- 1School of Biological Sciences, University of Bristol, Bristol, United Kingdom
- 2Departamento de Zoología, Facultad de Ciencias Naturales y Oceanográficas, Universidad de Concepción, Concepción, Chile
- 3Departamento de Patología y Medicina Preventiva, Facultad de Ciencias Veterinarias, Universidad de Concepción, Chillán, Chile
- 4Departamento de Ciencias Agrarias, Facultad de Ciencias Agrarias y Forestales, Universidad Católica del Maule, Los Niches, Chile
- 5Facultad de Medicina Veterinaria, Universidad San Sebastián, Concepción, Chile
Few studies have assessed the patterns of parasite populations of rodents over a longitudinal gradient in Chile. In this work, the gastrointestinal helminthic fauna of invasive rodents in Chile was examined to assess the association between their presence/absence and abundance with latitude, host sex, and host body condition, and to assess the coexistence and correlation of the abundance between parasite species. Rodents were obtained from 20 localities between 33 and 43°S. Helminths were extracted from the gastrointestinal tract and identified morphologically. Overall, 13 helminth taxa were obtained. The most frequently identified parasite species was Heterakis spumosa, and the most abundant was Syphacia muris, while Physaloptera sp. was the most widely distributed. No locality presented with a coexistence that was different from that expected by chance, while the abundance of five helminthic species correlated with the abundance of another in at least one locality, most likely due to co-infection rather than interaction. Host sex was associated with parasite presence or abundance, and female sex-biased parasitism was notably observed in all cases. Body condition and latitude presented either a positive or negative association with the presence or abundance of parasites depending on the species. It is notable that the likely native Physaloptera sp. is widely distributed among invasive rodents. Further, gravid females were found, suggesting spillback of this species to the native fauna. The low frequency and abundance of highly zoonotic hymenolepid species suggest that rodents are of low concern regarding gastrointestinal zoonotic helminths.
Introduction
Invasive rodents, mainly Mus musculus, Rattus rattus, and Rattus norvegicus with a worldwide distribution, and Rattus exulans with a Pacific distribution, have been recognized as they perform several roles ecologically (1) and epidemiologically (2). From an ecological standpoint, invasive rodents not only directly impact native communities by predation or competition (3), but they also indirectly impact communities by both introducing allochthones parasites or amplifying native ones (4). From an epidemiological and public health standpoint, rodents are important reservoir hosts of several zoonotic helminths (5–9), and, although several rodents can harbor zoonotic parasites (10–12), most of the reported zoonotic parasites have been found in invasive rodents (Rattus spp. and Mus spp.) (13).
In Chile, some studies have assessed the parasitism of rodents by helminths at the parasite level, at the host population level, and the host community level. Thus, respectively, those studies have described new species (14, 15), component populations, and communities (16, 17), and they have assessed the sharing of parasites among different host populations within a host community (18, 19); however, few have focused merely on the parasites of an invasive rodent species. Existing research has explored parasites in reduced geographical areas (20–22), while another included invasive rodents in a host community-level study (19). Studies focused on zoonotic helminths in invasive rodents mostly focused on a single parasite species, Trichinella spiralis (23, 24), even though many zoonotic helminths that were reported in rodents in Chile are parasites introduced with invasive rodents (13).
Studies focused on the ecology of helminths in these invasive rodents have assessed the sharing of helminths with native rodents (19). Little information is available about what happens within component communities, and no studies have examined the factors affecting the presence and abundance of these parasites. It is known that the coexistence of parasite infrapopulations could lead to either increased loads (synergism) or reduced loads (antagonism), given that different causes lead to direct interaction or immune-mediated interactions (25). Although the coexistence or lack of coexistence can be caused by interactions, they can be also caused by similar or different infection routes, respectively, among other factors (26), and their study is worthy. Thus, the objective of this study was to describe the gastrointestinal helminthic fauna of introduced rodents in Chile along a latitudinal gradient, to assess the association between the occurrence (presence/absence) of parasites and their abundance with latitude, and to host sex and host body condition. Further, this study also assessed the coexistence and association between the abundance of parasite species.
Materials and Methods
The Rattus rattus (n = 159), R. norvegicus (n = 30), and Mus musculus (n = 91) included in this study were obtained from previous studies, which had other objectives (19, 24, 27, 28); the trapping and euthanasia by an overdose of anesthesia of those rodents were described in those articles. In addition to ethic and/or legal authorizations provided for these articles, new samplings were approved by the Comité de Bioética of the Facultad de Ciencias Veterinarias (CBE-34-2019) of the Universidad de Concepción. Several localities were included in the study (Table 1), most of which were visited once; however, some localities were visited two or three times. Therefore, we defined a study unit (SU) as a set of host specimens obtained from a single locality over <31 days. Thus, two SUs from the same locality were not expected to represent the same parasite community given the expected temporal variation of the presence and abundance of parasites (29). A total of 27 SUs were studied between the Coquimbo and Los Lagos regions (see the localities in Figure 1). The gastrointestinal tract was examined for the presence of helminths under a stereomicroscope. Nematodes were cleared with lactophenol or ethanol–glycerin, and cestodes were stained with carmine–HCl, and they were identified under a light microscope following the keys of Anderson et al. (30) and Khalil et al. (31).
