- 1Jiangxi Provincial Key Laboratory for Animal Health, Institute of Animal Population Health, College of Animal Science and Technology, Jiangxi Agricultural University, Nanchang, China
- 2Department of Mathematics and Statistics, Wright State University, Dayton, OH, United States
This study aims to investigate the effect of heat stress on the physiological metabolism of young laying hens and whether N-acetyl-l-cysteine (NAC) can effectively alleviate heat stress. 120 Hy-Line Brown laying hens aged 12 weeks were randomly divided into four groups: the control group (fed on basal diet under thermal neutral condition), HS group (fed on basal diet under heat stress condition), CN group (fed on the basic meal supplemented with 1,000 mg NAC per kg under thermal neutral condition), and HS+N group (fed on the basic meal was supplemented with 1000 mg NAC per kg under heat stress condition). The HS and HS+N groups were exposed to 36 ± 1°C for 10 h/day. The effects of NAC on the changes of serum concentrations of T3, T4, and CORT and hypothalamic gene and protein expressions induced by heat stress were measured. Results showed that heat stress upregulated the contents of T3, T4, and CORT, while NAC reduced the contents of T3, T4, and CORT. In addition, NAC downregulated AgRP expression, while upregulated the expression of POMC. Moreover, the expressions of AMPKα1, LKB1, and CPT1 were inhibited by NAC, while the expressions of AKT1, ACC, GPAT, and PPARα were increased after NAC treatment, and HMGR did not change significantly. Western blot and comprehensive immunofluorescence section of AMPK in the hypothalamus showed that NAC attenuated the activity of AMPK. In conclusion, NAC can enhance the resistance of laying hens to heat stress by alleviating the metabolic disorders of serum T3, T4, and CORT induced by heat stress, inhibiting the activation of the AMPK pathway and regulating the expression of appetite-related genes in the hypothalamus.
Introduction
Heat stress (HS) refers to a series of specific responses to high temperature when the ambient temperature exceeds the upper limit that the animal body can control. In recent years, intensive farming has increased growing density, making birds more susceptible to heat stress as a result of their unique structure (1). The high temperature in summer makes it difficult for the chicken house to cool down, which induces hens suffer from heat stress. Heat stress is mainly manifested as a decrease in animal feed intake, slow body growth, reduced glucose control, lipid metabolism disorders, and decreased immune function (2, 3). Heat stress caused huge economic losses in the poultry industry (4). Hypothalamus is a key brain region that regulates metabolic homeostasis and energy balance, and plays a core role in regulating metabolic functions such as food intake, nutrient metabolism, body water balance, hormone secretion, basic metabolism, body temperature, and circadian rhythm (5). The physiological function of hypothalamus could be damage by heat stress.
In the state of heat stress, the body needs to mobilize the energy reserves of internal organs to fight the high-temperature environment, resulting in destroying metabolic homeostasis (6). Adenosine 5'-monophosphate-activated protein kinase (AMPK) regulates intracellular energy balance and even energy metabolism throughout the body (7). In the hypothalamus, AMPK participates in fatty acid metabolism and regulates food intake and energy metabolism to a certain extent, as well as mediating the effects of peripheral signals (hormones) (8). AMPK activation can regulate appetite promoting and suppressing factors to a certain extent (9). For example, AMPK- aminocyclopropane-1-carboxylate (ACC)- carnitine palmitoyltransferase I (CPT1) pathway can promote fatty acid oxidation and regulate the transcription and expression of appetite promoting peptide agouti-related peptide (AgRP) and neuropeptide y (NPY) (10, 11). However, it remains unclear the correction of heat stress and AMPK signaling pathway and appetite in the hypothalamus.
N-Acetyl-l-cysteine (NAC) was discovered in 1970 and is primarily considered to be an antioxidant. NAC has good antioxidant, immunomodulatory and anti-apoptotic effects in the clinic (12, 13). It has been pointed out that NAC has significant resistance to oxidative stress and inflammatory response caused by heat stress (14). However, it remains unclear whether NAC can effectively improve the energy metabolism disorder or regulate the expression of appetite genes caused by heat stress. Therefore, in this study, we set out to investigated the regulatory mechanism of heat stress on AMPK activity in the hypothalamus of laying hens and the integration of peripheral hormones in energy homeostasis. It also discussed whether N-acetylcysteine (NAC) can effectively relieve heat stress.
