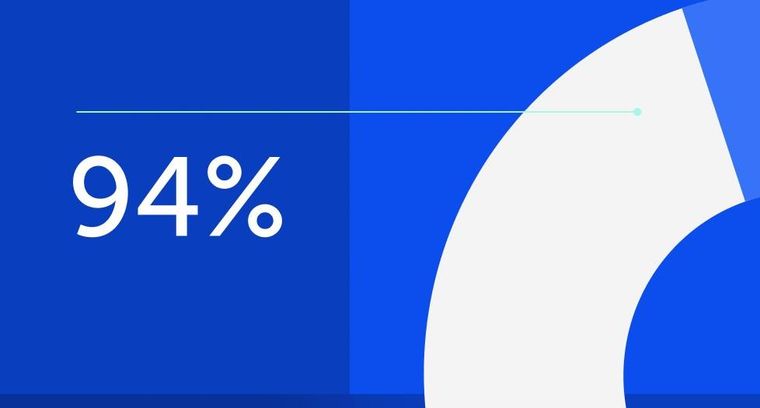
94% of researchers rate our articles as excellent or good
Learn more about the work of our research integrity team to safeguard the quality of each article we publish.
Find out more
ORIGINAL RESEARCH article
Front. Vet. Sci., 09 August 2022
Sec. Veterinary Clinical, Anatomical, and Comparative Pathology
Volume 9 - 2022 | https://doi.org/10.3389/fvets.2022.932474
Cancer is the leading cause of death in companion animals, and successful early treatment has been a challenge in the veterinary field. We have developed the Non-Invasive Cancer Screening (N.C.S.) Study to perform cancer detection through the analysis of canine urine samples. The test makes use of the strong olfactory system of the nematode Caenorhabditis elegans, which was previously shown to positively respond to urine samples from human cancer patients. We performed a proof-of-concept study to optimize the detection capability in urine samples obtained from dogs with naturally occurring cancers. In this study, we established a scale for identifying the cancer risk based on the magnitude of the chemotaxis index of C. elegans toward a canine urine sample. Through validation, the N.C.S. Study achieved a sensitivity of 85%, showing that it is highly sensitive to indicate the presence of cancer across multiple types of common canine cancers. The test also showed a 90% specificity to cancer samples, indicating a low rate of over-identifying cancer risk. From these results, we have demonstrated the ability to perform low-cost, non-invasive cancer detection in companion animals—a method that can increase the ability to perform cancer diagnosis and treatment.
There are over 200 million companion animals (dogs, cats, and horses) in the United States, and cancer is the leading cause of death among them (1). Approximately one in four dogs and one in five cats will develop cancer in their lifetimes, according to the Veterinary Cancer Society (2). Cancer in companion animals is difficult to treat successfully because few symptoms are evident in its early stages, and by the time symptoms become apparent, the cancer is usually advanced with high mortality. It has been shown that approximately half of all canine cancers are treatable if diagnosed early enough, and new therapeutic approaches are continuously being established (3–6). Existing tests are available for cancer screening (7) but can be too expensive or invasive to be conducted regularly for some dog owners. Thus, there is an urgent need for the development of a novel economical and non-invasive method for cancer screening to increase the probability of successful treatment.
Caenorhabditis elegans is a simple multicellular organism that is often used as a model to study biological phenomena such as cellular signaling, neural development, and aging in higher multicellular animals (8). Breeding isogenic populations of C. elegans is straightforward, as nearly all animals in a wild-type population are hermaphrodites who reproduce through self-fertilization and are fed a diet of Escherichia coli. Caenorhabditis elegans possesses a highly sensitive olfactory system to navigate its environment and detect food through the identification of chemical cues (9, 10). Caenorhabditis elegans encodes at least 1,500 predicted G-protein-coupled receptors (GPCRs) (11). Some of these GPCRs are olfactory receptors that underlie the worm's odor detection capabilities. Caenorhabditis elegans has an excellent sense of smell and possesses approximately 1.5 times as many different types of olfactory receptors as a dog (12). Once C. elegans detects an attractive odorant, it aligns with the chemical odorant and travels toward it (a process known as chemotaxis). This acute sense of smell allows for C. elegans to detect distinct volatile organic compound (VOC) profiles within animal urine.
