- 1Key Laboratory of Livestock and Poultry Resources (Sheep & Goat) Evaluation and Utilization, Ministry of Agriculture and Rural Affairs, College of Animal Science and Technology, Jilin Agricultural University, Changchun, China
- 2Heilongjiang Academy of Agricultural Sciences, Animal Husbandry Research Institute, Harbin, China
Understanding whether and how melatonin (MT) may impact sheep oocyte development competence is central to our ability to predict how sheep oocytes will respond to artificially regulated estrus. Implanting MT can make sheep enter estrus during the non-breeding season. One study found that the blastocyst rate increased under MT treatment, while another found that the blastocyst rate decreased. Therefore, we conducted a meta-analysis of MT directly and indirectly influencing sheep oocytes. A total of 433 articles were collected from which 20 articles and 34 treatments were finally selected. A method for estimating the default value was established for the litter size analysis. We found that exogenous MT add into in vitro maturation medium was positively related to the blastocyst rate in the lab. However, subcutaneous implanting MT did not affect the in vivo ovulation rate, fertilization rate, blastocyst rate, or pregnancy rate at farm. MT did not affect the in vitro cleavage rate. However, MT improved the in vivo cleavage rate. We hypothesized that implanted MT could increase the concentration of MT in oviduct fluid in vivo, and also that in vitro MT could increase the early cleavage rate of sheep zygotes without affecting the total cleavage rate. In the analysis of oocyte apoptosis caused by injury, the results suggested that pyroptosis would be more suitable for further research. MT produces responses in all body organs, and thus implanting of MT during non-breeding seasons should consider the effect on animal welfare.
Introduction
Sheep oocyte development competence can be directly affected by adding exogenous melatonin (MT) during in vivo culture as well as indirectly by implanting MT in ewes. The concentration of MT in various tissues differs widely. The concentration of MT in the gastrointestinal tract is 10–100 times higher than that in blood (1). The concentration of MT is different in different size sheep follicles (2). This indicates that MT is positively correlated with the developmental ability of sheep oocytes. MT can maintain the reproductive process (3). MT has anti-inflammatory (4) and wound healing functions (5) and is associated with many signaling pathways (6). This article focuses on the impact of MT on sheep oocytes. Different drug delivery routes can have different effects; for example, magnesium sulfate is taken orally for catharsis (7), and intramuscular or intravenous injection can relieve muscle spasms (8). Therefore, we were curious to know how MT directly and indirectly affects sheep oocytes.
Sheep are seasonal estrous animals. The estrous period and intensity are significantly different depending on the region, breed, and other factors (9). Implanting MT can make sheep enter estrus in non-breeding seasons. However, this phenomenon is not uniform in all aspects. One study found that blastocyst cell number decreased (10), whereas another study found that blastocyst cell number increased (11). Some studies have suggested that the blastocyst rate is decreased (12), whereas others have found that the blastocyst rate is increased (13). In particular, litter size changes with the season (9, 14). According in vitro study, the blastocyst rate of cultured sheep oocytes can be improved by adding MT to in vitro maturation (IVM) (10, 11, 15). However, there is no report on the simultaneous control study of in vivo and in vitro studies. Therefore, we performed a meta-analysis and reviewed the results concerning the direct and indirect effects of MT on sheep oocytes.
Methods
Database Search Strategy
We collected articles concerning the effects of MT on sheep oocytes both in vitro and in vivo. A search of PubMed, Ovid, Science Direct, and ProQuest from 2006.01.01 to 2021.09.01 used the key search terms melatonin AND (Ovine OR sheep OR ewe) AND (oocyte OR follicle), producing a total of 238 articles. The effects of melatonin on litter size used the key search terms melatonin AND (ram OR ewe) AND implant, and 195 articles were found.
Data Extraction
We selected the relevant articles according to the criteria listed in Table 1. Specifically, oocytes cultured in vitro should have follicle growth data or embryonic development data. In the experiment of subcutaneous implantation in ewes, ovulation data or oocyte development data should be available. The data of litter size should be available in the experiment of ram subcutaneous implantation. The studies of melatonin's effect on sheep oocyte development tended to explore the mechanism, and many experimental groups had observed positive results. When extracting the data, only the groups with the largest positive value were retained. Each experimental group was studied in different seasons or in different sheep varieties about litter size study. Therefore, when extracting data, each experimental group was used as a treatment. When the experimental data were incomplete, we contacted the authors to obtain the data. We were unable to contact all the authors to obtain the raw data. When analyzing the effect on litter size, we supplemented the missing data using the default value; refer Supplementary Data 1 for the method.
Data Analysis
Review Manager (Copenhagen: Nordic Cochrane Center, Cochrane Collaboration, Version 5.4) was used for the meta-analysis. A standard mean difference (SMD) meta-analysis using a continuous model was used to calculate in vitro culture follicle diameter and blastocyst cell number. The effects of implanting MT on ovulation rate, fertilization rate, and litter size were also analyzed with a continuous model. Relative risk (RR) was analyzed with a dichotomous model. This included in vitro culture follicle normal rate, IVM rate, cleavage rate, and blastocyst rate. The effects of implanting MT on cleavage rate, blastocyst rate, and pregnancy rate were also analyzed with a dichotomous model.
Heterogeneity was analyzed by the Higgins I2 statistic (I2 >50% means a high level of heterogeneity) (16). When heterogeneity was found in the analysis, the source of the heterogeneity was identified according to the differences in follicle diameter and culture methods as well as the experimental details such as different research seasons (Table 2). The heterogeneous groups were analyzed by a random effects model. The groups without heterogeneity were analyzed by a fixed effects model. Litter size was analyzed without considering subgroups, as the location in the northern and southern hemispheres and different time periods of the studies were the sources of heterogeneity. Potential bias was identified using Begg's funnel plots and rechecked by Egger's linear regression (28) and Begg's rank correlation tests. Statistical analyses were performed using Stata 12.0 (Stata Corp, College Station, TX, USA). Furthermore, trial sequential analysis (TSA) was used to evaluate the reliability of our results. For the TSA we set the IVM rate control to 70% according to experimental experience.
Results
The process and results of screening 433 articles are shown in Figure 1. A final group of 20 studies is shown in Table 2 (9–15, 17–27, 29, 30). The studies comprised 34 treatments.