Materials and Methods
Animals
After 30-day adaptation period, a total of 120 12-weeks-old Hy-Line Brown laying hens were randomly divided into four groups: the control group (Con group), NAC treatment group (Control + NAC group, i.e., CN group), heat-stressed group (HS group) and heat-stress + NAC treatment group (HS + NAC group, i.e., HSN group). The variables were controlled by changing the environmental temperature. The Con group and HS group were given basal diet, CN group and HS + N group were given 1000 mg/ kg NAC. Con and CN groups were fed at a constant temperature (22 ± 1°C), while the room temperature of the HS group and the HS + N group was set to be 36 ± 1°C for 10 h a day (8 am to 6 pm), and 22 ± 1°C during the rest of the time, which means periodic heat stress. Food and water were fed ad libitum. The chickens were exposed to 12 h of light per day and the humidity was set to be between 50 and 55%.
Tissue Sample Collection
On day 7, 14, and 21, hens were prohibited from feeding and drinking for 12 hours before being euthanized. Blood samples were collected and centrifuged at 3,500 rpm for 10 minutes. The extracted serum was stored at −20°C. Hypothalamus tissue was washed with PBS, snap-frozen in liquid nitrogen, and stored at −80°C for subsequent experiments.
ELISA
Serum triiodothyronine (T3), thyroxine (T4), and corticosterone (CORT) contents were measured using ELISA kit (Beijing Huaying Biotechnology Research Institute, Beijing, China) based on the Quick EIATM ELISA protocol, according to the manufacturer's instructions.
Real-Time Quantitative PCR
Total RNA was isolated with TransZolUp Reagent (TransGen Biotech, Beijing, China) according to the protocol reported previously (15). In brief, according to the instructions of the kit, cDNA was synthesized using cDNA Synthesis SuperMix Reagent kit (TransGen Biotech, Beijing, China). qRT-PCR was performed using the Light Cycler 96 system according to the protocol. Data were analyzed by the 2−ΔΔCt method. The specific primer sequences were shown in Table 1.
Western Blotting
Protein from hypothalamus tissue was extracted using the kit (Wuhan Seville Biotechnology Co., Ltd., Wuhan, Hubei, China), and the protein concentration was measured by the BCA method. The protein samples were separated using SDS-PAGE (10% separation gel and 4% concentration gel) and then transferred to polyvinylidene fluoride (PVDF) membranes (0.45 μm). The membrane was incubated with 5% nonfat milk at room temperature for 2 h before overnight incubation with specific antibodies at 4°C. Then, it was incubated with the corresponding horseradish peroxidase (HRP)-coupled (1:5,000) secondary antibody for 1 h at room temperature. The signal was detected by a chemiluminescence kit (Changjiang Biotechnology Co., Ltd., Nanjing, Jiangsu, China). Finally, digital images were obtained using the Bio-Rad Gel Detection System (Bio-Rad Laboratories, Inc., Hercules, CA, United States), and the results were analyzed using ImageJ (Image processing software).
Immunofluorescence of AMPK in the Hypothalamus
The dissected hypothalamus was immediately wrapped in silver paper, which was rapidly frozen with liquid nitrogen for 60 s and then embedded with fully frozen brain tissue at optimal cutting temperature (O.C.T.). The frozen sections (8 μm) were placed at room temperature for 5 min, and then fixed with 4% neutral paraformaldehyde. After 10 min, they were sealed with 3% bovine serum and then incubated with primary antibody at 4°C overnight. The cell nuclei marker was DAPI, and the dilution ratio of the AMPK antibody was 1:100.
Statistical Analysis
Data analysis was conducted using SPSS 17.0 software. GraphPad Prism 7.0 software was used to plot the graphs. Results were presented in the form of mean value ± standard error (SE). ANOVA and t tests were used to make the comparison between groups. P > 0.05 indicates that the difference is not statistically significant. P < 0.05 indicates that the difference is statistically significant. P < 0.01 indicates that the difference is extremely statistically significant.
Results
Serum Indexes
The influence of NAC on the changes of serum chemical values of hens under heat stress was shown in Figure 1. On day 7 of heat stress, the contents of T3, T4 and CORT in the HS group were significantly upregulated than those in the control group (P < 0.01), while, NAC treatment downregulated the contents of T3, T4, and CORT in the HSN group (P < 0.01) compared to the HS group. On day 14, the content of CORT in the HS group was still significantly increased (P < 0.01). Compared with the HS group, the contents of T3, T4, and CORT in the HSN group were significantly decreased (P < 0.01). On day 21, the content of CORT in the HS group were increased than those in the control group (P < 0.01). Compared with the HS group, the T4 and CORT of the HSN group were significantly decreased (P < 0.01).
