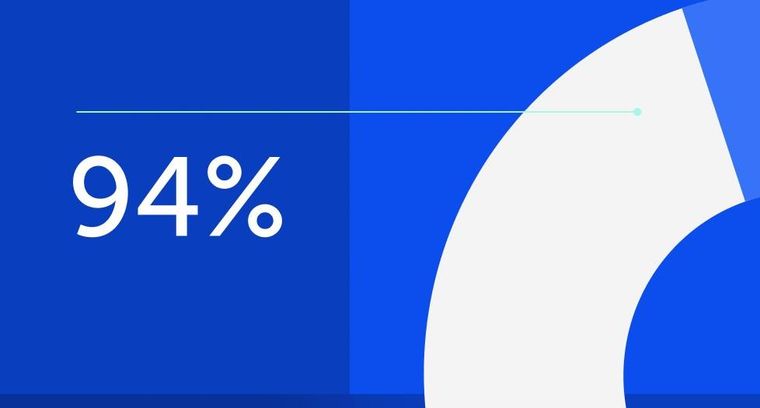
94% of researchers rate our articles as excellent or good
Learn more about the work of our research integrity team to safeguard the quality of each article we publish.
Find out more
ORIGINAL RESEARCH article
Front. Vet. Sci., 26 July 2022
Sec. Veterinary Infectious Diseases
Volume 9 - 2022 | https://doi.org/10.3389/fvets.2022.922867
In this study, we screened adjuvants for an inactivated vaccine against Erysipelothrix rhusiopathiae (E. rhusiopathiae). Inactivated cells of E. rhusiopathiae strain HG-1 were prepared as the antigen in five adjuvanted inactivated vaccines, including a mineral-oil-adjuvanted vaccine (Oli vaccine), aluminum-hydroxide-gel-adjuvanted vaccine (Alh vaccine), ISA201-biphasic-oil-emulsion-adjuvanted vaccine (ISA201 vaccine), GEL02-water-soluble-polymer-adjuvanted vaccine (GEL vaccine), and IMS1313-water-soluble-nanoparticle-adjuvanted vaccine (IMS1313 vaccine). The safety test results of subcutaneous inoculation in mice showed that Oli vaccine had the most severe side effects, with a combined score of 35, followed by the ISA201 vaccine (25 points), Alh vaccine (20 points), GEL vaccine (10 points), and IMS1313 vaccine (10 points). A dose of 1.5LD50 of strain HG-1 was used to challenge the mice intraperitoneally, 14 days after their second immunization. The protective efficacy of Oli vaccine and Alh vaccine was 100% (8/8), whereas that of the other three adjuvanted vaccines was 88% (7/8). Challenge with 2.5LD50 of strain HG-1 resulted in a 100% survival rate, demonstrating the 100% protective efficacy of the Oli vaccine, followed by the GEL vaccine (71%, 5/7), IMS1313 vaccine (57%, 4/7), ISA201 vaccine (43%, 3/7), and Alh vaccine (29%, 2/7). Challenge with 4LD50 of strain HG-1 showed 100% (7/7) protective efficacy of the Oli vaccine and 71% (5/7) protective efficacy of the GEL vaccine, whereas the protective efficacy of other three adjuvanted vaccine was 14% (1/7). The Alh and GEL vaccines were selected for comparative tests in piglets, and both caused minor side effects. A second immunization with these two adjuvanted vaccines conferred 60 and 100% protective efficacy, respectively, after the piglets were challenged via an ear vein with 8LD100 of strain HG-1. After challenge with 16LD100 of strain HG-1, the Alh and GEL vaccines showed 40% and 100% protective efficacy, respectively. Our results suggested that GEL is the optimal adjuvant for an inactivated vaccine against E. rhusiopathiae.
E. rhusiopathiae is a Gram-positive rod-shaped nonspore-forming bacterium with a capsule but no flagellum (1), belonging to the genus Erysipelothrix in the family Erysipelothrichaceae. The bacterium is widely distributed in nature, causing diseases in a wide range of animals, including mammals, birds and fish (2–5). Humans can be infected via trauma that results in skin lesions, thus acquiring erysipelas (6). In swine, E. rhusiopathiae causes swine erysipelas, an acute, febrile contagious disease. Its main clinical manifestations include acute sepsis, subacute exanthematous endocarditis, and chronic endocarditis (2, 7). Based on heat-stable cell wall antigen, Erysipelothrix spp. strains can be divided into at least 28 serotypes (types 1a, 1b, 2-26, and N) (2, 8, 9). Strains of E. rhusiopathiae were determined as belonging to serovars 1a, 1b, 2, 4–6, 8, 9, 11, 12, 15–17, 19, 21, 23, and N (7, 10). The serotypes display distinct virulence, and types 1a and 1b are more virulent than the other serotypes (2, 11). So far, types 1 and 2 are the most strongly epidemic serotypes in pigs (7, 10, 12–15).
In the 1980–1990s, erysipelas was widespread and caused great economic losses in the swine industries of North America, Europe, Asia, and Australia (2, 7). This disease was also one of the three major infectious diseases (swine fever, swine erysipelas, and swine pasteurellosis) that threatened the swine industry in China. However, after the introduction of antibiotics, vaccines, and large-scale farming, swine erysipelas was considered to have disappeared. However, since 2010, swine erysipelas outbreaks have occurred again in the United States, Japan, Brazil, China, and other countries (14–19). E. rhusiopathiae vaccines are generally considered effective in preventing erysipelas. Traditionally, inactivated and live attenuated vaccines of E. rhusiopathiae serovar 1a or 2 isolates are still used today on a global basis (10). Additionally, some researchers identified several key immunogenic surface proteins of E. rhusiopathiae include surface protective antigen A (SpaA) (20), choline binding protein B (CbpB), rhusiopathiae surface protein A (RspA), and Glyceraldehyde 3-phosphate dehydrogenase (GAPDH) (21). Among them, single SpaA protein can protect immunized animals against lethal dose challenge of E. rhusiopathiae, which has potential as a candidate protein of E. rhusiopathiae subunit vaccine (20–24). Commercial erysipelas bacterins are still widely used today, and the inactivated vaccine preparation technology is mature and low in cost.