The volatilome is the collection of VOCs which are present in the outputs of a biological organism (13). Cancer cells are known to emit VOCs that produce an odor that is distinguishable from that of non-cancer patients (14, 15). Changes in the volatilome of specimens in animals affected with cancer have been measured using both gas chromatography and mass spectrometry (16, 17). These odorant signatures are detectable in samples acquired from animals such as dogs (18) and mice (19), and thus, could serve as a marker for identifying cancer. It has been shown that C. elegans can quantitatively detect the presence of signature VOCs in both culture media of cancer cells in vitro and urine samples of human cancer patients through chemotaxis assays and calcium imaging of the AWC neuron (20–23). However, C. elegans has not yet been shown to identify cancerous VOC signatures in canine urine samples.
Here, we conducted the Non-Invasive Cancer Screening (N.C.S.) Study to measure the differences in C. elegans chemotaxis between urine samples from canine cancer patients and urine samples from healthy dogs with no diagnosed cancer. In the first part of the N.C.S. Study, we acquired initial data used to develop a screening method that identifies increased cancer risk through assays of canine urine samples. The study assesses multiple replicates of plate-based chemotaxis assays to measure the olfactory response through a mean chemotaxis index (CI). Based on these results, a risk assessment is made based on how the index relates to that of previously measured cancer and non-cancer samples. To validate the performance of our method, we assessed its ability to identify increased cancer risk using urine samples from dogs with four common types of canine cancer. In doing this, we demonstrate the potential for accurate, rapid, and non-invasive screening for cancer risk using urine samples from canine veterinary patients.
All samples from non-cancer patients, as well as the initial set of samples from cancer patients were obtained from Triangle Veterinary Hospital (Durham, NC), Lake Pine Animal Hospital (Apex, NC), Care First Animal Hospital (Raleigh, NC) New Light Animal Hospital (Wake Forest, NC), Bull City Veterinary Hospital (Durham, NC), and Knightdale Animal Hospital (Knightdale, NC). The second set of 40 cancer samples (10 samples each from patients with lymphoma, mast cell tumor, melanoma, and hemangiosarcoma) was obtained from the Ohio State University Center for Clinical and Translational Science. Upon acquisition, the urine samples were immediately stored at −20°C until assays are conducted. Each specimen was aliquoted into 100 μl portions to minimize repeat freezing and thawing each time an assay is performed.
Caenorhabditis elegans strain N2 and E. coli strain HB101 were obtained from the Caenorhabditis Genetics Center (University of Minnesota). Caenorhabditis elegans was age-synchronized by standard bleaching protocols and was cultured at 20°C on nematode growth media (NGM) plates seeded with HB101 bacterial lawns. The NGM plates were purchased pre-poured from LabExpress (Ann Arbor, MI).
Assays are performed using well-fed age-synchronized populations of N2 worms grown at 20°C for 3 days, and conducted on CTX plates (2% Agar, 5 mM KPO4 buffer at pH 6, 1 mM CaCl2, 1 mM MgSO4) which were purchased pre-poured from LabExpress (Ann Arbor, MI). The urine samples were thawed and diluted at a ratio of 1:2 of urine to CTX buffer (5 mM KPO4 buffer at pH 6, 1 mM CaCl2, 1 mM MgSO4). The urine samples were centrifuged and were then mixed at a ratio of 2:1 diluted urine to 1 M sodium azide. The urine mixture was then spotted at the “plus” marks on each chemotaxis plate. A control buffer mixture was prepared using a 2:1 ratio of CTX buffer to 1 M sodium azide and was spotted at the “minus” marks on each chemotaxis plate (Supplementary Figure 1). Young adult worms were washed from NGM plates using M9 buffer into conical tubes and were allowed to settle. The worms were then washed three times with CTX buffer to remove traces of the bacterial food source. Approximately 75–100 worms were placed at the center of each plate, which were placed in a 23°C incubator. After 1 h, each plate was removed and placed on a backlight, and an image of each plate was acquired using an iPhone X digital camera.
Data were collected by manually counting the animals in each quadrant using the Fiji ImageJ software. The replicates are discarded if one of the three conditions are met: (1) if the total for all four quadrants is <55 (2); if the highest total quadrant exceeds the sum of the remaining three quadrants; (3) if the quadrant across from the highest total quadrant has fewer than half the animals of any other quadrant (Supplementary Figure 2). Then, the CI is calculated using the following formula, where Qn is the number of worms in the nth quadrant:
A mean CI is calculated from the replicates for each assay that are not discarded.
