goblet cells, a phenomenon that usually accompanies the invasion of T. spiralis (8, 12, 14, 74, 75). Goblet cells secrete various types of active molecules, including mucin glycoproteins, which are essential for the formation of the mucus overlaying the surface of epithelial cells causing the trapping of the worms and their expulsion from the host (76–78). This has been confirmed by the observation of delayed worm expulsion in Stat6- or IL-4Ra-deficient mice infected with T. spiralis, as those mice were not able to develop goblet cell hyperplasia (12, 79). In addition to mucus, TFF3 (trefoil factor) is produced by goblet cells which helps prevent disturbances in the mucosa and improves regeneration (80).
Several reports have discussed the important role of TGF-β in adult-worm expulsion during intestinal trichinellosis (81–83). Therefore, in the present study, we used immunohistochemical staining to quantify the expression of TGF-β in intestinal tissues of T. spiralis-infected mice. According to our results, the production of TGF-β was increased in infected untreated mice, whereas lower numbers of TGF-β-positive cells were observed in treated animals. Similar findings were reported by previous studies which support the role of TGF-β in worm expulsion (81–84). Akiho et al. reported that gut contraction during T. spiralis infection is driven by Th2 cytokines and TGF-β via STAT6 and COX-2, respectively (85, 86). Moreover, Steel et al. stated that TGF-β is the main player in intestinal contraction and worm expulsion (87). Using mice which lacked the ability to produce TGF-β in experimental intestinal trichinellosis model, they observed that the mice lacked the ability to contract intestinal muscles and flush out the parasite (16, 87).
We showed that A. annua extract eliminated the adult worms from the intestine of T. spiralis-infected mice. Moreover, our extract treatment restored the normal intestinal architecture, villi length, and reduced inflammatory cellular infiltration. Moreover, treated mice showed modest expression of TGF-β, which has been proven to play a major role in worm expulsion. The therapeutic effect of A. annua extract was comparable to albendazole; however, the side effects and the reported resistance to benzimidazole derivatives, including albendazole support further testing of A. annua extract as a promising complementary herbal product for treatment of trichinellosis. More studies are required to investigate the anti-trichinella active components of A. annua and to develop more effective and safer derivatives that can be used in combination to limit the development of resistance.
The original contributions presented in the study are included in the article/supplementary material, further inquiries can be directed to the corresponding author/s.
The animal study was reviewed and approved by Research Ethics Committee of the Faculty of Veterinary Medicine, South Valley University, Egypt (Protocol code 20-4-9-2021).
All authors contributed to the conceptualization, experimental design, data analysis, writing, review, and editing of the manuscript. Additionally, HE and AE-k led the research team, supervised the study, critically analyzed the data and revised the manuscript. All authors contributed to the article and approved the submitted version.
Princess Nourah bint Abdulrahman University Researchers Supporting Project number (PNURSP2022R39), Princess Nourah bint Abdulrahman University, Riyadh, Saudi Arabia, and Researchers Supporting Project (Project number RSP-2021/174), King Saud University, Riyadh, Saudi Arabia.
The authors declare that the research was conducted in the absence of any commercial or financial relationships that could be construed as a potential conflict of interest.
All claims expressed in this article are solely those of the authors and do not necessarily represent those of their affiliated organizations, or those of the publisher, the editors and the reviewers. Any product that may be evaluated in this article, or claim that may be made by its manufacturer, is not guaranteed or endorsed by the publisher.
1. Luis Muñoz-Carrillo J, Maldonado-Tapia C, López- Luna A, Jesús Muñoz-Escobedo J, Armando Flores-De La Torre J, Moreno-García A. Current aspects in Trichinellosis. In: Parasites and Parasitic Diseases 2018 Nov 5. London, UK: IntechOpen (2019). p. 175–216.