To optimize the immune effects of an inactivated vaccine for erysipelas, the selection of the appropriate adjuvant is essential, which a good adjuvant enhances the immune response to the antigen, and thus the immune effects of the vaccine (25, 26). Aluminum hydroxide adjuvant and oil adjuvant, known as the conventional and commercial adjuvant, are widely used in veterinary vaccines globally (27, 28). In recent years, various new adjuvants have appeared, Montanide™ adjuvants are a well-established brand of vaccine adjuvants used in veterinary vaccines. Montanide™ ISA201 is a water-in-oil-in-water (W/O/W) mineral oil-based adjuvant emulsion inside (29). The main component is highly refined light mineral oil, and it also contains small amounts of plant-derived mannitol and oleic acid (30, 31). The vaccine prepared with this adjuvant had a different water/oil dosage form (W/O) from that of the Oli vaccine, which reduced the viscosity of the vaccine, improved its injectability, and reduced its side effects, while maintaining the antigen delivery capacity of a mineral oil adjuvant. Montanide™ IMS 1313 VG N is a ready-to-dilute adjuvants, consisting of a water-dispersed liquid nanoparticles combined with an immunostimulating compound. This adjuvant can induce a rapid immune response with a strong sustainability especially in case of two-shot vaccination regimen (32). Montanide™ GEL, a new adjuvant based on the dispersion of polymeric gel in water, has the advantages of a high antigen load, high stability, and easy emulsification (33–35). Due to polymer adsorption properties, this adjuvant improves the recruitment of the innate immune system, which provide a significant enhancement of the immune response in aqueous vaccines (36).
In previous research, we compared the efficacy of several novel adjuvants in the Gram-negative bacteria Glaesserella parasuis trivalent inactivated vaccine. In this study, the HG-1 strain of type 1a E. rhusiopathiae was isolated from diseased swine in Guangdong Province in June 2018, and we aimed to validate the efficacy of novel adjuvant ISA201, IMS1313 and GEL in the Gram-positive bacteria E. rhusiopathiae inactivated vaccine using the newly isolated strain against the current epidemic strain. In addition, two traditional adjuvants, aluminum gel and mineral oil, were used as controls to screen for the optimal inactivated vaccine for swine erysipelas.
The HG-1 strain of type 1a E. rhusiopathiae was isolated from diseased swine in Guangdong Province by the Veterinary and Biological Products Engineering Laboratory of Henan University of Science and Technology (Luoyang, China) in June 2018. According to Reed-Muench method (37), the 50% lethal dose (LD50) of this strain in 10–12-week-old BALB/c mice was 218 colony-forming units (CFU). Meanwhile, piglets were challenged with different doses of HG-1 strain, the 100% lethal dose (LD100) in 8–9-week-old healthy susceptible piglets was 1.0 ×104 CFU. Specific-pathogen-free female BALB/c mice (6–8 weeks old) were purchased from Charles River Inc. (Beijing, China), and 4–5-week-old healthy susceptible piglets were purchased from farmers in Yichuan County, Henan Province. Healthy susceptible piglets were defined as piglets from groups with no outbreak of erysipelas in the preceding 2 years, with no clinical symptoms of swine erysipelas, and that had not been vaccinated for swine erysipelas. The sera of the healthy piglets also tested negative for types 1 and 2 E. rhusiopathiae in an enzyme-linked immunosorbent assay (ELISA) (38). All animal experiments in this study were performed in strict accordance with the recommendations delineated in the guidelines of the Animal Care and Use Committee of Henan University of Science and Technology (No. 20200610002).
Tryptic soy agar (TSA) and tryptic soy broth (TSB) were purchased from Becton Dickinson Inc. (USA). Mycoplasma-free newborn bovine calf serum was purchased from TianHang Biotechnology Co, Ltd (Zhejiang, China). Sodium chloride and other reagents were purchased from Yili Reagents Co., Ltd (Beijing, China). The mineral oil adjuvant Marcol-52 (Oli) was purchased from ExxonMobil Corp. (USA); the aluminum hydroxide gel adjuvant (Alh) was purchased from General Chemical Corp. (USA); Montanide™ ISA201 VG biphasic oil emulsion adjuvant (ISA201), Montanide™ GEL02 PR soluble polymer adjuvant (GEL), and Montanide™ IMS 1313 VG N soluble nanoparticles (IMS1313) were produced by Seppic (Paris, France). ELISA reagents were purchased from Sangon Biotech (Shanghai, China).
E. rhusiopathiae strain HG-1 was inoculated with TSA medium containing 10% newborn bovine serum and incubated at 37°C for 24–48 h. Single colonies were picked and cultured in TSB medium containing 10% newborn bovine serum. The TSB liquid medium was then shaken at 37°C at 200 r/min for 16 h, and then diluted 1:100 with TSB medium containing 10% newborn bovine serum and shaken for 16 h at 37°C. The numbers of E. rhusiopathiae cells were enumerated by plate counts at 12 h. Formaldehyde (0.3% v/v) was added to the bacterial suspension, which was inactivated at 37°C for 48 h. The fully inactivated bacterial suspension was centrifuged and the supernatant discarded, and resuspended with sterile phosphate-buffered saline (PBS, 0.01 mol/L, pH 7.2), that is, HG-1 inactivated antigen was obtained. The inactivated Oli and Alh vaccines were prepared with previously reported methods (33, 39), with a 2:1 ratio of oil adjuvant to HG-1 antigen in oil vaccine preparation, and a 1:4 ratio of Alh to HG-1 antigen in Alh vaccine. The ISA201 vaccine, IMS1313 vaccine, and GEL vaccine were prepared according to their respective adjuvant manufacturer's instructions. Briefly, HG-1 antigen and ISA201 were pre-warmed separately in a water bath (31°C) for 30 min, and then HG-1 antigen was dropped into ISA201 slowly to a final ratio of 1:1 (w/w), with gentle shaking, as ISA201 vaccine. IMS1313 was dropped into HG-1 antigen to a final ratio of 1:1 (v/v) slowly, with gentle shaking, as IMS1313 vaccine. GEL 02 was slowly dropped into HG-1 antigen at a final ratio of 1:4 (v/v) and gently shaken, as GEL vaccine. The formulations were incubated for 30 min at room temperature with constant shaking. The final killed whole-cell vaccine (3.0 × 109 killed cells/mL) was stored at 4°C for further analysis.