Differences between cancer risk groups were assessed using the Welch t-test for data sets with unequal variance. Thresholds for cancer risk assessment were drawn to optimize the accuracy of the data analysis; 95% confidence intervals were specified based on the following formula
where C is the confidence interval, t95 is the t-score, s is the sample standard deviation, and n is the sample size.
We developed the N.C.S. Study based on the chemotaxis data that were previously generated showing a slight preference of C. elegans for urine samples acquired from cancer patients, as opposed to a slight aversive response to urine from non-cancer patients (20–22). Our first approach was to determine if the procedure previously used for human cancer detection could be applied to canine urine. While performing chemotaxis, we found that individual replicate outliers could cause drastic swings in the calculated mean CI. For this reason, replicates that deviated strongly from the other replicates in the assay were discarded. We chose to discard CI with a difference greater than 0.25 from the closest other replicates within the assay to reduce the distortion from extreme data points on the mean chemotaxis value. Plates with fewer than 55 worms in the four quadrant boundaries were discarded, as plates with fewer worms tended to yield a wider range of CI values, leading to greater distortions in the mean. We also discarded replicates with unusual distributions (Supplementary Figure 2). We defined plates as yielding outlier results when the total number of worms in one quadrant exceeded the total number in all three other quadrants combined, or when the number of worms across from the highest total quadrant was <50% of the total of any other quadrant. These arrangements indicated migration either toward or away from a particular quadrant rather than a particular chemical stimulus and did not provide reliable data for calculating a mean CI.
Through these optimizations, we developed the N.C.S. Study to accurately identify urine samples from canine cancer patients (Figure 1). Previous chemotaxis assays have used anywhere from three to six replicates to determine the mean CI (20–22). For canine urine assays, we often found variance in the response and magnitude in individual replicates within an assay (Supplementary Table 1). We found that it was necessary to acquire at least five non-discarded replicates for one urine test, and four replicates if all are positive or negative. From the CI replicates which were not discarded, we calculated the mean CI, which was then used to assess the level of risk.
Figure 1. The N.C.S. Study is conducted by placing 75–100 worms on each assay plate. A total of seven assay replicates are conducted, from which a mean CI is calculated and used to assess the level of cancer risk. Adapted from BioRender.
We performed tests on a series of cancer and non-cancer urine samples to determine if cancer can be detected through positive chemotaxis toward canine urine samples. We initially performed assays on a total of eight cancer samples and 14 non-cancer samples. We found that C. elegans was much more strongly attracted to cancerous urine samples than non-cancer samples (Figure 2A). From these results, we set a threshold for elevated cancer risk at CI = 0.038 specified by the upper 99.5 percentile determined from a Student t-distribution of the tested non-cancer samples. The mean CI values less than or equal to this value are classified as “low risk,” as that is the range of about 85% of non-cancer samples, while we designated results above the threshold as “moderate to high cancer risk.” We chose to assess the performance of our assay by calculating accuracy (fraction of total samples that are correctly classified), sensitivity (fraction of positive samples that are correctly classified), and specificity (fraction of negatives samples that are correctly identified). For this initial assay, we achieved an 88% sensitivity for cancer detection, and a 93% specificity for correctly classifying non-cancer samples (Figure 2B, Supplementary Table 2). We also ran replicates of a cancer and non-cancer sample which indicated replicable outcomes of the assay risk classification (Supplementary Table 3). Based on these results, we have shown a preliminary ability to classify the cancer risk of canines through C. elegans chemotaxis.
Figure 2. (A) Mean CI plotted for eight cancer and 14 non-cancer samples for which the N.C.S Study was conducted. A mean CI of 0.099 ± 0.038 for cancer samples versus a mean CI of −0.006 ± 0.032 in non-cancer samples (p = 0.0002). Red line indicates moderate to high cancer risk classification threshold. ***p < 0.001. (B) Levels of cancer risk set at the following range: low risk (<0.038) and moderate to high risk (>0.038).