2. Ribicich M, Gamble HR, Rosa A, Bolpe J, Franco A. Trichinellosis in Argentina: an historical review. Vet Parasitol. (2005) 132:137–42. doi: 10.1016/j.vetpar.2005.05.042
3. Tada K, Suzuki H, Sato Y, Morishima Y, Nagano I, Ishioka H, et al. Outbreak of Trichinella T9 infections associated with consumption of bear meat, Japan. Emerg Infect Dis. (2018) 24:1532–5. doi: 10.3201/eid2408.172117
4. Murrell KD, Pozio E. Worldwide occurrence and impact of human Trichinellosis, 1986-2009. Emerg Infect Dis. (2011) 17:2194–202. doi: 10.3201/eid1712.110896
5. Barruet R, Devez A, Dupouy-Camet J, Karadjian G, Plavsa D, Chydériotis G, et al. Outbreaks a common source for a Trichinellosis outbreak reported in France and Serbia in 2017. Eurosurveillance. (2020) 25:1–7. doi: 10.2807/1560-7917.ES.2020.25.24.1900527
6. Rózycki M, Korpysa-Dzirba W, Bełcik A, Pelec T, Mazurek J, Cencek T. Analysis of a Trichinellosis outbreak in poland after consumption of sausage made of wild boar meat. J Clin Med. (2022) 11:485. doi: 10.3390/jcm11030485
8. Piekarska J, Miśta D, Houszka M, Króliczewska B, Zawadzki W, Gorczykowski M. Trichinella spiralis: the influence of short chain fatty acids on the proliferation of lymphocytes, the goblet cell count and apoptosis in the mouse intestine. Exp Parasitol. (2011) 128:419–26. doi: 10.1016/j.exppara.2011.05.019
9. Ding J, Bai X, Wang XL, Wang YF, Shi HN, Rosenthal B, et al. Developmental profile of select immune cells in mice infected with Trichinella spiralis during the intestinal phase. Vet Parasitol. (2016) 231:77–82. doi: 10.1016/j.vetpar.2016.07.019
10. Khan WI, Collins SM. Immune-mediated alteration in gut physiology and its role in host defence in nematode infection. Parasite Immunol. (2004) 26:319–26. doi: 10.1111/j.0141-9838.2004.00715.x
11. Knight PA, Brown JK, Pemberton AD. Innate immune response mechanisms in the intestinal epithelium: potential roles for mast cells and goblet cells in the expulsion of adult Trichinella spiralis. Parasitology. (2008) 135:655–70. doi: 10.1017/S0031182008004319
12. Khan WI, Blennerhasset P, Ma C, Matthaei KI, Collins SM. Stat6 dependent goblet cell hyperplasia during intestinal nematode infection. Parasite Immunol. (2001) 23:39–42. doi: 10.1046/j.1365-3024.2001.00353.x
13. Kazura JW, Aikawa M. Host defense mechanisms against Trichinella spiralis infection in the mouse: eosinophil-mediated destruction of newborn larvae in vitro. J Immunol. (1980) 124:355–61.
14. Ishikawa N, Wakelin D, Mahida YR. Role of T helper 2 cells in intestinal goblet cell hyperplasia in mice infected with Trichinella spiralis. Gastroenterology. (1997) 113:542–9. doi: 10.1053/gast.1997.v113.pm9247474
15. Worthington JJ, Klementowicz JE, Rahman S, Czajkowska BI, Smedley C, Waldmann H, et al. Loss of the TGFβ-activating integrin αvβ8 on dendritic cells protects mice from chronic intestinal parasitic infection via control of type 2 immunity. PLoS Pathog. (2013) 9:e1003675. doi: 10.1371/journal.ppat.1003675
16. Ding J, Bai X, Wang X, Shi H, Cai X, Luo X, et al. Immune cell responses and cytokine profile in intestines of mice infected with Trichinella spiralis. Front Microbiol. (2017) 8:2069. doi: 10.3389/fmicb.2017.02069
17. Gottstein B, Pozio E, Nöckler K. Epidemiology, diagnosis, treatment, and control of Trichinellosis. Clin Microbiol Rev. (2009) 22:127–45. doi: 10.1128/CMR.00026-08