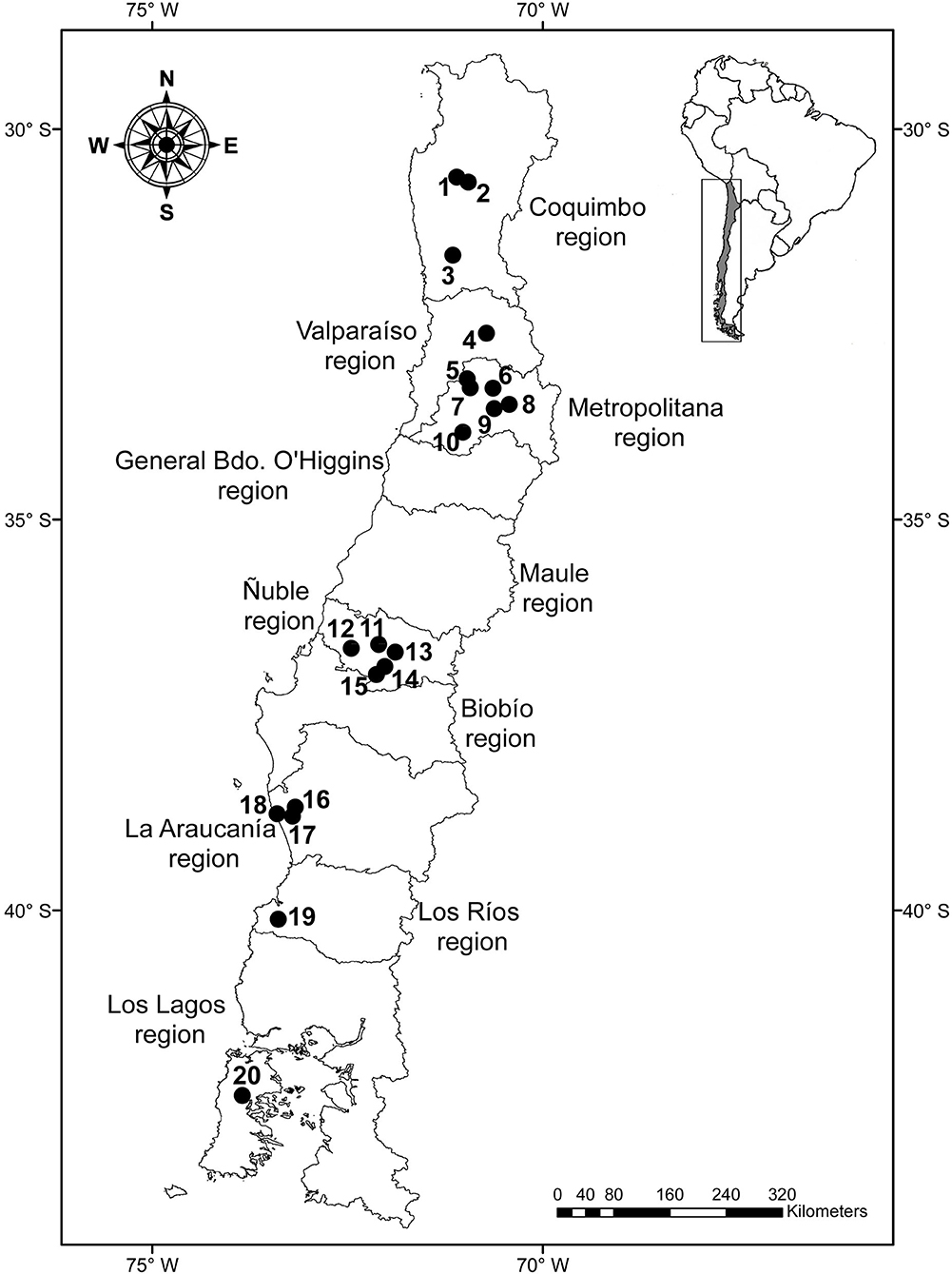
Figure 1. Map of Chile with the localities included in the study: 1 = Sotaquí, 2 = Monte Patria, 3 = Illapel, 4 = Putaendo, 5 = Maipú 1, 6 = San Ramón, 7 = Maipú 2, 8 = La Pintana, 9 = Calera de Tango, 10 = Talagante, 11 = Chillán, 12 = Nueva Aldea, 13 = Pinto, 14 = El Carmen, 15 = Pemuco, 16 = Carahue, 17 = Collico, 18 = Puerto Saavedra, 19 = Alerce Costero National Park, and 20 = Castro.
The prevalence (95% confidence interval [CI] with the Clopper–Pearson method), mean abundance (95% CI estimated by the bootstrapping method) (32), variance-to-mean range (V-M), Poulin's discrepancy index (D), and k parameter from a negative binomial distribution (33) were estimated by parasite population within the host species and SU. Species richness was estimated with the Chao2 method (34) by SU when the presence of frequent and rare species enabled the estimation of confidence intervals. Confidence intervals were obtained using the Quantitative Parasitology online application, QPweb (34).
Patterns of species co-occurrence at the component community, defining it at the SU level, were also examined. For this, we used the EcoSim version 7.0 (35) software to test for non-random patterns of species co-occurrence for each locality with the C-score index (36). We selected SUs that had at least 12 hosts with at least three parasite species with two of them presenting prevalence higher than 20%. The C-score measures the average number of “checkerboard units,” i.e., no co-occurrence, among all possible species pairs. We used the fixed–fixed model with 5,000 iterations. When the observed C-score index is higher than the simulated C-score, it indicates that the helminth species co-occur less frequently than by chance. Conversely, an observed C-score lower than the simulated score suggests that the helminth species co-occur more frequently than expected by chance. On the other hand, if the observed C-scores do not differ statistically from the simulated C-scores, then random patterns of species co-occurrence are observed (37). In addition, given that the abundance of parasites reflects the number of infection events, two species that co-occur are expected to have correlated abundance; therefore, we also tested whether the abundance of a species was associated with the abundance of the other species, between pairs of parasite species within SUs with more than 10 hosts, using Spearman correlation tests.