Thirty BALB/c mice were randomly divided into six groups (five mice per group), and the five adjuvanted vaccines (Oli vaccine, Alh vaccine, GEL vaccine, ISA201 vaccine, and IMS1313 vaccine) were used to vaccinate the five groups via subcutaneous injection on the back (0.2 mL per mouse). One control group of five mice was injected with sterile PBS (0.2 mL per mouse). The mice were observed and recorded for 14 days after vaccination. The safety assessment criteria include: 1. death; 2. clinical symptoms including depression, unkempt fur, appetite, vomiting, swelling at the injection site, and diarrhea, which lasts for at least 3 days; meanwhile, necropsied to check for inflammation, necrosis, and vaccine residue at the injection site. If none of the above symptoms occur, no points will be awarded. One point for mild symptoms; two points for moderate symptoms; three points for severe symptoms. A comprehensive score was calculated according to the intensity of the clinical symptoms and lesion indicators to determine the severity of the side effects in the vaccinated mice.
One hundred thirty-two mice were randomly divided into three groups: 48 in group 1 and 42 each in groups 2 and 3. Each group was randomly divided into six subgroups: subgroups 1–5 were vaccinated subcutaneously with one of the five adjuvanted vaccines and subgroup 6 was injected with sterile PBS (0.2 mL per mouse) as the control. A second vaccination was administered 21 days after the primary vaccination. Blood samples were collected from the mouse tail veins and the serum separated before vaccination and after the primary and secondary vaccinations, and were used to the test antibody levels with an ELISA (23). Fourteen days after the secondary vaccination (35 days after the primary vaccination), all three groups of mice were injected intraperitoneally with 1.5LD50, 2.5LD50, or 4LD50 (LD50 is 218 CFU) of strain HG-1. Their clinical symptoms, morbidity, and death were observed and recorded for 14 days. The dead mice were necropsied and the bacteria were isolated and identified.
Based on the results of the test described in sections 2.4 and 2.5, the Alh vaccine and GEL vaccine for E. rhusiopathiae were selected for further safety testing in piglets. Fifteen piglets were randomly divided into three groups (five per group), and the piglets in groups 1 and 2 were vaccinated with double-strength Alh vaccine or GEL vaccine via an intramuscular injection in the neck (4 mL each). The blank control group was injected with sterile PBS (4 mL each). The clinical symptoms of the piglets, including their mental status, appetite, and death, were recorded for 14 days. The piglets in each group were necropsied to check for inflammation, necrosis, and vaccine residue at the injection site.
Thirty piglets were randomly divided into six groups (five per group). Groups 1 and 2 were injected in the neck with 2 mL of Alh vaccine; groups 3 and 4 were injected in the neck with 2 mL of GEL vaccine; groups 5 and 6 were injected with sterile PBS (2 mL) as the control groups. A second immunization was performed 21 days later with the same dose. Neck vein blood samples were collected from each piglet before and after the primary immunization and after the secondary immunization. The sera were separated from the blood samples to test their antibody levels with an ELISA. Fourteen days after the second immunization, 8LD100 (8.2 × 104 CFU) of strain HG-1 (1.0 mL) was injected into the ear veins of the piglets in groups 1, 3, and 5, and 16LD100 (1.6 × 105 CFU) of strain HG-1 (1.0 mL) was injected into the ear veins of the piglets in groups 2, 4, and 6. Morbidity and mortality were observed and recorded for 14 days. The sick judgment criteria are as follows: 1. death. 2. clinical symptoms including depression, lethargy, pyrexia, inappetence, dyspnea, joint swelling, lameness, lying down, and characteristic pink, red, or purple raised firm rhomboid or squared “diamond skin” lesions, which lasts for at least 3 days; meanwhile, autopsy showed systemic septicemia, including lymph nodes, spleen and lung enlargement and congested, petechiae and ecchymosis may be found in the renal cortex and heart (epicardium and atrial myocardium). In addition, serosanguinous effusion in the joint cavity is also observed, and the joint capsule is often hyperemic. The dead piglets were necropsied for bacterial identification.
The data were analyzed with SPSS version 25.0. Serum antibody levels were analyzed with a t-test (Student's t-test) and one-way analysis of variance (ANOVA). Multiple comparisons were evaluated with Duncan's method. The protective efficacy of vaccine was tested by chi-square test. P < 0.05 was considered to indicate significance, and P < 0.01 to indicate extreme significance.
We recorded the mental state, appetite, vomiting, diarrhea, and death in mice during the safety test (Table 1), and any inflammation, necrosis, or vaccine residue at the injection site (Figure 1). The results showed that the Oli vaccine caused serious side effects in mice, including messy fur and swelling and necrosis at the injection site, whereas the ISA201 and Alh vaccines caused less-serious side effects. ISA201 mainly caused local irritation and therefore messy hair, and the Alh vaccine mainly caused nodules and vaccine residue. The GEL and IMS1313 vaccines caused only minor side effects.