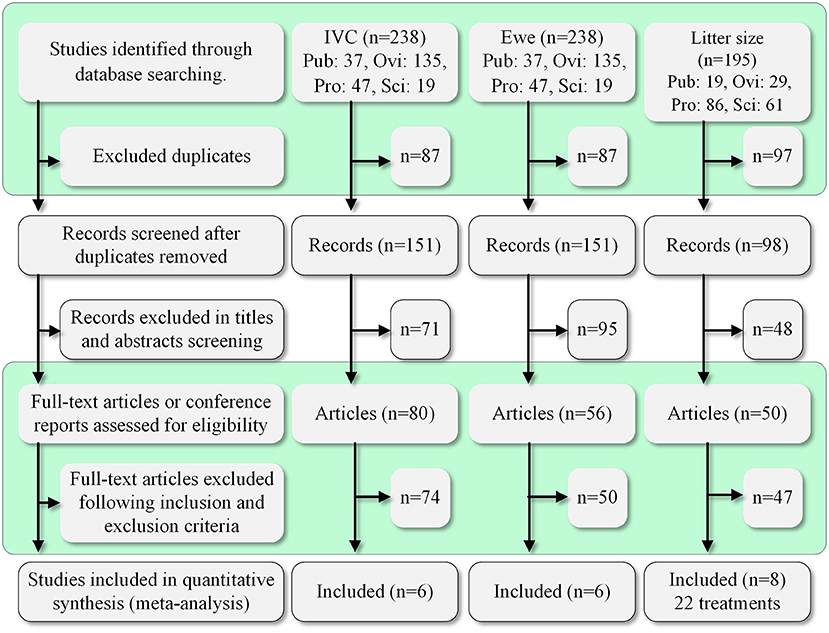
Figure 1. Summary of the study selection process. Pub, PubMed; Ovi, Ovid; Pro, ProQuest; Sci, ScienceDirect.
Under in vitro conditions, exogenous MT was positively related to follicle diameter (SMD = 4.03, 95% CI = 0.42–7.63; p = 0.03; Figure 2A), in vitro maturation (IVM), and blastocyst rate (Figure 2B). MT had no effect on follicle normal rate (RR = 1.76, 95% CI = 0.89–3.47; p = 0.11; Figure 2B), cleavage rate (RR = 1.22, 95% CI = 1.01–1.47; p = 0.04; Figure 2B), or blastocyst cell number (SMD = 4.07, 95% CI = −2.08 to 10.22; p = 0.19; Figure 2A). There was significant heterogeneity in follicle diameter and follicle normal rate. Subgroup analysis according to follicle diameter was performed using a random model. The heterogeneity was produced by different follicle diameters. Cleavage rate and blastocyst cell number were heterogeneous owing to different culture methods according to the subgroup analysis using a random model. The sources of heterogeneity were culture wells and culture droplet size.
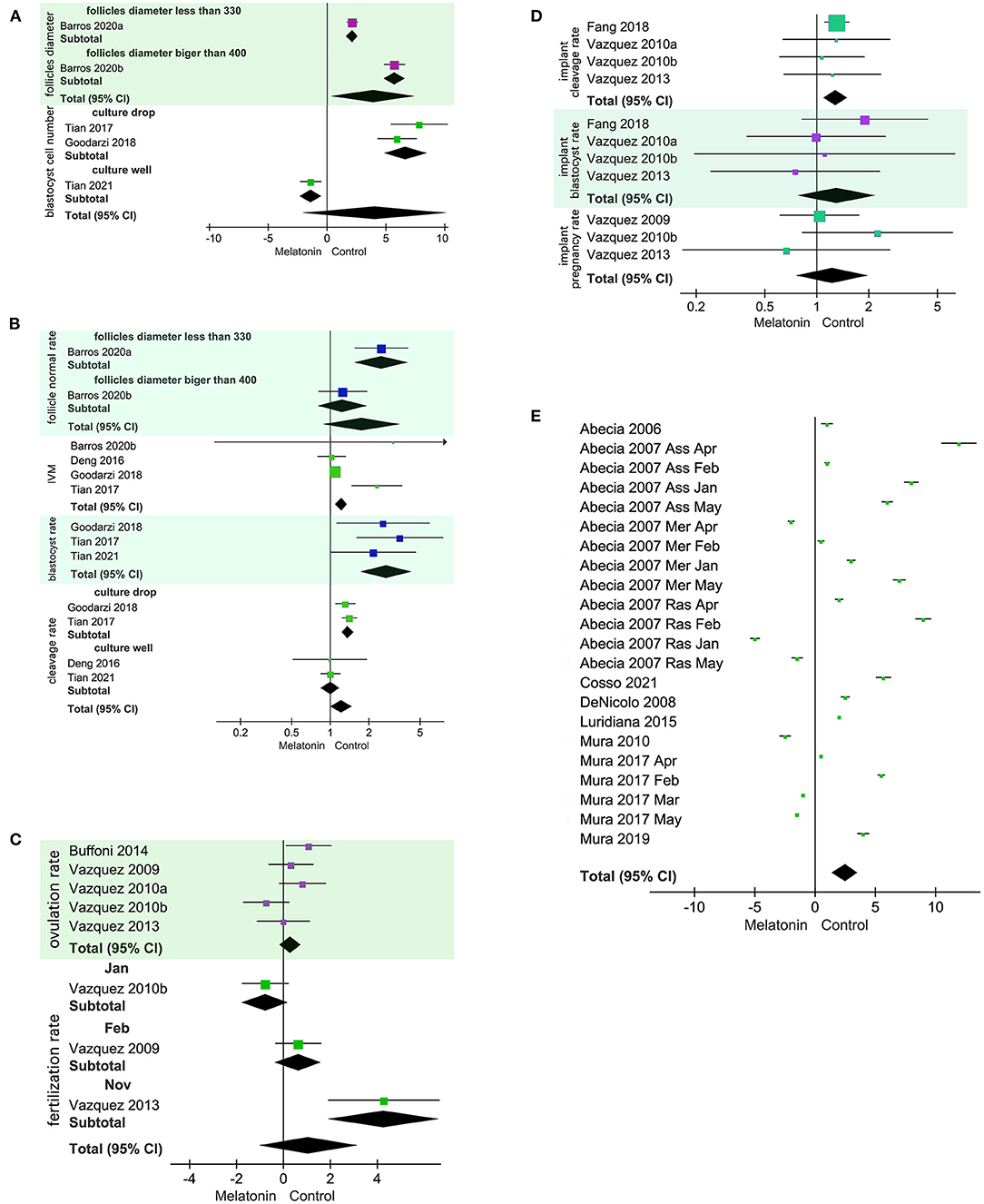
Figure 2. Forest plot of melatonin (MT) treatment effects on sheep oocytes. The subgroup analysis was based on different follicle diameters, different culture treatments, and different months. (A) SMD for the effects of in vitro culture of exogenous MT on follicle diameter and blastocyst cell number. Blastocyst cell number analysis culture droplet subgroup I2 = 36%. (B) RR for the effects of in vitro culture exogenous MT on follicle normal rate, IVM rate, cleavage rate, and blastocyst rate. Cleavage rate analysis of the culture droplet subgroup and the culture well subgroup yielded I2 = 0%. (C) SMD for implanted exogenous MT effects on ovulation rate and fertilization rate. (D) RR implanted exogenous MT effects on cleavage rate, blastocyst rate, and pregnancy rate. (E) Implanted exogenous MT effects on litter size.