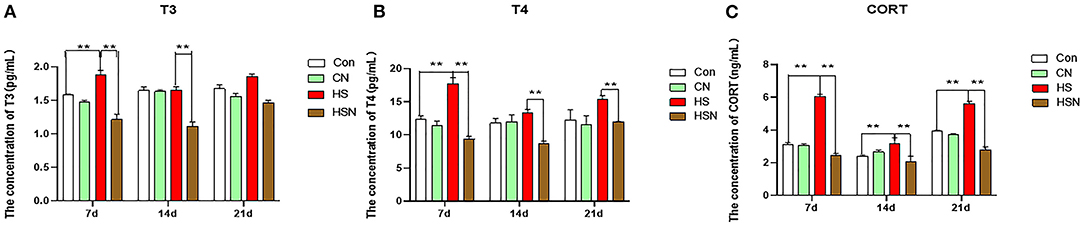
Figure 1. Effects of NAC and heat stress on serum T3, T4 and CORT in layers. (A) Serum T3 level. (B) Serum T4 level. (C) Serum CORT level. *P < 0.01; **P < 0.001.
Expressions of Appetite-Related Genes in the Hypothalamus
Expressions of appetite-related genes in hypothalamus of different groups were shown in Figure 2. On day 7 and 14, compared with the control group, AgRP mRNA expression in the HS group was significantly increased (P < 0.01), and decreased in day 21 (P < 0.01). Compared with the HS group, the expression of AgRP in the NAC-treated HSN group was significantly decreased (P < 0.01 or P < 0.05). Compared with the control group, the expression level of proopiomelanocortin (POMC) in the HS group was significantly higher (P < 0.01). The POMC expression in the HSN group was significantly lower than that in the HS group (P < 0.01 or P < 0.05).
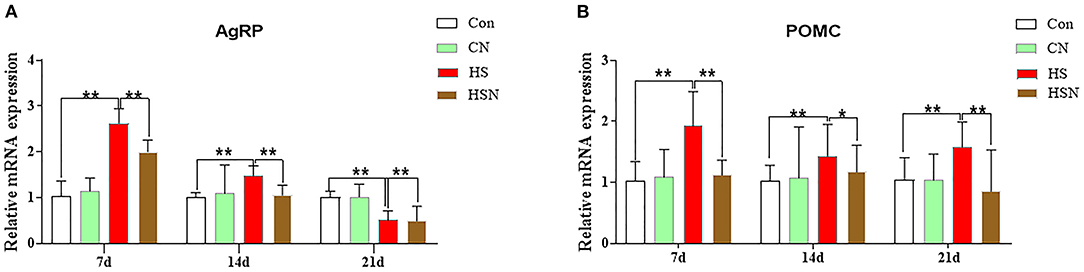
Figure 2. Effects of NAC and heat stress on appetite-related genes expression in the hypothalamus in layers. (A) Expression of AgRP mRNA. (B) Expression of POMC mRNA. *P < 0.01; **P < 0.001.
Expressions of AMPK Pathway-Related Genes in the Hypothalamus
Figure 3 showed the effect of NAC on the expression of AMPK pathway-related genes in the hypothalamus under heat stress. On day 7, the mRNA expression of the catalytic alpha subunit of AMPK (AMPKα1), liver kinase b1 (LKB1), and CPT1 in the HS group increased significantly (P < 0.05 or P < 0.01). The expression of AKT1, ACC, mammalian target of rapamycin (mTOR), and glycerol-3-hosphate acyltransferase enzyme (GPAT) decreased significantly (P < 0.05). The expression of 3-hydroxy-3-methyl-glutaryl-coenzyme reductase (HMGR) and peroxisome proliferator-activated receptor α (PPARα) decreased but not significantly (P > 0.05). Compared with the HS group, the expression levels of AMPKα1, LKB1, and CPT1 in the HSN group were significantly decreased (P < 0.01 or P < 0.05). The expression levels of AKT1, PPARα, ACC, and GPAT in the HS group were significantly increased (P < 0.05). On day 14, the AMPKα1 and LKB1 expression in HS group increased significantly (P < 0.01), and the AKT1 and ACC expression decreased significantly (P < 0.01 or P < 0.05). After NAC treatment, the AMPKα1 and LKB1 expression in the HSN group decreased significantly (P < 0.05), and the AKT1 and ACC expression increased significantly (P < 0.01 or P < 0.05). On day 21, the AMPKα1, LKB1 and CPT1 expression increased significantly in the HS group (P < 0.01 or P < 0.05), and the mTOR and AKT1 expression decreased significantly (P < 0.05). Compared with the HS group, the expression of AMPKα1 and LKB1 in the NAC-treated HSN group was significantly decreased (P < 0.05), and the AKT1 expression was significantly increased (P < 0.05).