Figure 1. Autopsy changes in mice immunized with differently adjuvanted vaccines. (A) indicates the mice immunized with Oli vaccine; (B) indicates Alh vaccine; (C) indicates ISA201 vaccine; (D) indicates GEL vaccine, (E) indicates IMS1313 vaccine; and (F) indicates PBS control group. The black arrows in the figure showed foci of inflammation, and white arrows showed an inflamed, fluid-filled cyst.
The ELISA results for the immunized mice (Figure 2) showed that, 21 days after the first immunization, the serum antibody levels of mice vaccinated with the ISA201 vaccine, Alh vaccine, GEL vaccine, IMS1313 vaccine, or Oli vaccine were extremely significantly higher than that before immunization and control group (P < 0.01). At 14 days after the secondary immunization, the antibody levels in the mice of the Oli vaccine group were significantly increased (P < 0.05), whereas those in the mice of the other four groups were not (P > 0.05), compared with that of the corresponding immunization group after the first immunization. The serum antibody level of Oli vaccine group was significantly higher than that of Alh vaccine group or ISA201 vaccine group (P < 0.05), but there were no significant differences among other groups (P > 0.05). These results indicated that all five adjuvanted vaccines induced high levels of antibodies in mice. However, after the second immunization, none of the adjuvanted vaccines, except the Oli vaccine, resulted in significantly increased antibody levels.
Figure 2. Antibody titers in mice immunized with the five differently adjuvanted vaccines. Blood samples were collected before vaccination and after the primary and secondary vaccinations. “ISA201 vaccine”, “Alh vaccine”, “IMS1313 vaccine”, “GEL vaccine” and “Oli vaccine” indicate that mice were subcutaneously immunized with the corresponding vaccine, respectively. “PBS control” indicates mice inoculated with sterile PBS buffer. The E. rhusiopathiae-specific antibody titers in the experimental and control groups were measured with an indirect ELISA and recorded at OD450. The error bars represent the SD.
Mice were challenged with strain HG-1 at three different doses, 1.5LD50, 2.5LD50 and 4LD50, 14 days after the boost vaccination (Table 2). With the increase of challenge dose, the protective effect of Alh, ISA201 and IMS1313 vaccine groups declined, particularly when the challenge dose of strain HG-1 was 4LD50, the protective rate of the three vaccines was only 14% (P > 0.05) compared with the control group. However, compared with the corresponding control group at the same challenge dose, the protective rates of Oil (P < 0.05) and GEL vaccine (P < 0.05) groups were 100% when challenged with 1.5LD50 and 2.5LD50, and 100% and 71% when challenged with 4LD50, respectively. In addition, there was no difference in the protection rate between the Oil group and GEL group at any same challenge dose (Table 2). Autopsy of dead mice showed the typical pathological changes associated with sepsis.
Piglets were vaccinated against E. rhusiopathiae with an intramuscular injection in the neck of the double-strength Alh vaccine or double-strength GEL vaccine (4 mL). There were no notable changes in the mental state or appetite of the piglets vaccinated with either vaccine. There were no marked differences in diarrhea, vomiting, or death between the vaccinated groups and the PBS-injected control group. The vaccinated piglets showed a transient increase in body temperature, but the average temperature increase did not exceed 1°C at 24 h after vaccination, and returned to normal within 48 h. Observation of the injection sites on the piglets showed no obvious local inflammation, tissue lesions, vaccine residue, or granulomas in the piglets vaccinated with either vaccine. Autopsies showed no notable differences between the vaccinated and the control groups (Figure 3). These results demonstrated the adequate safety of the two vaccines for piglets.
Figure 3. Vaccine residues in piglets immunized with differently adjuvanted vaccine. (A) represents GEL vaccine; (B) represents Alh vaccine; (C) represents PBS control group. After the observation period, the piglets were dissected to observe the intramuscular injection site of neck, and there was no visible difference between the two vaccine groups and the control group.
The ELISA results for the immunized piglets (Figure 4) showed that, 21 days after the primary immunization, the serum antibody levels in the Alh-vaccinated and GEL-vaccinated groups were extremely significantly higher than that before immunization and control group (P < 0.01). However, 14 days after the secondary immunization, the serum antibody levels were not significantly higher than those after the primary vaccination (P > 0.05). There was also no significant difference between the two immunized groups (P > 0.05). This indicated that the two adjuvanted vaccines induced increased antibody levels after immunization, but no significant increase was observed after the second immunization.
Figure 4. Antibody titers of piglets immunized with the two adjuvanted vaccines. Blood samples were collected before each immunization and before challenge. “Alh vaccine”, “GEL vaccine” and “PBS control” respectively indicate that piglets were subcutaneously immunized with the corresponding vaccine. The E. rhusiopathiae-specific antibody titers in the experimental and control groups were measured with an indirect ELISA and recorded at OD450. The error bars represent the SD.
After the primary and secondary immunization of piglets with the GEL or Alh vaccine, we used 8LD100 or 16LD100 of the highly toxic HG-1 strain to challenge them via an ear vein injection (Table 3). After the 8LD100 challenge, the piglets in the control group showed disease within 48 h, which manifested as clinical symptoms including depression, loss of appetite, joint swelling, lameness and mucosal cyanosis. Three deaths occurred within 4 days of challenge. All piglets in GEL and Alh vaccine groups survived, but two piglets in Alh vaccine group were seriously ill (Table 3). After challenge with 16LD100, all piglets in the control group had succumbed to disease by 36 h after challenge, and all died within 4 days of challenge. Three piglets in Alh vaccine group showed clear clinical symptoms, and two were dead within 14 days (Table 3). Therefore, the protection rate of the Alh vaccine group was 60%. All the piglets in GEL vaccine group survived, and only two showed transient depression and loss of appetite, and fully recovered within 24 h. Therefore, the protection rate of the GEL vaccine was 100%. Autopsies were performed immediately on the dead piglets, and all showed typical septic pathological changes. E. rhusiopathiae was isolated from the organs and tissues of the dead piglets, including the heart, liver, spleen, lung, kidney, brain, lymph nodes, large intestine, and small intestine.