18. Caner A, Döşkaya M, Degirmenci A, Can H, Baykan S, Uner A, et al. Comparison of the effects of Artemisia vulgaris and Artemisia absinthium growing in western Anatolia against trichinellosis (Trichinella spiralis) in rats. Exp Parasitol. (2008) 119:173–9. doi: 10.1016/j.exppara.2008.01.012
19. Prichard RK. Markers for benzimidazole resistance in human parasitic nematodes? Parasitology. (2007) 134:1087–92. doi: 10.1017/S003118200700008X
20. Piloiu C, Dumitrascu DL. Albendazole-induced liver injury. Am J Ther. (2021) 28:e335–40. doi: 10.1097/MJT.0000000000001341
21. Hogan J, Dehoux L, Niel O, Elenga N, Deschênes G, Dauger S. Hemolytic anemia and irreversible kidney and brain injuries after accidental intravenous injection of albendazole suspension in an infant. Clin Toxicol. (2016) 54:72–3. doi: 10.3109/15563650.2015.1114626
22. Dayan AD. Albendazole, mebendazole and praziquantel. Review of non-clinical toxicity and pharmacokinetics. Acta Trop. (2003) 86:141–59. doi: 10.1016/S0001-706X(03)00031-7
23. Shalaby MA, Moghazy FM, Shalaby HA, Nasr SM. Effect of methanolic extract of Balanites aegyptiaca fruits on enteral and parenteral stages of Trichinella spiralis in rats. Parasitol Res. (2010) 107:17–25. doi: 10.1007/s00436-010-1827-9
24. Basyoni MMA, El-Sabaa A-AA. Therapeutic potential of myrrh and ivermectin against experimental Trichinella spiralis infection in mice. Korean J Parasitol. (2013) 51:297–304. doi: 10.3347/kjp.2013.51.3.297
25. Abd-Elrahman SM, Dyab AK, Mahmoud AE, Mostafa SM, Elossily NA. Antiparasitic activity of myrrh crude extract and myrrh volatile oil compared to albendazole against Trichinella spiralis muscular larvae in vitro. J Egypt Soc Parasitol. (2020) 50:307–14. doi: 10.21608/jesp.2020.113052
26. Efferth T. From ancient herb to modern drug: Artemisia annua and artemisinin for cancer therapy. Semin Cancer Biol. (2017) 46:65–83. doi: 10.1016/j.semcancer.2017.02.009
27. Elfawal MA, Towler MJ, Reich NG, Golenbock D, Weathers PJ, Rich SM. Dried whole plant Artemisia annua as an antimalarial therapy. PLoS ONE. (2012) 7:e52746. doi: 10.1371/journal.pone.0052746
28. Towler MJ, Weathers PJ. Variations in key artemisinic and other metabolites throughout plant development in Artemisia annua L. for potential therapeutic use. Ind Crops Prod. (2015) 67:185–91. doi: 10.1016/j.indcrop.2015.01.007
29. Li K-M, Dong X, Ma Y-N, Wu Z-H, Yan Y-M, Cheng Y-X. Antifungal coumarins and lignans from Artemisia annua. Fitoterapia. (2019) 134:323–8. doi: 10.1016/j.fitote.2019.02.022
30. Bilia AR, Santomauro F, Sacco C, Bergonzi MC, Donato R. Essential oil of Artemisia annua L.: An extraordinary component with numerous antimicrobial properties. Evid Based Complement Alternat Med. (2014) 2014:159819. doi: 10.1155/2014/159819
31. Gupta P, Dutta B, Pant D, Joshi P, Lohar D. In vitro antibacterial activity of Artemisia annua linn. Growing in India. Int J Green Pharm. (2009) 3:255–58. doi: 10.22377/ijgp.v3i3.96
32. Viuda-Martos M, El Gendy AE-NGS, Sendra E, Fernández-López J, Abd El Razik KA, Omer EA, et al. Chemical composition and antioxidant and anti-Listeria activities of essential oils obtained from some Egyptian plants. J Agric Food Chem. (2010) 58:9063–70. doi: 10.1021/jf101620c
33. Abd-Elhamid TH, Abdel-Rahman IAM, Mahmoud AR, Allemailem KS, Almatroudi A, Fouad SS, et al. A complementary herbal product for controlling giardiasis. Antibiotics. (2021) 10: 477. doi: 10.3390/antibiotics10050477
34. de Oliveira TC, Silva DA, Rostkowska C, Bela SR, Ferro EA, Magalhaes PM, et al. Toxoplasma gondii: effects of Artemisia annua L. on susceptibility to infection in experimental models in vitro and in vivo. Exp Parasitol. (2009) 122:233–41. doi: 10.1016/j.exppara.2009.04.010
