- 1Departamento de Patología y Medicina Preventiva, Facultad de Ciencias Veterinarias, Universidad de Concepción, Chillán, Chile
- 2Centro de Espectroscopía y Microscopía Electrónica, Universidad de Concepción, Concepción, Chile
- 3Departamento de Biología Célular, Facultad de Ciencias Biológicas, Centro de Microscopía Avanzada, Universidad de Concepción, Concepción, Chile
- 4Hospital Dr. Víctor Ríos, Servicio de Salud Bío Bío, Los Ángeles, Chile
- 5Departamento de Ciencia Animal, Facultad de Ciencias Veterinarias, Universidad de Concepción, Chillán, Chile
Biofilms in milking equipment on dairy farms have been associated with failures in cleaning and sanitizing protocols. These biofilms on milking equipment can be a source of contamination for bulk tank milk and a concern for animal and public health, as biofilms can become on-farm reservoirs for pathogenic bacteria that cause disease in cows and humans. This report describes a cross-sectional study on 3 dairy farms, where hoses used to divert waste milk, transition milk, and colostrum were analyzed by culture methods and matrix-assisted laser desorption/ionization time-of-flight mass spectrometry (MALDI-TOF MS) to assess the presence of pathogenic bacteria such as Staphylococcus aureus, Pseudomonas aeruginosa, and Klebsiella spp. In addition, the presence of biofilms was analyzed using scanning electron microscopy and confocal spectral microscopy. Biofilms composed of multispecies microbial communities were observed on the surfaces of all milk hoses. In two dairy farms, S. aureus, P. aeruginosa, Klebsiella pneumoniae, and Klebsiella oxytoca were isolated from the milk hose samples collected. Cleaning and sanitation protocols of all surfaces in contact with milk or colostrum are crucial. Hoses used to collect waste milk, colostrum, and transition milk can be a source of biofilms and hence pathogenic bacteria. Waste milk used to feed calves can constitute a biosecurity issue and a source of pathogens, therefore an increased exposure and threat for the whole herd health and, potentially, for human health.
Introduction
Biofilms are microbial communities attached to surfaces by means of an exopolymeric matrix (1). This matrix is made of several substances, including polysaccharides, proteins, DNA, and other matrix-entrapped environmental substrates (2, 3). The presence of biofilms has been previously documented on dairy farms, including on-farm cooling systems (4), water troughs (5), milk tank, and milking equipment (6–9).
Several microorganisms known for their ability to form biofilms, such as Pseudomonas spp., Staphylococcus aureus, Listeria monocytogenes strains, Bacillus strains, and Klebsiella spp. have often been found on dairy farms or in bulk tank milk (10–15). In addition, pathogenic microorganisms such as S. aureus have the ability to adhere to materials that are often used for the manufacture of milking equipment parts, such as stainless steel and rubber (9, 16, 17). Microorganisms such as Pseudomonas spp., which can have increased ability to produce exopolymeric matrix (18), have also been reported on dairy farms in places such as teat cups, drains, water, and sand used for bedding (19, 20). Microorganisms in biofilms usually live within microbial communities formed by several species (7, 21), where the detachment of bacteria to the environment can be used as a dispersal strategy (21). In the case of biofilms on milking equipment surfaces, because bacteria can be dislodged by the milk flow during milking (6, 17) the biofilm's microbiota can be transported to other locations within the milking system or the milk tank. These events are relevant to milk quality and food hygiene, as bacteria from biofilms in milking equipment can not only cause contamination of bulk tank milk (7, 9, 17), but also colonize and persist for long periods of time within the milking system (15). A recent study (9) also shows that biofilms in rubber liners on milking machine claws can be a source of S. aureus for the udder, thus posing a risk of intramammary infections due to the close contact of biofilm-fouled liners with the teat during milking.
In dairy operations around the world, colostrum and transition milk, milk from cows with clinical mastitis, cows undergoing antibiotic or anti-inflammatory treatments, or intramammary-infected high somatic-cell-count cows are usually diverted from the bulk tank, as required by law or by the milk processors. Likewise, in many places of the world, it is a common practice to segregate clinical mastitis and treatment cows, as well as fresh cows, to milk them at the end of the milking routine. Thus, their milk, colostrum, or transition milk is collected using milk cans. Standard cleaning and sanitation protocols for these milk cans, hoses, and other parts of milking equipment used to harvest waste milk/colostrum are, however, often neglected. The presence of milk and colostrum residues in poorly cleaned or non-cleaned milking equipment provides not only a source of nutrients for the proliferation of microorganisms (22), but also a “conditioning film” (23) for surfaces. These factors can create ideal conditions for bacteria colonization and the subsequent biofilm formation in such areas of the milking equipment, where cleaning and sanitation protocols may not have been properly conducted. If formed, these overlooked biofilms may pose a threat for both animal and public health, as they can become on-farm reservoirs for pathogenic bacteria known to cause disease in cows and humans (7, 9).
