- 1State Key Laboratory of Animal Nutrition, Institute of Animal Sciences, Chinese Academy of Agricultural Sciences, Beijing, China
- 2Joint Laboratory on Integrated Crop-Tree-Livestock Systems of the Chinese Academy of Agricultural Sciences (CAAS), Ethiopian Institute of Agricultural Research (EIAR) and World Agroforestry Centre (ICRAF), Beijing, China
- 3Hunan Co-Innovation Center of Safety Animal Production, Changsha, China
The objective of this study was to evaluate the effects of supplying ruminally protected Lys (RPL) and ruminally protected Met (RPM) to transition cows' diets on the efficiency of subsequent lactation. A total of 120 prepartum Holstein cows were assigned into four treatments blocked by the anticipated calving date, previous lactation milk yield, number of lactations, and body condition score and fed either RPL, RPM, or the combination (RPML) or control diet (CON) throughout the transition period (3 weeks before till 3 weeks after calving). From 22 to 150 days in milk (DIM), all animals (100 cows) were fed a combination of RPM and RPL (0.17% RPM and 0.41% RPL of DM; n = 25 cows/treatment) as follows; CON–RPML, RPM–RPML, RPL–RPML, and RPML–RPML. Milk production and dry matter intake (DMI) were measured daily; milk and blood samples were taken at 21, 30, 60, 90, 120, and 150 DIM. Supplemented amino acids (AA) were mixed with the premix and added to the total mixed ration during the experiment. DMI (p < 0.001) and energy-corrected milk (ECM, p = 0.04) were higher for cows that were fed RPML–RPML than other cows. Compared with CON–RPML, yields of milk total protein, lactose, and nitrogen efficiency were increased (p < 0.01), whereas milk urea nitrogen (MUN; p = 0.002) was decreased for other treatments. However, supplemental AA did not affect milk lactose percentage, fat yield, feed efficiency, or serum total protein concentration (p > 0.10). Transition cows that consumed AA had a greater peak of milk yield (p < 0.01), as well as quickly reached the peak of milk (p < 0.004). There were differences in β-hydroxybutyrate concentration during the early lactation, with a lower level for AA groups (p < 0.05), and the difference faded with the progression of lactation (p > 0.10). Fertility efficiency as measured by pregnancy rate was improved by supplemental AA during the perinatal period (p < 0.05). In conclusion, transition cows consumed RPM and RPL, increased post-calving DMI, milk production, milk protein yield, nitrogen efficiency, and improved fertility performance.
Introduction
Balanced nutrition is necessary for the body to perform its various functions optimally while maintaining life. Nutrition deficiency causes a reduction of fertility (1, 2), alteration of embryonical growth, or fetal development at multiple stages of gestation (3, 4), and it may even lead to pregnancy loss (5, 6), mainly of these malnutrition deficiencies, which occurs during the periparturient period. There are several common nutritional strategies to improve reproduction in dairy cows without any adverse effect on lactation efficiency, such as feeding high-quality forages, supplementation with amino acids (AA, especially in a rumen-protected form), direct-fed microbial feed during the transition period, or supplemental fats in the diet which are one of the most popular ways to improve energy intake in dairy cows. Amino acid nutrition to dairy cattle is recognized as one of the promising nutritional strategies that have an essential role in reproduction, in addition to the benefit of milk production and other performance aspects. Studies have been proved that several AAs are concentrated in the oviductal and uterine histotroph and the amniotic and allantoic fluids, in comparison with circulating AA concentrations, and many studies have proposed a significant role for these increased AA concentrations in normal embryonic growth and fetal development (7, 8). Methionine (Met) is an essential amino acid (EAA) that could limit reproduction in milking dairy cows (9), in addition to lysine and arginine as well. In mammals, a Met codon is used to initiate most protein synthesis leading to a fundamental role for this AA in all portions of mammalian cellular functions (10, 11).
Studies have evaluated the effects of supplied rumen-protected amino acids (RPAAs), such as rumen-protected lysine (RPL) and rumen-protected methionine (RPM), on milk production and composition; some indicated an increase in milk yield, milk protein percentage, and usually, milk protein yield (12–18), often but not general. For reproduction, lactating cows' diets supplied with RPM or RPL have had positive reproductive outcomes (9, 19) or minor or no effects on reproductive outcomes (20, 21). Some studies have correlated Met concentrations with optimal development in the early embryonic period (22, 23). In addition, the recent studies in both sheep (24, 25) and cattle (7, 26) have confirmed that Met is concentrated in uterine and embryonic fluids, emphasizing the role of increased uterine Met in normal embryonic development and survival. Despite these studies that associate Met and Lys with milk production and reproductive processes, to our knowledge, no previous studies have assessed the effects of feeding a combination of RPL and Met on fertility and pregnancy rate in lactating Holstein dairy cows.
Research on transition and lactating dairy and beef cows (13, 14, 27, 28) has reported improvement in health, milk production, animal performance, and liver function, as well as subsequent offspring due to the dietary provision of RPM and RPL, in some cases but not all. This study hypothesized that supplementing either RPL or RPM or their combination to transition cows and continual feeding their combination during the high milking period would enhance the production and fertility in lactating dairy cows. We specifically hypothesized that RPL and RPM feeding would increase milk protein content and production, as observed in this study during the transition period (18, 29), leading to improved nitrogen utilization. Further, we hypothesized that providing a combination of RPL and RPM would accelerate cows to be conceived, this leads to an increase in the conception rate, and therefore, there would be an increase in the pregnancy rate. The objectives were to evaluate the effects of daily TMR feeding with a combination of RPL and RPM on milk production and composition, nitrogen efficiency, and pregnancy rate.
The results have been presented in partial form during the 2021 Annual Meeting of the American Dairy Science Association (ADSA), Abstract No# 245 “Supplementing ruminally protected methionine or lysine improved milk production in transition cows” and (18, 29, 30).
Materials and Methods
Experimental Design and Animals
This research is a part of a large project to study the effect of ruminally protected amino acids on transition dairy cows and their neonatal calves. Cows, calves management, sampling, analysis procedures, and results were previously reported (18, 29, 30). Briefly, this study lasted from 3 weeks before calving until 5 months after calving (approximately 6 months), the study on the dairy cows contained two majors stages; (1) transition period (3 weeks before through 3 weeks after calving), (2) high milking period (from 22 to 150 days in milk), besides the trial on neonatal calves born from the transition cows that were used in this study. During the transition period, a total of 120 late-pregnant Holstein dairy cows were used, and the cows were distributed into eight groups (n = 15), and two groups were assigned to each of four experimental treatments (n = 30 cows/treatment). Cows were fed either the control diet (CON), or RPL, or RPM or two in combination (RPML). Cows were selected and assigned to treatment based on the days of pregnancy (250 ± 2 days, p = 0.84), previous lactation milk production (11,512 ± 1,837 kg; 305-day milk yield, p = 0.90), parity (3.09 ± 1.56, p = 0.94), and body condition score (BCS; 3.58 ± 0.26, P = 0.86). The experiment was conducted as a completely randomized design with treatments arranged in a 2 × 2 factorial. The cows were fed their respective diets starting at about 3 weeks [transition period, (25.0 ± 3.31 days) before the anticipated calving to 3 weeks post-calving (24.0 ± 3.31 days)]. A statistical power analysis was performed before the study using G–power 3.1 software (31), and power = 0.95, α = 0.05, and 0.32 effect size indicated a minimum group size of 100 cows, more details in our previous studies (18, 30). Until the end of the first stage of the study (about 21 DIM), some cows were culled, diseased, sold, or died, and the cows removed from the herd for any reason were excluded from the statistical analysis [refer to our previous paper of Elsaadawy et al. (18)].
In the second stage of the study (during the high milking period), a total of 100 dairy cows have completed the transition period and continued and entered the second stage of the study (25 cows/treatment). The cows were distributed during the high milking period based on their treatment during the transition period as follows: CON–RPML, RPM–RPML, RPL–RPML, and RPML–RPML. All cows were fed a combination of RPM and RPL at the rate of 0.17% RPM and 0.41% RPL of DM during the second stage of the study. During the period between 22 and 150 DIM, some cows were culled, diseased, sold, or died, and the cows removed from the herd for any reason were excluded from the statistical analysis (details in the RESULT Section).
Cows were fed total mixed rations (TMRs) ad libitum four times per day at 06:00, 12:00, 18:00, and 24:00 h and milked in the automatic rotary parlor four times per day just prior to being fed, at about 6-h intervals. Feed offered was managed to achieve around 5% refusal each day. The diet's TMR composition, chemical analyses, and EAA profile were illustrated (Tables 1–3). Cows were housed in a ventilated, four-row, free-stall barn (center feed alley with two rows of stalls on each side). After calving, they were moved to the colostrum barn for 1–3 days and then relocated to the milking barn. Manure was removed by mechanical scraper 4 times per day when the cows were at the milking parlor at 06:00, 12:00, 18:00, and 24:00 h. Sand bedding was groomed four times/day, and new sand was added two times/day at 06:00 and 12:00 h. Cows had access to ad libitum freshwater during the trial. The trial was conducted at the AustAsia Dairy Group, Shandong, China. Temperature humidity index (THI) was measured for the cows' barns using humidity detector PCE-HT 112 (PCE Instruments Ltd, UK). The temperature humidity index was calculated according to the equation of the National Research Council (32).
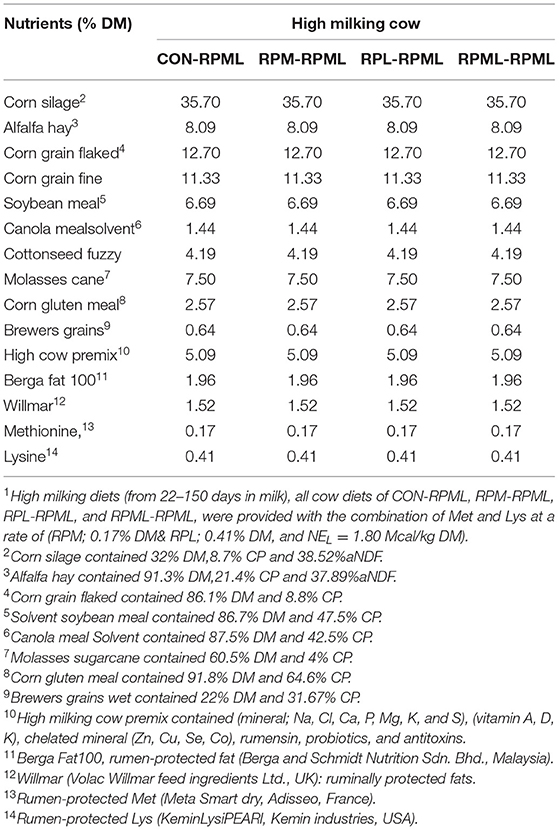
Table 1. Ingredients of the total mixed ration (TMR) fed to Holstein dairy cows during the high milking periods1.
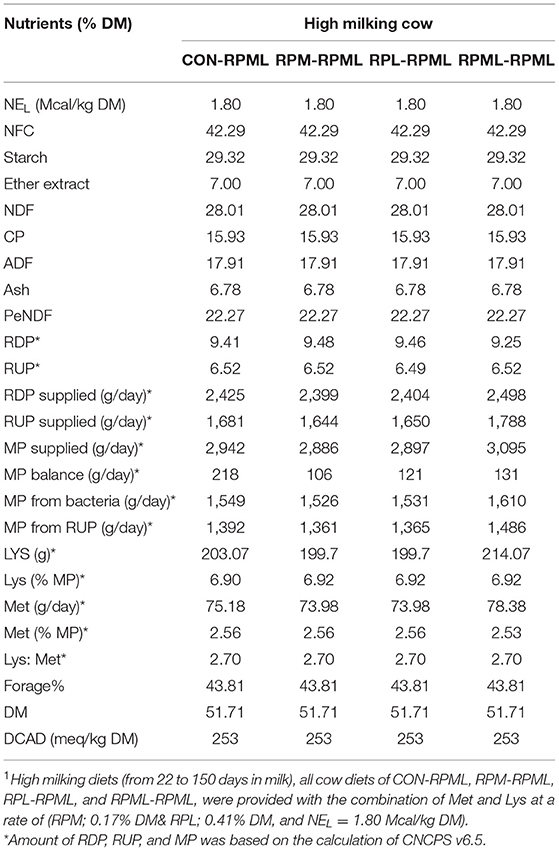
Table 2. Chemical composition of the total mixed ration (TMR) fed to Holstein dairy cows during the High milking periods1.