For in vivo studies using treated ewes, implanting MT had no effect on ovulation rate, fertilization rate (SMD = 1.08, 95% CI = −1.00 to 3.16; p = 0.31; Figure 2C), blastocyst rate, or pregnancy rate (RR = 1.29, 95% CI = 1.1–1.5; p = 0.56; Figure 2D). Implanting MT was positively related to cleavage rate (Figure 2D). The results for fertilization rate were heterogeneous due to differences in experimental starting month as decided by a subgroup analysis using a random model. When implanted MT treatment was used for rams or ewes, implanting MT was positively related to litter size (Figure 2E). The implanted MT litter size was 1.3395, and the control litter size was 1.2795.
The funnel plot (Figures 3A,B) shows that there was no potential bias, and this result was corroborated by Egger's test (IVM rate, p = 0.972; implant ovulation rate p = 0.529) and Begg's test (IVM rate Pr > |z| = 1; implant ovulation rate, Pr > |z| = 0.602). The TSA results showed that the in vitro exogenous MT effect on sheep oocyte IVM was real (Figure 3C). It should be noted that the actual IVM rate was 80%−90%. We set the IVM rate control to 70%. Setting the control rate to 50% according to the statistical method would not allow the significance threshold to be reached. The influence of implanted MT on ewe oocytes needs further research (Figure 3D). Although the number of studies is large, the actual number of samples is small.
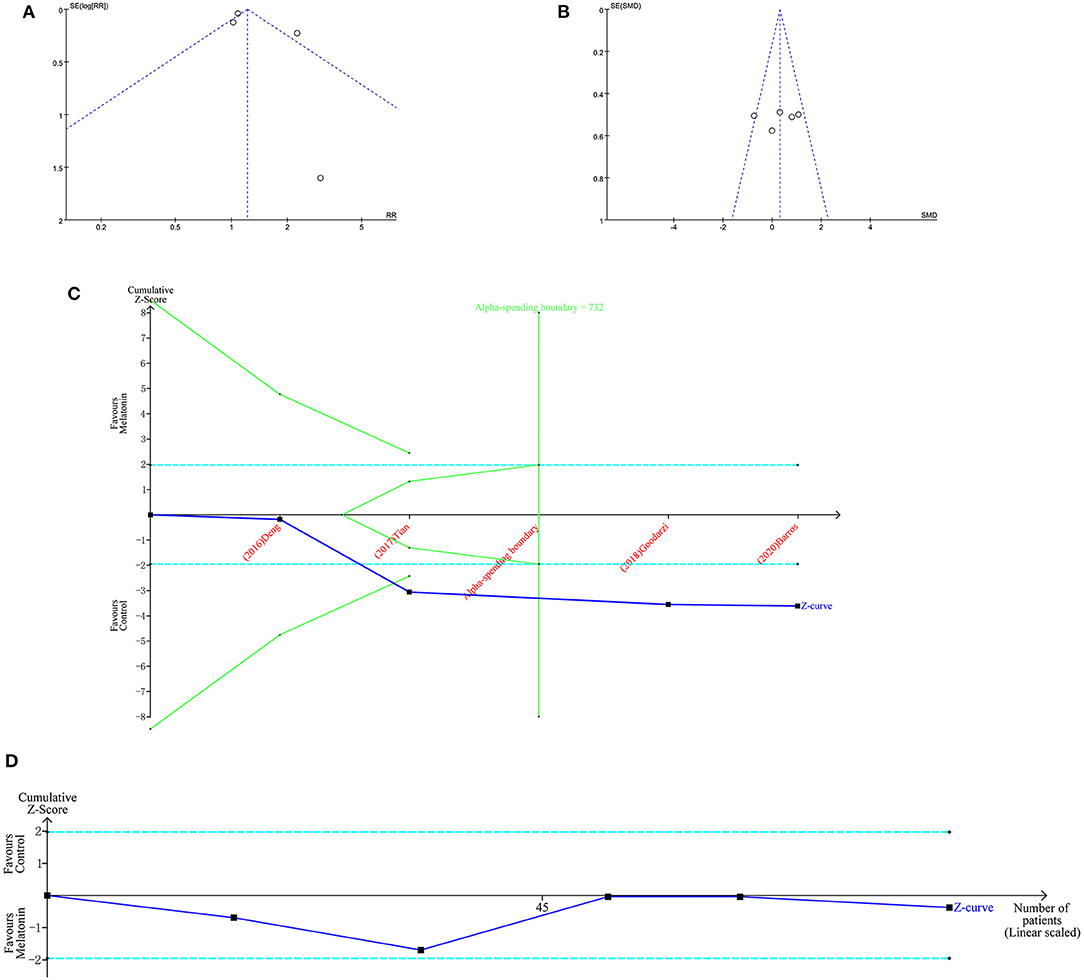
Figure 3. Bias analysis and TSA of MT of sheep oocytes. Funnel plots of IVM rate (A) and implant ovulation rate (B). TSA of IVM rate (C) and implant ovulation rate (D).
Discussion
Understanding how MT directly and indirectly influences sheep oocyte development competence is an important goal. MT also significantly promoted the development of embryos in vitro, for example, in mice (31), bovines (32), swine (33), and goats (34).
Direct Exogenous MT Employed in in vitro Culture
The beneficial effects of MT on early embryos depend on two key factors. An important function of it used in vitro is antioxidation. As an effective antioxidant, MT can significantly reduce the level of intracellular ROS and improve the in vitro maturation (IVM) of oocytes and the in vitro developmental potential of embryos (32). MT can significantly reduce the apoptosis of cultured cells and improve the quality of embryos. Previous studies have confirmed that MT can inhibit apoptosis and decreases in cell proliferation of sheep granulosa cells under heat stress (35). MT can inhibit the expression of the apoptosis genes p53 and Bax and promote the expression of antioxidant genes SOD1 and GPX4 in embryos (36). In addition to its antioxidant properties, MT activates the SIRT1/PGC-1α pathway to compensate for mitochondrial depletion and energy deficiency caused by environmental toxin exposure and thereby promotes mitochondrial biosynthesis (33). MT can activate two G protein coupled receptors, MT1 and MT2, which regulate the growth of oocytes (37, 38). In addition, the effect of MT on oocytes may also be related to apoptosis, mitochondrial function (39), antioxidant enzymes (40), DNA methylation (41), cumulus cell expansion (42), and histone acetylation (43).
Our results show that MT is positively related to follicle growth in vitro. When cumulus oocyte complexes (COCs) were cultured alone, MT was positively related to IVM and blastocyst rate. That does not affect the quality of blastocysts, because blastocyst cell number is generally used as the standard to measure the quality of zygotes.