Vaccination is the key to preventing swine erysipelas. For bacterins various adjuvant are added to provide longer immunity. Aluminum hydroxide, mineral oil, ISA201, GEL, and IMS1313 adjuvants, low-cost and enhancement of immune response, have been widely used in animal vaccine research. According to adjuvant manufacturer's instructions, the cost of different adjuvants from low to high is mineral oil which is 0.067 /mL using 66.7% adjuvant in bacterin preparation, Alh adjuvant which is 0.09
/mL using 20% in bacterin preparation, ISA201 adjuvant (0.09
/mL, 50%), IMS1313 adjuvant (0.25
/mL, 50%), and GEL adjuvant (0.26
/mL, 20% adjuvant for vaccine preparation), respectively. Traditional adjuvants have advantages in vaccine preparation costs, but the cost of novel adjuvants is acceptable. The safety and efficacy of vaccines are important indicators of their quality, and appropriate adjuvants are critical to the safety and efficacy of swine erysipelas vaccines.
Our study has shown that the Oli-adjuvanted vaccine induced the highest antibody titers in immunized mice and afforded complete (100%) protection against E. rhusiopathiae. However, this adjuvant also caused serious side effects, including messy fur, swelling, necrosis, and vaccine residue at the injection site (Table 1). This is consistent with the side effects observed in piglets immunized with the Oli vaccine, which included loss of appetite, slow growth, inflammation and necrosis at injection site, and granuloma (33, 40). Mineral oil adjuvants are gradually being replaced by a variety of other novel adjuvants in biological products for swine.
The ISA201 adjuvant is a new type of water/oil/water (W/O/W) dual-phase oil emulsion adjuvant, which has recently been widely used in a variety of animal vaccines (41–43). However, in this study, although ISA201 showed better safety in mice than traditional mineral oil adjuvants, its immune efficacy was significantly lower. The IMS1313 adjuvant is a water-soluble nanoadjuvant developed in recent years. Vaccines prepared with this adjuvant provided good protection in the low dose challenge experiment, but poor immunization efficacy in the high dose challenge experiment.
GEL is a new type of water-soluble polymer adjuvant, the main component of which is polyethylene acrylic acid sodium. The excellent effects of the GEL adjuvant have been verified in vaccines against several viruses, including an inactivated Porcine circovirus vaccine (44), an inactivated Porcine reproductive and respiratory syndrome virus vaccine (35), an inactivated equine influenza virus vaccine (45), a live attenuated porcine epidemic encephalitis vaccine (34), and a Bovine herpesvirus DNA vaccine (46). However, this adjuvant showed a poor adjuvant effect in an inactivated sheep paratuberculosis vaccine (30), a Rhodococcusequi subunit vaccine (47), and a bovine mite disease subunit vaccine (48). There have been few reports of GEL-adjuvanted bacterial vaccines. In our previous study of adjuvants for an G. parasuis vaccine, the GEL adjuvant showed markedly better safety than a mineral oil adjuvant and 100% protective efficacy, and was also more efficacious than other adjuvants, including aluminum gel, IMS1313, and ISA760 (33, 49, 50).
In this study, we compared the effects of different adjuvants on an inactivated vaccine against Gram-positive E. rhusiopathiae in swine. In tests on mice, the GEL adjuvant was safe, induced high antibody levels and provided better protection than the conventional aluminum hydroxide adjuvants and the novel ISA201 and IMS1313 adjuvants. Because an aluminum gel adjuvant was used with most conventional inactivated swine erysipelas vaccines, we also compared the GEL adjuvant with the Alh adjuvant in piglets. Our results showed the excellent safety of both adjuvants, but the GEL adjuvant conferred greater protection (100%). The Alh vaccine showed a 60% protection rate. The antibody levels induced by the Alh vaccine and GEL vaccine after the second immunization were similar, but conferred different rates of protection in the challenge experiment. This may be attributable to we only measured the total IgG antibody levels in this study, but the aluminum gel adjuvant mainly stimulates the Th2 reaction and thus the humoral immune response, which the corresponding antibodies produced are mostly IgG1 (28, 51, 52). The GEL adjuvant is a new polymer adjuvant that has been used in recent years. Recent studies have shown that it not only induces humoral immunity, but also significantly promotes T-lymphocyte proliferation and differentiation, resulting in high-level cellular immunity (34, 44). Therefore, the two vaccines may cause different immune responses. In addition, it is puzzling that after booster immunization, the serum antibody levels of piglets and mice (except Oli vaccine) immunized group did not increase significantly compared with that after the first immunization, which needs further analysis.
Our results indicated that the GEL adjuvant not only showed great efficacy and a higher protection rate when used in an inactivated vaccine for Gram-negative G. parasuis (33), but also has great potential utility in inactivated bacterial vaccines against Gram-positive E. rhusiopathiae.
In this study, we used the type 1a HG-1 strain of E. rhusiopathiae as the antigen to construct an inactivated vaccine against erysipelas with five different adjuvants, and tested the safety and immunization efficacy of the five differently adjuvanted vaccines in mice. This preliminary evaluation suggested that the GEL adjuvant was an excellent adjuvant, with good immunoprotective efficacy. When the GEL vaccine and the conventional Alh vaccine were used to immunize piglets, the GEL vaccine exerted fewer side effects and conferred markedly better immune protection than the Alh vaccine. This study provides strong evidence upon which to base the development of new inactivated vaccines against swine erysipelas.
The original contributions presented in the study are included in the article/supplementary material, further inquiries can be directed to the corresponding author.
All procedures performed in studies involving animals were approved by the Animal Care and Use Committee of Henan University of Science and Technology (No. 20200610002).