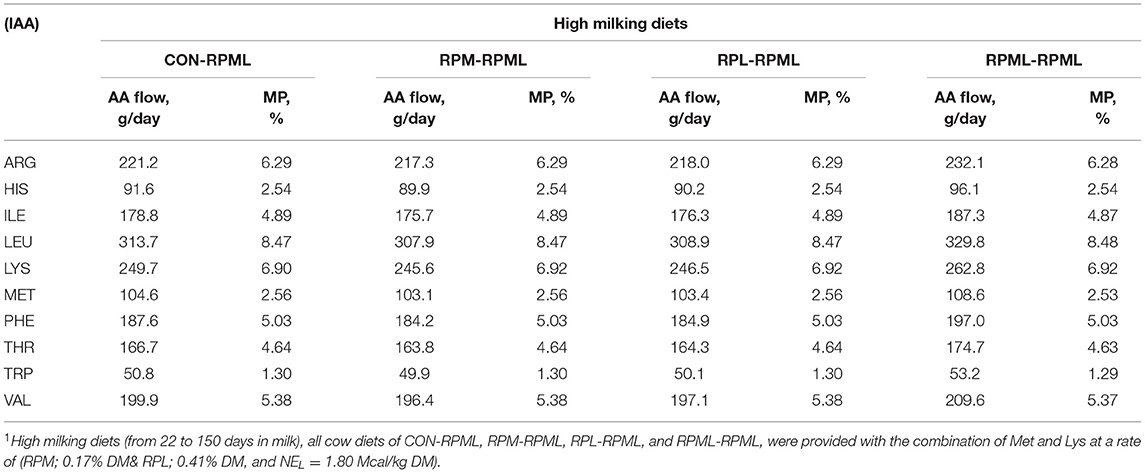
Table 3. Duodenal flows of the indispensable amino acids (IAA) based on the actual consumed DMI during high milking period1 using CNCPS v6.5.
where Tdb–dry bulb temperature (°C) and RH—relative humidity (%).
Ration Formulation
As discussed in our previous studies (18, 30), TMR was formulated using Cornell net carbohydrate and protein system [(CNCPS) v.6.5.5 (33)] as executed in AMTS.Cattle.Professional v.4.7.2 (2016, AMTS LLC, USA) to meet or exceed the requirements of dairy cows (Tables 1, 2). The high-lactating diet was formulated for cows at 50 DIM, 600 kg of BW, 3.25 BCS, producing 55 kg/day milk with a target of 4.0% of milk fat and 3.16% of true protein, and a predicted DMI of 26 kg/day. The dietary content of Met and Lys was balanced according to the recommendations of CNCPS v6.5; to be within 6.15%−7.2% MP-Lys and 2.1%−2.35% MP-Met to meet the Lys-to-Met ratio within the range of 2.7:1 to 3:1. Ruminally protected amino acids were mixed with the premix using a mixer and then top-dressed on the TMR and mixed into the TMR 1 time/day at 06:00 h during the experiment using a Supreme Vertical Feed Mixer (Supreme International Limited, USA). The Goke intelligent feeding system with measurable feed intakes was used (GokeAgri, Beijing, China). Dietary was provided with RPL and RPM at 0.41 and 0.17% of DM, respectively (Tables 1, 2).
The isopropyl ester of 2-hydroxy-4-(methylthio)-butanoic acid (HMBi) was provided in a dry powder form (MetaSmart, Adisseo, France). According to the manufacturer, the product contains 57% HMBi, which is 78% Met equivalency, and 50% of it is absorbed via the rumen wall (34). Thus, each gram of the product gives 0.22 g of metabolizable Met. The RPL was provided as a dry powder containing 47.5% L-Lys monohydrochloride (3.2.3) with 70% of bioavailability, according to the manufacturer. Thus, each gram of the product provided 0.33 g of metabolizable Lys-HCl (LysiPEARL, Kemin Industries, USA).
Sampling, Measurements, and Analysis
Feed and Physically Effective NDF
Analysis techniques have been described in detail in our previous study (18). In general, feed offered and refusal were measured daily for each treatment. Diets and the major dietary ingredients were sampled and analyzed weekly and used to calculate nutrient concentrations. Feed samples were dried at 105°C for 4 h to determine the DM percentage and stored at –20°C for further analysis. The dried feed samples were ground through a 1-mm screen before analysis using a Cyclotec 1093 Mill (Tecator 1093, Tecator AB, Höganäs, Sweden). Feed samples were further dried at 105°C for 2 h to determine the absolute DM. Chemical analyses of dry matter (DM), crude protein (CP), ether extract (EE), neutral detergent fiber (aNDF), and acid detergent fiber (ADF) were performed using wet chemistry techniques at the State Key Laboratory of Animal Nutrition, Institute of Animal Sciences of Chinese Academy of Agricultural Sciences (CAAS), Beijing, China.
The content of CP (N × 6.25) in feed samples was analyzed using the macro-Kjeldahl nitrogen test [AOAC International, (35); method 984.13.4.09] with a Kjeltec digester 20 and a Kjeltec System 1026 distilling unit (Tecator AB). Ether extract content was analyzed using a soxhlet HT6 apparatus (Tecator AB) according to AOAC International (2000; 920.39), and ADF and aNDF were analyzed according to Van Soest et al. (36) using alpha-amylase with the addition of sodium sulfite. Ash content was analyzed by incineration at 550°C for 5 h, and the organic matter (OM) content was calculated by subtracting ash from 100. Non-fiber carbohydrates (NFC) were calculated by the difference according to the National Research Council (17).
Energy balance (EB), dietary energy density, and MP balance were determined based on the actual DMI of individual ingredients and their respective energy value and MP based on the CNCPS feed library.
Requirements for net energy (NE) fractions were calculated as
The requirement for net energy of lactation (NEL, Mcal/day):
The net energy requirement for pregnancy (NEY; Mcal/day) was calculated as follows
The sum of individual requirements was as follows:
Energy balance (EB, Mcal/day) was calculated as the following equation:
Energy corrected milk (ECM 3.5% fat) and fat-corrected milk (FCM) were calculated according to (37), as the following equations:
Physical effective NDF of the TMR (% of DM) was determined weekly using a Penn State Particle Separator (PSPS) sieve, which contains four sieve layers, upper (19-mm pore size), middle (8-mm pore size), lower (4-mm pore size) sieves, and the pan, and the peNDF content of the TMR was estimated by multiplying the NDF content of the feed by the percent of feed retained on PSPS sieve according to the previous description (38).
Blood Samples
Duplicate blood samples of approximately 15 ml were collected via a coccygeal vessel from individual cows on 21, 30, 60, 90, 120, and 150 DIM. Samples were collected into evacuated tubes containing clot activators of silica particles for serum separation (Jiangsu Kangjian Medical Apparatus Co., Ltd, China). Blood samples were then centrifuged at 3,000 × g for 15 min at 4°C for separation of the serum, and the supernates were stored at −20°C until further analysis. Blood BHB concentrations were measured immediately after collecting the blood using the BHBCheck Plus blood ketone test (PortaCheck, Inc. Moorestown, NJ, USA). Serum total protein concentrations were analyzed using a digital temperature-compensating refractometer (Model 300027, SPER Scientific Ltd., Scottsdale, Arizona, USA). Before testing each sample, the refractometer prism was cleaned and the refractometer was calibrated with distilled water.
Milk Samples and Income Over Cost Calculation
Milk sampling has been detailed in our previous paper (18). Briefly, milk samples were collected on 21, 30, 60, 90, 120, and 150 DIM. Each milk sample was preserved with bronopol-B2 preservative (D&F Control Systems Inc., Dublin, ON, Canada) and stored at 4°C with subsequent analysis for milk fat, total protein, lactose, total solids (TS), and milk urea nitrogen (MUN) using a mid-infrared machine (MilkoScan FT3; Foss-600, Foss Analytics, Hillerød, Denmark). Somatic cell count (SCC) was analyzed using a Somatic Cells Analyzer (Foss, Hillerød, Denmark). Somatic cell score (SCS) was then obtained by transforming SCC using the equation (39). Daily milk production for individual cows was automatically recorded using parlor milk weight sensors, and data were transferred to the dairy herd management software [(DelPro™ Software v5.4 (DeLaval Corporation, Sweden)]. Daily data were then summarized as average weekly and monthly data points for statistical analysis.
Milk price was calculated using the milk price of $19.78/cwt (cwt = hundredweight of milk), protein price of $7.12/lb, and fat price of $1.97/lb [U.S. Department Of Agriculture (USDA): Announcement of Advanced Pricing and Pricing Factors for August-2020] https://www.ams.usda.gov/mnreports/dymadvancedprices.pdf and protein yield and fat yield corresponding to each treatment.
The cost of the feed per each 1 kg DMI was calculated according to the actual purchase prices of each feedstuff ingredient during the experiment.
Body Condition Score
The evaluation of BCS has been described in detail in our previous study (18). Briefly, BCS was assessed for individual cows by the same two trained professionals on 21, 30, 60, 90, 120, and 150 DIM. A combination of visual appraisal and manual palpation of different body areas (between the hooks, hooks and pins, tailhead to pins, transverse processes, and rumen fill) was evaluated on a 1–5-point scale (17).
Fertility Performance
Reproductive parameters of DIM at 1st insemination, heat detection rate (HDR), conception rate (CR), and pregnancy rate (PR) were obtained by DelPro™ 5.4 Herd Management Software (DeLaval Corporation, Sweden). Cows on all treatments had a voluntary waiting period of 56 days post-calving. A double Ovsynch protocol was performed, which proceeded through two OvSynch protocols 7 days apart and was followed by timed artificial insemination (TAI) after the second protocol (40). Ultrasonography checking of the ovaries was conducted as a regular procedure in the reproduction management of dairy cattle herds using a portable ultrasound fitted with a 7.5 MHz linear array transducer (Shenzhen Bondway Electronics Co., Ltd, Shenzhen, China). Pregnancy diagnosis was performed using ultrasonography to evaluate embryonic and amniotic vesicle size to detect a viable conceptus on day 32 after artificial insemination (AI). Cows diagnosed as pregnant on day 32 were rechecked on days 47 and 65. The DIM at 1st insemination was defined as the days between calving and the first service. Heat detection rate is the percentage of cows inseminated over a 21-day period divided by the number of cows eligible to be bred over those 21 days. Conception rate is defined as the percentage of pregnant cows divided by the number of cows inseminated during 21 days. Pregnancy rate (21-day interval) was calculated by multiplying the heat detection rate by the conception rate [DelPro™ 5.4 Herd Management Software (DeLaval Corporation, Sweden)].
Statistical Analyses
The high milking period was restricted from 22 to 150 DIM. Daily DMI and milk yield were condensed to weekly and monthly averages. Data were analyzed using a model with treatment effects (RPL and RPM), time, and their interaction using PROC MIXED of SAS (v. 9.4, SAS Institute Inc., USA, 2013). The MIXED statistical model used for analysis was as follows:
where Yijk was the dependent, continuous variable, μ was the overall mean; Di was the fixed effect of diet (i = 1, 2, 3, or 4); Aij was the random effect of the jth cow within the ith treatment; Tk was the fixed effect of time (day); DTik was the interaction effect of diet and time; and εijk was the residual error. The Kenward-Roger option was used for computing the denominator degrees of freedom for testing hypotheses (41). The distribution of the residuals was assessed to determine normality and homoscedasticity. A log transformation was used for the milk SCC variable to convert it to SCS and to enhance the homogeneity of the distribution of residuals.
Fertility data were analyzed by chi-square test using SAS PROC FREQ procedures. SAS linear regression with PROC REG procedure was conducted to indicate the relationship between either peak of milk production or DIM at the peak of milk (dependent variable), and average DMI consumed during the fresh period (between 1 and 21 DIM, independent variable), with 95% prediction intervals that were calculated for various DMI, DIM, and milk yield.
Pearson correlation analysis using SAS PROC CORR procedure was performed to study the relationship between the consumed DMI during the fresh period (first 3 weeks of lactation) and the peak of milk production and DIM at the peak of milk for dairy cows fed either RPL or RPM or the combination throughout the transition period. The least-squares means were compared using the least significant difference (LSD), and statistical differences were declared significant at p ≤ 0.05. A tendency was determined at p > 0.05 to p ≤ 0.10.