35. Islamuddin M, Chouhan G, Tyagi M, Abdin MZ, Sahal D, Afrin F. Leishmanicidal activities of Artemisia annua leaf essential oil against Visceral Leishmaniasis. Front Microbiol. (2014) 5:626. doi: 10.3389/fmicb.2014.00626
36. Hwang D. Il, Won K-J, Kim D, Yoon SW, Park J-H, Kim B, Lee HM. Anti-adipocyte differentiation activity and chemical composition of essential oil from Artemisia annua. Nat Prod Commun. (2016) 11:539–42. doi: 10.1177/1934578X1601100430
37. Marinas IC, Oprea E, Chifiriuc MC, Badea IA, Buleandra M, Lazar V. Chemical composition and antipathogenic activity of Artemisia annua essential oil from Romania. Chem Biodivers. (2015) 12:1554–64. doi: 10.1002/cbdv.201400340
38. Brisibe EA, Umoren UE, Brisibe F, Magalhäes PM, Ferreira JFS, Luthria D, et al. Nutritional characterisation and antioxidant capacity of different tissues of Artemisia annua L. Food Chem. (2009) 115:1240–6. doi: 10.1016/j.foodchem.2009.01.033
39. Carbonara T, Pascale R, Argentieri MP, Papadia P, Fanizzi FP, Villanova L, et al. Phytochemical analysis of a herbal tea from Artemisia annua L. J Pharm Biomed Anal. (2012) 62:79–86. doi: 10.1016/j.jpba.2012.01.015
40. Dong Y, Liu L, Han J, Zhang L, Wang Y, Li J, et al. Worldwide research trends on artemisinin: a bibliometric analysis from 2000 to 2021. Front Med. (2022) 9:868087. doi: 10.3389/fmed.2022.868087
41. Willcox ML, Bodeker G. Traditional herbal medicines for malaria. BMJ. (2004) 329:1156–9. doi: 10.1136/bmj.329.7475.1156
42. Abate G, Zhang L, Pucci M, Morbini G, Mac Sweeney E, Maccarinelli G, et al. Phytochemical analysis and anti-inflammatory activity of different ethanolic phyto-extracts of Artemisia annua L. Biomolecules. (2021) 11:975. doi: 10.3390/biom11070975
43. Mohammed S, Dekabo A, Hailu T. Phytochemical analysis and anti-microbial activities of Artemisia spp. and rapid isolation methods of artemisinin. AMB Expr. (2022) 12:17. doi: 10.1186/s13568-022-01346-5
44. Tariq KA, Chishti MZ, Ahmad F, Shawl AS. Anthelmintic activity of extracts of Artemisia absinthium against ovine nematodes. Vet Parasitol. (2009) 160:83–8. doi: 10.1016/j.vetpar.2008.10.084
45. Kim MH, Seo JY, Liu KH, Kim JS. Protective effect of Artemisia annua L. extract against galactose-induced oxidative stress in mice. PLoS ONE. (2014) 9:3–9. doi: 10.1371/journal.pone.0101486
46. Alara OR, Abdurahman NH, Olalere OA. Ethanolic extraction of flavonoids, phenolics and antioxidants from Vernonia amygdalina leaf using two-level factorial design. J King Saud Univ - Sci. (2020) 32:7–16. doi: 10.1016/j.jksus.2017.08.001
47. Manzoor F, Nasim G, Saif S, Asma Malik S. Effect of ethanolic plant extracts on three storage grain pests of economic importance. Pakistan J Bot. (2011) 43:2941–6.
48. Gamble HR. Detection of Trichinellosis in pigs by artificial digestion and enzyme immunoassay. J Food Prot. (1996) 59:295–8. doi: 10.4315/0362-028X-59.3.295
49. Attia RAH, Mahmoud AE, Farrag HMM, Makboul R, Mohamed ME, Ibraheim Z. Effect of myrrh and thyme on Trichinella spiralis enteral and parenteral phases with inducible nitric oxide expression in mice. Mem Inst Oswaldo Cruz. (2015) 110:1035–41. doi: 10.1590/0074-02760150295
50. Shoheib Z, Abdin A, Shamloula M. El–Segaey O. Role Of α-chymotrypsin and colchicine as adjuvant therapy in experimental muscular Trichinellosis: parasitological, biochemical & immunohistochemical study. Egypt J Bacteriol. (2006) 15:773–90.