Finally, we assessed the association between the occurrence (presence/absence) and abundance of parasite species with the latitude, body condition, and sex of the host using multifactorial logistic and binomial negative regressions, respectively. In the case of the variable sex, “female” was considered as a basal level and “male” as a dummy variable, latitude was measured as degree and decimals, and body condition was calculated with Fulton's index (K = mass/lenght3). To select the best model, we began with the full model containing the three independent variables. The P-value of the variables was only considered as a criterion that was used to select the variable to remove in each step, with the variable with the highest P-value being the variable to remove. Likelihood ratio tests (LR tests) were used to choose the best model, where the removal of any variable implied a significant loss of likelihood and took place with a model P-value of ≤ 0.05. In addition, in the case of logistic regressions, goodness-of-fit (GOF) tests were performed to assess the selected models. If the observed parasite presence did not fit the expected model, the variable was not considered to be associated with the occurrence. Regressions, LR tests, GOF tests and, correlation tests were performed using Stata/BE 17 (StataCorp LLC). The significance level of the models and tests was P = 0.05. Only significant associations are reported in the results.
Results
Overall, the observed species richness was 13 species, and the estimated richness was 14 (95% CI: 14.1–27.1). The taxa found herein were: Heterakis spumosa, Syphacia obvelata, Syphacia muris, Aspiculuris tetraptera, Trichuris muris, Nippostrongylus brasiliensis, Physaloptera sp., Protospirura sp., Pterygodermatites (Paucipectines) sp., Gongylonema neoplasticum, Capillariidae, Hymenolepis diminuta, and Rodentolepis nana. The most frequently found parasite among examined animals was H. spumosa with 25.4% (CI 19.4–32.2. Mean abundance: 3.03; CI 1.91–5.13), identified among Rattus spp. hosts and was found in 12 SUs. Syphacia muris was the second most frequently found with a 17.5% (CI 12.3–23.6) prevalence rate among Rattus spp., but it presented the highest mean abundance among Rattus sp. (21.9; 9.6–50.6) and was present in 14 SUs. Physaloptera sp., with an overall 9.6% prevalence rate and 0.225 mean abundance, was the most widely distributed species, present in 17 SUs, including the northernmost and southernmost studied localities. Conversely, the rarest taxa were A. tetraptera and Capillariidae, which were found in only four individuals each. When considering parasites with an aggregation index measured in more than one SU, S. muris presented the highest average V-M = 197, with a maximum of 520 in a single SU, followed by H. spumosa which presented an average V-M = 14.6 with a maximum of 33.9. Conversely, Physaloptera sp. presented the highest average D-index, 0.85 (maximum 0.93), followed by S. muris, 0.82 (max. 0.85). Syphacia obvelata presented the lowest k parameter, 0.11, followed by Physaloptera sp., 0.21; however, this parameter was not obtained in many samples given that the maximum likelihood estimate of k could not be computed, which may be due to a lack of fitness with the negative binomial distribution given the small local sample size or its very low local prevalence. The abundances by host species and SU are given in Table 1. The details of the prevalence, mean abundance, and aggregation indices by host species and SU, as well as the estimated richness by SU, are given in Supplementary Table 1.
We analyzed the co-occurrence between parasite species in three-component communities, which showed a C-score similar to that expected by chance, indicating that the parasite species co-occur as frequently as expected (Supplementary Table 2).
On the other hand, among 45 pairwise correlation tests of abundance between parasite populations, only five significant associations were found, all of which were positive: H. spumosa–S. muris (ρ = 0.45; P = 0.04), H. spumosa–N. brasiliensis (ρ = 0.55; P = 0.01) and S. muris–N. brasiliensis (ρ = 0.6; P < 0.01) in Carahue; and H. spumosa–N. brasiliensis (ρ = 0.63; P = 0.02) and Physaloptera sp.–Protospirura sp. (ρ = 0.73; P < 0.01) in Pinto. The details of all pairwise tests with their ρ and P-values are given in Supplementary Table 3.
Finally, sex was associated with the presence of H. spumosa [odds ratio (OR) = 0.37], N. brasiliensis (OR = 0.16), and H. diminuta (OR =0.67) and was associated with the abundance of T. muris (coefficient = −3.88), N. brasiliensis (coef. = −5.18), and Physaloptera sp. (coef. = −1.36); the presence was more frequent and/or the abundance was higher in females than in males in all cases. Body condition was negatively associated with the occurrence of H. spumosa (OR = 0.67) and Physaloptera sp. (OR = 0.41), negatively associated with the abundance of H. spumosa (coef. = −1.2) and positively associated with the abundance of S. obvelata (coef. = 1.83). Latitude was positively associated with the occurrence (OR = 1.21) and abundance (coef. = 0.47) of H. spumosa, and negatively associated with the occurrence of H. diminuta (OR = 0.67) and the abundance of Physaloptera sp. (coef. = −0.32) and Capillariidae (coef. = −1.62). The details of the selected models are given in Supplementary Tables 4–13. When the P-value of the variable was higher than 0.05, the LR-test output that the remotion of the variable caused a significant loss of likelihood. In each selected model, the likelihood of the model was significantly higher than the null model (see the P-value of the log-likelihood).
Discussion
A total of 13 gastrointestinal helminth species were found; however, the richness estimation suggests that additional work is necessary to better determine parasite richness and to identify all parasite species inhabiting the gastrointestinal tracts of rats and mice in Chile. Two of the helminth species reported herein, H. diminuta and R. nana, have been frequently reported to infect humans elsewhere (38–40); however, they were found with low frequency and abundance in the present study. Other zoonotic species, S. obvelata, and S. muris, were more prevalent and abundant in their hosts, M. musculus and Rattus spp., respectively, but there are only a few reports of them infecting humans (41, 42). Finally, T. muris has also been seldomly reported to infect humans, and was scarcely found in this study. Thus, the results suggest that, regarding helminthic infections, invasive rodents in Chile are of a minor, but not null, concern from a public health standpoint since their cycles are maintained by these invasive rodents. This aligns with the lack of reports of Syphacia infection in Chilean people coupled with the decreasing prevalence of hymenolepids (43).