Results
Dry Matter Intake, Barn Characteristics, Cow Exclusions, and Pen State Particle Separator
There were significant Trt × Time interactions (p < 0.001), as the DMI of cows that fed RPML–RPML supplements was always greater (27 kg DMI, p < 0.001) than other treatments at all time points: 25.8, 25.3, and 25.4, for CON–RPML, RPM–RPML, and RPL–RPML, respectively (Table 4, Figure 1). There were time effects for DMI, in which DMI was higher at 90 and 120 DIM (26.7 kg) than other time points 24.03, 25.8, and 26.1 kg for feed consumption at 30, 60, and 150 DIM, respectively (p < 0.001). The physical characteristics of high milking TMR were (DM bases, mean ± SD) 10.9 ± 2.5% on upper, 36.9 ± 2.1% on middle, 19.4 ± 1.3% on lower sieves, and 32.8 ± 2.8% in the pan. The average THI was 56.3 ± 4.2 for the barn during the high milking period. A total of five cows were excluded from the statistical analysis during the second stage of the study due to lameness (RPML–RPML, n = 1, at 84 DIM), due to mastitis (CON–RPML, n = 1, at 144 DIM), breaking (CON–RPML, n = 1, at 110 DIM), or digestive problems (RPM–RPML, n = 1, at 138 DIM; and RPL–RPML, n = 1, at 96 DIM). So, in total, 95 animals were used for the statistical analysis as follows: CON–RPML = 23 cows, RPM–RPML = 24 cows, RPL–RPML = 24 cows, and RPML–RPML = 24 cows.
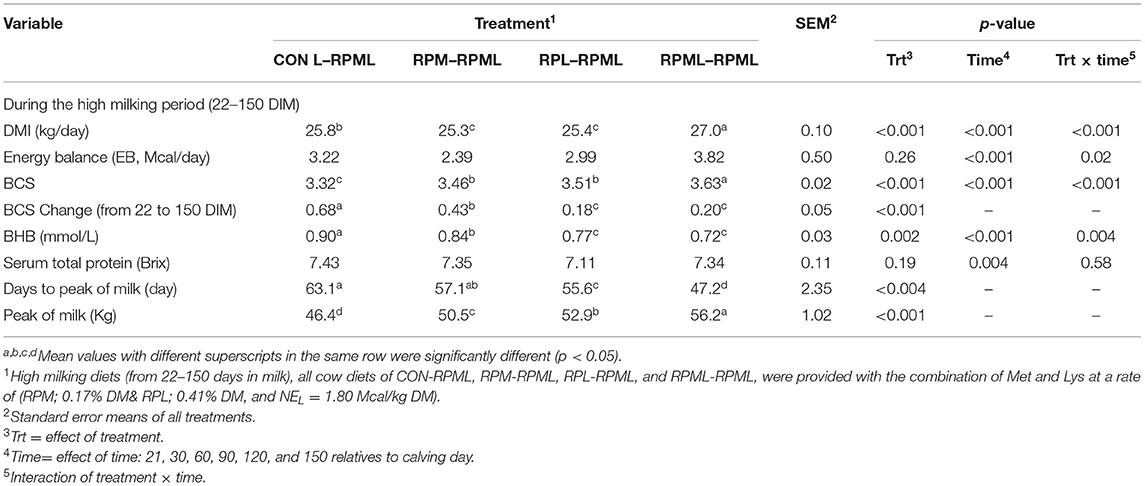
Table 4. Feeding ruminally protected Met and Lys to transition dairy cows and its subsequent effect on post-calving performance.
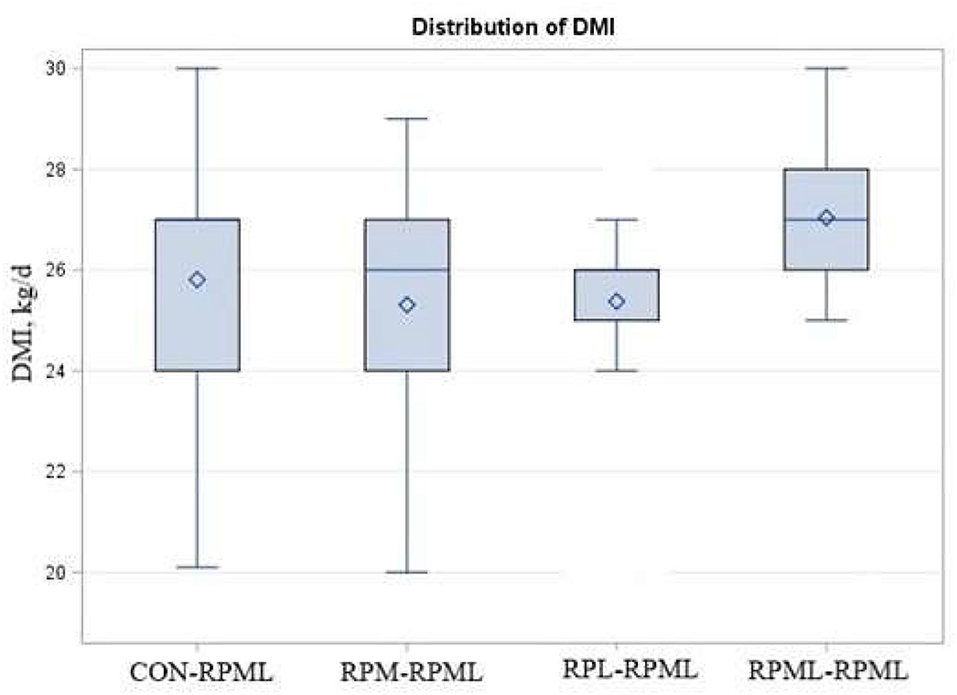
Figure 1. Effect of supply ruminally protected Met and Lys to transition and milking dairy cows on dry matter intake. Values are means; standard errors represented by vertical bars.
Metabolizable Protein Balance and CNCPS Evaluation of Diets
The mean chemical compositions of feed ingredients throughout the experiment were used to evaluate the high milking diets by the CNCPS model. Unlike early lactation, high milking metabolizable protein (MP) balance was positive across all rations. The CON–RPML diet provided an average of 112, 97, and 87 g/day more MP balance than either RPM–RPML, RPL–RPML, and RPML–RPML diet, respectively (Table 2).
Effects of Amino Acids Supply on Energy Balance and Body Condition Score
The main effects of supplementing a combination of Lys and Met during the high milking period on EB, BCS, and BCS changes are presented (Table 4). Energy balance was not differed between treatments during the high milking period (p = 0.26). There was a time effect on EB (p < 0.001), in which EB at 30 DIM was lower than other time points –0.41 vs. 2.5, 3.79, 4.58, and 5.06 Mcal/day for EB at 30, 60, 90, 120, and 150 DIM, respectively (p < 0.001), and lower (p < 0.05) EB at 60 DIM (2.5 Mcal) in comparison with EB at 120 DIM (4.58 Mcal) and 150 DIM (5.06 Mcal). There were Trt × Time interactions for EB (p = 0.02; Table 4), because cows consumed RPL–RPML (1.84 Mcal) and RPML–RPML (1.44 Mcal) had a greater (p < 0.05) EB than those cows that received CON–RPML (−2.32 Mcal) or RPM–RPML (−2.59 Mcal) at 30 DIM; and a tendency (p = 0.09) at 60 DIM for greater EB for RPML–RPML (3.69 Mcal) compared with RPM–RPML (0.93 Mcal); and cows that fed CON–RPML (6.11 Mcal) had a greater (p = 0.02) EB than cows in RPL–RPML group (2.16 Mcal) at 120 DIM, and also cows in RPML–RPML (5.15 Mcal) had a greater EB (p = 0.04) than cows in RPL–RPML (2.16 Mcal) at 120 DIM; and a tendency (p = 0.07) for greater EB for CON–RPML (6.94 Mcal) compared with cows in RPM–RPML group (3.99 Mcal) at 150 DIM (Table 4).
Transition cows fed supplemental AA diets still had greater BCS than other cows (p < 0.001) 3.46, 3.51, 3.63, and 3.32, for RPM–RPML, RPL–RPML, RPML–RPML, and CON–RPML, respectively (Table 4, Figure 2). There were time effects for BCS (p < 0.001), as the BCS increased with the progression of lactation 3.25, 3.41, 3.52, 3.61, and 3.63 for 30, 60, 90, 120, and 150 DIM, respectively (p < 0.001). There were Trt × Time interactions for BCS (p < 0.001; Table 4, Figure 2), because cows consumed RPM–RPML (3.18) or RPL–RPML (3.41) or RPML–RPML (3.51) had a greater (p < 0.001) BCS than those cows that received CON–RPML (2.89) at 30 DIM; and at 60 DIM, cows fed RPL–RPML (3.46) or RPML–RPML (3.57) diets had a greater (p < 0.001) BCS than those cows that fed CON–RPML (3.27) at 30 DIM; and at 90 DIM, cows consumed RPM–RPML (3.56) or RPL–RPML (3.52) or RPML–RPML (3.65) had a greater (p < 0.001) BCS than those cows that received CON–RPML (3.33). The change of BCS from 22 to 150 DIM was affected (p < 0.001) by RPAA supply during transition period and that effect was continuing to appear on subsequent lactation during the high milking period and later on.
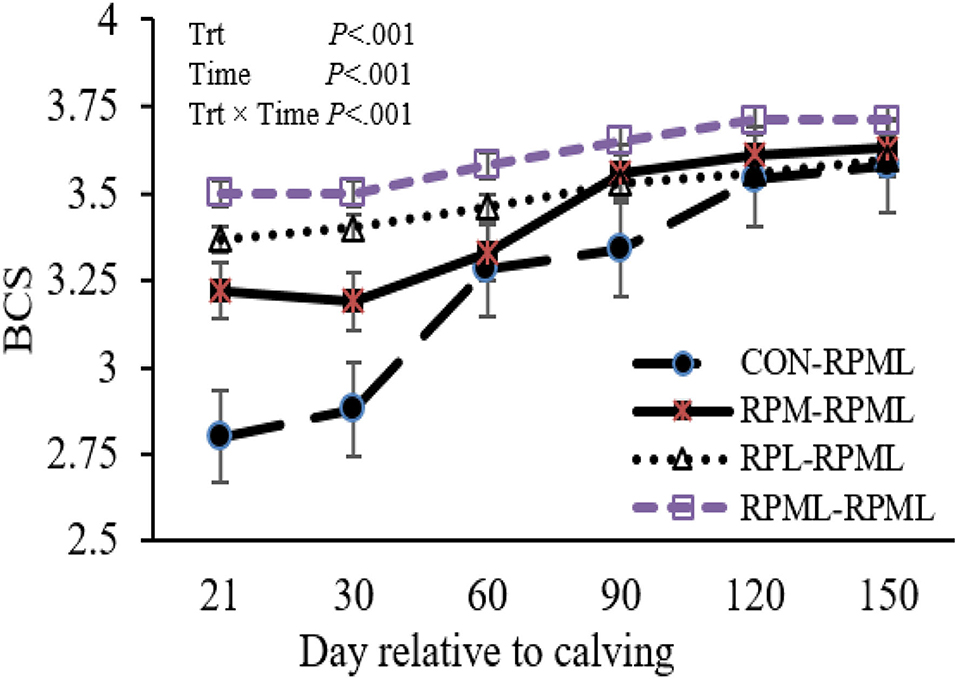
Figure 2. Effect of supply ruminally protected Met and Lys to transition and milking dairy cows on body condition score. Values are means; standard errors are represented by vertical bars.
Effect of Amino Acids Supply on Days to Peak and Peak of Milk
There was a significant (p < 0.004) effect of AA supply on days to reach the peak of milk (Table 4, Figure 3), as transition cows fed RPML–RPML (47.2 days) quickly (p < 0.05) reached the peak of milk than CON–RPML (63.1 days), RPM–RPML (57.1 days), and RPL–RPML (55.6 days); there was a difference (p = 0.004) between RPL–RPML (55.6 days) and CON–RPML (63.1 days); and there was a tendency (p = 0.09) between RPM–RPML (57.1 days) and CON–RPML (63.1 days).