51. Salama MAM, Mostafa NE, Abd El-Aal NF, Moawad HSF, Hammad SK, Adel R, et al. Efficacy of Zingiber officinale and Cinnamomum zeylanicum extracts against experimental Trichinella spiralis infection. J Parasit Dis. (2022) 46:24–36. doi: 10.1007/s12639-021-01412-y
52. Denham DA. Studies with methyridine1 1 2-(β-methoxyethyl) pyridine. ICI “Promintic” and Trichinella spiralis I Effect upon the intestinal phase in mice. Exp Parasitol. (1965) 17:10–4. doi: 10.1016/0014-4894(65)90003-2
53. Sadaow L, Intapan PM, Boonmars T, Morakote N, Maleewong W. Susceptibility of laboratory rodents to Trichinella papuae. Korean J Parasitol. (2013) 51:629–32. doi: 10.3347/kjp.2013.51.6.629
54. Nashaat EN, Amira FA. Worm and larval burden, histopathological and ultrastructural evaluation of T spiralis vaccination using crude worms and/or larvae antigens: experimental studies. (2010).
55. Carleton HM, Drury RA, Wallington EA. Carleton's Histological Technique. Oxford, NY: Oxford University Press (1980).
56. Hwang H. Bin, Kim HS. Phototoxic effects of an operating microscope on the ocular surface and tear film. Cornea. (2014) 33:82–90. doi: 10.1097/ICO.0000000000000001
57. Schneider CA, Rasband WS, Eliceiri KW. NIH Image to imageJ: 25 years of image analysis. Nat Methods. (2012) 9:671–5. doi: 10.1038/nmeth.2089
58. García D, Escalante M, Delgado R, Ubeira FM, Leiro J. Anthelminthic and antiallergic activities of Mangifera indica L. stem bark components Vimang and mangiferin. Phytother Res. (2003) 17:1203–8. doi: 10.1002/ptr.1343
59. Bany J, Zdanowska D, Zdanowski R, Skopińska-Rózewska E. The effect of herbal remedy on the development of Trichinella spiralis infection in mice. Pol J Vet Sci. (2003) 6:6–8.
60. Abu El Ezz NMT. Effect of Nigella sativa and Allium cepa oils on Trichinella spiralis in experimentally infected rats. J Egypt Soc Parasitol. (2005) 35:511–23.
61. Abou Rayia DM, Saad AE, Ashour DS, Oreiby RM. Implication of artemisinin nematocidal activity on experimental trichinellosis: In vitro and in vivo studies. Parasitol Int. (2017) 66:56–63. doi: 10.1016/j.parint.2016.11.012
62. Mishina YV, Krishna S, Haynes RK, Meade JC. Artemisinins inhibit Trypanosoma cruzi and Trypanosoma brucei rhodesiense in vitro growth. Antimicrob Agents Chemother. (2007) 51:1852–4. doi: 10.1128/AAC.01544-06
63. Jiao J, Yang Y, Liu M, Li J, Cui Y, Yin S, et al. Artemisinin and Artemisia annua leaves alleviate Eimeria tenella infection by facilitating apoptosis of host cells and suppressing inflammatory response. Vet Parasitol. (2018) 254:172–7. doi: 10.1016/j.vetpar.2018.03.017
64. Munyangi J, Cornet-Vernet L, Idumbo M, Lu C, Lutgen P, Perronne C, et al. Effect of Artemisia annua and Artemisia afra tea infusions on schistosomiasis in a large clinical trial. Phytomedicine. (2018) 51:233–40. doi: 10.1016/j.phymed.2018.10.014
65. Han X, Chen Z, Yuan J, Wang G, Han X, Wu H, et al. Artemisia annua water extract attenuates DNCB-induced atopic dermatitis by restraining Th2 cell mediated inflammatory responses in BALB/c mice. J Ethnopharmacol. (2022) 291:115160. doi: 10.1016/j.jep.2022.115160
66. Herndon FJ, Kayes SG. Depletion of eosinophils by anti-IL-5 monoclonal antibody treatment of mice infected with Trichinella spiralis does not alter parasite burden or immunologic resistance to reinfection. J Immunol. (1992) 149:3642–7.