To further determine the accuracy of the N.C.S. Study at detecting the presence of cancer, we performed assays on 10 additional samples of each of four different types of cancer that are commonly diagnosed in domestic dogs: lymphoma, mast cell tumor, melanoma, and hemangiosarcoma (24) (Figure 3, Supplementary Tables 4, 5). Additionally, we performed assays on samples from 16 more dogs without a confirmed cancer diagnosis (Supplementary Tables 6, 7). We found that all samples yielded a higher mean CI than non-cancer samples. By combining the data acquired from these 40 additional cancer samples and 16 additional non-cancer samples with that in the preliminary data set, we found that the N.C.S. Study yielded a sensitivity of 85% of identifying at least a moderate risk of cancer in each confirmed cancer patient, as compared to the 10% of non-cancer samples identified as at least a moderate cancer risk (Table 1). Overall, by combining all measured CI values for cancer and non-cancer samples, we achieved an accuracy of 87%. Our results also showed a statistically significant difference between the mean CI for each type of cancer and the non-cancer samples (Figure 3).
Figure 3. Average CI for urine samples obtained from lymphoma, mast cell tumor, melanoma, and hemangiosarcoma patients as compared to non-cancer samples. (Lymphoma: 0.079 ± 0.050, mast cell tumor: 0.081 ± 0.059, melanoma: 0.081 ± 0.051, and hemangiosarcoma: 0.077 ± 0.028 vs. non-cancer: −0.014 ± 0.027). Red line indicates moderate to high cancer risk classification threshold. **p < 0.01, ***p < 0.001.
In this study, we assessed how the presence of cancer in canines affects the odor of urine samples as perceived by the nematode C. elegans and to determine how it compares to the detected odor in human cancer and non-cancer patients. Additionally, while we were able to replicate the significantly more attractive chemotaxis response in cancer-positive canine samples, we observed a mean CI across all non-cancer samples that was approximately zero. Urine is composed of a mix of salts, minerals, hormones, and other chemicals, all of which contribute to the chemotaxis response for a sample (25, 26). Thus, the difference in the composition between human and canine urine could yield differing mean chemotaxis responses for non-cancer samples.
Each of the four types of cancer for which we screened 10 samples was detected at a high rate in the N.C.S. Study, with no cancer type having a sensitivity below 70%. We thus observe that the risk of multiple types of cancer can be identified through urine chemotaxis assays. Two of the eight initial cancer assays were performed on samples from patients with soft-tissue sarcoma, a type of cancer which is less prevalent but still occurs in some canines. We found that both samples were detected by chemotaxis assays, showing that it is another type of cancer that potentially can be detected at a high rate using urine chemotaxis assays. While the test can identify the presence of cancer at a high rate, the test does not give any information on which type of cancer a patient has developed. Moreover, a positive result in our test does not constitute a cancer diagnosis, but a warning of a risk that cancer may be present at a certain stage in a canine patient. Further diagnosis and monitoring of symptoms are necessary to confirm the presence of cancer and its type. In addition, it has previously been shown that attractive VOCs are present in the urine during both early and late stages of cancer (20, 22), so it is likely that in future assays, we can demonstrate the ability to screen for cancer at the earliest stages.
In this study, we have measured the effects of cancer on the odor profile of canine urine. It is likely that other biological factors and disease states can affect the perceived odor of a urine sample. It has previously been shown in human cancer patients that the release of hormones at stages of the menstrual cycle can cause a cyclic change in the chemotaxis index. In this study, we only performed the assay on one female dog that was not spayed. Therefore, the hormonal effects on urine odor should further be studied in both male and female dogs. Aside from cancer, inflammation of the respiratory system and inflammatory bowel cause an increase, in particular, in the VOCs in biowaste (27), which could lead to a false positive cancer diagnosis. Moreover, the effects of urinary tract infection (UTI) on C. elegans odor detection are not well-studied and should be investigated further to determine the limits of our assay. We must also assess other factors that differ between the cancer and non-cancer groups. Due to the increased risk of cancer with age, the mean age of the group of cancer patients is higher than the mean age of the set of non-cancer patients. However, there is no clear trend toward either false positive or false negative classifications that are associated with age, suggesting that age alone does not have a significant influence on the odorant profile of urine (Supplementary Figure 3). Additionally, little is known about the effects that cancer treatment and medication might have on the concentration of metabolites or other components in canine urine. These effects can be assessed by performing sequential assays on individual cancer patients at different stages of disease progression and treatment.