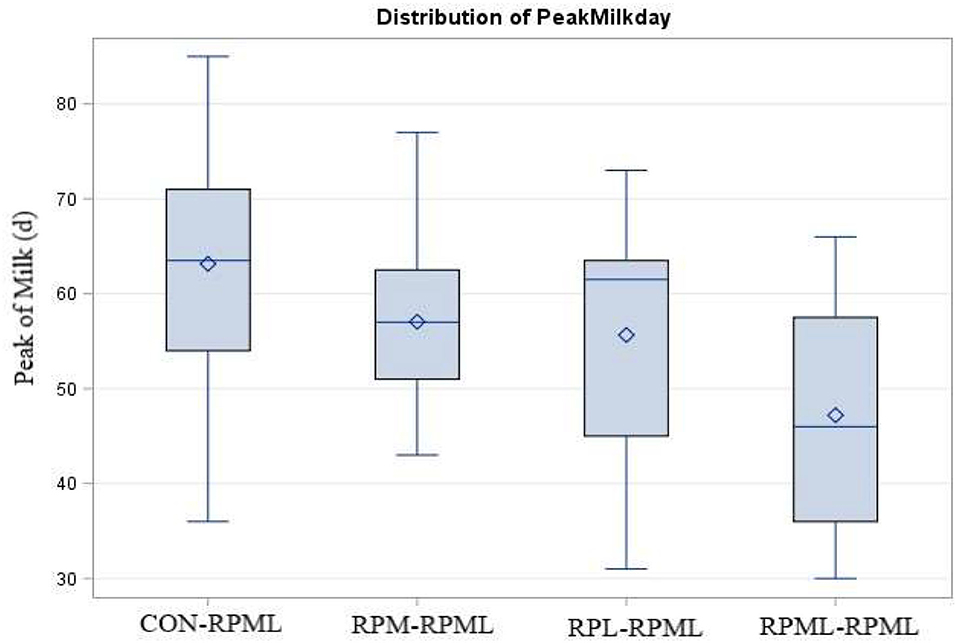
Figure 3. Effect of supply ruminally protected Met and Lys to transition and milking dairy cows on the peak of milk production in days. Values are means; standard errors are represented by vertical bars.
The results of regression analysis showed that there were significantly (p < 0.0001) positive linear associations between DMI consumed during the fresh period (first 21 DIM) and the peak of milk production (r = 0.65, Figure 4). Linear regression of DIM to reach the peak of milk by the average DMI consumed during the fresh period indicated a significance (r = 0.41, p < 0.0001). The correlation analysis results showed a strong correlation between the average DMI consumed during the first 3 weeks of lactation and the peak of milk production (r = 0.67, p < 0.0001). There was a negative linear correlation between DMI and DIM to reach the peak of milk (r = −0.48, p < 0.0001). There was a negative linear correlation between the peak of milk yield and DIM to reach the peak of milk (r = −0.63, p < 0.0001).
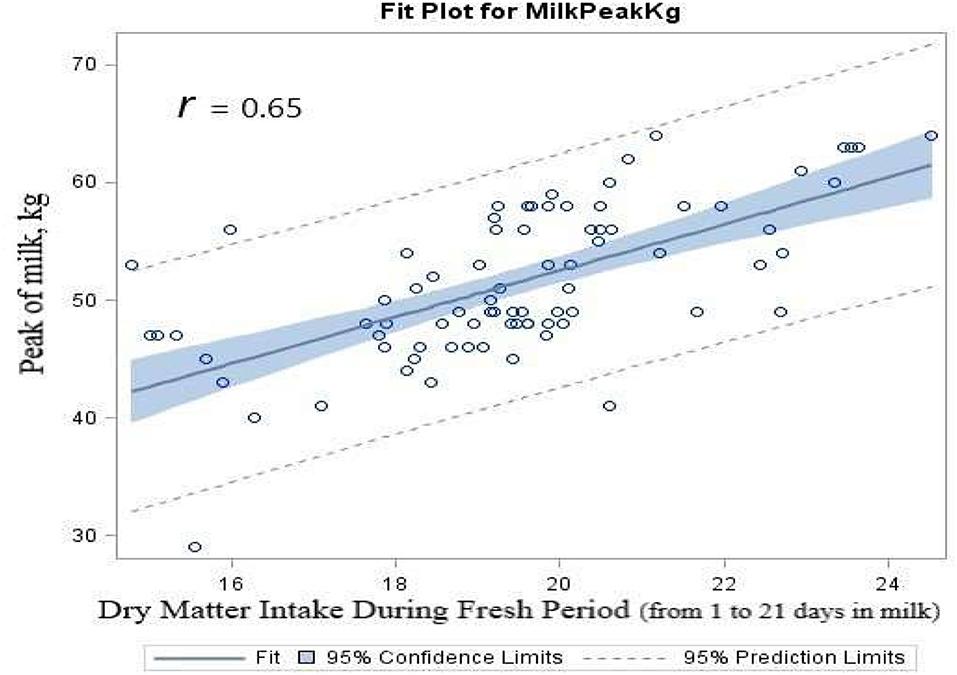
Figure 4. Scatterplot of the peak of milk (kg) by dry matter intake that was consumed during the fresh period (first 3 weeks of lactation) overlaid with the fit line, a 95% confidence band and lower and upper 95% prediction limited, for dairy cows fed either ruminally protected Lys or Met or the combination during the transition period.
There was a significant (p < 0.001) effect of supplementing RPAA on the peak of milk (Table 4, Figure 5), in which transition cows provided RPML–RPML diet (56.20 kg/day) produced more (p < 0.001) milk yield during the peak of milk in comparisons with CON–RPML (46.4 kg/day), RPM–RPML (50.5 kg/day), and RPL–RPML (52.9 kg/day); there was no difference (p > 0.10) between RPM–RPML and RPL–RPML.
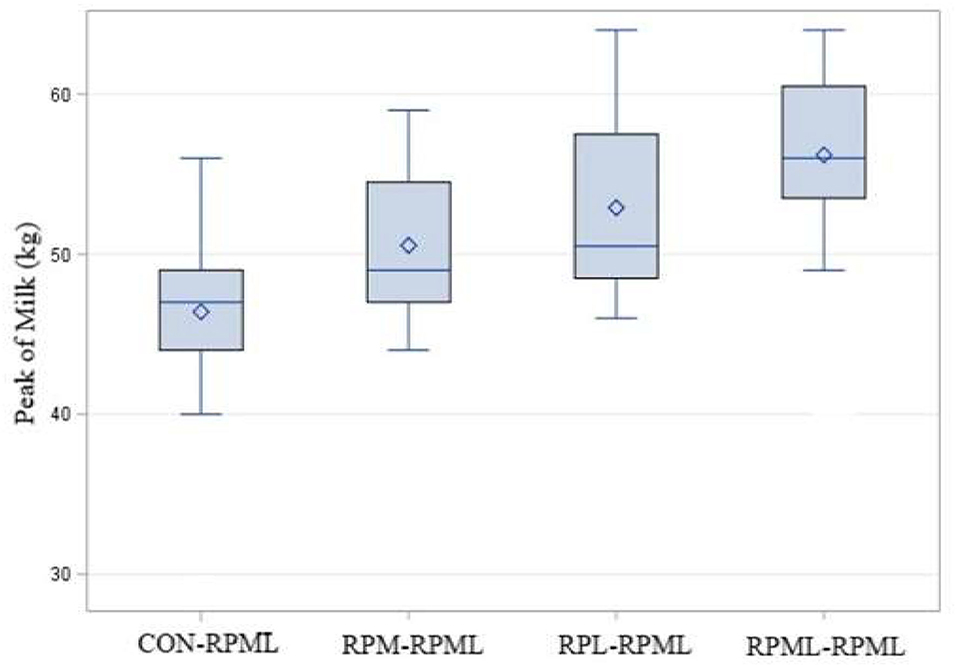
Figure 5. Effect of supply ruminally protected Met and Lys to transition and milking dairy cows on the peak of milk. Values are means; standard errors are represented by vertical bars.
Effect of Amino Acids Supply on Concentrations of β-Hydroxybutyrate and Serum Total Protein
There was an effect (p < 0.002) of RPAA supply on BHB concentration, as cows consumed RPML–RPML diet (0.72 mmol/L) had a lower (p < 0.05) BHB concentration than cows that fed either CON–RPML (0.90 mmol/L) or RPM–RPML (0.84 mmol/L), but no difference (p > 0.10) between RPL–RPML and RPML–RPML (Table 4). There was a time effect (p < 0.001) for BHB, as the concentration of BHB decreased (p < 0.001) with the progression of lactation. There were Trt × Time interactions for BHB concentration (p = 0.004; Table 4), as cows fed CON–RPML (1.20 mmol/L) had higher (p < 0.05) BHB than RPM–RPML (0.99 mmol/L), RPL–RPML (0.85 mmol/L), and RPML–RPML (0.76 mmol/L) at 30 DIM; and a difference (p = 0.002) between RPM–RPML (0.99 mmol/L) and RPML–RPML (0.76 mmol/L) at 30 DIM. Serum total protein concentration did not differ (p = 0.19) between the treatments; there were no interactions between Trt × Time for serum total protein concentration (p = 0.58). There was a time effect for serum total protein concentration (p = 0.004; Table 4), with serum total protein concentration higher (p = 0.003) at 150 DIM compared to the other time points.
Effects of Amino Acids Supply on Milk Production and Composition
As indicated in Table 5, Figure 6, transition cows fed RPAA supply are still producing more milk during the high milking period in comparison with transition cows that there were fed the control diet, with cows fed RPML–RPML diet (45 kg/day) producing more (p = 0.009) milk yield than CON–RPML (42.1 kg/day), RPM–RPML (42.8 kg/day), and RPL–RPML (42.6 kg/day). Time affected milk yield (p = 0.001), in which milk yield at 150 DIM, was the lowest (p < 0.05) compared with other time points at 30, 60, 90, and 120 DIM.
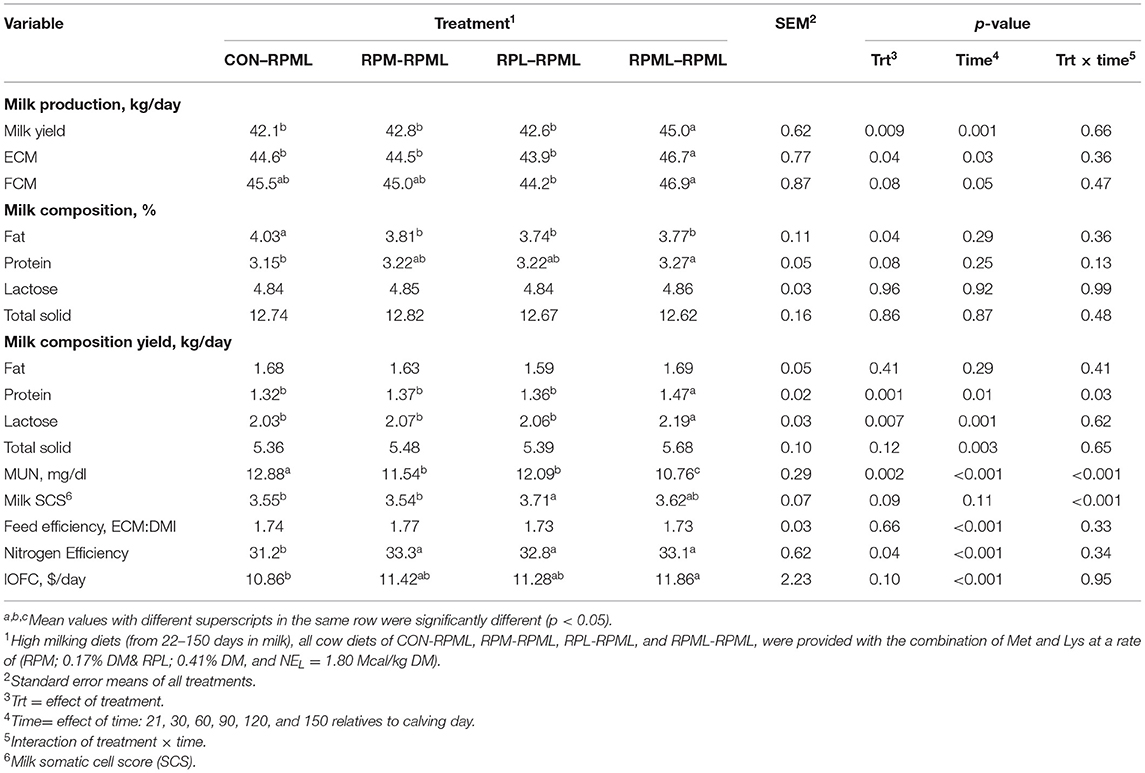
Table 5. Feeding ruminally protected Met and Lys to transition dairy cows and its subsequent effect on post-calving performance.