In addition to the five mentioned helminthic species, H. spumosa, A. tetraptera, N. brasiliensis, and G. neoplasticum have been reportedly found in Rattus spp. and M. musculus elsewhere in both laboratory and feral specimens (8, 42, 44–47). The enemy release hypothesis states that invasive animals present with fewer parasite species than in their original territory (48) given the small sample size of translocated animals, the loss of parasites during the translocation process, and/or the adaptation of the parasite to the new territory (49). Thus, the number of parasite species reported herein is larger than expected since it is similar to the richness reported in the Palearctic (50, 51), but higher than in other invaded territories (52). This suggests that there have been several introduction processes, i.e., processes of translocation of rodents from overseas to Chile, that favor the sampling of different parasite species in each introduction process. The low prevalence and abundance of many parasite species may favor the process of invasion by these allochthones rodents. Conversely, some parasites reported herein have not been reported in Rattus spp. and Mus musculus with worldwide distribution; rather, they seem to be native parasites from Neotropical rodents. Physaloptera sp. and Protospirura sp. are parasite genera frequently reported in native rodents in Chile (16, 19, 53, 54), and in both cases, gravid females were found, which supports the hypothesis that there is a spillback of parasites (55–57); however, new temporal studies are necessary to determine whether the parasitic loads increase in native rodent populations after the arrival of invasive rodents. In the case of Pterygodermatites sp., although the species was not identified given the low availability of males worms, species found in native rodents in Chile (19) are morphologically different from that reported herein, at least in terms of the distance of the first cuticular projection and the anterior end, as well as and the number of cuticular projections, suggesting that it could be a co-introduced species. Pterygodermatites spp. have been reported previously in Rattus spp. in Taiwan (58) and Thailand (46), with P. tani and P. whartoni being the reported species.
The positive associations between parasites agree with the fact that they have similar cycles. Thus, S. muris, H. spumosa, and N. brasiliensis, which co-abound in Carahue, have a direct cycle, suggesting that the correlation of their abundance could be due to similar transmission methods. On the other hand, Physaloptera sp. and Protospirura sp. have an indirect cycle, with Orthoptera, Coleoptera, and Dictyoptera insects being reported as intermediates hosts of Physaloptera sp. (59), and Dermaptera insects being reported as intermediate hosts of Protospirura sp. (60). In addition, both helminthic taxa seem to have native rodents as part of their reservoir, insofar as their coexistence with native rodents and their predatory behavior on insects are factors that favor the infection of both parasites by the same host. Therefore, although correlated abundances do not seem to be the general rule, which agrees with the lack of significant co-occurrence, the results suggest that if they do exist, they can result from co-infection rather than interspecies interactions between parasite species.
Sex is reportedly a significant factor affecting the presence and abundance of parasites; however, female sex-biased parasitism is rare in the literature (61), as males are usually more likely to be parasitized than females (29, 62–64). Some factors, such as pregnancy and lactation, have been mentioned in the literature as weakening the resistance of female mammals to parasitic infection (65–67); however, in this study, the pregnancy or lactation conditions of female rodents were not recorded transversally, and this factor could not be assessed. Behavioral factors, such as feeding, have also been assessed, with female bank voles more likely to be exposed to spirurid parasites given their higher proportion of invertebrate animal consumption (61, 68). However, this differential behavior should be assessed in invasive rats, and might only explain the association between sex, the presence of H. diminuta, and the abundance of Physaloptera sp., which are transmitted by invertebrate intermediate hosts.
There were no common patterns of variation related to the body condition-associated parasitism, as most cases were not significantly associated. Significant associations may have been because the larger host's body could offer more resources to the parasite (69), that larger hosts could have offered greater opportunities for parasite infection (70) (positive association) or that parasitism could result in damage to the host (negative association) (70, 71). It is not easy to explain that the body condition is positively associated with the infection with S. obvelata and negatively associated with the infection with H. spumosa, given that both parasites present a direct cycle. Thus, results suggest that H. spumosa is more pathogenic than S. obvelata. However, this difference could be influenced by the host, since S. obvelata is mainly a parasite of M. musculus while H. spumosa parasitize mainly Rattus spp. Further studies are necessary to test these hypotheses. On the other hand, the negative association of Physaloptera sp. infection with the body condition suggests a damage to the host by the parasite, which is in agreement with previous records in other species (72, 73).
Heterakis spumosa was more prevalent and abundant in the south, which suggests that cold and humid climates favor the persistence of infecting stages of this parasite in the environment. Conversely, H. diminuta and Physaloptera sp. were more prevalent or abundant to the north, which suggests that warmer or drier climates favor their intermediates hosts. Capillariidae was also more abundant to the north, but further studies are needed to determine the species and the life cycle of this taxa to understand this association. The contrasting associations between latitude and parasitic rates suggest that the persistence of free-living parasites, or the intermediate host stages of parasites, could explain these associations. More studies assessing these hypotheses are necessary to establish a cause.
Conclusion
In this work, we reported 13 gastrointestinal helminth species of R. rattus, R. norvegicus, and M. musculus. Although some of these parasites reportedly infect humans, the low prevalence and abundance of these parasites suggest that they are of low concern for public health. The presence of gravid females of native parasites in invasive rodents supports the spillback hypothesis, but more studies are needed to test this hypothesis. Coinfection and correlated abundances are not frequent among helminth communities of rodents. The host's sex was the factor that is most frequently associated with parasitism, notably with female sex-biased parasitism observed in all cases.