This report describes the findings on 3 Chilean dairy farms, where pieces of milking equipment used to collect waste milk, transition milk, and colostrum were removed and analyzed to assess the presence of biofilms and pathogenic bacteria such as S. aureus, P. aeruginosa, and Klebsiella spp. These three organisms were sought due to both their putative high potential to form biofilms (24–29) and their documented importance in animal and human health. To our knowledge, this is the first report assessing the role of biofilms in hoses used for waste milk, and the relevance of neglecting parts of milking equipment used for purposes other than collecting bulk tank milk.
Methods
Study farms
A cross-sectional study was conducted on three dairy farms of the Ñuble Region of Chile. The three farms, namely Farm A, Farm B, and Farm C had 74, 170, and 46 lactating cows, respectively. Farm A had a year-round grazing system, whereas Farms B and C had a free-stall seasonal confinement system. All farms had automated milking equipment and a bulk milk tank for cooling and storage of salable milk.
Washing and sanitation procedures for the milking equipment used for harvesting salable milk in Farm A consisted in a disinfection step before milking using peracetic acid, a main wash using an alkaline dairy detergent, and a final rinse using peracetic acid. On the other hand, Farm B used a disinfection step before milking with a chlorine disinfectant, and a main wash with an alkaline dairy detergent. Farm C did not use any disinfectant before milking, but a main wash with an alkaline detergent and then disinfection of milking equipment using a chlorinated dairy product. Both Farms B and C used an acid-rinse of milking equipment once per week.
The cows in Farms A, B, and C were milked twice a day. Milk collected from cows undergoing antibiotic treatments, diagnosed with clinical mastitis, or producing transition milk or colostrum was diverted, using the milking system vacuum and the individual milking units with attached milk tubes (milk hoses) connected to a milk can, to divert the waste milk away from the bulk tank.
Microbiology and microscopy analysis
Milk hoses used to collect waste milk, transition milk, and colostrum on Farms A, B, and C were visually inspected to assess the presence of macroscopic adherences or films (i.e., visible films or adherences attached to surfaces) using a ~10.000 lx flashlight (9). The inner part of milk hoses showing macroscopic adherences was swabbed using sterile cotton swabs moistened with Neutralizing Buffer (Difco; BD Diagnostics, Sparks, MD) for microbiology analysis (6) at the Milk and Dairy Safety Laboratory, College of Veterinary Sciences, Universidad de Concepción, Chile. After swab sampling, the milk hoses were removed, replaced with new ones, and transported in a cooler with ice packs to the laboratory, where they were aseptically cut and processed for Scanning Electron Microscopy (SEM) and Confocal Spectral Microscopy (CSM) at the Spectroscopy and Electronic Microscopy Center (CESMI) and at the Advanced Microscopy Center (CMA Biobío) of the Universidad de Concepción, Chile, respectively.
Upon arrival to the Milk and Dairy Safety Laboratory, all swab samples were analyzed for the presence of S. aureus, P. aeruginosa and Klebsiella spp. For this purpose, swab samples were enriched in 10 mL Brain Heart Infusion broth for 48 h at 37°C (7, 9). After enrichment, 10 μL aliquots were streaked onto CHROMagar™ Staph. aureus (CHROMagar, France) (9), Cetrimide Agar (Merck, Darmstadt, Germany), and onto MacConkey agar (Oxoid, Basingstoke, UK) supplemented with 10 mg/L of ampicillin (11), for the isolation of S. aureus, P. aeruginosa, and Klebsiella spp., respectively. Putative S. aureus colonies (i.e., pink to mauve colonies), P. aeruginosa (i.e., yellow-green fluorescent colonies), and Klebsiella spp colonies (i.e., pink, dome-shaped mucoid colonies) were identified and transferred to a new plate for purification and storage at −80°C in Microbank Beads (Pro-Lab Diagnostics, Round Rock, TX) for further analysis. Subsequent confirmation of bacterial species was done by matrix-assisted laser desorption/ionization time-of-flight mass spectrometry (MALDI-TOF MS) MALDI Biotyper (Bruker Daltonics GmbH, Bremen, Germany) at Dr. Víctor Ríos Hospital in Los Angeles, Chile.
For microscopy analysis, the removed hoses were aseptically cut using scissors, as described by Latorre et al. (7). Staining and fixation of samples for SEM were done following the protocol described by Latorre et al. (9). Briefly, after staining, samples were put in 2.5% glutaraldehyde, washed 3 times with 1X Phosphate Buffered Saline (PBS) at room temperature, and then gradually dehydrated using graded series of ethanol. Samples were then stored at 4°C overnight and transported to CESMI for SEM analysis.