The N.C.S. Study yielded performance metrics that fell into the range of accuracies that were previously determined for human cancer samples. The performance metrics were slightly weaker than what was previously measured by Hirotsu et al., who detected several types of cancer from human urine using C. elegans chemotaxis with a sensitivity of 96% and a specificity of 95%, yielding an overall accuracy of 95% (20). However, the performance was comparable to that achieved by Lanza et al. through chemotaxis (accuracy of 86%) (21) and exceeded that achieved by Thompson et al. (accuracy of 70%) (22). Thus, our results lend evidence to the hypothesis that patterns in cancerous VOC signatures that have been well-studied in humans are comparable with that in canines. Since VOCs have also been detected in urine samples from cancerous mice (23), there is strong evidence that similar methods can be utilized to detect cancer in feline, equine, and other mammalian veterinary patients. However, further studies are necessary to determine for which mammalian species this test can be applied, and how effective this method is at distinguishing cancer samples from non-cancer samples.
While we were able to measure a significant difference in chemotaxis toward cancer and non-cancer urine samples, in some instances, we found a high level of variance between individual replicates within an assay. While we have not identified the source of this variation, these results indicate that the locomotion of C. elegans is highly random. However, we also observed that cancer samples producing a strongly positive chemotaxis index will have few if any negative replicates. Nonetheless, assays with a wide range of replicate CI values are more likely to produce different results when repeated. For this reason, by achieving a stronger chemosensory attraction toward cancer urine samples, or by reducing the variance of individual replicates, the accuracy and replicability of cancer detection through odor can be improved. It has previously been shown that a response to positive volatile odorants present in cancerous urine samples can be identified through calcium gradients in the C. elegans AWC sensory neuron (20, 21). This characteristic calcium gradient is indicative of the presence of an attractive odorant and is strongly distinguishable from the low gradient that has been measured for non-cancer samples. Moreover, a much lower noise level is achieved using this method as compared to odor detection through chemotaxis. We hypothesize that by measuring calcium gradients, we could achieve an even higher accuracy of cancer detection from canine urine samples.
In recent years, the number of pet owners in the United States and around the world has undergone a steady increase. This, combined with the strong emotional bonds that owners have with their pets, creates a higher demand than ever for treatments to protect pets from life-threatening illnesses. The collection of urine samples is routine and non-invasive, and the test can be conducted accurately at a high rate in a basic laboratory setting. By detecting more attractive urine chemotaxis in multiple common cancer types, we present evidence toward urine odor as an effective method for cancer screening in canines.
The original contributions presented in the study are included in the article/Supplementary material, further inquiries can be directed to the corresponding authors.
DM grew and maintained stocks of C. elegans and conducted all chemotaxis experiments and was the primary author of the article. CN developed the research idea, assisted in experimental development, and collected urine samples. MHL assisted in experimental design and setup and contributed to the writing and editing of the article. JHK contributed to data interpretation and the writing and editing of the article. All authors contributed to the article and approved the submitted version.
This work was supported by Animal Cancer Dx to CN and DM, and the National Science Foundation (IOS-2132286) to MHL. This publication was supported, in part, by the National Center for Advancing Translational Sciences of the National Institutes of Health under Grant Number UL1TR002733. Strains were provided by the CGC, which is funded by the NIH Office of Research Infrastructure Programs (P40 OD010440).
The content is solely the responsibility of the authors and does not necessarily represent the official views of the National Institutes of Health.
Authors CN and DM were employed by the company Animal Cancer Dx. Author JHK is a shareholder and a member of the scientific advisory board of Animal Cancer Dx.
The remaining authors declare that the research was conducted in the absence of any commercial or financial relationships that could be construed as a potential conflict of interest.
The authors declare that this study received funding from Animal Cancer Dx. The funder had the following involvement in the study: developed the research idea, performed and conducted experiments, and wrote and edited the article.
All claims expressed in this article are solely those of the authors and do not necessarily represent those of their affiliated organizations, or those of the publisher, the editors and the reviewers. Any product that may be evaluated in this article, or claim that may be made by its manufacturer, is not guaranteed or endorsed by the publisher.