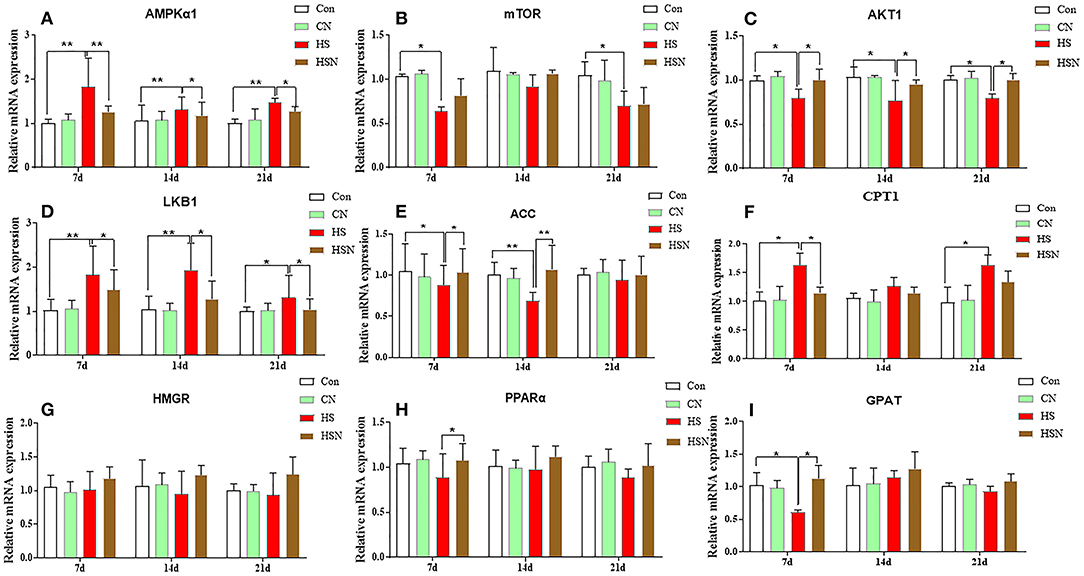
Figure 3. Effects of NAC and heat stress on the mRNA expression levels of AMPK pathway-related genes in the hypothalamus. (A) Expression of AMPKα1 mRNA. (B) Expression of mTOR mRNA. (C) Expression of AKT1 mRNA. (D) Expression of LKB1 mRNA. (E) Expression of ACC mRNA. (F) Expression of CPT1 mRNA. (G) Expression of HMGR mRNA. (H) Expression of PPARα mRNA. (I) Expression of GPAT mRNA. *P < 0.01; **P < 0.001.
AMPK Protein Expression in the Hypothalamus
The grayscale scanning results of AMPK expression level were shown in Figure 4. On day 7, the AMPK protein expression level in control group was significantly lower than that in the HS group (P < 0.01), and the AMPK protein expression level in the HSN group was significantly lower than that in the HS group (P < 0.01).
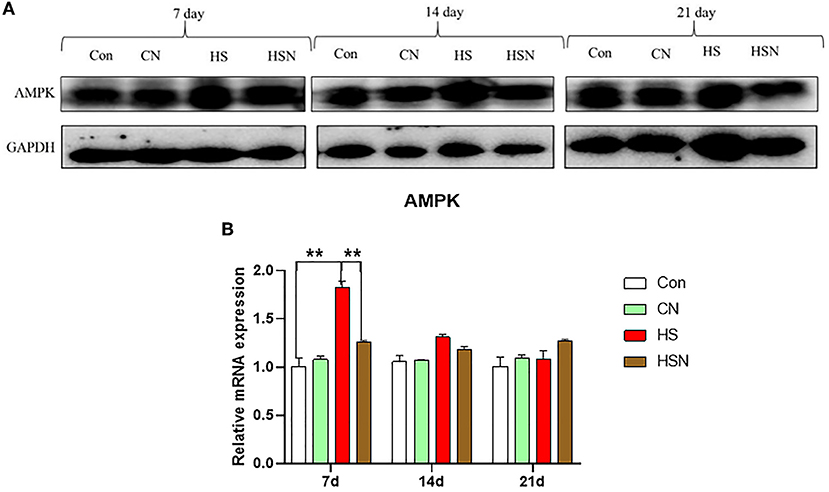
Figure 4. Effects of NAC and heat stress on the expression levels of key proteins in hypothalamus. (A) AMPK protein expression levels. (B) Relative mRNA expression level of AMPK. *P < 0.01; **P < 0.001.