Samples prepared for CSM were also aseptically cut as described earlier, and then they were immersed in 4% paraformaldehyde at 4°C overnight for fixation before transportation to CMA for analysis. For CSM analysis, pieces of the hoses were incubated in the dark for 2 min in a Propidium Iodide (Sigma Aldrich, Darmstadt, Germany) solution prepared with PBS (1:500). After incubation, samples were washed 3 times with Phosphate-Buffered Saline and then mounted in a specimen support for scanning of the concave surface of the hoses where the adherences were attached. Images were obtained using a Confocal Spectral Microscope LSM 780 (Zeiss, Oberkochen, Germany) using a spectral scan at 405, 488, 543, and 633 nm with an objective Plan-Apochromat 40x.
Propidium Iodide staining was analyzed exciting the samples using 543 nm and scanning the Z-axis of the adherences attached to the hoses. In addition, a white-colored adherence in the milk hose sample collected from Farm A was analyzed using Nomarski microscopy and fluorescence was detected at 405 and 488 nm with an objective Plan-Apochromat 20x on a single confocal Z plane. Image reconstruction for all samples was done using the software Zen Lite (Zeiss, Oberkochen, Germany).
Results
Both thick and thinner adherences were observed on the surfaces of all three milk hoses. All adherences were confirmed as microbial biofilms by microscopy analysis (Figure 1). Biofilms were composed of multispecies microbial communities, where associations between bacteria and other microorganisms within the biofilms were often observed. By SEM, the presence of cocci, rod-shaped bacteria, yeasts, and molds were observed on the samples (Figures 1A,B,D). In addition, exopolymeric matrixes, both in lax and compact shapes, were observed surrounding or anchoring the microbiota to the surface of the hoses. Biofilms on the surfaces of milk hoses from Farm A and B were abundant and fully covered the surface of the samples analyzed by SEM microscopy (Figures 1A–D); while on the milk hose collected from Farm C, the biofilms were present in a sparser fashion, resembling “patches” of compact multispecies biofilms (Figure 1E). In Farm A, differences were observed within the biofilm structure, where in areas closer to the surface (where milk circulates and a more expedite supply of nutrients and water exists) a greater abundance of yeasts and molds was observed, as compared to deeper areas of the biofilm where a predominance of bacteria was noted.
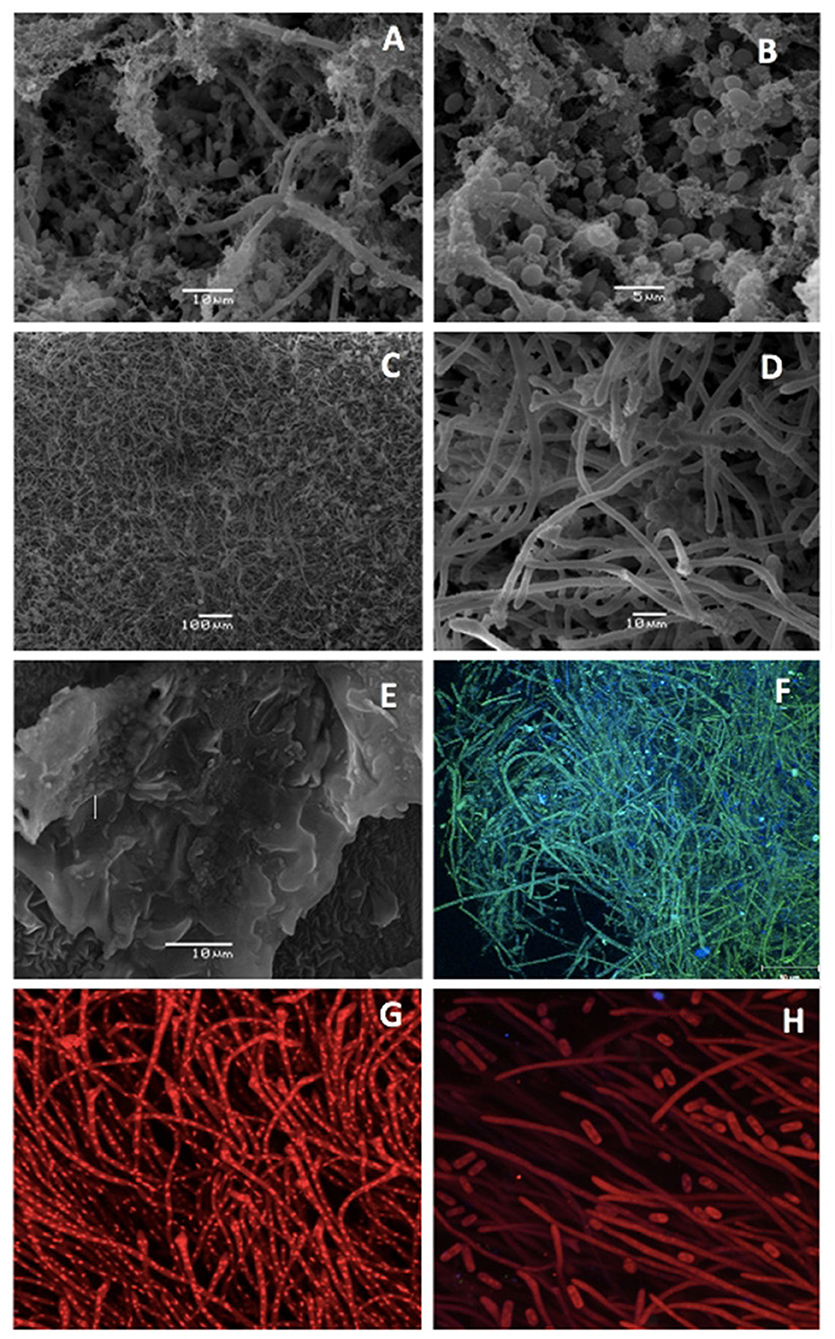