Implanting MT in Ewes/Rams: Indirect Effects
Sheep are seasonal estrous animals, and the Assaf sheep are more seasonal than Merino sheep (9). MT is the key endogenic hormone regulating the estrus of sheep and that secretion is affected by light and by the season (44, 45). It can be secreted by cells of all tissues (1). MT is lipophilic and can penetrate all cell membranes and enter all tissues (38) and is particularly concentrated in follicles (46). The concentration of MT in follicles varies according to follicle size (2). The accumulation of ROS in the ovary will reduce the quality of oocytes and lead to the apoptosis of granulosa cells (47). MT can significantly reduce the level of intracellular ROS (32). It can penetrate the blood-testis barrier (48). When it was implanted in rams, the concentration of MT in seminal vesicles increased (49), and implanted MT affected semen quality. Oral administration of MT can also improve the human fertilization rate (50) and increase the number of high-quality embryos (51). Differences of implanted MT can cause differences in the release rate. One study found that the concentration of MT in seminal plasma peaked after 7 days and then decreased (52). Another study showed that the concentration of MT in seminal plasma gradually increased, reached the highest level after 90 days, and then decreased (53). In addition, the peak time of MT concentration differed according to different implant seasons (54). About 90% of MT is degraded through the liver (55).
Our results show that sheep from different regions and varieties can be made to enter estrus by implanting MT. It is positively related to litter size. Interestingly, MT has clear effects in vitro culture sheep oocytes and improves the blastocyst rate, although the embryo quality is unaffected in lab experimentation. Embryo quality is determined by the number of blastocyst cells number. MT has no effect on the cleavage rate in vivo but improves the rate in vitro. That declare the direct and indirect effects of MT on sheep oocytes are completely different.
Other studies have shown that there are MT receptors MT1 and MT2 in the sheep oviduct, and MT1 and MT2 are regulated by E2 (56). Cleavage occurs in the oviduct, and thus, the hypothesis is that implanted MT could increase the concentration of MT in oviduct fluid. During the present study, we noted that in vitro studies observed that the effect on the cleavage rate occurred 48 h after fertilization. The in vivo studies observed that most of the cleavage rate effect occurred 24–36 h after fertilization. In this way, different key time points can distinguish early cleavage rate and total cleavage rate (57). Hence the hypothesis that MT can improve the early cleavage rate, while the total cleavage rate will not be affected.
Proposed Relation of MT to Sheep Oocyte Developmental Competence
MT is produced by the pineal gland. The normal secretion is a necessary condition for regulating physiological functions. However, excessive MT carries potential risks (58, 59). MT inhibits rat testosterone secretion by downregulating the hypothalamus-pituitary-gonadal axis (60). No studies have determined whether exogenic MT inhibits normal pituitary function (Figure 4A). MT is known to be involved in many oocyte-related signaling pathways. It is involved in the Hedgehog signaling pathway to promote swing oocyte IVM (61). MT reduces glyphosate herbicide toxicity during mouse oocyte maturation by regulating the GPER signaling pathway (62). MT-adjusted Nrf2 signaling decreased ROS in COCs and promoted swine (63) and mouse (64) oocyte IVM (Figure 4B). MT inhibited nicotinamide methylation signaling, promoting bovine oocyte IVM (65). In addition, MT is also involved in the Notch signaling pathway (66), the H4K16 deacetylation pathway (43), and the mTOR pathway (67).
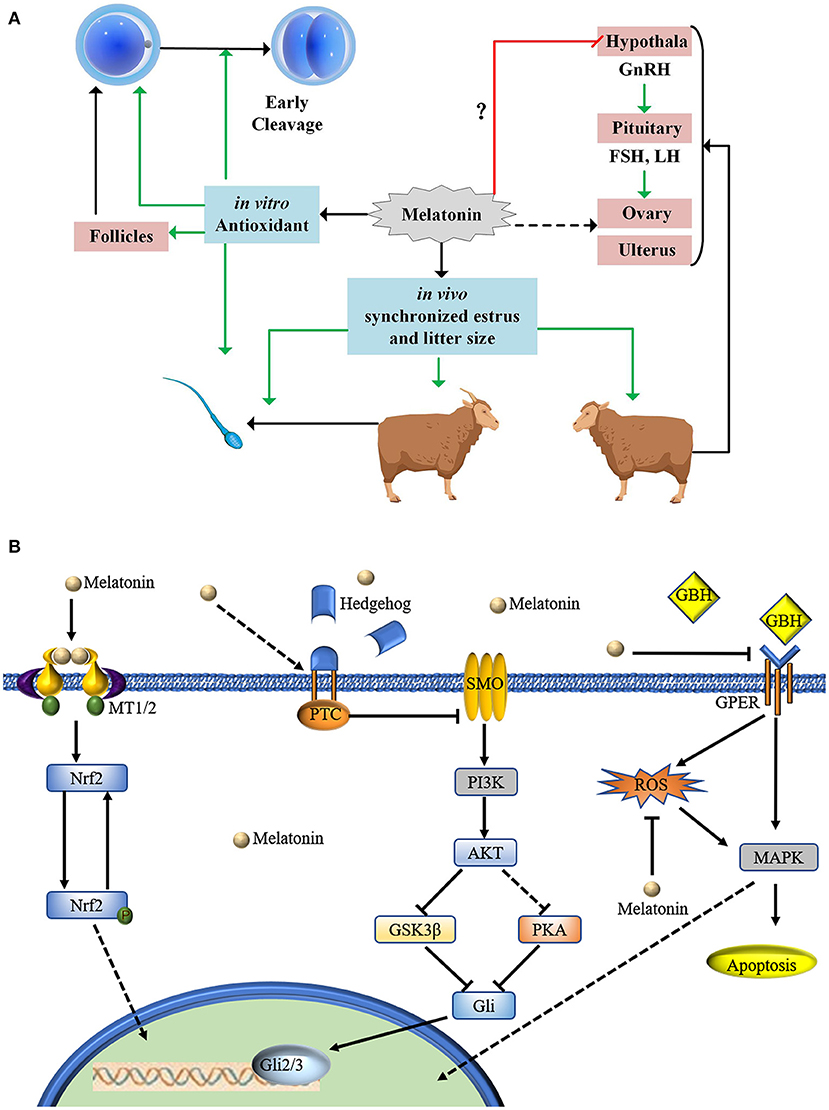
Figure 4. Proposed mode of action of MT in relation to sheep oocyte developmental competence. (A) MT treatment of sheep oocytes cultured in vitro has antioxidant effects and can increase oocyte development potential. MT can improve early cleavage. Implantation of MT in ewes and rams can promote estrus. There is no evidence to indicate whether implanting MT in ewes inhibits the hypothalamus-gonadal axis. Implanted MT can be enriched in follicles through the blood ovum barrier. Our statistical results show that implanting MT can increase the number of fetuses. (B) MT is involved in many oocyte-related signaling pathways.