The Supplementary Material for this article can be found online at: https://www.frontiersin.org/articles/10.3389/fvets.2022.932474/full#supplementary-material
1. Fleming JM, Creevy KE, Promislow DEL. Mortality in North American dogs from 1984 to 2004: an investigation into age-, size-, and breed-related causes of death. J Vet Intern Med. (2011) 25:187–98. doi: 10.1111/j.1939-1676.2011.0695.x
2. FAQs| Veterinary Cancer Society. Available online at: http://vetcancersociety.org/pet-owners/faqs/ (accessed March 16, 2022).
3. Sánchez D, Cesarman-Maus G, Amador-Molina A, Lizano M. Oncolytic viruses for canine cancer treatment. Cancers. (2018) 10:404. doi: 10.3390/cancers10110404
4. Park JS, Withers SS, Modiano JF, Kent MS, Chen M, Luna JI, et al. Canine cancer immunotherapy studies: linking mouse and human. J Immunother Cancer. (2016) 4:1–11. doi: 10.1186/s40425-016-0200-7
5. Nytko KJ, Thumser-Henner P, Weyland MS, Scheidegger S, Bley CR. Cell line-specific efficacy of thermoradiotherapy in human and canine cancer cells in vitro. PLoS ONE. (2019) 14:e0216744. doi: 10.1371/journal.pone.0216744
6. Horise Y, Maeda M, Konishi Y, Okamoto J, Ikuta S, Okamoto Y, et al. Sonodynamic therapy with anticancer micelles and high-intensity focused ultrasound in treatment of canine cancer. Front Pharmacol. (2019) 10:545. doi: 10.3389/fphar.2019.00545
7. Flory A, Kruglyak KM, Tynan JA, Mclennan LM, Rafalko Id JM, Christian P, et al. Clinical validation of a next-generation sequencing-based multi-cancer early detection “liquid biopsy” blood test in over 1,000 dogs using an independent testing set: the CANcer Detection in Dogs (CANDiD) study. PLoS ONE. (2022) 17:e0266623. doi: 10.1371/journal.pone.0266623
8. Corsi AK. A Transparent window into biology: a primer on Caenorhabditis elegans. WormBook. (2015) 1−31. doi: 10.1895/wormbook.1.177.1 Availabe online at: http://www.wormbook.org/
9. Melkman T, Sengupta P. The worm's sense of smell: development of functional diversity in the chemosensory system of Caenorhabditis elegans. Dev Biol. (2004) 265:302–19. doi: 10.1016/j.ydbio.2003.07.005
11. Spehr M, Munger SD. Olfactory receptors: G protein-coupled receptors and beyond. J Neurochem. (2009) 109:1570–83. doi: 10.1111/j.1471-4159.2009.06085.x
12. Hirotsu, Bio Science Inc,. | HIROTSU バイオサイエンス. Available online at: https://hbio.jp/en/srv/nnose3 (accessed December 31, 2021).
13. Opitz P, Herbarth O. The volatilome - Investigation of volatile organic metabolites (VOM) as potential tumor markers in patients with head and neck squamous cell carcinoma (HNSCC). J Otolaryngol - Head Neck Surg. (2018) 47:1–13. doi: 10.1186/s40463-018-0288-5
14. Gordon SM, Szidon JP, Krotoszynski BK, Gibbons RD, O'Neill HJ. Volatile organic compounds in exhaled air from patients with lung cancer. Clin Chem. (1985) 31:1278–82. doi: 10.1093/clinchem/31.8.1278
15. Heiden MGV, Cantley LC, Thompson CB. Understanding the Warburg effect: The metabolic requirements of cell proliferation. Science (80-). (2009) 324:1029–33. doi: 10.1126/science.1160809
16. Phillips M, Cataneo RN, Ditkoff BA, Fisher P, Greenberg J, Gunawardena R, et al. Prediction of breast cancer using volatile biomarkers in the breath. Breast Cancer Res Treat. (2006) 99:19–21. doi: 10.1007/s10549-006-9176-1
17. Silva CL, Passos M, Cmara JS. Investigation of urinary volatile organic metabolites as potential cancer biomarkers by solid-phase microextraction in combination with gas chromatography-mass spectrometry. Br J Cancer. (2011) 105:1894–904. doi: 10.1038/bjc.2011.437
18. McCulloch M, Jezierski T, Broffman M, Hubbard A, Turner K, Janecki T. Diagnostic accuracy of canine scent detection in early- and late-stage lung and breast cancers. Integr Cancer Ther. (2006) 5:30–9. doi: 10.1177/1534735405285096