67. Vallance BA, Blennerhassett PA, Deng Y, Matthaei KI, Young IG, Collins SM. IL-5 contributes to worm expulsion and muscle hypercontractility in a primary T. spiralis infection. Am J Physiol. (1999) 277:G400–8. doi: 10.1152/ajpgi.1999.277.2.G400
68. Bruschi F, Korenaga M, Watanabe N. Eosinophils and Trichinella infection: toxic for the parasite and the host? Trends Parasitol. (2008) 24:462–7. doi: 10.1016/j.pt.2008.07.001
69. Huang L, Gebreselassie NG, Gagliardo LF, Ruyechan MC, Lee NA, Lee JJ, et al. Eosinophil-derived IL-10 supports chronic nematode infection. J Immunol. (2014) 193:4178–87. doi: 10.4049/jimmunol.1400852
70. Gebreselassie NG, Moorhead AR, Fabre V, Gagliardo LF, Lee NA, Lee JJ, et al. Eosinophils preserve parasitic nematode larvae by regulating local immunity. J Immunol. (2012) 188:417–25. doi: 10.4049/jimmunol.1101980
71. Fabre V, Beiting DP, Bliss SK, Gebreselassie NG, Gagliardo LF, Lee NA, et al. Eosinophil deficiency compromises parasite survival in chronic nematode infection. J Immunol. (2009) 182:1577–83. doi: 10.4049/jimmunol.182.3.1577
72. Birchenough GM, Johansson ME, Gustafsson JK, Bergstrom JH, Hansson GC. New developments in goblet cell mucus secretion and function. Mucosal Immunol. (2015) 8:712–9. doi: 10.1038/mi.2015.32
73. Deplancke B, Gaskins HR. Microbial modulation of innate defense: goblet cells and the intestinal mucus layer. Am J Clin Nutr. (2001) 73:1131S–41S. doi: 10.1093/ajcn/73.6.1131S
74. Sagar M, Padol I, Khan WI, Bonin RP, Blennerhassett PA, Hunt RH. Establishment of T-Helper-2 immune response based gerbil model of enteric infection. Scand J Gastroenterol. (2004) 39:668–73. doi: 10.1080/00365520410005315
75. Chen Y, Huang B, Huang S, Yu X, Li Y, Song W, et al. Coinfection with Clonorchis sinensis modulates murine host response against Trichinella spiralis infection. Parasitol Res. (2013) 112:3167–79. doi: 10.1007/s00436-013-3493-1
76. Miller HR, Huntley JF, Wallace GR. Immune exclusion and mucus trapping during the rapid expulsion of Nippostrongylus brasiliensis from primed rats. Immunology. (1981) 44:419.
77. Newlands GF, Gibson S, Knox DP, Grencis R, Wakelin D, Miller HR. Characterization and mast cell origin of a chymotrypsin-like proteinase isolated from intestines of mice infected with Trichinella spiralis. Immunology. (1987) 62:629.