L-JG, S-XP, and Z-QZ conceived and designed the study. L-JG and S-XP performed the experiments. J-JS, P-FZ, and Y-NH analyzed the data. L-JG, S-XP, YX, KD, and Z-QZ wrote and revised the manuscript. All authors read and approved the final version.
This work was supported by grants from the National Natural Science Foundation of China (U1704117 and 32072899).
The authors thank all staff at Laboratory of Veterinary Biologics Engineering for providing support.
The authors declare that the research was conducted in the absence of any commercial or financial relationships that could be construed as a potential conflict of interest.
All claims expressed in this article are solely those of the authors and do not necessarily represent those of their affiliated organizations, or those of the publisher, the editors and the reviewers. Any product that may be evaluated in this article, or claim that may be made by its manufacturer, is not guaranteed or endorsed by the publisher.
1. Brooke CJ, Riley TV. Erysipelothrix rhusiopathiae: bacteriology, epidemiology and clinical manifestations of an occupational pathogen. J Med Microbiol. (1999) 48:789–799. doi: 10.1099/00222615-48-9-789
2. Wang Q, Chang BJ, Riley TV. Erysipelothrix rhusiopathiae. Vet Microbiol. (2010) 405–417. doi: 10.1016/j.vetmic.2009.08.012
3. Fiorito CD, Bentancor A, Lombardo D, Bertellotti M. Erysipelothrix rhusiopathiae isolated from gullinflicted wounds in southern right whale calves. Dis Aquat Organ. (2016) 121:67–73. doi: 10.3354/dao03041
4. Ersdal C, Jørgensen HJ, Lie KI. Acute and Chronic Erysipelothrix rhusiopathiae Infection in Lambs. Vet Pathol. (2015) 52:635–43. doi: 10.1177/0300985814556187
5. Janßen T, Voss M, Kühl M, Semmler T, Philipp HC, Ewers C, et al. combinational approach of multilocus sequence typing and other molecular typing methods in unravelling the epidemiology of Erysipelothrix rhusiopathiae strains from poultry and mammals. Vet Res. (2015) 46:1–15. doi: 10.1186/s13567-015-0216-x
6. Upapan P. Human erysipelothrix rhusiopathiae infection: Unsolved issues and possible solutions. J Med Assoc Thail. (2015) 98(Suppl. 9)S170–6.
7. McNeil M, Gerber PF, Thomson J, Williamson S, Opriessnig T. Serotypes and Spa types of Erysipelothrix rhusiopathiae isolates from British pigs (1987 to 2015). Vet J. (2017) 225:13–5. doi: 10.1016/j.tvjl.2017.04.012
8. Wood RL, Haubrich DR, Harrington R. Isolation of previously unreported serotypes of Erysipelothrix rhusiopathiae from swine. Am J Vet Res. (1978) 39:1958–61.
9. Opriessnig T, Wood RL. Erysipelas. In: Zimmerman JJ, Karricker LA, Ramirez A, Schwartz KJ, Stevenson GW, editors. Diseases of Swine. IA: Wiley-Blackwell (2012). p. 835–43.
10. Opriessnig T, Forde T, Shimoji Y. Erysipelothrix Spp.: past, present, and future directions in vaccine research. Front Vet Sci. (2020) 7:174. doi: 10.3389/fvets.2020.00174
11. Ogawa Y, Shiraiwa K, Nishikawa S, Eguchi M, Shimoji Y. Identification of the chromosomal region essential for serovarspecific antigen and virulence of serovar 1 and 2 strains of Erysipelothrix rhusiopathiae. Infect Immun. (2018) 86:e00324–18. doi: 10.1128/IAI.00324-18
12. Imada Y, Takase A, Kikuma R, Iwamaru Y, Akachi S, Hayakawa Y. Serotyping of 800 strains of Erysipelothrix isolated from pigs affected with erysipelas and discrimination of attenuated live vaccine strain by genotyping. J Clin Microbiol. (2004) 42:2121–6. doi: 10.1128/JCM.42.5.2121-2126.2004
13. Crespo R, Bland M, Opriessnig T. Use of commercial swine live attenuated vaccine to control an Erysipelothrix rhusiopathiae outbreak in commercial cage-free layer chickens. Avian Dis. (2019) 63:520–4. doi: 10.1637/12004-112118-Case.1
14. To H, Sato H, Tazumi A, Tsutsumi N, Nagai S, Iwata A, et al. Characterization of Erysipelothrix rhusiopathiae strains isolated from recent swine erysipelas outbreaks in Japan. J Vet Med Sci. (2012) 74:949–53. doi: 10.1292/jvms.11-0533
15. Coutinho TA, Imada Y, De Barcellos DESN, de Oliveira SJ, Moreno AM. Genotyping of Brazilian Erysipelothrix spp. strains by amplified fragment length polymorphism. J Microbiol Methods. (2011) 84:27–32. doi: 10.1016/j.mimet.2010.10.003
16. Silva AP, Cooper G, Blakey J, Jerry C, Shivaprasad HL, Stoute S. Retrospective summary of Erysipelothrix rhusiopathiae. diagnosed in avian species in California (2000-19). Avian Dis. (2020) 64:499–506. doi: 10.1637/aviandiseases-D20-00038
17. Zou Y, Zhu X, Muhammad HM, Jiang P, Li Y. Characterization of Erysipelothrix rhusiopathiae strains isolated from acute swine erysipelas outbreaks in Eastern China. J Vet Med Sci. (2015) 77:653–60. doi: 10.1292/jvms.14-0589