Data Availability Statement
The original contributions presented in the study are included in the article/Supplementary Material, further inquiries can be directed to the corresponding author.
Ethics Statement
The animal study was reviewed and approved by Comité de Bioética of the Facultad de Ciencias Veterinarias.
Author Contributions
CG-T, FF-S, AG-O, and CL-A examined the sample and mounted and identified parasites. JB-V, JS-R, BB-G, FL-C, HE-R, MS, AH, LM, and CL-A caught and processed the rodents. LM and CL-A designed the study and analyzed the data. CL-A wrote the first manuscript draft. All authors revised and expanded upon the original draft and approved the submitted manuscript.
Funding
This study was funded by the Fondo Nacional de Desarrollo Científico y Tecnológico (ANID/FONDECYT Grant Nos. 11170294 and 11150875) and the Vicerrectoría de Investigación y Desarrollo of the Universidad de Concepción (Grant No. 220.113.099).
Conflict of Interest
The authors declare that the research was conducted in the absence of any commercial or financial relationships that could be construed as a potential conflict of interest.
Publisher's Note
All claims expressed in this article are solely those of the authors and do not necessarily represent those of their affiliated organizations, or those of the publisher, the editors and the reviewers. Any product that may be evaluated in this article, or claim that may be made by its manufacturer, is not guaranteed or endorsed by the publisher.
Acknowledgments
The authors thank Juana Correa, Juan Ramírez, Claudio Yáñez-Crisóstomo, Javiera Muñoz-Galaz, Gonzalo Torres, Nicole Lizama, and Mario Espinoza Carniglia for their fieldwork support.
Supplementary Material
The Supplementary Material for this article can be found online at: https://www.frontiersin.org/articles/10.3389/fvets.2022.929208/full#supplementary-material
References
1. St Clair JJH. The impacts of invasive rodents on island invertebrates. Biol Conserv. (2011) 144:68–81. doi: 10.1016/j.biocon.2010.10.006
2. Bordes F, Blasdell K, Morand S. Transmission ecology of rodent-borne diseases: new frontiers. Integr Zool. (2015) 10:424–35. doi: 10.1111/1749-4877.12149
3. Banks PB, Hughes NK, A. review of the evidence for potential impacts of black rats (Rattus rattus) on wildlife and humans in Australia. Wildlife Res. (2012) 39:78–88. doi: 10.1071/WR11086
4. Morand S, Bordes F, Chen H-W, Claude J, Cosson J-F, Galan M, et al. Global parasite and Rattus rodent invasions: the consequences for rodent-borne diseases. Integr Zool. (2015) 10:409–23. doi: 10.1111/1749-4877.12143
5. Panti-May JA, Servían A, Ferrari W, Zonta ML, Hernández-Mena DI, Hernández-Betancourt SF, et al. Morphological and molecular identification of hymenolepidid cestodes in children and synanthropic rodents from rural Mexico. Parasitol Int. (2020) 75:102042. doi: 10.1016/j.parint.2019.102042
6. Fitte B, Cavia R, Robles MDR, Dellarupe A, Unzaga JM, Navone GT. Predictors of parasite and pathogen infections in urban rodents of central Argentina. J Helminthol. (2021) 95:523. doi: 10.1017/S0022149X21000523
7. Fitte B, Robles MR, Dellarupe A, Unzaga JM, Navone GT. Hymenolepis diminuta and Rodentolepis nana (Hymenolepididae: Cyclophyllidea) in urban rodents of Gran La Plata: association with socio-environmental conditions. J Helminthol. (2018) 92:549–53. doi: 10.1017/S0022149X17000864
8. Galán-Puchades MT, Sanxis-Furió J, Pascual J, Bueno-Marí R, Franco S, Peracho V, et al. First survey on zoonotic helminthosis in urban brown rats (Rattus norvegicus) in Spain and associated public health considerations. Vet Parasitol. (2018) 259:49–52. doi: 10.1016/j.vetpar.2018.06.023
9. Souza FN, Aguiar Santos M, Almeida Alves D, Cecília Vieira De Melo L, Jessé Gonçalves Da Mota D, Cristina Pertile A, et al. Angiostrongylus cantonensis in urban populations of terrestrial gastropods and rats in an impoverished region of Brazil. Parasitology. (2021) 148:994–1002. doi: 10.1017/S0031182021000597
10. Dick TA, Pozio E. Tichinella spp. and trichinellosis. In: WM Samuel, MJ Pybus, AA Kocan, editors, Parasitic Diseases of Wild Mammals, 2nd ed. (Ames, IA: Iowa State University Press), (2000). p. 380–96. doi: 10.1002/9780470377000.ch15
11. Fugassa MH. Updated checklist of helminths found in terrestrial mammals of Argentine Patagonia. J Helminthol. (2020) 94:e170. doi: 10.1017/S0022149X20000462
12. Palmeirim M, Bordes F, Chaisiri K, Siribat P, Ribas A, Morand S. Helminth parasite species richness in rodents from Southeast Asia: role of host species and habitat. Parasitol Res. (2014) 113:3713–26. doi: 10.1007/s00436-014-4036-0
13. Landaeta-Aqueveque C, Moreno Salas L, Henríquez A, Silva-de la Fuente MC, González-Acuña D. Parasites of native and invasive rodents in Chile: ecological and human health needs. Front Vet Sci. (2021) 8:643742. doi: 10.3389/fvets.2021.643742
14. Babero BB, Murua RB. A new species of whipworm from a south american hystricomorph rodent. Memórias do Instituto Oswaldo Cruz. (1990) 85:211–3. doi: 10.1590/S0074-02761990000200012
15. Digiani MC, Landaeta-Aqueveque C, Serrano PC, Notarnicola J. Pudicinae (Nematoda: Heligmonellidae) parasitic in endemic chilean rodents (Caviomorpha: Octodontidae and Abrocomidae): description of a new species and emended description of Pudica degusi (Babero and Cattan) n. comb. J Parasitol. (2017) 103:736–46. doi: 10.1645/17-81
16. Landaeta-Aqueveque C, Robles MdR, Cattan PE. Helmintofauna del roedor Abrothrix olivaceus (Sigmodontinae) en áreas sub-urbanas de Santiago de Chile. Parasitología Latinoamericana. (2007) 62:134–41. doi: 10.4067/S0717-77122007000200006
17. Babero BB, Cabello C, Kinard JE. Helmintofauna de Chile. V. Parásitos del Coipo, Myocastor coypus (Molina, 1782). Boletin Chileno de parasitologia. (1979) 34:26–31.