Immunofluorescence Analysis
Figure 5 showed immunofluorescence expression of AMPK in the hypothalamus. On day 14 of heat stress, the AMPK fluorescence intensity was considerably greater in the HS group than in the control group, while NAC dramatically lowered AMPK fluorescence intensity. The white arrow in Figure 5 indicated the positive expression of the AMPK protein.
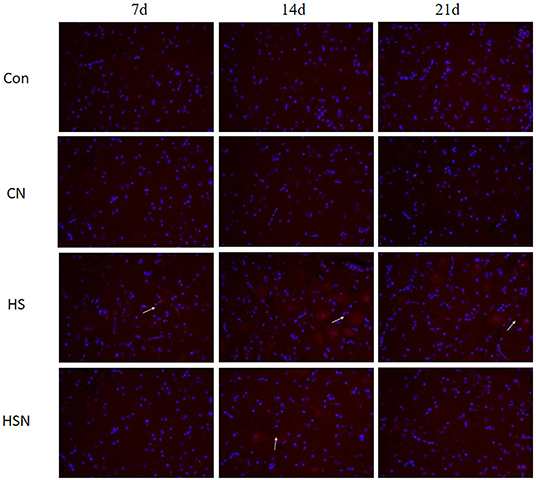
Figure 5. Effect of heat stress and NAC on protein levels of AMPK in the hypothalamus (400 ×) (the red part pointed by the arrow is the AMPK protein).
Discussion
Heat stress can lead to metabolic disorders, including glucose metabolism disorders, fat metabolism disorders, and hormone metabolism disorders (16–18). Hypothalamus is an important organ with the function of integrating various metabolic regulatory signals. When laying hens suffer heat stress, the hypothalamus can be easily damaged, which changes the metabolism of laying hens and inhibits the performance of laying hens. NAC has been proved to have a certain therapeutic effect on oxidative stress and inflammation induced by heat stress (14). Whether this treatment of injury can effectively regulate the body's metabolic disorders remain unclear. The purpose of this study was to investigate the regulatory role of NAC in energy metabolism disorders induced by heat stress.
Among different metabolic pathways, AMPK has attracted a lot of attention as a measure of systemic energy homeostasis. Activation of AMPK inhibits the anabolic pathway of ATP consumption and induces the catabolic pathway of ATP production (19). These pathways work by regulating gene expression of key metabolic enzymes such as fatty acids, cholesterol, and protein synthesis (20). Studies have shown that the fatty acid biosynthesis pathway includes two key enzymes: ACC and FAS, both of which are expressed in the hypothalamus (21). Malonyl-CoA is not only a mediator of fatty acid biosynthesis but also plays an important role in the control of metabolic conversion between fat production and fatty acid oxidation. AMPK plays an important role in fatty acid biosynthesis. After activation, AMPK phosphorylates and inhibits ACC, prevents malonyl-CoA production, further stimulates CPT1, and ultimately promotes mitochondrial fatty acid oxidation (21). Our results showed that heat stress activated AMPK and inhibited the expression of ACC, which could further stimulate the expression of CPT1. LKB1 is an activation enzyme upstream of AMPK that is responsible for activating energy stress. The LKB1-AMPK signaling pathway promotes fatty acid oxidation by inhibiting glycogen production in response to energy stress. Finally, the results showed that the LKB1 expression in the hypothalamus was significantly increased under heat stress (22). HMGR is an enzyme that affects the differentiation of adipocytes and catalyzes the reduction of 3-hydroxy-3-meglutarate mono-acyl-CoA in cholesterol biosynthesis (23). Activation of AMPK inhibits the expression of HMGR. PPARα is an important gene which is located downstream of AMPK. It is involved in fatty acid metabolism and can maintain cell energy homeostasis (24). The high expression of GPAT can prevent acyl-CoA from entering the mitochondrial matrix, weaken the competition of fatty acid pools, and accelerate the synthesis rate of triglycerides (25). In our results, the changes in the expression levels of these three genes were not significant. In addition, when ATP is depleted, activation of AMPK can lead to inhibition of the mTOR signaling pathway downstream of AMPK, which in turn can inhibit protein synthesis, enabling AMPK to conserve cellular energy even in a low-energy state (26). In addition, mTOR is regulated by AKT, and the activated AKT can directly phosphate mTOR (27). Our results showed that activation of AMPK induced by heat stress could inhibit the expression of mTOR and AKT1. However, after NAC treatment, it could effectively inhibit the abnormal expression of AMPK-related genes induced by heat stress, and make the abnormal expression of genes tend to be normal. This indicated that in our experiment, NAC could effectively inhibit the activation of hypothalamus AMPK caused by heat stress, effectively reduce the decomposition of fatty acids, and maintain the energy metabolism homeostasis of hypothalamus. In addition, it has been found that AMPK acts as a sensor and regulator of energy homeostasis throughout the body, linking the metabolic state with the neuropeptide system and ultimately regulating food intake (28).