Melatonin promotes the excretion of exosomes (68), and it promotes exosome secretion from mesenchymal stem cells also (69). Early embryonic blastomeres are totipotent stem cells (70). The concentration of MT differs in follicles according to follicle diameter (2). One aspect that needs to be considered in this area is how MT is enriched from the blood and how it can pass through the blood ovum barrier. It is involved in the cell crosstalk of oocytes and cumulus cells (71). If MT is secreted by granulosa cells or cumulus cells, this suggests that we should study the effect of it on oocytes from the aspects of endogenous MT autocrine and paracrine functions. MT can inhibit oocyte apoptosis (71). Regarding studies on oocyte apoptosis caused by injury (72, 73), we suggest that this should be confirmed by pyroptosis.
Implication of This Research
Melatonin's effect on sheep oocyte development in vitro is conducive to the study of the underlying molecular mechanism, because itself is a hormone secreted by the body. The MT extracted from sheep is best used in the research process. Future research will focus on the mechanism of the interaction between MT and other hormones, the signaling pathways in which MT participates, and how it is transported across membranes against a concentration gradient.
Implanting MT to promote estrus is no longer the most suitable method of estrus regulation. The sheep and goats of our farms all use the method of vaginal sponges impregnated with progesterone for estrus synchronization, and then use laparoscope minimally invasive surgery to inject semen into the oviduct; this method has achieved good results.
Future studies need to determine whether MT can improve the early cleavage rate of sheep embryos without affecting the total cleavage rate. It is also necessary to determine whether implanting MT can increase the MT level in sheep oviduct fluid. Implanting MT affects all body organs and produces side effects such as promoting the growth of goat hair (74). It also affects the cardiovascular system (75), the neurological system (76), the endocrine system (77), and metabolism (78). Thus, implanting MT in non-breeding seasons should consider the effects on animal welfare.
Data Availability Statement
The original contributions presented in the study are included in the article/Supplementary Material, further inquiries can be directed to the corresponding author/s.
Author Contributions
YC and ZG collected the data and conducted the analysis. YC, XS, and ZG checked the data. HJ and ZG designed the experiment. All authors contributed to the article and approved the submitted version.
Funding
This work was supported by the Jilin Scientific and Technological Developing Program (20200402039NC). National Key Research and Development Project of China (2021YFF1000702). The funders had no role in study design or preparation of the manuscript.
Conflict of Interest
The authors declare that the research was conducted in the absence of any commercial or financial relationships that could be construed as a potential conflict of interest.
Publisher's Note
All claims expressed in this article are solely those of the authors and do not necessarily represent those of their affiliated organizations, or those of the publisher, the editors and the reviewers. Any product that may be evaluated in this article, or claim that may be made by its manufacturer, is not guaranteed or endorsed by the publisher.
Acknowledgments
We would like to thank LetPub (www.letpub.com) for its linguistic assistance during the preparation of this manuscript.
Supplementary Material
The Supplementary Material for this article can be found online at: https://www.frontiersin.org/articles/10.3389/fvets.2022.903195/full#supplementary-material
References
1. Tordjman S, Chokron S, Delorme R, Charrier A, Bellissant E, Jaafari N, et al. Melatonin: pharmacology, functions and therapeutic benefits. Curr Neuropharmacol. (2017) 15:434–43. doi: 10.2174/1570159X14666161228122115
2. Xiao L, Hu J, Song L, Zhang Y, Dong W, Jiang Y, et al. Profile of melatonin and its receptors and synthesizing enzymes in cumulus-oocyte complexes of the developing sheep antral follicle-a potential estradiol-mediated mechanism. Reprod Biol Endocrinol. (2019) 17:1. doi: 10.1186/s12958-018-0446-7
3. Russo M, Forte G, Montanino Oliva M, Lagana AS, Unfer V. Melatonin and Myo-inositol: supporting reproduction from the oocyte to birth. Int J Mol Sci. (2021) 22:8433. doi: 10.3390/ijms22168433
4. Martin Gimenez VM, Chuffa LGA, Simao VA, Reiter RJ, Manucha W. Protective actions of vitamin D, anandamide and melatonin during vascular inflammation: epigenetic mechanisms involved. Life Sci. (2022) 288:120191. doi: 10.1016/j.lfs.2021.120191
5. Al-Otaibi AM, Al-Gebaly AS, Almeer R, Albasher G, Al-Qahtani WS, Abdel Moneim AE. Melatonin pre-treated bone marrow derived-mesenchymal stem cells prompt wound healing in rat models. Biomed Pharmacother. (2022) 145:112473. doi: 10.1016/j.biopha.2021.112473
6. Yu LM, Dong X, Zhao JK, Xu YL, Xu DY, Xue XD, et al. Activation of PKG-CREB-KLF15 by melatonin attenuates angiotensin II-induced vulnerability to atrial fibrillation via enhancing branched-chain amino acids catabolism. Free Radic Biol Med. (2022) 178:202–14. doi: 10.1016/j.freeradbiomed.2021.11.043
7. Wang W, Li J, Ma G, Li N, Wang P, Xiao Q, et al. [Effect of rhubarb as the main composition of sequential treatment in patients with acute paraquat poisoning: a prospective clinical research]. Zhonghua Wei Zhong Bing Ji Jiu Yi Xue. (2015) 27:254–8. doi: 10.3760/cma.j.issn.2095-4352.2015.04.006
8. Lu JF, Nightingale CH. Magnesium sulfate in eclampsia and pre-eclampsia: pharmacokinetic principles. Clin Pharmacokinet. (2000) 38:305–14. doi: 10.2165/00003088-200038040-00002