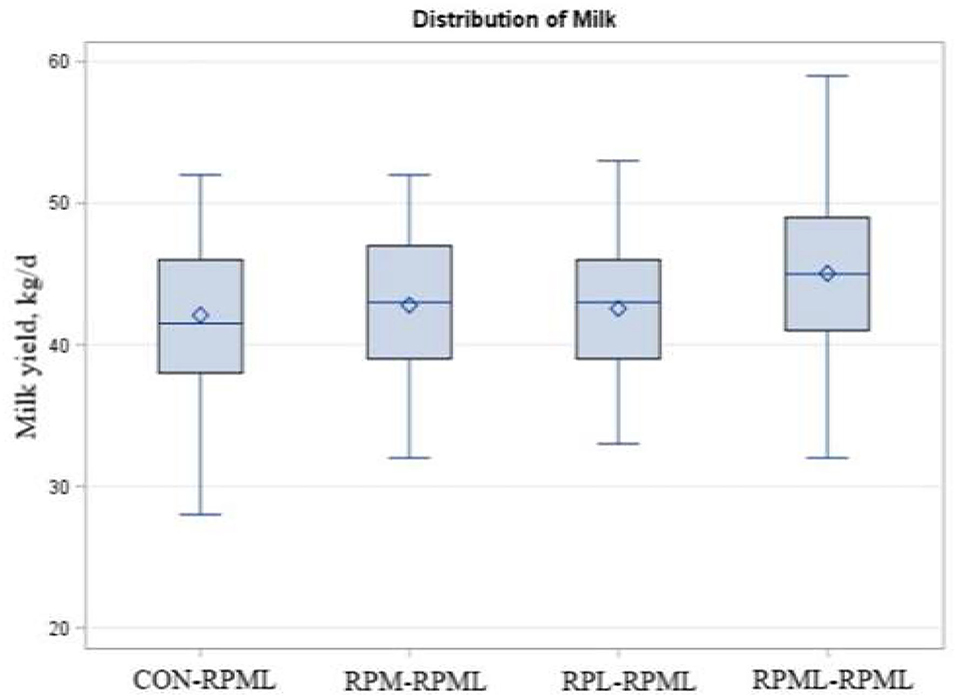
Figure 6. Effect of supply ruminally protected Met and Lys to transition and milking dairy cows on milk yield. Values are means; standard errors are represented by vertical bars.
As shown in Table 5, Figure 7, energy-corrected milk yield significantly increased (p = 0.04) for those cows that received RPML–RPML (46.7 kg/day) than CON–RPML (44.6 kg/day), RPM–RPML (44.5 kg/day), and RPL–RPML (43.9 kg/day). Time had an effect on ECM yield (p = 0.03), with ECM yield showed the lowest at 150 DIM compared with other time points at 30, 60, 90, and 120 DIM (p = 0.01). There were no Trt × Time interactions (p = 0.36) for ECM yield (Table 5). Fat-corrected milk tended (p = 0.08) to be affected by supplemental AA, with cows fed RPML–RPML diet (46.9 kg) produced more (p = 0.003) FCM yield than RPL–RPML (44.2 kg/day), and cows fed RPML–RPML diet (46.9 kg) tended (p = 0.10) to increase FCM than CON–RPML (45.5 kg) and RPM–RPML (45.0 kg). Time affected (p = 0.05) FCM yield, with FCM yield, showed the lowest (p = 0.01) at 150 DIM compared with other time points at 30, 60, 90, and 120 DIM.
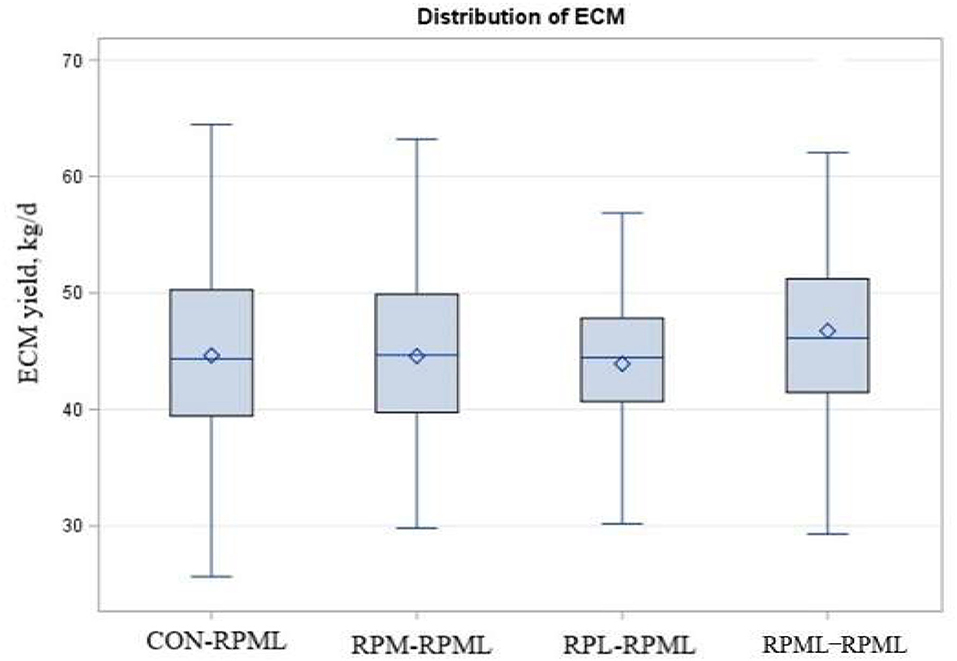
Figure 7. Effect of supply ruminally protected Met and Lys to transition and milking dairy cows on ECM yield during the subsequent lactation. Values are means; standard errors are represented by vertical bars.
As indicated in Table 5, milk fat content significantly (p = 0.04) differs between treatments, in which cows fed CON–RPML (4.03 %) had greater milk fat content than RPM–RPML (3.81 %), RPL–RPML (3.74 %), and RPML–RPML (3.77%). As shown also in Table 5, milk protein content responded (p = 0.08) to AA supply, as milk produced from cows fed RPML–RPML had the higher (p = 0.05) milk protein content (3.27%) than CON–RPML (3.15%), and tended (p = 0.09) to increase than RPM–RPML (3.22%) and RPL–RPML (3.22%).
As shown in Table 5, milk protein yields positively responded (p = 0.001) to the supply of AA, with cows that consumed RPML–RPML had a greater (p = 0.001) protein yield (1.47 kg) in comparison with CON–RPML (1.32 kg), RPM–RPML (1.37 kg), and RPL–RPML (1.36 kg). There was no difference (p > 0.10) in milk protein yield between CON–RPML, RPM–RPML, and RPM–RPML (Table 5). Time had an effect (p = 0.01) on milk protein yield, in which protein yield at 150 DIM was the lowest (p < 0.05) compared with other time points at 30, 60, and 90 DIM, and a strong tendency (p = 0.06) for decrease at 120 DIM. There were Trt × Time interactions for milk protein yield (p = 0.03; Table 5), with cows consumed RPML–RPML (1.57 kg) which had higher (p < 0.05) milk protein yield than CON–RPML (1.19 kg), RPM–RPML (1.36 kg), and RPL–RPML (1.41 kg) at 30 DIM and a higher (p = 0.008) milk protein yield in RPML–RPML (1.49 kg) than CON–RPML (1.29 kg) at 120 DIM; milk protein yield tended (p = 0.08) to increase in RPML–RPML (1.49 kg) compared to RPM–RPML (1.36 kg) at 120 DIM; and there was a tendency (p = 0.09) to increase milk protein yield for cows that fed RPL–RPML (1.37 kg) in comparison with CON–RPML (1.25 kg) at150 DIM.
Lactose yield was affected (p = 0.007) by RPAA supply, as cows provided with RPML–RPML (2.19 kg) supplementation had a greater (p = 0.007) lactose yield compared to CON–RPML (2.03 kg), RPM–RPML (2.07 kg), and RPL–RPML (2.06 kg; Table 5). The difference in lactose yield between CON–RPML, RPM–RPML, and RPM–RPML was insignificant (p > 0.10). Time affected lactose yield (p = 0.001), as the lowest (p < 0.05) lactose yield, produced at 150 DIM compared with other time points at 30, 60, and 90 DIM.
As shown in Table 5, MUN decreased (p = 0.002) with RPAA supply, as those cows that were fed diet supplied with RPML–RPML (10.76 mg/dl) had a lower (p < 0.05) MUN than cows in CON–RPML (12.88 mg/dl), RPM–RPML (11.54 mg/dl), and RPL–RPML (12.09 mg/dl). Milk urea nitrogen was affected by time (p < 0.001), as MUN increased linearly (p < 0.05) with the progression of lactation. There were Trt × Time interactions for MUN (p < 0.001; Table 5), as RPM–RPML (11.47 mg/dl) had higher (p < 0.05) MUN level than CON–RPML (9.54 mg/dl) RPL–RPML (9.43 mg/dl) and RPML–RPML (9.34 mg/dl) at 60 DIM; and milk from cows that consumed CON–RPML diet (15.84 mg/dl) contained a higher (p < 0.05) MUN than RPM–RPML (9.66 mg/dl), RPL–RPML (13.40 mg/dl), and RPML–RPML (9.43 mg/dl) at 90 DIM.
Somatic cell count tended (p = 0.09) to decrease with supplemental AA (Table 5), with cows that received RPL–RPML diet (3.71 log-transformed) had a higher (p < 0.05) SCC compared with CON–RPML (3.55 log-transformed) and RPM–RPML (3.54 log-transformed), and tended (p = 0.09) to increase compared with RPML–RPML (3.62 log-transformed). There were Trt × Time interactions for SCC (p < 0.001), in which cows that consumed RPML–RPML (4.05 log-transformed) had higher (p < 0.05) SCC at 150 DIM than cows that were fed RPM–RPML (3.7 log-transformed) and RPL–RPML (3.3 log-transformed).
Effect of Amino Acids Supply on Feed and Nitrogen Efficiency and Income Over Feed Cost
The effect of AA supply on feed and nitrogen efficiency is shown in Table 5. Feed efficiency was not affected (p = 0.66) by AA supplementation. There was a time effect for feed efficiency (p < 0.001), in which feed efficiency was higher (p < 0.001) at 30 DIM (1.91 ECM: DMI) than feed efficiency at 60 DIM (1.77 ECM: DMI), 90 DIM (1.72 ECM: DMI), 120 DIM (1.69 ECM: DMI), and 150 DIM (1.64 ECM: DMI). Nitrogen efficiency (N efficiency = milk protein N/N feed intake) significantly differed (p = 0.04, Figure 8), with a lower (p = 0.04) N efficiency for CON–RPML (31.2%) compared to RPM–RPML (33.3%), RPL–RPML (32.8%), and RPML–RPML (33.1%). Nitrogen efficiency was affected (p < 0.001) by time, because N efficiency linearly decreased with the progression of lactation from 30 to 150 DIM, with the highest (p < 0.05) N efficiency at 30 DIM and the lower one at 150 DIM.
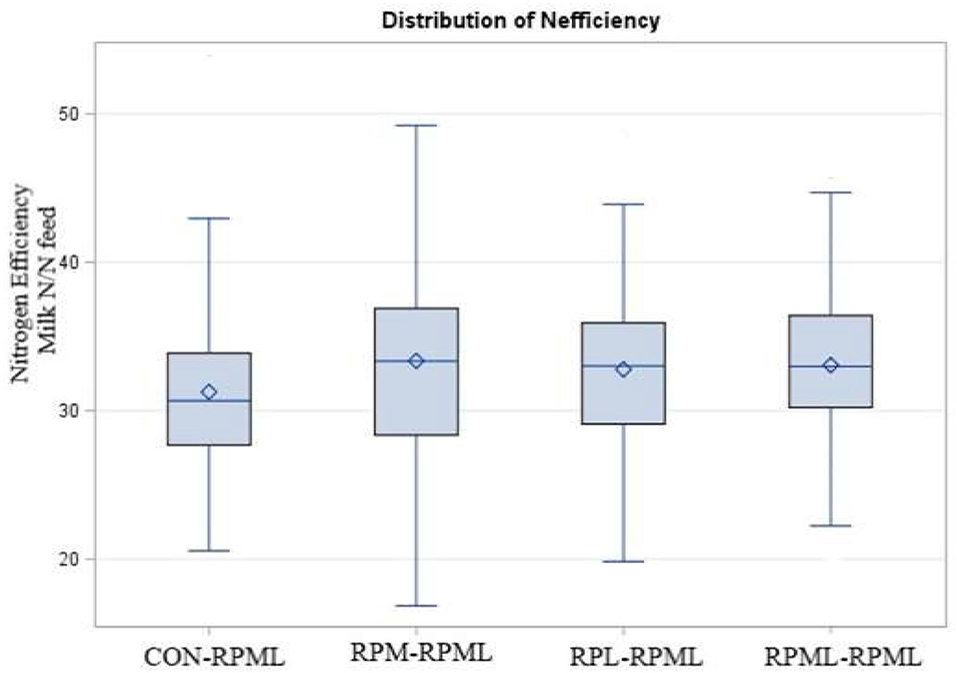
Figure 8. Effect of supply ruminally protected Met and Lys to transition and milking dairy cows on nitrogen efficiency (Milk protein N/N feed intake). Values are means; standard errors are represented by vertical bars.