The hypothalamus is a key center for regulating appetite and energy balance by regulating the expression of specific neurotransmitters/neuropeptides in response to nutrient/hormone signals, thereby promoting the balance of energy intake and expenditure (29). The center in the hypothalamus that controls food intake consists of two groups of neurons that receive peripheral nutrient signals. On the one hand, they participate in the regulation of feeding through the expression of different appetite peptides, such as AgRP. On the other hand, anti-eating neuropeptides include POMC and so on. As a regulator of energy homeostasis throughout the body, activation of AMPK can stimulate feeding, whereas inhibition of AMPK activity in the hypothalamus can lead to anorexia. Our experimental results showed that the expression of the appetite-promoting AgPR gene was significantly increased under heat stress, while the expression of the appetite-suppressing POMC gene was significantly decreased. The addition of NAC in the diet effectively inhibited the overexpression of AgRP and promoted the expression of POMC. This indicated that NAC effectively inhibited heat stress, mitigated its effects and effectively controlled the expression of appetite genes, and further maintained the normal energy balance of the body.
Heat stress can also stimulate the body's systemic neuroendocrine response to activate the hypothalamic-pituitary-adrenal system and stimulate the secretion of adrenal corticosteroids (30). The secretion rate of adrenocortical hormone is related to basal metabolic rate and heat production (28). The common adrenal corticosteroid is the glucocorticoid CORT. On the one hand, glucocorticoids can directly affect the metabolism of the body. On the other hand, they can directly damage hippocampal neurons through metabolism and other ways (31). Our experimental results showed that heat stress significantly increased the content of CORT in serum, indicating that heat stress activated the hypothalamic-pituitary-adrenal axis and improved the basic metabolism of the body.
Conclusion
Our results indicated that NAC can effectively ameliorate the pathological changes in hypothalamus caused by heat stress by alleviating the metabolic disorders of T3, T4, and CORT in serum, inhibiting the activation of the hypothalamus AMPK pathway, and regulating the expression of appetite genes.
Data Availability Statement
The original contributions presented in the study are included in the article/Supplementary Material, further inquiries can be directed to the corresponding authors.
Ethics Statement
The animal study was reviewed and approved by Animal Health and Utilization Committee of Jiangxi Agricultural University.
Author Contributions
ZL: data management, research, methodology, and writing—manuscript. YZha: data analysis, investigation, and method. YZhu: visualization. ZX and CW: verification. PL and GH: project management. GL: analysis. WC: software. XGa: correction and editor. XGu: conceptualization, funding access, methods, and supervision. All authors contributed to the article and approved the submitted version.
Funding
This work was supported by National Natural Science Foundation of China (Nos. 32060760 and 31460679), Natural Science Foundation of Jiangxi Province (No. 2017ACB20012), and the Technology System of Modern Agricultural Poultry Industry of Jiangxi Province (JXARS).
Conflict of Interest
The authors declare that the research was conducted in the absence of any commercial or financial relationships that could be construed as a potential conflict of interest.
Publisher's Note
All claims expressed in this article are solely those of the authors and do not necessarily represent those of their affiliated organizations, or those of the publisher, the editors and the reviewers. Any product that may be evaluated in this article, or claim that may be made by its manufacturer, is not guaranteed or endorsed by the publisher.