Figure 1. Scanning Electron Microscopy (A–E) and Confocal Spectral Microscopy (F–H) images of biofilms on the surface of milk hoses used to divert milk on three dairy farms: Farm A (A,B,F), Farm B (C,D,G,H), and Farm C (E). Panel (F) shows a multispecies biofilm analyzed using Nomarsky microscopy (405/488 nm) and Panels (F,G) show a biofilm stained with Propidium Iodide (543 nm).
By CSM, on the milk hose sample from Farm A, it was possible to observe biofilms composed of long-green filamentous structures, as well as numerous blue structures of 1–2 μm. The filamentous structures may either correspond to mold hyphae or associations of numerous rod-shaped bacteria, while the blue structures correspond to bacteria (Figure 1F). In addition, biofilms positive to propidium iodide staining (Figures 1G,H) were observed on Farm B. Long filamentous, as well as smaller structures, both positive to the DNA markers used on these samples, were observed on this milk hose. Confocal Spectral Microscopy for the milk hose removed from farm C was not available due to malfunction of the microscope at the time of analyzing this sample.
In the microbiology analysis, combinations of S. aureus, P. aeruginosa, K. pneumoniae, and K. oxytoca were isolated from milk hose samples collected from Farms A and B (Table 1). From the milk hose sample collected from Farm C, none of the targeted bacteria were isolated, although bacterial growth was observed during the Brain Heart Infusion enrichment step.
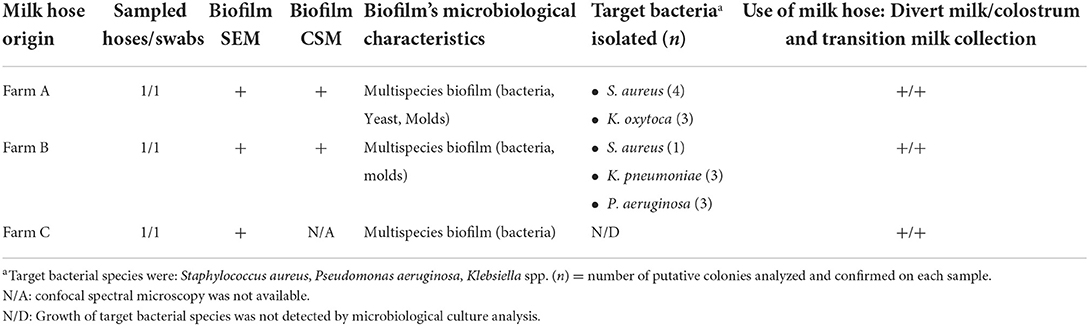
Table 1. Characteristics of biofilms on milk hoses utilized to divert milk and colostrum on three Chilean dairy farms, evaluated by scanning electron microscopy (SEM), confocal spectral microscopy (CSM) analyses, and microbiological cultures.
Discussion
In Chile, as in many parts of the world, diverting transition milk and colostrum, or milk from cows that are suffering from clinical mastitis or undergoing antibiotic treatments, are common practices. As milk or colostrum deflected from the bulk tank is not sold to processors, the cleaning and sanitation procedures of the milking equipment used to collect this milk, are usually not part of the standard cleaning and sanitation procedures. On the farms analyzed in this study, waste milk, transition milk, and colostrum were collected using milk cans and a milk hose used only for this purpose. These milk cans for colostrum and waste milk (and their respective hoses) were not part of the standard cleaning and sanitation protocol in place for the milking equipment used to harvest salable milk on Farms A, B, and C.