18. Cattan PE, Núñez H, Yáñez J. Comunidades de parásitos en roedores: una comparación entre octodóntidos y cricétidos. Boletín del Museo Nacional de Historia Natural, Chile. (1992) 43:93–103.
19. Landaeta-Aqueveque C, Robles MdR, Henríquez A, Yáñez-Meza A, Correa JP, González-Acuña D, et al. Phylogenetic and ecological factors affecting the sharing of helminths between native and introduced rodents in Central Chile. Parasitology. (2018) 145:1570–6. doi: 10.1017/S0031182018000446
20. Landaeta-Aqueveque C, Robles MDR, Cattan PE. The community of gastrointestinal helminths in the housemouse, Mus musculus, in Santiago, Chile. Parasitología Latinoamericana. (2007) 62:165–9. doi: 10.4067/S0717-77122007000200010
21. Ruiz del Río A. Contribución al estudio de las enfermedades parasitarias humanas transmitidas por las ratas en Concepción. Boletín de la Sociedad de Biología de Concepción. (1939) 13:47-82.
22. Torres P, Lopetegui O, Gallardo M. Investigaciones sobre algunos nematodos parásitos de Rattus norvegicus y Ctenomys maulinus de Chile. Boletín Chileno de Parasitología. (1976) 31:39–42.
23. Schenone H, Jacob C, Rojas A, Villarrel F. Infección por Trichinella spiralis en Rattus norvegicus capturados en el Matadero Municipal de Santiago. Boletín Chileno de Parasitología. (1967) 22:176.
24. Espinoza-Rojas H, Lobos-Chávez F, Silva-de la Fuente MC, Echeverry DM, Muñoz-Galaz J, Yáñez-Crisóstomo C, et al. Survey of Trichinella in American minks (Neovison vison Schreber, 1777) and wild rodents (Muridae and Cricetidae) in Chile. Zoonoses Public Health. (2021) 68:842–8. doi: 10.1111/zph.12845
25. Karvonen A, Jokela J, Laine A-L. Importance of sequence and timing in parasite coinfections. Trends Parasitol. (2019) 35:109–18. doi: 10.1016/j.pt.2018.11.007
26. Haukisalmi V, Henttonen H. Coexistence in helminths of the bank vole Clethrionomys glareolus. I. Patterns of co-occurrence. J Anim Ecol. (1993) 62:221–9. doi: 10.2307/5353
27. Moreno Salas L, Espinoza-Carniglia M, Lizama Schmeisser N, Torres LG, Silva-de la Fuente MC, Lareschi M, et al. Fleas of black rats (Rattus rattus) as reservoir host of Bartonella spp. in Chile. PeerJ. (2019) 7:e7371. doi: 10.7717/peerj.7371
28. Moreno-Salas L, Espinoza-Carniglia M, Lizama-Schmeisser N, Torres-Fuentes LG, Silva-de La Fuente MC, Lareschi M, et al. Molecular detection of Rickettsia in fleas from micromammals in Chile. Parasites Vectors. (2020) 13:523. doi: 10.1186/s13071-020-04388-5
29. Veloso-Frias J, Silva-De La Fuente MC, Rubio AV, Moreno L, González-Acuña D, Simonetti JA, et al. Variation in the prevalence and abundance of mites parasitizing Abrothrix olivacea (Rodentia) in the ive forest and Pinus radiata plantations in central Chile. Hystrix Italian J Mammal. (2019) 30:107–11. doi: 10.4404/hystrix-00171-2019
30. Anderson RC, Chabaud AG, Willmontt S. Keys to the Nematode Parasites of Vertebrates. Wallingford: CAB International (2009). doi: 10.1079/9781845935726.0000
31. Khalil LF, Jones A, Bray TA. Keys to the Cestode Parasites of Vertebrates. Wallingford: CAB International (1994).
32. Bush AO, Lafferty KD, Lotz JM, Shostak AW. Parasitology meets ecology on its own terms: Margolis et al. Revisited. J Parasitol. (1997) 83:575–83. doi: 10.2307/3284227
33. Poulin R. Evolutionary Ecology of Parasites. Princeton, NJ: Princeton University Press (2007). doi: 10.1515/9781400840809
34. Reiczigel J, Marozzi M, Fábián I, Rózsa L. Biostatistics for parasitologists – a primer to quantitative parasitology. Trends Parasitol. (2019) 35:277–81. doi: 10.1016/j.pt.2019.01.003
35. Gotelli NJ, Entsminger GL. EcoSim: Null Models Software for Ecology, Version 7.0. (2001). Available online at: http://homepages.together.net/gentsmin/ecosim.htm (accessed April 19, 2022).