Income over feed cost tended (p = 0.10) to increase with AA supply (Table 5, Figure 9); as cows in the group fed RPML–RPML (11.86 $/h/day) had a higher (p < 0.05) IOFC than CON–RPML (10.86 $/h/day), and IOFC tended (p = 0.08) to increase in RPML–RPML (11.86 $/h/day) compared to RPM–RPML (11.42 $/h/day) and RPL–RPML (11.28 $/h/day). Time affected IOFC (p < 0.001), in which the highest (p < 0.05) IOFC (12.45 $/h/day), showed at 30 DIM compared to IOFC at 60 DIM (12.02 $/h/day), 90 DIM (11.27 $/h/day), 120 DIM (11.11 $/h/day), and 150 DIM (9.9 $/h/day), meaning that the IOFC decreases linearly (p < 0.05) with the progression of lactation from 30 to 150 DIM, when the cows cross over the peak of milk.
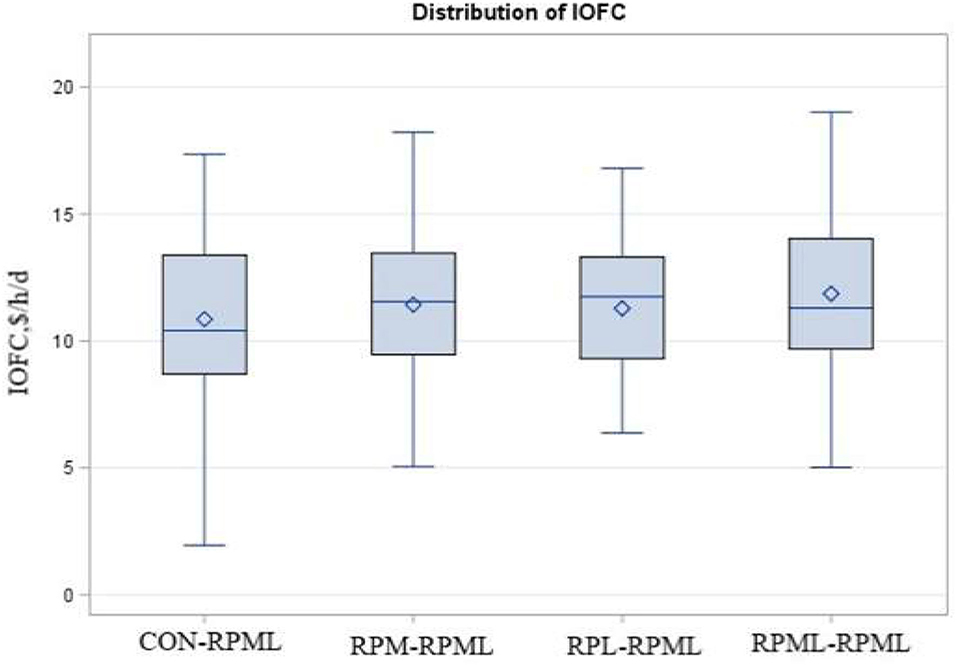
Figure 9. Effect of supply ruminally protected Met and Lys to transition and milking dairy cows on income over feed cost (IOFC). Values are means; standard errors are represented by vertical bars.
Effect of Amino Acids Supply on Fertility
The effect of feeding RPL and RPM to transition dairy cows on fertility is presented in Table 6. There was a significant Trt effect on DIM at 1st insemination (p < 0.004), as cows in CON–RPML treatment spent long days to get inseminated (73.85 days) than other cows 70.19, 86.65, and 65.33 days for RPM–RPML and RPL–RPML and RPML–RPML, respectively (Table 6, Figure 10). There was no difference on DIM at 1st insemination between RPM–RPML and RPL–RPML and RPML–RPML (p > 0.10). Heat detection rate was lower in cows fed CON–RPML diet (p < 0.002) compared with those cows that were fed other diets (65.96 vs. 69.62, 70.25, and 69.85%), for CON–RPML, RPM–RPML, RPL–RPML, and RPML–RPML, respectively (Table 6, Figure 11). Conception rate was higher (p < 0.05) for cows in RPML–RPML group than other cows (Table 6, Figure 12). There was no difference in conception rate between CON–RPML, RPM–RPML, and RPL–RPML (p > 0.10). The pregnancy rate was affected by RPAA supplementation, in which transition cows that received RPAA had a higher pregnancy rate than those cows that fed an unsupplemented diet (p < 0.004; Table 6, Figure 13). Cows that consumed RPML–RPML (23.74%) had a higher (p = 0.04) pregnancy rate than CON–RPML (20.22%), RPM–RPML (22.23%), or RPL–RPML (22.0%). Pregnancy rate was similar (p = 0.81) between RPM–RPML and RPL–RPML.
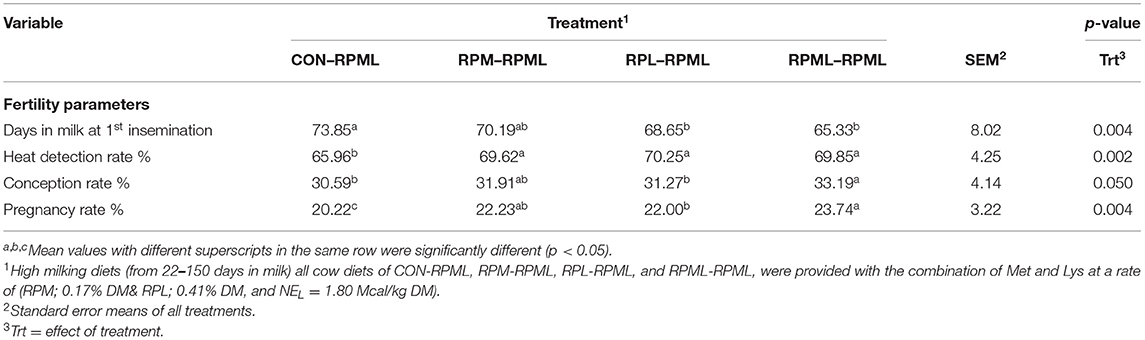
Table 6. Feeding ruminally protected Met and Lys to transition dairy cows and its subsequent effect on fertility.
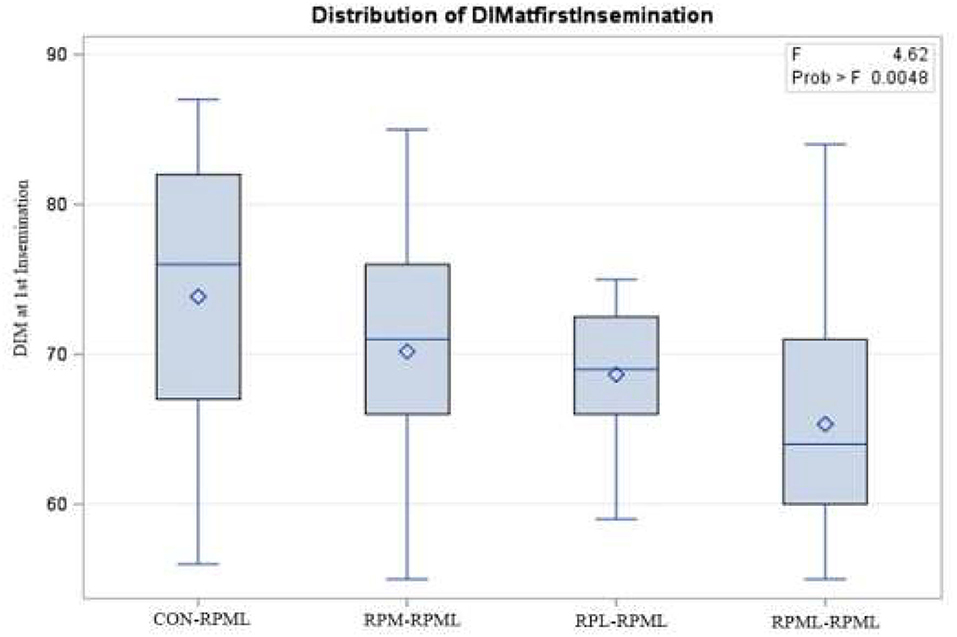
Figure 10. Effect of supply ruminally protected Met and Lys to transition and milking dairy cows on days to 1st insemination. Values are means; standard errors are represented by vertical bars.
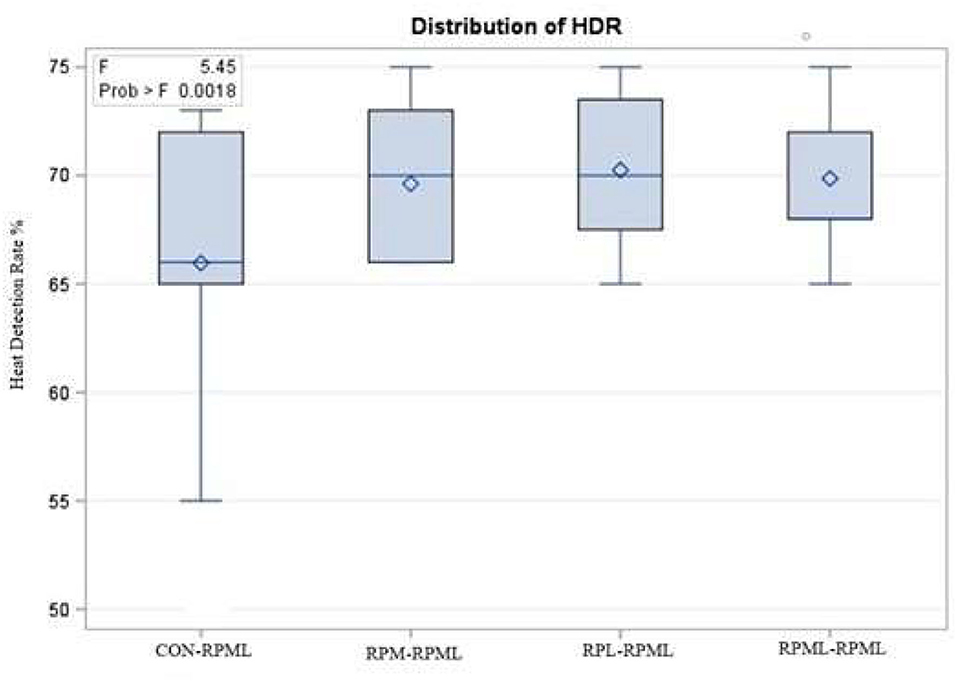
Figure 11. Effect of supply ruminally protected Met and Lys to transition and milking dairy cows on heat detection rate. Values are means; standard errors are represented by vertical bars.
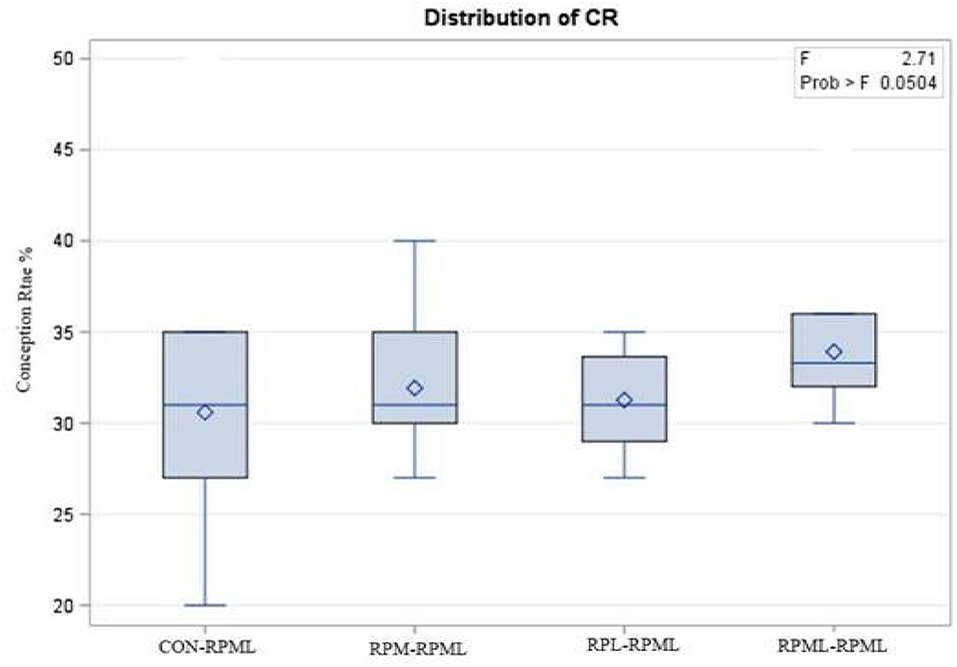
Figure 12. Effect of supply ruminally protected Met and Lys to transition and milking dairy cows on conception rate. Values are means; standard errors are represented by vertical bars.