Neglecting cleaning and sanitation protocols or inadequate washing of milking equipment, caused by insufficient temperature, chemical, and physical conditions (30) or even deficiencies in the flow of wash water (16), can cause the presence of milk residues in surfaces. These milk residues may aid the attachment of microorganisms acting as a conditioning film (23). These factors create ideal conditions for bacteria colonization and subsequent biofilm formation. The presence of biofilms in these milk hoses is relevant to cows and herd health because bacteria or other microorganisms can be sloughed from these biofilms as milk or colostrum passes through the hoses during milking, and biofilm microbiota can become part of the collected milk or colostrum. This waste milk, transition milk, and colostrum are often used to feed calves, exposing them to potentially harmful or pathogenic bacteria. For example, S. aureus was isolated from two of the three hoses which may have caused S. aureus exposure for calves fed this unpasteurized milk. Similarly, Klebsiella spp. were isolated from these same hoses, which may expose calves to this pathogen, which has been involved in respiratory infections (31–34) and meningoencephalitis (35) in bovines. Moreover, the third detected species, Pseudomonas spp., have also been implicated in respiratory (36), urinary tract (37), and otitis (38) in calves. Other microorganisms of importance for calves' health were not studied as they were beyond the scope of this research. Nevertheless, we cannot rule out the presence of other pathogens contained in the biofilms on these farms. Particularly, Farm A often reported morbidity and mortality of calves due to diarrhea and pneumonia. Although, these problems may have been triggered by other on-farm management practices, the role of pathogens in biofilms of milk hoses used to collect milk fed to calves cannot be ruled out.
Biofilms in milking equipment can cause milk contamination for long periods of time, therefore, the presence of biofilms in milking equipment used to divert milk from the bulk tank could represent a consistent, long-term exposure to pathogenic microorganisms for calves that are fed with waste milk or unpasteurized transition milk collected with this tainted equipment. In addition, the presence of bacterial pathogens in the herd environment, like the ones observed in biofilms from milk hoses on Farms A and B, cannot be disregarded. Previous reports have demonstrated that on-farm bacterial strains present in biofilms and bulk tank milk can also be found in the farm environment, including fecal samples from cows (15). Although the source or direction of pathogenic microorganisms' contamination could not be established in a previous study (15) the role of biofilms may play a relevant role as a source of on-farm pathogens. For example, at first calving, about 2–50% of heifers may present S. aureus intramammary infections. The role of flies and cross-suckling in calves have been reported as two plausible reasons to explain this rather high intramammary infection prevalence in heifers (39, 40). However, our findings suggest that biofilms may also play a role in the epidemiology of S. aureus-intramammary infections in heifers due to the potential transferring of pathogenic bacteria from biofilms to the oral epithelium of calves during feeding, and the subsequent contamination of teats of female calves due to cross-suckling. In addition, the presence of Klebsiella and Pseudomonas-containing biofilms could be a persistent source of these pathogens on dairy farms. These microorganisms could be then spread to the farm environment by different means such as fecal shedding (11) of calves fed with contaminated waste milk, handling of biofilm-contaminated milking equipment by dairy personnel, or even insects including flies. Therefore, more research on these topics is warranted to better understand the epidemiology of pathogenic microorganisms of importance in dairy herd health.
In addition, as milk from cows undergoing antibiotic treatments is also diverted from the bulk tank, bacteria contained in the biofilms of milking equipment used for this purpose are continually exposed to antibiotic residues contained in the diverted milk. This mechanism could be a source of risk for the development of antimicrobial resistant bacteria potentially contained in the biofilms. Eventually, these bacteria may pass to the milk used for animal feed and their environment. Penati et al. (41) reported diarrhea and changes in gut microbiome of calves that were fed with waste milk containing antibiotic residues. Therefore, limiting the exposure of calves to both pathogens and antibiotic residues that might be present in waste milk is of utmost importance on dairy farms to protect this group and the overall herd health.
From a public health perspective, although milk commercialized for human consumption must be pasteurized according to Chilean regulations that have been in place since 1930, there still exists an informal raw-milk market in Chile. Although no detailed data regarding the quantities of raw milk being sold, nor the milk quality or its source is available from these informal markets, it is unofficially known that some vendors may acquire diverted/waste milk from farms to be sold for human consumption through informal markets. This practice is usually done under the assumption that milk is always boiled before consumption, which may not necessarily be true in all cases, thus creating a public health risk due to the presence of pathogens in that milk.
Drinking raw milk is a common practice in many Latin American countries. This practice is mostly non-regulated and neither the sanitary status nor the origin of milk (whether bulk tank or discarded milk) are known. The public health risks associated to the potential presence of pathogens in raw milk (42, 43) is further compounded for the presence of potentially harmful bacteria from biofilms in milking equipment used to harvest waste milk, and also for the risk of antimicrobial resistant bacteria from this source.
Despite the fact that this study was carried out on only three farms, the specific practices observed on them are common among other dairy farms, particularly, the neglecting of cleaning and sanitation of milking equipment used to collect waste milk. The findings of this study are relevant for a better understanding of the on-farm persistence of pathogens involved in the epidemiology of diseases of the young stock and, ultimately, the whole herd.