36. Stone L, Roberts A. The checkerboard score and species distributions. Oecologia. (1990) 85:74–9. doi: 10.1007/BF00317345
37. Gotelli N. Null model analysis of species co-occurrence ptterns. Ecology. (2000) 81:2606–21. doi: 10.1890/0012-9658(2000)081[2606:NMAOSC]2.0.CO;2
38. Panti-May JA, Rodríguez-Vivas RI, García-Prieto L, Servián A, Costa F. Worldwide overview of human infections with Hymenolepis diminuta. Parasitol Res. (2020) 119:1997–2004. doi: 10.1007/s00436-020-06663-x
39. Goudarzi F, Mohtasebi S, Teimouri A, Yimam Y, Heydarian P, Salehi Sangani G, et al. A systematic review and meta-analysis of Hymenolepis nana in human and rodent hosts in Iran: a remaining public health concern. Comp Immunol Microbiol Infect Dis. (2021) 74:101580. doi: 10.1016/j.cimid.2020.101580
40. Pérez-Chacón G, Pocaterra LA, Rojas E, Hernán A, Jiménez JC, Núñez L. Coinfection with Hymenolepis nana, Hymenolepis diminuta, Giardia intestinalis, and human immunodeficiency virus: a case report with complex immunologic interactions. Am J Trop Med Hyg. (2017) 2017:16-0413. doi: 10.4269/ajtmh.16-0413
41. Riley WA. A mouse oxyurid, Syphacia obvelata, as a parasite of man. J Parasitol. (1919) 6:89–93. doi: 10.2307/3270899
42. Stone WB, Manwell RD. Potential helminth infections in humans from pet or laboratory mice and hamsters. Public Health Rep. (1966) 81:647–53. doi: 10.2307/4592796
43. Vidal FS, Toloza ML, Cancino FB. Evolución de la prevalencia de enteroparasitosis en la ciudad de Talca, Región del Maule, Chile. Revista chilena de infectología. (2010) 27:336–40. doi: 10.4067/S0716-10182010000500009
44. Robles MdR, Navone GT, Gómez Villafañe IE. New morphological details and first records of Heterakis spumosa and Syphacia muris from Argentina. Comp Parasitol. (2008) 75:145–9. doi: 10.1654/4277.1
45. Panti-May JA, Palomo-Arjona EE, Gurubel-González YM, Barrientos-Medina RC, Digiani MC, Robles MR, et al. Patterns of helminth infections in Rattus rattus and Mus musculus from two Mayan communities in Mexico. J Helminthol. (2019) 94:e30. doi: 10.1017/S0022149X19000063
46. Paladsing Y, Boonsri K, Saesim W, Changsap B, Thaenkham U, Kosoltanapiwat N, et al. Helminth fauna of small mammals from public parks and urban areas in Bangkok Metropolitan with emphasis on community ecology of infection in synanthropic rodents. Parasitol Res. (2020) 119:3675–90. doi: 10.1007/s00436-020-06897-9
47. Diagne C, Granjon L, Tatard C, Ribas A, Ndiaye A, Kane M, et al. Same invasion, different routes: helminth assemblages may favor the invasion success of the house mouse in senegal. (2021) 8. doi: 10.3389/fvets.2021.740617
48. Torchin ME, Lafferty KD, Dobson AP, McKenzie VJ, Kuris AM. Introduced species and their missing parasites. Nature. (2003) 421:628–30. doi: 10.1038/nature01346
49. MacLeod CJ, Paterson AM, Tompkins DM, Duncan RP. Parasites lost – do invaders miss the boat or drown on arrival? Ecol Lett. (2010) 13:516–27. doi: 10.1111/j.1461-0248.2010.01446.x
50. Milazzo C, Gouy de Bellocq J, Cagnin M, Casanova J-C, Bella C, Feliu C, et al. Helminths and ectoparasites of Rattus rattus and Mus musculus from Sicily, Italy. Comp Parasitol. (2003) 70:199–204. doi: 10.1654/4109.1
51. Pakdel N, Naem S, Rezaei F, Chalehchaleh A-A. A survey on helminthic infection in mice (Mus musculus) and rats (Rattus norvegicus and Rattus rattus) in Kermanshah, Iran. Vet Res For. (2013) 4:105–9. Available online at: http://vrf.iranjournals.ir/article_2674.html
52. Pisanu B, Chapuis JL, Durette-Desset MC. Helminths from introduced small mammals on Kerguelen, Crozet, and Amsterdam Islands (Southern Indian Ocean). J Parasitol. (2001) 87:1205–8. doi: 10.1645/0022-3395(2001)087[1205:HFISMO]2.0.CO;2
53. Riquelme M, Salgado R, Simonetti JA, Landaeta-Aqueveque C, Fredes F, Rubio AV. Intestinal helminths in wild rodents from native forest and exotic pine plantations (Pinus radiata) in Central Chile. Animals. (2021) 11:384. doi: 10.3390/ani11020384
54. Sutton C. Contribution to the knowledge of Argentina's parasitological fauna XVII. Spirurida (Nematoda) from Neotropical Cricetidae: Physaloptera calnuensis n sp and Protospirura numidica criceticola Quentin, Karimi and Rodríguez De Almeida Bulletin Du Museum National D'histoire Naturelle, Paris, 4° Série. (1989) 11:61–7.