9. Abecia JA, Valares JA, Forcada F, Palacín I, Martín S, Martino A. The effect of melatonin on the reproductive performance of three sheep breeds in Spain. Small Rumin Res. (2007) 69:10–16. doi: 10.1016/j.smallrumres.2005.12.018
10. Tian H, Qi Q, Yan F, Wang C, Hou F, Ren W, et al. Enhancing the developmental competence of prepubertal lamb oocytes by supplementing the in vitro maturation medium with sericin and the fibroblast growth factor 2 - leukemia inhibitory factor - insulin-like growth factor 1 combination. Theriogenology. (2021) 159:13–19. doi: 10.1016/j.theriogenology.2020.10.019
11. Goodarzi A, Zare Shahneh A, Kohram H, Sadeghi M, Moazenizadeh MH, Fouladi-Nashta A, et al. Effect of melatonin supplementation in the long-term preservation of the sheep ovaries at different temperatures and subsequent in vitro embryo production. Theriogenology. (2018) 106:265–70. doi: 10.1016/j.theriogenology.2017.10.009
12. Vazquez MI, Forcada F, Sosa C, Casao A, Sartore I, Fernandez-Foren A, et al. Effect of exogenous melatonin on embryo viability and uterine environment in undernourished ewes. Anim Reprod Sci. (2013) 141:52–61. doi: 10.1016/j.anireprosci.2013.07.007
13. Fang Y, Deng S, Zhang J, Liu H, Li Y, Zhang X, et al. Melatonin-mediated development of ovine cumulus cells, perhaps by regulation of DNA methylation. Molecules. (2018) 23:494. doi: 10.3390/molecules23020494
14. Mura MC, Luridiana S, Farci F, Di Stefano MV, Daga C, Pulinas L, et al. Melatonin treatment in winter and spring and reproductive recovery in Sarda breed sheep. Anim Reprod Sci. (2017) 185:104–8. doi: 10.1016/j.anireprosci.2017.08.009
15. Tian X, Wang F, Zhang L, He C, Ji P, Wang J, et al. Beneficial effects of melatonin on the in vitro maturation of sheep oocytes and its relation to melatonin receptors. Int J Mol Sci. (2017) 18:834. doi: 10.3390/ijms18040834
16. de la Cruz ML, Conrado I, Nault A, Perez A, Dominguez L, Alvarez J. Vaccination as a control strategy against Salmonella infection in pigs: a systematic review and meta-analysis of the literature. Res Vet Sci. (2017) 114:86–94. doi: 10.1016/j.rvsc.2017.03.005
17. Barros VRP, Monte APO, Santos JMS, Lins T, Cavalcante AYP, Gouveia BB, et al. Melatonin improves development, mitochondrial function and promotes the meiotic resumption of sheep oocytes from in vitro grown secondary follicles. Theriogenology. (2020) 144:67–73. doi: 10.1016/j.theriogenology.2019.12.006
18. Barros VRP, Monte APO, Santos JMS, Lins T, Cavalcante AYP, Gouveia BB, et al. Effects of melatonin on the in vitro growth of early antral follicles and maturation of ovine oocytes. Domest Anim Endocrinol. (2020) 71:106386. doi: 10.1016/j.domaniend.2019.106386
19. Deng SL, Chen SR, Wang ZP, Zhang Y, Tang JX, Li J, et al. Melatonin promotes development of haploid germ cells from early developing spermatogenic cells of Suffolk sheep under in vitro condition. J Pineal Res. (2016) 60:435–47. doi: 10.1111/jpi.12327
20. Buffoni A, Vozzi PA, Gonzalez DM, Rios G, Viegas-Bordeira H, Abecia JA. The effect of melatonin and season on in vivo embryo production of Dohne Merino ewes. Small Rumin Res. (2014) 120:121–4. doi: 10.1016/j.smallrumres.2014.05.003
21. Vazquez MI, Forcada F, Casao A, Abecia JA, Sosa C, Palacin I. Undernutrition and exogenous melatonin can affect the in vitro developmental competence of ovine oocytes on a seasonal basis. Reprod Domest Anim. (2010) 45:677–84. doi: 10.1111/j.1439-0531.2008.01329.x
22. Vazquez MI, Abecia JA, Forcada F, Casao A. Effects of exogenous melatonin on in vivo embryo viability and oocyte competence of undernourished ewes after weaning during the seasonal anestrus. Theriogenology. (2010) 74:618–26. doi: 10.1016/j.theriogenology.2010.03.007
23. Abecia JA, Palacin I, Forcada F, Valares JA. The effect of melatonin treatment on the ovarian response of ewes to the ram effect. Domest Anim Endocrinol. (2006) 31:52–62. doi: 10.1016/j.domaniend.2005.09.003
24. Cosso G, Luridiana S, Pulinas L, Curone G, Pich G, Carcangiu V, et al. Melatonin treatment in rams and their replacement with novel treated rams advance first lambing and increase fertility in Sarda ewe lambs. Animals. (2021) 11:1227. doi: 10.3390/ani11051227
25. Luridiana S, Mura MC, Daga C, Farci F, Di Stefano MV, Zidda F, et al. Melatonin treatment in spring and reproductive recovery in sheep with different body condition score and age. Anim Reprod Sci. (2015) 160:68–73. doi: 10.1016/j.anireprosci.2015.07.004
26. Mura MC, Luridiana S, Vacca GM, Bini PP, Carcangiu V. Effect of genotype at the MTNR1A locus and melatonin treatment on first conception in Sarda ewe lambs. Theriogenology. (2010) 74:1579–86. doi: 10.1016/j.theriogenology.2010.06.028
27. Mura MC, Luridiana S, Pulinas L, Bizzarri D, Cosso G, Carcangiu V. Melatonin treatment and male replacement every week on the reproductive performance in Sarda sheep breed. Theriogenology. (2019) 135:80–4. doi: 10.1016/j.theriogenology.2019.06.008
28. Egger M, Davey Smith G, Schneider M, Minder C. Bias in meta-analysis detected by a simple, graphical test. BMJ. (1997) 315:629–34. doi: 10.1136/bmj.315.7109.629
29. Vázquez MI, Forcada F, Casao A, Sosa C, Palacín I, Abecia JA. Effects of melatonin and undernutrition on the viability of ovine embryos during anestrus and the breeding season. Anim Reprod Sci. (2009) 112:83–94. doi: 10.1016/j.anireprosci.2008.04.004
30. DeNicolo G, Morris ST, Kenyon PR, Morel PC, Parkinson TJ. Melatonin-improved reproductive performance in sheep bred out of season. Anim Reprod Sci. (2008) 109:124–33. doi: 10.1016/j.anireprosci.2007.10.012
31. Caglar K, Kocabay A, Taskin AC, Arat S. Treatment with melatonin enhances the embryo quality and the development of vitrified/warmed eight cell mouse embryos by solid surface vitrification (SSV). Cryo Lett. (2020) 41:62–7.