We can conclude that hoses used to divert milk, transition milk or colostrum can be a source of biofilms and pathogenic bacteria. Therefore, the use of separate hoses to divert milk from cows undergoing antibiotic treatments or mastitis, and to collect colostrum or transition milk is warranted. Furthermore, cleaning and sanitation protocols of all surfaces in contact with milk or colostrum are crucial, as well as the pasteurization of transition and waste milk. In addition, waste milk used to feed calves constitutes not only a biosecurity issue and a source of calf pathogens, but also an increased exposure and threat for the whole herd health and, potentially, for human health.
Data availability statement
The raw data supporting the conclusions of this article will be made available by the authors, without undue reservation.
Ethics statement
No animals were used during this study and ethical considerations were reviewed and approved by the Institutional Bioethics and Biosafety Committee of the Universidad de Concepción, Chile.
Author contributions
AL: field work, sample collection, microbiology analysis, sample fixation for microscopy, SEM visualization of samples, and preparation and drafting of the manuscript. MM: field work, sample collection, sample fixation for microscopy, and preparation and drafting of the manuscript. RO, JP, and AE: sample preparation, visualization, and image analysis by SEM. FN and KS: sample preparation, visualization, and image analysis by CSM. NG: sample preparation and analysis of isolates by MALDI-TOF MS. All authors have read, contributed to, and approved the final manuscript.
Funding
Financial support was provided by the Chilean Commission for Scientific and Technological Research, FONDECYT Project No 11130343 (Santiago, Chile) and also financial support for open access publication fees were provided by Vicerrectoría de Investigaciǿn y Desarrollo (VRID), Universidad de Concepción-Chile.
Acknowledgments
We thank dairy producers who allowed to conduct this study on their dairy farms.
Conflict of interest
The authors declare that the research was conducted in the absence of any commercial or financial relationships that could be construed as a potential conflict of interest.
Publisher's note
All claims expressed in this article are solely those of the authors and do not necessarily represent those of their affiliated organizations, or those of the publisher, the editors and the reviewers. Any product that may be evaluated in this article, or claim that may be made by its manufacturer, is not guaranteed or endorsed by the publisher.
References
1. Hall-Stoodley L, Costerton JW, Stoodley P. Bacterial biofilms: from the natural environment to infectious diseases. Nat Rev Microbiol. (2004) 2:95–10. doi: 10.1038/nrmicro821
2. Flemming HC, Neu TR. Wozniak DJ. The EPS matrix : the “house of biofilm cells”. J Bacteriol. (2007) 189:7945–7. doi: 10.1128/JB.00858-07
3. Flemming HC, Wingender J. The biofilm matrix. Nat Rev Microbiol. (2010) 8:623–33. doi: 10.1038/nrmicro2415
4. Shpigel NY, Pasternak Z, Factor G, Gottlieb Y. Diversity of bacterial biofilm communities on sprinklers from dairy farm cooling systems in Israel. PLoS ONE. (2015) 10:e0139111. doi: 10.1371/journal.pone.0139111
5. Hayer JJ, Heinemann C, Schulze-Dieckhoff BG, Steinhoff-Wagner J. A risk-oriented evaluation of biofilm and other influencing factors on biological quality of drinking water for dairy cows. J Anim Sci. (2022) 100:skac112. doi: 10.1093/jas/skac112
6. Latorre A A, Van Kessel JAS, Karns JS, Zurakowski MJ, Pradhan AK, Zadoks RN, et al. Molecular ecology of Listeria monocytogenes: evidence for a reservoir in milking equipment on a dairy farm. Appl Environ Microbiol. (2009) 75:1315–23. doi: 10.1128/AEM.01826-08
7. Latorre AA, Van Kessel JAS, Karns JS, Zurakowski MJ, Pradhan AK, Boor KJ, et al. Biofilm in milking equipment on a dairy farm as a potential source of bulk tank milk contamination with Listeria monocytogenes. J Dairy Sci. (2010) 93:2792–802. doi: 10.3168/jds.2009-2717
8. Weber M, Liedtke J, PlattesS, Lipski A. Bacterial community composition of biofilms in milking machines of two dairy farms assessed by a combination of culturedependent and -independent methods. PLoS ONE. (2019) 14:1–21. doi: 10.1371/journal.pone.0222238
9. Latorre AA, Pachá PA, González-Rocha G, San Martín I, Quezada-Aguiluz M, Aguayo-Reyes A, et al. On-farm surfaces in contact with milk: the role of Staphylococcus aureus-containing biofilms for udder health and milk quality. Foodborne Pathog Dis. (2020) 17:44–51. doi: 10.1089/fpd.2019.2704
10. Cousin MA. Presence and activity of psychrotrophic microorganisms in milk and dairy products: a review. J Food Prot. (1982) 45:172–207. doi: 10.4315/0362-028X-45.2.172
11. Munoz MA, Ahlström C, Rauch BJ, Zadoks RN. Fecal shedding of Klebsiella pneumoniae by dairy cows. J Dairy Sci. (2006) 89:3425–30. doi: 10.3168/jds.S0022-0302(06)72379-7
12. Huck JR, Sonnen M, Boor KJ. Tracking heat-resistant, cold-thriving fluid milk spoilage bacteria from farm to packaged product. J Dairy Sci. (2008) 91:1218–28. doi: 10.3168/jds.2007-0697
13. USDA-APHIS. Prevalence of Contagious Mastitis Pathogens on US Dairy Operations, 2007. APHIS Veterinary Services Info Sheet. #N533.1008. Washington, DC: APHIS, USDA (2008).