Supplementary Material
The Supplementary Material for this article can be found online at: https://www.frontiersin.org/articles/10.3389/fvets.2022.936250/full#supplementary-material
References
1. Hartanto S, Ko HS, Jee SH, Kang JU, Seo JS, Kang YH, et al. Effect of dietary nutmeg oil on heat-stress tolerance-related parameters in korean native chicken reared under hot temperature. J Anim Physiol Anim Nutr (Berl). (2019) 103:1160–7. doi: 10.1111/jpn.13113
2. Akşit M Yalçin S Ozkan S Metin K Ozdemir D. Effects of temperature during rearing and crating on stress parameters and meat quality of broilers. Poult Sci. (2006) 85:1867–74. doi: 10.1093/ps/85.11.1867
3. He S, Yu Q, He Y, Hu R, Xia S, He J. Dietary resveratrol supplementation inhibits heat stress-induced high-activated innate immunity and inflammatory response in spleen of yellow-feather broilers. Poult Sci. (2019) 98:6378–87. doi: 10.3382/ps/pez471
4. Lu Z, He X, Ma B, Zhang L, Li J, Jiang Y, et al. Chronic heat stress impairs the quality of breast-muscle meat in broilers by affecting redox status and energy-substance metabolism. J Agric Food Chem. (2017):11251–8. doi: 10.1021/acs.jafc.7b04428
5. Almabrouk TAM, White AD, Ugusman AB, Skiba DS, Katwan OJ, Alganga H, et al. High fat diet attenuates the anticontractile activity of aortic PVAT via a mechanism involving AMPK and reduced adiponectin secretion. Front Physiol. (2018) 9:51. doi: 10.3389/fphys.2018.00051
6. Belhadj Slimen I, Najar T, Ghram A, Abdrrabba M. Heat stress effects on livestock: molecular, cellular and metabolic aspects, a review. J Anim Physiol Anim Nutr. (2016) 100:401–12. doi: 10.1111/jpn.12379
7. Carling D. AMPK signalling in health and disease. Curr Opin Cell Biol. (2017) 45:31–7. doi: 10.1016/j.ceb.2017.01.005
8. Hardie DG. AMP-activated protein kinase—an energy sensor that regulates all aspects of cell function. Genes Dev. (2011) 25:1895–908. doi: 10.1101/gad.17420111
9. Hu X, Kong L, Xiao C, Zhu Q, Song Z. The AMPK-mTOR signaling pathway is involved in regulation of food intake in the hypothalamus of stressed chickens. Comp Biochem Physiol A Mol Integr Physiol. (2021) 258:110979. doi: 10.1016/j.cbpa.2021.110979
10. Stark R, Ashley SE, Andrews ZB. Ampk and the neuroendocrine regulation of appetite and energy expenditure. Mol Cell Endocrinol. (2013) 366:215–23. doi: 10.1016/j.mce.2012.06.012
11. Fernandez-Felipe J, Valencia-Avezuela M, Merino B, Somoza B, Cano V, Sanz-Martos AB, et al. Effects of saturated versus unsaturated fatty acids on metabolism, gliosis, and hypothalamic leptin sensitivity in male mice. Nutr Neurosci. (2022) 2022:1–14. doi: 10.1080/1028415X.2022.2029294
12. Aldini G, Altomare A, Baron G, Vistoli G, Sergio F. N-acetylcysteine as an antioxidant and disulphide breaking agent: the reasons why. Free Radic Res. (2018) 52:1–12. doi: 10.1080/10715762.2018.1468564
13. Kang R, Li R, Dai P, Li Z, Li Y, Li C. Deoxynivalenol induced apoptosis and inflammation of IPEC-J2 cells by promoting ROS production. Environ Pollut. (2019) 251:689–98. doi: 10.1016/j.envpol.2019.05.026
14. Zhao Y, Zhuang Y, Shi Y, Xu Z, Zhou C, Guo L, et al. Effects of N-acetyl-l-cysteine on heat stress-induced oxidative stress and inflammation in the hypothalamus of hens. J Therm Biol. (2021) 98:102927. doi: 10.1016/j.jtherbio.2021.102927
15. Zhao Y, Zhou C, Wu C, Guo X, Hu G, Wu Q, et al. Subchronic oral mercury caused intestinal injury and changed gut microbiota in mice. Sci Total Environ. (2020) 721:137639. doi: 10.1016/j.scitotenv.2020.137639
16. Eto K, Yamashita T, Matsui J, Terauchi Y, Noda M, Kadowaki T. Genetic manipulations of fatty acid metabolism in beta-cells are associated with dysregulated insulin secretion. Diabetes. (2002) 51:S414–20. doi: 10.2337/diabetes.51.2007.s414
17. Garriga C, Hunter RR, Amat C, Planas JM, Mitchell MA, Moretó M. Heat stress increases apical glucose transport in the chicken jejunum. Am J Physiol Regul Integr Comp Physiol. (2006) 290:R195–201. doi: 10.1152/ajpregu.00393.2005