18. Wu C, Lv C, Zhao Y, Zhu W, Liu L, Wang T, et al. Characterization of Erysipelothrix rhusiopathiae isolates from diseased pigs in 15 Chinese provinces from 2012 to 2018. Microorganisms. (2021) 9:2615. doi: 10.3390/microorganisms9122615
19. Ceccolini ME, Wessels M, Macgregor SK, Deaville R, Perkins M, Jepson PD, et al. Systemic Erysipelothrix rhusiopathiae in seven free-ranging delphinids stranded in England and Wales. Dis Aquat Organ. (2021) 145:173–84. doi: 10.3354/dao03609
20. To H, Nagai S. Genetic and antigenic diversity of the surface protective antigen proteins of Erysipelothrix rhusiopathiae. Clin Vaccine Immunol. (2007) 14:813–20. doi: 10.1128/CVI.00099-07
21. Zhu W, Wu C, Kang C, Cai C, Wang Y, Li J, et al. Evaluation of the protective efficacy of four newly identified surface proteins of Erysipelothrix rhusiopathiae. Vaccine. (2018) 36:8079–83. doi: 10.1016/j.vaccine.2018.10.071
22. Makino SI, Yamamoto K, Murakami S, Shirahata T, Uemura K, Sawada T, et al. Properties of repeat domain found in a novel protective antigen, SpaB, of Erysipelothrix rhusiopathiae. Microb Pathog. (1998) 25:101–9. doi: 10.1006/mpat.1998.0216
23. Shimoji Y, Mori Y, Fischetti VA. Immunological characterization of a protective antigen of Erysipelothrix rhusiopathiae: Identification of the region responsible for protective immunity. Infect Immun. (1999) 67:1646–51. doi: 10.1128/IAI.67.4.1646-1651.1999
24. Cheun HI, Kawamoto K, Hiramatsu M, Tamaoki H, Shirahata T, Igimi S, et al. Protective immunity of SpaA-antigen producing Lactococcus lactis against Erysipelothrix rhusiopathiae infection. J Appl Microbiol. (2004) 96:1347–53. doi: 10.1111/j.1365-2672.2004.02283.x
25. Jin XH, Zheng LL, Song MR, Xu WS, Kou YN, Zhou Y, et al. A nano silicon adjuvant enhances inactivated transmissible gastroenteritis vaccine through activation the Toll-like receptors and promotes humoral and cellular immune responses. Nanomed Nanotechnol Biol Med. (2018) 14:1201–12. doi: 10.1016/j.nano.2018.02.010
26. Reed SG, Tomai M, Gale MJ. New horizons in adjuvants for vaccine development. Curr Opin Immunol. (2020) 30:23–32. doi: 10.1016/j.it.2008.09.006
27. Gu P, Wusiman A, Zhang Y, Cai G, Xu S, Zhu S, et al. Polyethylenimine-coated PLGA nanoparticles-encapsulated Angelica sinensis polysaccharide as an adjuvant for H9N2 vaccine to improve immune responses in chickens compared to Alum and oil-based adjuvants. Vet Microbiol. (2020) 251:108894. doi: 10.1016/j.vetmic.2020.108894
28. He P, Zou Y, Hu Z. Advances in aluminum hydroxide-based adjuvant research and its mechanism. Hum Vaccin Immunother. (2015) 11:477–88. doi: 10.1080/21645515.2014.1004026
29. SEPPIC. MontanideTM Adjuvant Ranges. (2022). Available online at: https://www.seppic.com/en/montanide-isa-w-o-w/ (Accessed June 5, 2022).
30. Begg DJ, Dhungyel O, Naddi A, Dhand NK, Plain KM, de Silva K, et al. The immunogenicity and tissue reactivity of Mycobacterium avium subsp paratuberculosis inactivated whole cell vaccine is dependent on the adjuvant used. Heliyon. (2019) 5:e01911. doi: 10.1016/j.heliyon.2019.e01911
31. Jang SI, Lillehoj HS, Lee SH, Lee KW, Park MS, Bauchan GR, et al. Immunoenhancing effects of MontanideTM ISA oil-based adjuvants on recombinant coccidia antigen vaccination against Eimeria acervulina infection. Vet Parasitol. (2010) 172:221–8. doi: 10.1016/j.vetpar.2010.04.042
32. SEPPIC. MontanideTM Adjuvant Ranges. (2022). Available online at: https://www.seppic.com/en/montanide-ims/. (Accessed June 5, 2022).
33. Xue Q, Zhao Z, Liu H, Chen K, Xue Y, Wang L. First comparison of adjuvant for trivalent inactivated Haemophilus parasuis serovars 4, 5 and 12 vaccines against Glässer's disease. Vet Immunol Immunopathol. (2015) 168:153–8. doi: 10.1016/j.vetimm.2015.11.001
34. Xu Y, Wang Q, Wei B, Huang X, Wen Y, Yan Q, et al. Enhanced immune responses against Japanese encephalitis virus infection using Japanese encephalitis live-attenuated virus adjuvanted with Montanide GEL 01 ST in mice. Vector-Borne Zoonotic Dis. (2019) 19:835–43. doi: 10.1089/vbz.2018.2419
35. Tabynov K, Sansyzbay A, Tulemissova Z, Tabynov K, Dhakal S, Samoltyrova A, et al. Inactivated porcine reproductive and respiratory syndrome virus vaccine adjuvanted with MontanideTM Gel 01 ST elicits virus-specific cross-protective inter-genotypic response in piglets. Vet Microbiol. (2016) 192:81–9. doi: 10.1016/j.vetmic.2016.06.014
36. SEPPIC. MontanideTM Adjuvant Ranges. (2022). Available online at: https://www.seppic.com/en/montanide-gel/. (Accessed June 5, 2022).