14. Shaheen R, Svensson B, Andersson MA, Christiansson A, Salkinoja-Salonen M. Persistence strategies of Bacillus cereus spores isolated from dairy silo tanks. Food Microbiol. (2010) 27:347–55. doi: 10.1016/j.fm.2009.11.004
15. Latorre AA, Van Kessel JS, Karns JS, Zurakowski MJ, Pradhan AK, Boor KJ, et al. Increased in vitro adherence and on-farm persistence of predominant and persistent Listeria monocytogenes strains in the milking system. Appl Environ Microbiol. (2011) 77:3676–84. doi: 10.1128/AEM.02441-10
16. Lee SHI, Mangolin BLC, Gonçalves JL, Neeff DV, Silva MP, Cruz AG et al. Biofilm-producing ability of Staphylococcus aureus isolates from Brazilian dairy farms. J Dairy Sci. (2014) 97:1812–6. doi: 10.3168/jds.2013-7387
17. Pacha PA, Munoz MA, González-Rocha G, San Martín I, Quezada-Aguiluz M, Aguayo-Reyes A, et al. Molecular diversity of Staphylococcus aureus and the role of milking equipment adherences or biofilm as a source for bulk tank milk contamination. J Dairy Sci. (2021) 104:3522–31. doi: 10.3168/jds.2020-19121
18. Mann EE, Wozniak DJ. Pseudomonas biofilm matrix composition and niche biology. FEMS Microbiol Rev. (2012) 36:893–916. doi: 10.1111/j.1574-6976.2011.00322.x
19. Nucera DM, Lomonaco S, Morra P, Ortoffi MF, Giaccone D, Grassi MA. Dissemination and persistence of Pseudomonas spp. in small-scale dairy farms. Ital J Food Saf. (2016) 5:91–4. doi: 10.4081/ijfs.2016.5652
20. Pilch HE, Steinberger AJ, Sockett DC, Aulik N, Suen G, Czuprynski CJ. Assessing the microbiota of recycled bedding sand on a Wisconsin dairy farm. J Anim Sci Biotechnol. (2021) 12:114. doi: 10.1186/s40104-021-00635-6
21. Moons P, Michiels CW, Aertsen A. Bacterial interactions in biofilms. Crit Rev Microbiol. (2009) 35:157–68. doi: 10.1080/10408410902809431
22. Murphy SC, Boor KJ. Trouble-shooting sources and causes of high bacteria counts in raw milk. Dairy Food Environ Sanit. (2000) 20:606–11.
23. Zottola EA, Sasahara KC. Microbial biofilms in the food processing industry—Should they be a concern? Int J Food Microbiol. (1994) 23:125–48. doi: 10.1016/0168-1605(94)90047-7
24. Wang G, Zhao G, Chao X, Xie L, Wang H. The characteristic of virulence, biofilm and antibiotic resistance of Klebsiella pneumoniae. Int J Environ Res Public Health. (2020) 17:6278. doi: 10.3390/ijerph17176278
25. Clegg S, Murphy CN. Epidemiology and virulence of Klebsiella pneumoniae. Microbiol Spectr. (2016) 4:UTI-0005-2012. doi: 10.1128/microbiolspec.UTI-0005-2012
26. Tran Thi MT, Wibowo D, Rehm BHA. Pseudomonas aeruginosa biofilms. Int J Mol Sci. (2020) 21:8671. doi: 10.3390/ijms21228671
27. Lee K, Yoon SS. Pseudomonas aeruginosa biofilm, a programmed bacterial life for fitness. J Microbiol Biotechnol. (2017) 27:1053–64. doi: 10.4014/jmb.1611.11056
28. Otto M. Staphylococcal biofilms. Microbiol Spectrum. (2018) 6:GPP3-0023-2018. doi: 10.1128/microbiolspec.GPP3-0023-2018
29. Moormeier DE, Bayles KW. Staphylococcus aureus biofilm: a complex developmental organism. Mol Microbiol. (2017) 104:365–76. doi: 10.1111/mmi.13634
30. National Mastitis Council. Troubleshooting Cleaning Problems in Milking Systems. Madison, WI: Natl. Mastitis Counc. Inc. (2004).