19. Matsumura K, Opiekun M, Oka H, Vachani A, Albelda SM, Yamazaki K, et al. Urinary volatile compounds as biomarkers for lung cancer: a proof of principle study using odor signatures in mouse models of lung cancer. PLoS ONE. (2010) 5:e8819. doi: 10.1371/journal.pone.0008819
20. Hirotsu T, Sonoda H, Uozumi T, Shinden Y, Mimori K, Maehara Y, et al. A highly accurate inclusive cancer screening test using Caenorhabditis elegans scent detection. PLoS ONE. (2015) 10:e0118699. doi: 10.1371/journal.pone.0118699
21. Lanza E, Rocco M, Schwartz S, Caprini D, Milanetti E, Ferrarese G, et al. C. elegans-based chemosensation strategy for the early detection of cancer metabolites in urine samples. Sci Rep. (2021) 11:1–16. doi: 10.1038/s41598-021-96613-z
22. Thompson M, Feria NS, Yoshioka A, Tu E, Civitci F, Estes S, et al. A Caenorhabditis elegans behavioral assay distinguishes early stage prostate cancer patient urine from controls. Biol Open. (2021) 10:bio057398. doi: 10.1242/bio.057398
23. Ueda Y, Kawamoto K, Konno M, Noguchi K, Kaifuchi S, Satoh T, et al. Application of C. elegans cancer screening test for the detection of pancreatic tumor in genetically engineered mice. Oncotarget. (2019) 10:5412. doi: 10.18632/oncotarget.27124
24. Common Cancers In Dogs - Flint Animal Cancer Center. Available online at: https://www.csuanimalcancercenter.org/2019/11/14/common-cancers-in-dogs/ (accessed March 31, 2022).
25. Stevenson AE, Markwell PJ. Comparison of urine composition of healthy Labrador retrievers and miniature schnauzers. Am J Vet Res. (2001) 62:1782–6. doi: 10.2460/ajvr.2001.62.1782
26. Bouatra S, Aziat F, Mandal R, Guo AC, Wilson MR, Knox C, et al. The Human Urine Metabolome. PLoS One. (2013) 8:e73076. doi: 10.1371/journal.pone.0073076
27. van Schooten FJ, Boots AW, Smolinska A, Dallinga JW. Volatile organic compounds as exhaled biomarkers of inflammation and oxidative stress in respiratory diseases. In: Ganguly NK, Jindal SK, Biswal S, Barnes PJ, Pawankar R, editors. Studies on Respiratory Disorders. New York, NY: Springer Science and Business Media (2014). p. 67–84. doi: 10.1007/978-1-4939-0497-6_4
Keywords: Caenorhabditis elegans, olfaction, volatile organic compounds, cancer detection, chemotaxis
Citation: Namgong C, Kim JH, Lee MH and Midkiff D (2022) Non-invasive cancer detection in canine urine through Caenorhabditis elegans chemotaxis. Front. Vet. Sci. 9:932474. doi: 10.3389/fvets.2022.932474
Received: 29 April 2022; Accepted: 12 July 2022;
Published: 09 August 2022.
Edited by:
Giuseppe Conte, University of Pisa, ItalyReviewed by:
Enrico Lanza, Sapienza University of Rome, ItalyCopyright © 2022 Namgong, Kim, Lee and Midkiff. This is an open-access article distributed under the terms of the Creative Commons Attribution License (CC BY). The use, distribution or reproduction in other forums is permitted, provided the original author(s) and the copyright owner(s) are credited and that the original publication in this journal is cited, in accordance with accepted academic practice. No use, distribution or reproduction is permitted which does not comply with these terms.
*Correspondence: Chan Namgong, Y2hhbkBhbmltYWxjYW5jZXJkeC5jb20=; Daniel Midkiff, ZGFubnlAYW5pbWFsY2FuY2VyZHguY29t
Disclaimer: All claims expressed in this article are solely those of the authors and do not necessarily represent those of their affiliated organizations, or those of the publisher, the editors and the reviewers. Any product that may be evaluated in this article or claim that may be made by its manufacturer is not guaranteed or endorsed by the publisher.
Research integrity at Frontiers
Learn more about the work of our research integrity team to safeguard the quality of each article we publish.