55. Carolus H, Muzarabani KC, Hammoud C, Schols R, Volckaert FAM, Barson M, et al. A cascade of biological invasions and parasite spillback in man-made Lake Kariba. Sci Tot Environ. (2019) 659:1283–92. doi: 10.1016/j.scitotenv.2018.12.307
56. Kelly DW, Paterson RA, Townsend CR, Poulin R, Tompkins DM. Parasite spillback: a neglected concept in invasion ecology? Ecology. (2009) 90:2047–56. doi: 10.1890/08-1085.1
57. Mastitsky SE, Veres JK. Field evidence for a parasite spillback caused by exotic mollusc Dreissena polymorpha in an invaded lake. Parasitol Res. (2010) 106:667–75. doi: 10.1007/s00436-010-1730-4
58. Hasegawa H, Kobayashi J, Otsuru M. Helminth parasites collected from Rattus rattus on Lanyu, Taiwan. J Helminthol Soc Washington. (1994)61:95–102.
59. Schell SC. Studies on the life cycle of Physaloptera hispida schell (Nematoda: Spiruroidea) a parasite of the cotton rat (Sigmodon hispidus littoralis Chapman). J Parasitol. (1952) 38:462. doi: 10.2307/3273926
60. Quentin JC. Cycle biologique de Protospirura muricola Gedoelst, 1916 Nematoda Spiruridae. Ann Parasitologie Humaine et Comparée. (1969) 44:485–503. doi: 10.1051/parasite/1969444485
61. Grzybek M, Bajer A, Behnke-Borowczyk J, Al-Sarraf M, Behnke JM. Female host sex-biased parasitism with the rodent stomach nematode Mastophorus muris in wild bank voles (Myodes glareolus). Parasitol Res. (2015) 114:523–33. doi: 10.1007/s00436-014-4214-0
62. Froeschke G, Van der Mescht L, McGeoch M, Matthee S. Life history strategy influences parasite responses to habitat fragmentation. Int J Parasitol. (2013) 43:1109–18. doi: 10.1016/j.ijpara.2013.07.003
63. Morand S, De Bellocq JG, Stanko M, Miklisová D. Is sex-biased ectoparasitism related to sexual size dimorphism in small mammals of Central Europe? Parasitology. (2004) 129:505–10. doi: 10.1017/S0031182004005840
64. Poulin R. Helminth growth in vertebrate hosts: does host sex matter? Int J Parasitol. (1996) 26:1311–5. doi: 10.1016/S0020-7519(96)00108-7
65. Connan RM. The effect of host lactation on the self-cure of Nippostrongylus brasiliensis in rats. Parasitology. (1970) 61:27–33. doi: 10.1017/S0031182000040816
66. Dineen JK, Kelly JD. The suppression of rejection of Nippostrongylus brasiliensis in lactating rats: the nature of the immunological defect. Immunology. (1972) 22:1–12.
67. Lloyd S. Effect of pregnancy and lactation upon infection. Vet Immunol Immunopathol. (1983) 4:153–76. doi: 10.1016/0165-2427(83)90057-0
68. Gebczyńska Z. Food habits of the bank vole and phenological phases of plants in an oak hornbeam forest. Acta Theriol. (1976) 21:223–36. doi: 10.4098/AT.arch.76-21
69. Van Der Mescht L, Le Roux PC, Matthee S. Remnant fragments within an agricultural matrix enhance conditions for a rodent host and its fleas. Parasitology. (2013) 140:368–77. doi: 10.1017/S0031182012001692
70. Sánchez CA, Becker DJ, Teitelbaum CS, Barriga P, Brown LM, Majewska AA, et al. On the relationship between body condition and parasite infection in wildlife: a review and meta-analysis. Ecol Lett. (2018) 21:1869–84. doi: 10.1111/ele.13160
71. Giorgi MS, Arlettaz R, Christe P, Vogel P. The energetic grooming costs imposed by a parasitic mite (Spinturnix myoti) upon its bat host (Myotis myotis). Proc Royal Soc Lond Ser B. (2001) 268:2071–5. doi: 10.1098/rspb.2001.1686
72. Naem S, Farshid AA, Marand VT. Pathological findings on natural infection with Physaloptera praeputialis in cats. Vet Arh. (2006) 79:315–21. Available online at: https://hrcak.srce.hr/5140
Keywords: coinfection, helminthiasis, invasive rodents, mice, rats, sex-biased parasitism, spillback, rodent diseases
Citation: Grandón-Ojeda A, Moreno L, Garcés-Tapia C, Figueroa-Sandoval F, Beltrán-Venegas J, Serrano-Reyes J, Bustamante-Garrido B, Lobos-Chávez F, Espinoza-Rojas H, Silva-de la Fuente MC, Henríquez A and Landaeta-Aqueveque C (2022) Patterns of Gastrointestinal Helminth Infections in Rattus rattus, Rattus norvegicus, and Mus musculus in Chile. Front. Vet. Sci. 9:929208. doi: 10.3389/fvets.2022.929208
Received: 26 April 2022; Accepted: 30 May 2022;
Published: 28 June 2022.
Edited by:
Raquel Simões, Universidade Federal Rural do Rio de Janeiro, BrazilReviewed by:
Thiago Cardoso, Oswaldo Cruz Foundation (FIOCRUZ), BrazilAna Paula Gomes, Federal University of Rio de Janeiro, Brazil
Copyright © 2022 Grandón-Ojeda, Moreno, Garcés-Tapia, Figueroa-Sandoval, Beltrán-Venegas, Serrano-Reyes, Bustamante-Garrido, Lobos-Chávez, Espinoza-Rojas, Silva-de la Fuente, Henríquez and Landaeta-Aqueveque. This is an open-access article distributed under the terms of the Creative Commons Attribution License (CC BY). The use, distribution or reproduction in other forums is permitted, provided the original author(s) and the copyright owner(s) are credited and that the original publication in this journal is cited, in accordance with accepted academic practice. No use, distribution or reproduction is permitted which does not comply with these terms.
*Correspondence: Carlos Landaeta-Aqueveque, clandaeta@udec.cl
†These authors share first authorship