31. Sen S, Albay MK. Aetiology of respiratory tract infections in calves and evaluation of treatment with combination of amoxycillin and clavulanic acid. Indian Vet J. (2003) 80:519–21.
32. Icen H, Sekin S, Yesilmen S, Isik N, Simsek A. Viral and bacterial pathogen isolated and identified from pneumonic calves in region of Diyarbakir and its treatment with tulathromycin. J Anim Vet Adv. (2009) 8:1545–50.
33. Cheng F, Lia Z, Lana S, Liua W, Lia X, Zhoua Z, et al. Characterization of Klebsiella pneumoniae associated with cattle infections in southwest China using multi-locus sequence typing (MLST), antibiotic resistance and virulence-associated gene profile analysis. Braz J Microbiol. (2018) 49S:93–100. doi: 10.1016/j.bjm.2018.06.004
34. Choudharya M, Choudharya BK, Ghoshb RC, Bhoyarc S, Chaudharic S, Barbuddhea SB. Cultivable microbiota and pulmonary lesions in polymicrobial bovine pneumonia. Microb Pathog. (2019) 134:103577. doi: 10.1016/j.micpath.2019.103577
35. Komatsu T, Yoshida E, Shigenaga A, Yasuie N, Uchiyama S, Takamura Y, et al. Fatal suppurative meningoencephalitis caused by Klebsiella pneumoniae in two calves. J Vet Med Sci. (2021) 83:1113–9, 202. doi: 10.1292/jvms.21-0166
36. Bojkovski J, Milanov D, Savic S, Vasic A, Zdravkovic N, Rogožarski D, et al. Respiratory diseases of calves on dairy cow farm. Bull UASVM Vet Med. (2014) 71:313–20. doi: 10.15835/buasvmcn-vm:10325
37. Yeruham D, Elad Y, Avidar T, Goshen E. Four-year survey of urinary tract infections in calves in Israel. Vet Rec. (2004) 154:204–6. doi: 10.1136/vr.154.7.204
38. Duarte ER, Hamdan JS. Otitis in cattle, an aetiological review. J Vet Med B. (2004) 51:1–7. doi: 10.1046/j.1439-0450.2003.00719.x
39. De Vliegher S, Fox LK, Piepers S, McDougall S, Barkema HW. Invited review: mastitis in dairy heifers: nature of the disease, potential impact, prevention, and control. J Dairy Sci. (2012) 95:1025–40. doi: 10.3168/jds.2010-4074
40. Anderson KL, Lyman R, Moury K, Ray D, Watson DW, Correa MT. Molecular epidemiology of Staphylococcus aureus mastitis in dairy heifers. J Dairy Sci. (2012) 95:4921–30. doi: 10.3168/jds.2011-4913
41. Penati M, Sala G, Biscarini F, Boccardo A, Bronzo V, Castiglioni B, et al. Feeding pre-weaned calves with waste milk containing antibiotic residues is related to a higher incidence of diarrhea and alterations in the fecal microbiota. Front Vet Sci. (2021) 8:650150. doi: 10.3389/fvets.2021.650150
42. Oliver SP, Jayarao BM, Almeida RA. (2005). Foodborne pathogens in milk and the dairy farm environment: food safety and public health implications. Foodborne Pathog Dis. (2005) 2:115–29. doi: 10.1089/fpd.2005.2.115
Keywords: biofilms, milking equipment, milk hoses, colostrum, diverted milk
Citation: Latorre AA, Oliva R, Pugin J, Estay A, Nualart F, Salazar K, Garrido N and Muñoz MA (2022) Biofilms in hoses utilized to divert colostrum and milk on dairy farms: A report exploring their potential role in herd health, milk quality, and public health. Front. Vet. Sci. 9:969455. doi: 10.3389/fvets.2022.969455
Received: 15 June 2022; Accepted: 11 August 2022;
Published: 26 August 2022.
Edited by:
Orhan Sahin, Iowa State University, United StatesReviewed by:
Ramon Armengol, Universitat de Lleida, SpainBetsy Karle, University of California System, United States
Valerio Bronzo, University of Milan, Italy
Copyright © 2022 Latorre, Oliva, Pugin, Estay, Nualart, Salazar, Garrido and Muñoz. This is an open-access article distributed under the terms of the Creative Commons Attribution License (CC BY). The use, distribution or reproduction in other forums is permitted, provided the original author(s) and the copyright owner(s) are credited and that the original publication in this journal is cited, in accordance with accepted academic practice. No use, distribution or reproduction is permitted which does not comply with these terms.
*Correspondence: Alejandra A. Latorre, alatorre@udec.cl