32. Hao T, Xu X, Hao H, Du W, Pang Y, Zhao S, et al. Melatonin improves the maturation and developmental ability of bovine oocytes by up-regulating GJA4 to enhance gap junction intercellular communication. Reprod Fertil Dev. (2021) 33:760–71. doi: 10.1071/RD21145
33. Niu YJ, Zhou W, Nie ZW, Shin KT, Cui XS. Melatonin enhances mitochondrial biogenesis and protects against rotenone-induced mitochondrial deficiency in early porcine embryos. J Pineal Res. (2020) 68:e12627. doi: 10.1111/jpi.12627
34. Soto-Heras S, Catala MG, Roura M, Menendez-Blanco I, Piras AR, Izquierdo D, et al. Effects of melatonin on oocyte developmental competence and the role of melatonin receptor 1 in juvenile goats. Reprod Domest Anim. (2019) 54:381–90. doi: 10.1111/rda.13378
35. Fu Y, He CJ, Ji PY, Zhuo ZY, Tian XZ, Wang F, et al. Effects of melatonin on the proliferation and apoptosis of sheep granulosa cells under thermal stress. Int J Mol Sci. (2014) 15:21090–104. doi: 10.3390/ijms151121090
36. Su J, Wang Y, Xing X, Zhang L, Sun H, Zhang Y. Melatonin significantly improves the developmental competence of bovine somatic cell nuclear transfer embryos. J Pineal Res. (2015) 59:455–68. doi: 10.1111/jpi.12275
37. Zhao XM, Hao HS, Du WH, Zhao SJ, Wang HY, Wang N, et al. Melatonin inhibits apoptosis and improves the developmental potential of vitrified bovine oocytes. J Pineal Res. (2016) 60:132–41. doi: 10.1111/jpi.12290
38. Yong W, Ma H, Na M, Gao T, Zhang Y, Hao L, et al. Roles of melatonin in the field of reproductive medicine. Biomed Pharmacother. (2021) 144:112001. doi: 10.1016/j.biopha.2021.112001
39. Mou Q, Yang YW, Chen L, Fang T, Yao YC, Du ZQ, et al. Melatonin mitigates chloroquine-induced defects in porcine immature Sertoli cells. Theriogenology. (2022) 177:1–10. doi: 10.1016/j.theriogenology.2021.10.005
40. Pal Chowdhury J, Haldar C. Stress associated ovarian dysfunctions in a seasonal breeder Funambulus pennanti: role of glucocorticoids and possible amelioration by melatonin. Gen Comp Endocrinol. (2022) 316:113962. doi: 10.1016/j.ygcen.2021.113962
41. Shi F, Qiu J, Zhang S, Zhao X, Feng D, Feng X. Exogenous melatonin protects preimplantation embryo development from decabromodiphenyl ethane-induced circadian rhythm disorder and endogenous melatonin reduction. Environ Pollut. (2022) 292:118445. doi: 10.1016/j.envpol.2021.118445
42. Zhu T, Guan S, Lv D, Zhao M, Yan L, Shi L, et al. Corrigendum: Melatonin modulates lipid metabolism in porcine cumulus-oocyte complex via its receptors. Front Cell Dev Biol. (2021) 9:727612. doi: 10.3389/fcell.2021.727612
43. Li C, He X, Huang Z, Han L, Wu X, Li L, et al. Melatonin ameliorates the advanced maternal age-associated meiotic defects in oocytes through the SIRT2-dependent H4K16 deacetylation pathway. Aging. (2020) 12:1610–23. doi: 10.18632/aging.102703
44. Chesneau D, Guillaume D, Chemineau P, Malpaux B. Continuous light after 2 months of long days stimulates ram testis volume and increases fertility in spring. Animal. (2017) 11:1189–95. doi: 10.1017/S1751731116002299
45. Abecia JA, Chemineau P, Keller M, Delgadillo JA. Extended day length in late winter/early spring, with a return to natural day length of shorter duration, increased plasma testosterone and sexual performance in rams with or without melatonin implants. Reprod Domest Anim. (2017) 52:851–6. doi: 10.1111/rda.12988
46. Tamura H, Takasaki A, Taketani T, Tanabe M, Kizuka F, Lee L, et al. The role of melatonin as an antioxidant in the follicle. J Ovarian Res. (2012) 5:5. doi: 10.1186/1757-2215-5-5
47. Yang L, Chen Y, Liu Y, Xing Y, Miao C, Zhao Y, et al. The role of oxidative stress and natural antioxidants in ovarian aging. Front Pharmacol. (2020) 11:617843. doi: 10.3389/fphar.2020.617843
48. Deng SL, Zhang BL, Reiter RJ, Liu YX. Melatonin ameliorates inflammation and oxidative stress by suppressing the p38MAPK signaling pathway in LPS-induced sheep orchitis. Antioxidants. (2020) 9:1277. doi: 10.3390/antiox9121277
49. Abd-Elhafeez HH, Hassan AHS, Hussein MT. Melatonin administration provokes the activity of dendritic reticular cells in the seminal vesicle of Soay ram during the non-breeding season. Sci Rep. (2021) 11 872. doi: 10.1038/s41598-020-79529-y
50. Tamura H, Takasaki A, Miwa I, Taniguchi K, Maekawa R, Asada H, et al. Oxidative stress impairs oocyte quality and melatonin protects oocytes from free radical damage and improves fertilization rate. J Pineal Res. (2008) 44:280–7. doi: 10.1111/j.1600-079X.2007.00524.x
51. Hu K .L, Ye X, Wang S, Zhang D. Melatonin application in assisted reproductive technology: a systematic review and meta-analysis of randomized trials. Front Endocrinol. (2020) 11:160. doi: 10.3389/fendo.2020.00160
52. Pool KR, Rickard JP, Pini T, de Graaf SP. Exogenous melatonin advances the ram breeding season and increases testicular function. Sci Rep. (2020) 10:9711. doi: 10.1038/s41598-020-66594-6
53. Satta V, Manca ME, Torres-Rovira L, Succu S, Mereu P, Nehme M, et al. Effects of melatonin administration on seminal plasma metabolites and sperm fertilization competence during the non-reproductive season in ram. Theriogenology. (2018) 115:16–22. doi: 10.1016/j.theriogenology.2018.04.018
54. Abecia JA, Chemineau P, Gomez A, Keller M, Forcada F, Delgadillo JA. Presence of photoperiod-melatonin-induced, sexually-activated rams in spring advances puberty in autumn-born ewe lambs. Anim Reprod Sci. (2016) 170:114–20. doi: 10.1016/j.anireprosci.2016.04.011
55. Pardridge WM, Mietus LJ. Transport of albumin-bound melatonin through the blood-brain barrier. J Neurochem. (1980) 34:1761–3. doi: 10.1111/j.1471-4159.1980.tb11272.x
56. Hu JJ, Xiao LF, Song LL, Ge WB, Duan HW, Jiang Y. The expression of melatonin receptors MT1 and MT2 is regulated by E2 in sheep oviduct. Gen Comp Endocrinol. (2020) 286:113135. doi: 10.1016/j.ygcen.2019.03.004
57. Guo Z, Islam MS, Liu D, Liu G, Lv L, Yang Y, et al. Differential effects of follistatin on porcine oocyte competence and cumulus cell gene expression in vitro. Reprod Domest Anim. (2018) 53:3–10. doi: 10.1111/rda.13035
58. Johnson HE, Dotson JM, Ellis CS, Hill KK. Severe hypotension in an adolescent after a melatonin overdose. J Child Adolesc Psychopharmacol. (2019) 29:726–7. doi: 10.1089/cap.2019.0108
59. Holliman BJ, Chyka PA. Problems in assessment of acute melatonin overdose. South Med J. (1997) 90:451–3. doi: 10.1097/00007611-199704000-00020
60. Yilmaz B, Kutlu S, Mogulkoc R, Canpolat S, Sandal S, Tarakci B, et al. Melatonin inhibits testosterone secretion by acting at hypothalamo-pituitary-gonadal axis in the rat. Neuro Endocrinol Lett. (2000) 21:301–6.