78. Shekels LL, Anway RE, Lin J, Kennedy MW, Garside P, Lawrence CE, et al. Coordinated Muc2 and Muc3 mucin gene expression in Trichinella spiralis infection in wild-type and cytokine-deficient mice. Dig Dis Sci. (2001) 46:1757–64. doi: 10.1023/A:1010622125040
79. Horsnell WGC, Cutler AJ, Hoving JC, Mearns H, Myburgh E, Arendse B, et al. Delayed goblet cell hyperplasia, acetylcholine receptor expression, and worm expulsion in SMC-specific IL-4Ralpha-deficient mice. PLoS Pathog. (2007) 3:e1. doi: 10.1371/journal.ppat.0030001
80. Dehlawi MS, Mahida YR, Hughes K, Wakelin D. Effects of Trichinella spiralis infection on intestinal pathology in mice lacking interleukin-4 (IL-4) or intestinal trefoil factor (ITF/TFF3). Parasitol Int. (2006) 55:207–11. doi: 10.1016/j.parint.2006.05.002
81. Gawaziuk JP. X, Sheikh F, Cheng Z-Q, Cattini PA, Stephens NL. Transforming growth factor-beta as a differentiating factor for cultured smooth muscle cells. Eur Respir J. (2007) 30:643–52. doi: 10.1183/09031936.00141805
82. Lee BS, Nowak RA. Human leiomyoma smooth muscle cells show increased expression of transforming growth factor-beta 3 (TGF beta 3) and altered responses to the antiproliferative effects of TGF beta. J Clin Endocrinol Metab. (2001) 86:913–20. doi: 10.1210/jc.86.2.913
83. Moore-Olufemi SD, Olsen AB, Hook-Dufresne DM, Bandla V, Cox CSJ. Transforming growth factor-beta 3 alters intestinal smooth muscle function: implications for gastroschisis-related intestinal dysfunction. Dig Dis Sci. (2015) 60:1206–14. doi: 10.1007/s10620-014-3439-1
84. Shao J, Xia Z, Li Y, Yu S, Deng W. [Expression of cellular phenotype switching markers-matrix protein Gla, mRNA and collagen I, III and V of human airway smooth muscle cells in vitro after TGF-beta1 stimulation]. Chin J Pediatr. (2006) 44:531–4.
85. Akiho H, Deng Y, Blennerhassett P, Kanbayashi H, Collins SM. Mechanisms underlying the maintenance of muscle hypercontractility in a model of postinfective gut dysfunction. Gastroenterology. (2005) 129:131–41. doi: 10.1053/j.gastro.2005.03.049
86. Akiho H, Blennerhassett P, Deng Y, Collins SM. Role of IL-4, IL-13, and STAT6 in inflammation-induced hypercontractility of murine smooth muscle cells. Am J Physiol Gastrointest Liver Physiol. (2002) 282:G226–32. doi: 10.1152/ajpgi.2002.282.2.G226
87. Steel N, Faniyi AA, Rahman S, Swietlik S, Czajkowska BI, Chan BT, et al. TGFβ-activation by dendritic cells drives Th17 induction and intestinal contractility and augments the expulsion of the parasite Trichinella spiralis in mice. PLoS Pathog. (2019) 15:e1007657. doi: 10.1371/journal.ppat.1007657
Keywords: Artemisia annua, T. spiralis, goblet cells, TGF-β, trichinellosis
Citation: El-kady AM, Abdel-Rahman IAM, Sayed E, Wakid MH, Alobaid HM, Mohamed K, Alshehri EA, Elshazly H, Al-Megrin WAI, Iqbal F, Elshabrawy HA and Timsah AG (2022) A potential herbal therapeutic for trichinellosis. Front. Vet. Sci. 9:970327. doi: 10.3389/fvets.2022.970327
Received: 15 June 2022; Accepted: 29 July 2022;
Published: 23 August 2022.
Edited by:
Ilias Giannenas, Aristotle University of Thessaloniki, GreeceReviewed by:
Xiaolei Liu, Jilin University, ChinaCopyright © 2022 El-kady, Abdel-Rahman, Sayed, Wakid, Alobaid, Mohamed, Alshehri, Elshazly, Al-Megrin, Iqbal, Elshabrawy and Timsah. This is an open-access article distributed under the terms of the Creative Commons Attribution License (CC BY). The use, distribution or reproduction in other forums is permitted, provided the original author(s) and the copyright owner(s) are credited and that the original publication in this journal is cited, in accordance with accepted academic practice. No use, distribution or reproduction is permitted which does not comply with these terms.
*Correspondence: Asmaa M. El-kady, YXNtYWEuZWxrYWR5QG1lZC5zdnUuZWR1LmVn; Hatem A. Elshabrawy, aGF0ZW0uZWxzaGFicmF3eUBzaHN1LmVkdQ==
Disclaimer: All claims expressed in this article are solely those of the authors and do not necessarily represent those of their affiliated organizations, or those of the publisher, the editors and the reviewers. Any product that may be evaluated in this article or claim that may be made by its manufacturer is not guaranteed or endorsed by the publisher.
Research integrity at Frontiers
Learn more about the work of our research integrity team to safeguard the quality of each article we publish.