18. Baumgard LHR Jr. Effects of heat stress on postabsorptive metabolism and energetics. Annu Rev Anim Biosci. (2012) 1:311–37. doi: 10.1146/annurev-animal-031412-103644
19. Kahn BB, Alquier T, Carling D, Hardie DG. AMP-activated protein kinase: ancient energy gauge provides clues to modern understanding of metabolism. Cell Metab. (2005) 1:15–25. doi: 10.1016/j.cmet.2004.12.003
20. Wu L, Zhang L, Li B, Jiang H, Duan Y, Xie Z, et al. AMP-activated protein kinase (AMPK) regulates energy metabolism through modulating thermogenesis in adipose tissue. Front Physiol. (2018) 9:122. doi: 10.3389/fphys.2018.00122
21. Gao S, Serra D, Keung W, Hegardt FG, Lopaschuk GD. Important role of ventromedial hypothalamic carnitine palmitoyltransferase-−1a in the control of food intake. Am J Physiol Endocrinol Metab. (2013) 305:E336–47. doi: 10.1152/ajpendo.00168.2013
22. Beirowski B. The LKB1-AMPK and mTORC1 metabolic signaling networks in Schwann cells control axon integrity and myelination: assembling and upholding nerves by metabolic signaling in Schwann cells. Bioessays. (2019) 41:e1800075. doi: 10.1002/bies.201800075
23. Lin H, Wei C, Zhang X, You W, Liu G. HMGR overexpression and interference affect the expression of steroidogenic genes and cholesterol content in bovine intramuscular adipocytes. Sci Rep. (2020) 10:16606. doi: 10.1038/s41598-020-73626-8
24. Staels B, Pawlak M, Lefebvre P. Molecular mechanism of PPAR alpha action and its impact on lipid metabolism, inflammation and fibrosis in non-alcoholic fatty liver disease. J Hepatol. (2015) 62:720–33. doi: 10.1016/j.jhep.2014.10.039
25. Cooper DE, Grevengoed TJ, Klett EL, Coleman RA. Glycerol-3-phosphate acyltransferase isoform-4 (GPAT4) limits oxidation of exogenous fatty acids in brown adipocytes. J Biol Chem. (2015) 290:15112–20. doi: 10.1074/jbc.M115.649970
26. Garza-Lombó C, Schroder A, Reyes-Reyes EM, Franco R. mTOR/AMPK signaling in the brain: cell metabolism, proteostasis and survival. Curr Opin Toxicol. (2018) 8:102–10. doi: 10.1016/j.cotox.2018.05.002
27. Missiaglia E, Dalai I, Barbi S, Beghelli S, Scarpa A. Pancreatic endocrine tumors: expression profiling evidences a role for AKT-mTOR pathway. J Clin Oncol. (2009) 28:245–55. doi: 10.1200/JCO.2008.21.5988
28. Bagath M, Krishnan G, Devaraj C, Rashamol VP, Pragna P, Lees AM, et al. The impact of heat stress on the immune system in dairy cattle: a review. Res Vet Sci. (2019) 126:94–102. doi: 10.1016/j.rvsc.2019.08.011
29. Friedman JM. Leptin and the endocrine control of energy balance. Net Metab. (2019) 1:754–64. doi: 10.1038/s42255-019-0095-y
30. Lv Q, Yang Q, Cui Y, Yang J, Wu G, Liu M, et al. Effects of taurine on ACE, ACE2 and HSP70 expression of hypothalamic-pituitary-adrenal axis in stress-induced hypertensive rats. Adv Exp Med Biol. (2017) 2:871–86. doi: 10.1007/978-94-024-1079-2_69
Keywords: hypothalamus, heat stress, AMPK pathway, appetite, N-acetyl-l-cysteine
Citation: Li Z, Zhao Y, Zhuang Y, Xu Z, Wu C, Liu P, Hu G, Li G, Chen W, Gao X and Guo X (2022) Effects of N-Acetyl-L-Cysteine on Serum Indices and Hypothalamic AMPK-Related Gene Expression Under Chronic Heat Stress. Front. Vet. Sci. 9:936250. doi: 10.3389/fvets.2022.936250
Received: 05 May 2022; Accepted: 18 May 2022;
Published: 15 June 2022.
Edited by:
Xiaolong Gu, Yunnan Agricultural University, ChinaReviewed by:
Xingxiang Chen, Nanjing Agricultural University, ChinaJianzhu Liu, Shandong Agricultural University, China
Copyright © 2022 Li, Zhao, Zhuang, Xu, Wu, Liu, Hu, Li, Chen, Gao and Guo. This is an open-access article distributed under the terms of the Creative Commons Attribution License (CC BY). The use, distribution or reproduction in other forums is permitted, provided the original author(s) and the copyright owner(s) are credited and that the original publication in this journal is cited, in accordance with accepted academic practice. No use, distribution or reproduction is permitted which does not comply with these terms.
*Correspondence: Xiaona Gao, eGlhb25hLmdhb0BqeGF1LmVkdS5jbg==; Xiaoquan Guo, eHFndW8yMDcyMEBqeGF1LmVkdS5jbg==
†These authors share first authorship