61. Lee S, Jin JX, Taweechaipaisankul A, Kim GA, Ahn C, Lee BC. Melatonin influences the sonic hedgehog signaling pathway in porcine cumulus oocyte complexes. J Pineal Res. (2017) 63. doi: 10.1111/jpi.12424
62. Cao M, Wang Y, Yang F, Li J, Qin X. Melatonin rescues the reproductive toxicity of low-dose glyphosate-based herbicide during mouse oocyte maturation via the GPER signaling pathway. J Pineal Res. (2021) 70:e12718. doi: 10.1111/jpi.12718
63. Kim EH, Ridlo MR, Lee BC, Kim GA. Melatonin-Nrf2 signaling activates peroxisomal activities in porcine cumulus cell-oocyte complexes. Antioxidants. (2020) 9:1080. doi: 10.3390/antiox9111080
64. Guo S, Yang J, Qin J, Qazi IH, Pan B, Zang S, et al. Melatonin promotes in vitro maturation of vitrified-warmed mouse germinal vesicle oocytes, potentially by reducing oxidative stress through the Nrf2 pathway. Animals. (2021) 11:2324. doi: 10.3390/ani11082324
65. El-Sheikh M, Mesalam AA, Song SH, Ko J, Kong IK. Melatonin alleviates the toxicity of high nicotinamide concentrations in oocytes: potential interaction with nicotinamide methylation signaling. Oxid Med Cell Longev. (2021) 2021:5573357. doi: 10.1155/2021/5573357
66. Li Z, Li X, Bi J, Chan MTV, Wu WKK, Shen J. Melatonin protected against the detrimental effects of microRNA-363 in a rat model of vitamin A-associated congenital spinal deformities: involvement of Notch signaling. J Pineal Res. (2019) 66:e12558. doi: 10.1111/jpi.12558
67. Kechiche S, Venditti M, Knani L, Jablonska K, Dziegiel P, Messaoudi I, et al. First evidence of the protective role of melatonin in counteracting cadmium toxicity in the rat ovary via the mTOR pathway. Environ Pollut. (2021) 270:116056. doi: 10.1016/j.envpol.2020.116056
68. Amini H, Rezabakhsh A, Heidarzadeh M, Hassanpour M, Hashemzadeh S, Ghaderi S, et al. An examination of the putative role of melatonin in exosome biogenesis. Front Cell Dev Biol. (2021) 9:686551. doi: 10.3389/fcell.2021.686551
69. Yea JH, Yoon YM, Lee JH, Yun CW, Lee SH. Exosomes isolated from melatonin-stimulated mesenchymal stem cells improve kidney function by regulating inflammation and fibrosis in a chronic kidney disease mouse model. J Tissue Eng. (2021) 12:20417314211059624. doi: 10.1177/20417314211059624
70. Lin PY, Yang D, Chuang CH, Lin H, Chen WJ, Chen CY, et al. Comparative analyses of single-cell transcriptomic profiles between in vitro totipotent blastomere-like cells and in vivo early mouse embryonic cells. Cells. (2021) 10:3111. doi: 10.3390/cells10113111
71. Zhang H, Li C, Wen D, Li R, Lu S, Xu R, et al. Melatonin improves the quality of maternally aged oocytes by maintaining intercellular communication and antioxidant metabolite supply. Redox Biol. (2021) 49:102215. doi: 10.1016/j.redox.2021.102215
72. Wang Y, Xing CH, Zhang HL, Pan ZN, Sun SC. Exposure to nivalenol declines mouse oocyte quality via inducing oxidative stress-related apoptosis and DNA damagedagger. Biol Reprod. (2021) 105:1474–83. doi: 10.1093/biolre/ioab171
73. Xing CH, Wang Y, Liu JC, Pan ZN, Zhang HL, Sun SC, et al. Melatonin reverses mitochondria dysfunction and oxidative stress-induced apoptosis of Sudan I-exposed mouse oocytes. Ecotoxicol Environ Saf . (2021) 225:112783. doi: 10.1016/j.ecoenv.2021.112783
74. Yang CH, Xu JH, Ren QC, Duan T, Mo F, Zhang W. Melatonin promotes secondary hair follicle development of early postnatal cashmere goat and improves cashmere quantity and quality by enhancing antioxidant capacity and suppressing apoptosis. J Pineal Res. (2019) 67:e12569. doi: 10.1111/jpi.12569
75. Hansell JA, Richter HG, Camm EJ, Herrera EA, Blanco CE, Villamor E, et al. Maternal melatonin: effective intervention against developmental programming of cardiovascular dysfunction in adult offspring of complicated pregnancy. J Pineal Res. (2022) 72:e12766. doi: 10.1111/jpi.12766
76. Gunata M, Parlakpinar H, Acet HA. Melatonin: a review of its potential functions and effects on neurological diseases. Rev Neurol. (2020) 176:148–65. doi: 10.1016/j.neurol.2019.07.025
77. Ivanov DO, Evsyukova II, Mironova ES, Polyakova VO, Kvetnoy IM, Nasyrov RA. Maternal melatonin deficiency leads to endocrine pathologies in children in early ontogenesis. Int J Mol Sci. (2021) 22:2058. doi: 10.3390/ijms22042058
Keywords: animal welfare, ewe, follicle, meta-analysis, development
Citation: Chen Y, Shan X, Jiang H and Guo Z (2022) Exogenous Melatonin Directly and Indirectly Influences Sheep Oocytes. Front. Vet. Sci. 9:903195. doi: 10.3389/fvets.2022.903195
Received: 24 March 2022; Accepted: 05 May 2022;
Published: 26 May 2022.
Edited by:
Valeria Pasciu, University of Sassari, ItalyReviewed by:
Jaime Palomino, Universidad Bernardo O'Higgins, ChileIgnacio Contreras Solís, University of Sassari, Italy
Copyright © 2022 Chen, Shan, Jiang and Guo. This is an open-access article distributed under the terms of the Creative Commons Attribution License (CC BY). The use, distribution or reproduction in other forums is permitted, provided the original author(s) and the copyright owner(s) are credited and that the original publication in this journal is cited, in accordance with accepted academic practice. No use, distribution or reproduction is permitted which does not comply with these terms.
*Correspondence: Huaizhi Jiang, MTM3NTYyOTM4NThAMTI2LmNvbQ==; Zhenhua Guo, Z3poaDAwQDE2My5jb20=
†These authors have contributed equally to this work