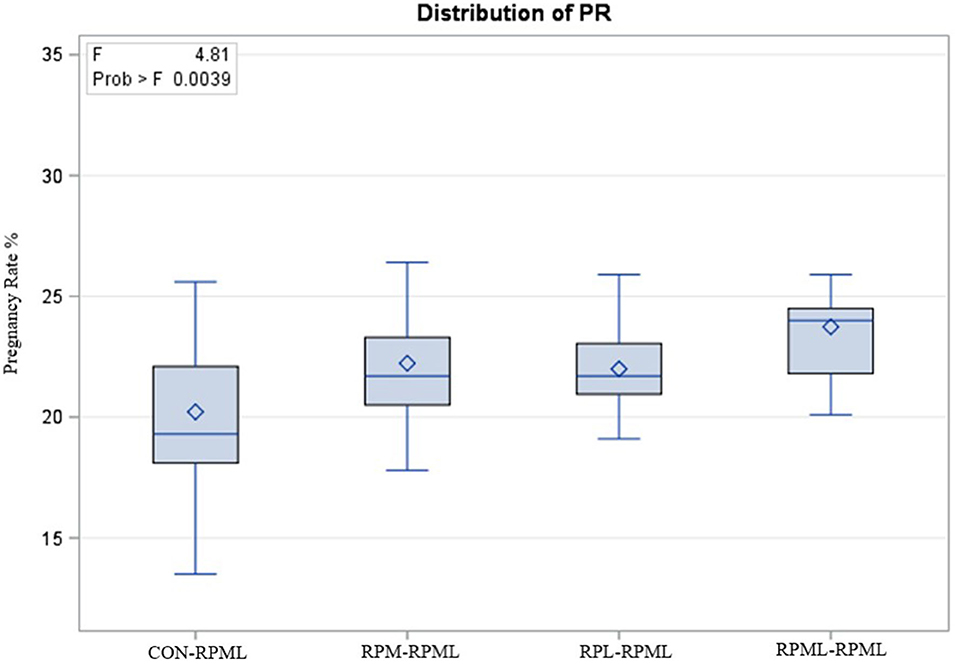
Figure 13. Effect of supply ruminally protected Met and Lys to transition and milking dairy cows on pregnancy rate. Values are means; standard errors are represented by vertical bars.
Discussion
The important results from this study were as follows: (1) an increase in the peak of milk production and the less time to reach to peak with a supply combination of RPL and RPM, (2) increased milk protein percentage and ECM yield due to dietary RPL and RPM supply, with no effects on feed efficiency, (3) improve N efficiency with given RPL and RPM, and (4) increased pregnancy rate by providing RPL and RPM continual from transition till 150 DIM, and consequently improve the fertility efficiency.
Effect of Amino Acids Supply on Dry Matter Intake
In the first stage of this study that focused on a continual supply of either RPL or RPM or two in combination during the transition period that lasted for 3 weeks before until 3 weeks after calving, we observed an increase in DMI in response to the supplies of RPL and RPM to transition dairy cows (18). The response of DMI to RPAA supply during the beyond period to the transition period is almost matched with that response that has previously been detected during the transition period (in which all cows received a combination of RPL and RPM). Stimulating DMI resulted in greater energy and total MP intake, which supported greater production and spared BW loss in this study, mainly to increase indispensable amino acids (IAA) efficiency.
Increased DMI was generally reported in response to the supply of RPL and RPM separately or in combination to dry and lactating cows (16, 42–45), consistent with the current findings as well as with our results during the transition period using the same cows that have continued in this study, but the response not universally (12, 27, 46–50). So far, the mechanism of action that governs the response of feed intake to amino acids supply in ruminants has not yet been completed. Nevertheless, the mechanism linking AA and DMI has been shown in rodents to affect food intake. There is considerable evidence for neural control of food intake in response to imbalanced AA diets in rats (51, 52), and this can also apply to ruminants in the same mechanism. Also, there is evidence that other factors are operating for cows deficient in multiple AA (53). However, the mechanisms underlying the control of the feeding response to the dietary model remain not fully understood (52), and more research is needed. This is the first study that evaluated the effects of feeding a combination of RPL and RPM on milk production coupled with the reproductive efficiency of lactating Holstein cows.
Metabolizable Protein Balance and CNCPS Evaluation of Diets
In this study, the high MP balance observed for cows fed CON–RPML diet may be explained by the increase in MP intake (driven by the increase of DMI also, and at the same time dietary balance for AA profile assist in improving DMI) at a greater rate than AA secreted in the milk, thus making a higher MP balance compared to the other cows. However, a 524 g/day MP deficiency was detected for cows consumed the CON–RPML diet during the early lactation period (18), and this decrease is accompanied by a decrease in milk production and its components and a decrease in BCS also during the first 3 weeks of lactation compared to other treatments (18). The improvement in MP balance in CON-RPML beyond of transition period (when a combination of RPL and RPM was fed) resulted in an improved AA profile and increased milk production during the high milking period. Perhaps, the improvement in MP balance explains how the milk production increased in CON-RPML and became almost similar to RPM–RPML and RPL–RPML treatments during the high milking period.
Effects of Amino Acids Supply on Body Condition Score
Body condition score improved in this study due to the high DMI supported by supplementing RPAA, which minimized BCS changes at 150 DIM compared with BCS at 21 DIM. At the same time, the cows with normal BCS during the post-calving period are still maintained at the level of the BCS. In this study, we detected that the increase in BCS during the high lactation period is mostly proportional to the BCS during the early lactation period; this means that cows with normal BCS during the early lactation period are better now (RPML–RPML). Additionally, the cows with the lowest BCS during the early post-calving period are the lowest BCS during the high milking period as well (CON–RPML). Dissimilarly, other studies indicated that providing RPM or RPL did not affect the BCS of dairy cows (12, 16, 43, 44, 54, 55); in most of these studies, but not all, DMI did not increase, or less increase, and perhaps, this explains the lack of response of BCS to RPAA supply, unlike the results of the current experiment. Body condition score is a dynamic indicator to determine the mobilization of fatty tissue from the body stores; therefore, BCS is a very important measure from a practical point of view as an indication for evaluating the level of nutrition and farm management efficiency in commercial dairy farms.
Effect of Amino Acids Supply on Days to Peak and Peak of Milk
In this study, the higher peak of milk yield and shorter days-to-peak of milk could be explained by the success of the transition period as a result of the addition of RPAA, and a reasonable increase in DMI during both transition and early lactation period, as it is used as an indicator of nutritional management of transition cows. This is also connected with a reduction in the concentration of BHB in the blood in cows fed RPAA, which leads to improved animal health. All these factors combined lead to high milk production during the peak period and reaching the peak in a short period after calving for cows consumed RPAA during the transition period. The transition phase represents the time of greatest risk to the dairy cow. Metabolic disorders and physiological changes, combined with other factors, are the events that limit the cow in its ability to achieve higher peaks. The health events have both major nutritional influences and environmental and social causes and most often are intertwined. Most nutritional efforts have been focused on pre-and post-calving nutrition periods. Over the years, these improved feeding practices have been responsible for much of the increase in milk production in dairy farming.
The nutrition side of transition is much more complicated, with interactions between protein, minerals, vitamins, and energy status opening the door to metabolic challenges. This, combined with the effects of weather and farm management level, provides the most opportunity for things to go wrong at any time in the calendar of the cows. In this study, supplementing a combination of RPL and RPM may help to improve AA profile, which stimulates DMI, leading to greater EB and metabolizable protein intake, sparing BCS, consequently assisting minimize the occurrence of ketosis and helping the cow metabolize fat more efficiently during the transition and beyond period. In addition to the good farm management, all the above factors can allow cows to achieve better performance in early lactation, leading to a higher peak of milk and reaching the peak of milk in the shortest possible time in this study.
Effect of Amino Acids Supply on β-Hydroxybutyrate Concentration
In this study, as we reported earlier during the transition period, Elsaadawy et al. (18) we showed that the lower BHB in response to the supply of a combination of RPL and RPM can be explained mainly by the greater consumption of DMI that is driven by balancing AA profile (18), in complement to other auxiliary factors. Excessive lipid mobilization results in incomplete hepatic oxidation and release of ketone bodies (56, 57), such as acetoacetate, BHB, and acetone. While, during the high milking period (from 22 to 150 DIM), all cows were fed a mixture of RPL and RPM and all become in positive energy and metabolizable protein balance, meaning that there is no mobilization for adipose tissue, this explains the normal level of BHB for all cows after 30 DIM (<1.2 mmol/L), in addition to increase DMI. During negative energy balance (around calving and up to a few weeks after calving), there is a large uptake of adipose tissue-derived long-chain fatty acid (FA) by the liver, which would have resulted in incomplete oxidation of non-esterified fatty acid (NEFA) and, consequently, elevated ketone bodies (56), which minimized with RPAA supply in this study.
Supplemental RPAA to dairy cows had varied effects on β-hydroxybutyrate concentration. Decreased BHB concentration had been reported when RPL and RPM were fed to dairy cows (27, 43, 58), but not in all cases (13). The difference in BHB concentration in response to RPAA supply is explained by many reasons, including the concentration of RPAA, length of the experimental period, type of animal (multiparous or primiparous), time of supplementation (e.g., close-up or post-calving, or both, or high milking, or mid-lactation cows), the composition of AA (e.g., RPAA, or analog, or AA derivatives), the methods of addition (e.g., mixed with TMR, or top-dressed), and the environmental condition as well as the farm management level. One or both of these factors may affect the animal's response and the concentration of BHB to AA supplementation.
The liver's free fatty acid oxidation rate appears to regulate feed intake in ruminants. Allen and Piantoni (59) reported that hepatic oxidation of NEFA stimulated brain feeding centers via hepatic vagal nerve to suppress intake. Based on this observation, it is probable that liver concentrations of FA or FA-derived metabolites such as BHB are being sensed rather than oxidation. In either case, exporting more FA as very low-density lipoprotein (VLDL) would lead to less oxidative stress in the liver and avoid feed intake suppression, this partially explains the reason for the low BHB concentrations in this experiment. Another reason to explain the lower BHB with AA supply suggests that RPM and RPL might have improved hepatic lipid metabolism and increased carnitine bioavailability. Osorio et al. (16) showed a tendency to decrease the occurrence of clinical ketosis when RPM was fed to transition cows, suggesting that supplied RPM might have improved hepatic lipid metabolism. Lipotropic agents such as Met or choline assist in lipid export from the liver by stimulating VLDL formation (56). Consequently, that response may lead to a decrease in hepatic triacylglycerol (TAG) accumulation and formation of ketone bodies (56, 60), which may also assist in the decreasing of BHB during the transition period and the later period by RPAA supply.
Effects of Amino Acids Supply on Milk Production and Composition
In this study, matched with our first hypothesis, continual feeding of a combination of RPL and RPM throughout the transition and beyond (during the high milking period from 22 to 150 DIM) allows the cows to produce more milk protein yield and higher content, besides the increased milk production. Some EAAs are considered limiting for milk protein synthesis under specific dietary conditions in dairy cows, typically Met and Lys (17), and probably His. The increase in milk protein percentage and yield observed in this study in response to RPL and RPM agreed with what was previously obtained (9, 14, 16, 61, 62), but not always (27, 63, 64). Consistent with other studies, there was a positive response of dietary RPL and RPM supply on milk yield for transition and lactating and beef cows (13, 14, 16, 61, 65, 66), but this response is not universal (12, 27, 67–69).
In this study, milk lactose yield and protein increased with RPAA supply resulting in the use of a greater proportion of the unsupplemented IAA for milk protein. Junior et al. (61) demonstrated that improving AA absorption efficiency increases milk production in high-lactating dairy cows. Metabolizable protein supply has been recognized as the limiting factor for the absorption of AA in the mammary gland, which will be decided the yields of protein, lactose, and subsequently milk production (70, 71), as milk yield is a derivative from these components. Increasing the supply of MP would increase protein yield, milk production, and the amount required for lactose synthesis (13, 70), which was also observed in this study. Milk protein responses to Met are well documented, and responses to Lys and His are somewhat more changeable but have often been observed (72, 73). Besides Met, Lys, and His, it shows that the other two IAAs of leucine (Leu) and isoleucine (Ile) are also involved in driving milk protein production (74, 75).