37. Reed LJ, Muench H. A simple method of estimating fifty percent endpoints. Am J Epidemiol. (1938) 27:493–7. doi: 10.1093/oxfordjournals.aje.a118408
38. Rosskopf-Streicher U, Johannes S, Wilhelm M, Cussler K. Quality control of inactivated erysipelas vaccines: results of an international collaborative study to establish a new regulatory test. Vaccine. (2001) 19:1477–83. doi: 10.1016/S0264-410X(00)00346-7
39. Guan L-J, Song J-J, Xue Y, Ai X, Liu Z-J, Si L-F, et al. Immune protective efficacy of China's traditional inactivated and attenuated vaccines against the prevalent strains of Pasteurella multocida in mice. Vaccines. (2021) 9:1155. doi: 10.3390/vaccines9101155
40. Stewart-Tull DE. The use of adjuvants in experimental vaccines : II. Water-in-Oil Emulsions: Freund's Complete and lncomplete Adjuvants. Methods Mol Med. (1996) 4:141–5. doi: 10.1385/0-89603-334-1:141
41. Dar P, Kalaivanan R, Sied N, Mamo B, Kishore S, Suryanarayana VVS, et al. Montanide TM ISA201 adjuvanted FMD vaccine induces improved immune responses and protection in cattle. Vaccine. (2013) 31:3327–32. doi: 10.1016/j.vaccine.2013.05.078
42. Ibrahim EES, Gamal WM, Hassan AI, Mahdy SED, Hegazy AZ, Abdel-Atty MM. Comparative study on the immunopotentiator effect of ISA 201, ISA 61, ISA 50, ISA 206 used in trivalent foot and mouth disease vaccine. Vet World. (2015) 8:1189–98. doi: 10.14202/vetworld.2015.1189-1198
43. Bouguyon E, Goncalves E, Shevtsov A, Maisonnasse P, Remyga S, Goryushev O, et al. A new adjuvant combined with inactivated influenza enhances specific CD8 T cell response in mice and decreases symptoms in swine upon challenge. Viral Immunol. (2015) 28:524–31. doi: 10.1089/vim.2014.0149
44. Arous JB, Bertrand F, Gaucheron J, Verkhovsky OA, Kotelnikov AP, Shemelkov EV, et al. Adjuvant formulations designed to improve swine vaccine stability: application to PCV2 vaccines. Procedia Vaccinol. (2013) 7:34–9. doi: 10.1016/j.provac.2013.06.007
45. Mathew MK, Virmani N, Bera BC, Anand T, Kumar R, Balena V, et al. Protective efficacy of inactivated reverse genetics based equine influenza vaccine candidate adjuvanted with montanideTM pet gel in murine model. J Vet Med Sci. (2019) 81:1753–62. doi: 10.1292/jvms.19-0399
46. Di Giacomo S, Quattrocchi V, Zamorano P. Use of adjuvants to enhance the immune response induced by a DNA vaccine against bovine herpesvirus-1. Viral Immunol. (2015) 28:343–6. doi: 10.1089/vim.2014.0113
47. Cauchard S, Bertrand F, Barrier-Battut I, Jacquet S, Laurentie M, Barbey C, et al. Assessment of the safety and immunogenicity of Rhodococcus equi-secreted proteins combined with either a liquid nanoparticle (IMS 3012) or a polymeric (PET GEL A) water-based adjuvant in adult horses and foals–identification of promising new candidate an. Vet Immunol Immunopathol. (2014) 157:164–74. doi: 10.1016/j.vetimm.2013.12.003
48. Petermann J, Bonnefond R, Mermoud I, Rantoen D, Meynard L, Munro C, et al. Evaluation of three adjuvants with respect to both adverse effects and the efficacy of antibody production to the Bm86 protein. Exp Appl Acarol. (2017) 72:303–15. doi: 10.1007/s10493-017-0156-4
49. Zhao Z, Liu H, Zhang K, Xue Q, Chen K, Xue Y. Minimum dose, antigen content, and immunization duration of a trivalent vaccine of inactivated Haemophilus parasuis serovars 4, 5, and 12 against Glässer's disease in pigs. Can J Vet Res. (2016) 80:287–93.
50. Zhao Z, Liu H, Xue Y, Chen K, Liu Z, Xue Q, et al. Analysis of efficacy obtained with a trivalent inactivated Haemophilus parasuis serovars 4, 5, and 12 vaccine and commercial vaccines against Glässer's disease in piglets. Can J Vet Res. (2017) 81:22–7.
51. Ma X, Guo Z, Shen Z, Wang J, Hu Y, Wang D. The immune enhancement of propolis adjuvant on inactivated porcine parvovirus vaccine in guinea pig. Cell Immunol. (2011) 270:13–8. doi: 10.1016/j.cellimm.2011.03.020
Keywords: Erysipelothrix rhusiopathiae, swine, vaccine, adjuvant, protective efficacy
Citation: Guan L-J, Pei S-X, Song J-J, Zhan P-F, Han Y-N, Xue Y, Ding K and Zhao Z-Q (2022) Screening immune adjuvants for an inactivated vaccine against Erysipelothrix rhusiopathiae. Front. Vet. Sci. 9:922867. doi: 10.3389/fvets.2022.922867
Received: 27 April 2022; Accepted: 05 July 2022;
Published: 26 July 2022.
Edited by:
Massimo Amadori, Italian Network of Veterinary Immunology, ItalyReviewed by:
Weifeng Zhu, Hebei Agricultural University, ChinaCopyright © 2022 Guan, Pei, Song, Zhan, Han, Xue, Ding and Zhao. This is an open-access article distributed under the terms of the Creative Commons Attribution License (CC BY). The use, distribution or reproduction in other forums is permitted, provided the original author(s) and the copyright owner(s) are credited and that the original publication in this journal is cited, in accordance with accepted academic practice. No use, distribution or reproduction is permitted which does not comply with these terms.
*Correspondence: Zhan-Qin Zhao, emhhb3poYW5xaW5AMTI2LmNvbQ==
Disclaimer: All claims expressed in this article are solely those of the authors and do not necessarily represent those of their affiliated organizations, or those of the publisher, the editors and the reviewers. Any product that may be evaluated in this article or claim that may be made by its manufacturer is not guaranteed or endorsed by the publisher.
Research integrity at Frontiers
Learn more about the work of our research integrity team to safeguard the quality of each article we publish.