The significant milk production response to RPAA in this study may be due to the increased efficiency of absorbed AA use (in addition to providing sufficient MP) that stimulates DMI and drive milk production. However, there are many reasons for the inconsistent in milk yield and constituents in response to RPAA supply; it is explained by the variability of protection methods for RPAA, which would make a difference in bioavailability, which may have accounted for the differences in results between studies; there were differences in the basal diets feedstuff, milk production level, and the metabolizable amount of Met and Lys g/MP. Additionally, the study length and animal type (multiparous, primiparous, pregnant, etc.), lactation phase, farm management level, and cow's well-being may also affect animal response to RPAA supply.
A deficiency in energy, excess dietary protein, and an imbalance between energy and protein could increase MUN (76, 77); this did not happen in this study. The lower MUN shown in this study indicates greater efficiency of N utilization. Lowered MUN has previously been observed by supplying RPAA form, including Met, Lys, leucine, threonine, and isoleucine separately or two in combination to lactating dairy cows (28, 29, 78), with no response in others (13, 27, 43, 61, 68). In this study, continual supplementing RPAA to transition and lactating dairy cows may improve udder health as indicated by the lowered milk SCC, as reported earlier in this study during the perinatal period (18, 29). Supply RPM plays a vital role in enhancing the immune status in dairy cows through enhanced phagocytosis and oxidative functions, which has been revealed when RPM was fed to dairy cows pre-calving and post-calving continuously (66), and it may apply for Lys as well. The current result of lowered SCC was consistent with other studies that fed RPM and RPL to pre-calving dairy cows (27), but this effect is not common (9, 13, 61, 78). Many reasons can cause an increase in milk SCC: one of these inflammation in the mammary gland tissues (79), cow productivity, health status, lactation, lactation phase, and animal breed (80).
Effect of Amino Acids Supply on Nitrogen Efficiency and Income Over Feed Cost
The improvement in N efficiency in this study can be clarified by the increase in milk production, driven by the increase in DMI and dietary AA balance. Nitrogen efficiency is relatively low in dairy cows, with an average of 25%−35% of N feed intake secreted into N milk protein (81) and nearly all the residual N excreted into feces and urine with an average rate of 72% (82). A previous study showed that feeding of Met or Lys or both could increase N efficiency (83), similar to the results from this study. However, in the period of the peak of milk (38 ± 15 DIM), RPL and RPM supply (5 g/day Met and 10 g/day Lys, intestinally absorbed) observed a decrease in N efficiency in Holstein dairy cows (84); this may be due to the small amount of absorbed RPL and RPM that were consumed or the lack of control of AA balance in that study. Other authors reported no improvement in N efficiency when they evaluated Met supplementation in a digestibility experiment (85).
Income over feed cost was increased for cows that consumed RPML-RPML in this study due to the greater ECM production. The authors mentioned IOFC here to light the importance of RPAA supply from an economic point of view. For example, the IOFC from the cows fed RPML-RPML would be $1.0 more than that from CON-RPML cows. It could improve many aspects of production efficiency besides the economic profit. So, the IOFC is a gross margin concept.
Effect of Amino Acids Supply on Fertility and Reproductive Parameters
The mechanisms that underlie the increased pregnancy rate by feeding RPL and RPM in multiparous Holstein cows remain unclear. The effects of Met could be at different stages of embryonic development and are linked to multiple biochemical pathways caused by insufficient Met, such as an overall depression in embryonic protein production, decreased the activity of one-carbon (1-C) metabolism, probably leading to a reduction of DNA methylation, or reduction in embryonic polyamines. Toledo et al. (9) indicated that multiparous cows might be more prone to Met deficiency than primiparous cows due to increased milk protein yield and milk production and thus could lead to an increased need for Met; this is one of the main reasons for using multiparous cows in the present experiment. Toledo et al. (9) also reported that the Met circulating rate was lower in multiparous cows compared to primiparous cows (9). Thus, insufficient circulating Met concentrations in multiparous cows may limit intrauterine Met concentrations, leading to a delay in embryonic development. A recent study has shown that multiparous cows have a larger uterine size and lower fertility than primiparous cows (86). There is a large increase in concentrations of AA in the uterine histotroph during early pregnancy in sheep (8) and cattle (7), including a large increase in Met, Lys, and other EAA as well, and this may be important for optimal embryonic development. Toledo et al. (9) showed that cows with a larger uterine volume may have reduced AA concentrations in the uterine histotroph and probably larger AA requirements during the elongation and early placentation period of pregnancy. Besides, an in vivo study reported that providing RPM to lactating dairy cattle rations created dramatic modulation in gene expression in embryos, in general decreasing the concentrations of mRNA in early embryos (87).
Feeding a combination of RPL and RPM in this study may induce changes in the early embryo that are consequently visible in the later phases of pregnancy. Souza et al. (88) demonstrated that Met consumption did not impact fertilization and gross morphological quality of day 7 embryos, but the diet supplied with Met altered embryonic gene expression, particularly leading to reducing the expression of many specific genes correlated to fetus development (e.g., VIM, BCL2A1, IFI6, and TBX15) and immune response (e.g., LCP1, NKG7, TYROBP, SLAMF7, and BLA-DQB). Recently, it has been proven that arginine has an important role on pregnancy in the early stages. Sun et al. (89) reported that maternal rumen-protected arginine supply to pregnant ewes fed a restricted diet (50% of the requirement between days 35 and 110 of pregnancy) improving the endocrine metabolic homeostasis in the fetus and increasing the availability of AA in the embryonic liver and longissimus dorsi muscle as well as its effect on the expression of somatotropic axis genes, and it is possible that this mechanism applies to Lys and thus helps us in understanding the mechanism of Lys in dairy cows. An in vitro study (22) indicated a surprisingly low Met requirement (7–21 μM) for developing morphologically normal bovine embryos. However, treatment of in vitro-produced bovine embryos with ethionine (a Met anti-metabolite) reduced embryo development at the blastocyst stage, and the addition of S-adenosylmethionine (SAM) could partially restore embryonic development in the presence of methionine (90).
There may be a need for supplementing an appropriate amount of Met to the cows' diets to ensure a proper embryo development because of the effects on DNA methylation, fetal gene expression, general protein synthesis pathways, and specific metabolic pathways, such as polyamines, which may be pivotal for optimal progression of the pregnancy and Lys as well. Lately, an in vivo study demonstrated that cows received RPM-generated early embryos with lower DNA methylation but higher lipid content proposing that Met supply may affect energy metabolism and, consequently, embryo survival (23). Moreover, in other species, Met metabolism participates in synthesizing polyamines (91, 92). The reduction of polyamines is generally correlated with the weakness of early embryo development, attachment, growth of extra-embryonic structures and placenta, and steroidogenesis (25, 93); these highlight other possible pathways that may make pregnancy loss in animals with deficient Met, and this may be one of the reasons to explain the improvement of pregnancy in this study as a result of feeding the Met in addition to Lys as well.
Fertility efficiency has been improved in response to dietary supply of RPAA, either RPL or RPM or two in combination with dairy cows (9, 19), or small or even no effects (20, 21). Toledo et al. (9) indicated that RPM supply (21.2 g/h/day; to be within 2.34% of MP) from 30 until 126 DIM to multiparous lactating cows decreased pregnancy loss between days 32 to 61 of pregnancy. Ardalan et al. (19) reported that dairy cows that consumed RPM (18 g/day) from 4 weeks pre-calving through 20 weeks post-calving improved conception rate and consequently decreased open days, in line with the results obtained from this current experiment. Additionally, studies in sheep (24, 25) and dairy cattle (7, 26) have reported that Met was concentrated in uterine and embryonic fluids, emphasizing the role of increased uterine Met in normal embryonic development and survival, and this partly explains the improvement of fertility in this study in dairy cows as a result of supplementing RPAA during the transition period and later period. Feeding dairy cows a low protein diet supplied with Met (14% CP, 40 g/day Met) from parturition to 120 DIM had a less effect on reproductivity, as 1st conception rate was 28.5 and days open was 134 days (94), which was lower values than what was obtained in this study by providing RPL and RPM. In contrast, Polan et al. (21) reported that supply RPM and RPL had no effects on DIM at 1st insemination, service per conception, and calving interval in Holstein dairy cows.
Conclusions
In this study, continual consumption of a combination of RPL and RPM throughout the transition and high milking period increased DMI, ECM, and milk composition production, with no effects on feed efficiency. There was an improvement in N utilization as well as enhanced fertility efficiency in response to AA supply.
Data Availability Statement
The raw data supporting the conclusions of this article will be made available by the authors, without undue reservation.
Ethics Statement
All procedures were approved by the Animal Care and Use Committee of the Institute of Animal Science, Chinese Academy of Agricultural Sciences, Beijing, China (no. IAS20180115).
Author Contributions
SE: conceptualization, conducted the experiment, laboratory analysis, writing—original draft, and formal analysis. ZW: investigation. DB: supervision, project administration, and funding acquisition.
Funding
This research was partially supported by the Key Research and Development Program of the Ningxia Hui Autonomous Region (2021BEF02018), the International Atomic Energy Agency Technical Co-operation and Assistance Programme (no. CPR5025), the Agriculture Science and Technology Innovation Program (ASTIP-IAS07-1), Chinese Academy of Agricultural Science and Technology Innovation Project (CAAS-XTCX2016011-01), and Beijing Dairy Industry Innovation Team (BAIC06-2022).
Conflict of Interest
The authors declare that the research was conducted in the absence of any commercial or financial relationships that could be construed as a potential conflict of interest.
Publisher's Note
All claims expressed in this article are solely those of the authors and do not necessarily represent those of their affiliated organizations, or those of the publisher, the editors and the reviewers. Any product that may be evaluated in this article, or claim that may be made by its manufacturer, is not guaranteed or endorsed by the publisher.
Acknowledgments
SE was supported by the Graduate School of the Chinese Academy of Agricultural Sciences (GSCAAS) scholarship to perform his Ph.D. studies at the Chinese Academy of Agricultural Sciences (CAAS), Beijing, China. The authors gratefully acknowledge the AustAsia Dairy Group, Shandong, China, for their assistance in conducting this study.
References
1. Chapinal N, LeBlanc SJ, Carson ME, Leslie KE, Godden S, Capel M, et al. Herd-level association of serum metabolites in the transition period with disease, milk production, and early lactation reproductive performance. J Dairy Sci. (2012) 95:5676–82. doi: 10.3168/jds.2011-5132
2. Carvalho PD, Souza AH, Amundson MC, Hackbart KS, Fuenzalida MJ, Herlihy MM, et al. Relationships between fertility and postpartum changes in body condition and body weight in lactating dairy cows. J Dairy Sci. (2014) 97:3666–83. doi: 10.3168/jds.2013-7809
3. Hansen PJ, Denicol AC, Dobbs KB. Maternal embryokines that regulate development of the bovine preimplantation embryo. Turk J Vet Anim Sci. (2014) 38:589–98. doi: 10.3906/vet-1405-96
4. Bazer FW, Wu G, Johnson GA, Kim J, Song G. Uterine histotroph and conceptus development: select nutrients and secreted phosphoprotein 1 affect mechanistic target of rapamycin cell signaling in Ewes1. Biol Reprod. (2011) 85:1094–107. doi: 10.1095/biolreprod.111.094722
5. Roche JR, Burke CR, Meier S, Walker CG. Nutrition × reproduction interaction in pasture-based systems: is nutrition a factor in reproductive failure? Anim Prod Sci. (2011) 51:1045. doi: 10.1071/AN10162
6. Lucy MC, Butler ST, Garverick HA. Endocrine and metabolic mechanisms linking postpartum glucose with early embryonic and foetal development in dairy cows. Animal. (2014) 8:82–90. doi: 10.1017/S1751731114000482
7. Groebner AE, Rubio-Aliaga I, Schulke K, Reichenbach HD, Daniel H, Wolf E, et al. Increase of essential amino acids in the bovine uterine lumen during preimplantation development. Reproduction. (2011) 141:685–95. doi: 10.1530/REP-10-0533
8. Gao H, Wu G, Spencer TE, Johnson GA Li X, Bazer FW. Select nutrients in the ovine uterine lumen. I amino acids, glucose, and ions in uterine lumenal flushings of cyclic and pregnant Ewes1. Biol Reprod. (2009) 80:86–93. doi: 10.1095/biolreprod.108.071597
9. Toledo MZ, Baez GM, Garcia-Guerra A, Lobos NE, Guenther JN, Trevisol E, et al. Effect of feeding rumen-protected methionine on productive and reproductive performance of dairy cows. PLoS ONE. (2017) 12:e0189117. doi: 10.1371/journal.pone.0189117
10. Brosnan JT, Brosnan ME, Bertolo RFP, Brunton JA. Methionine: a metabolically unique amino acid. Livest Sci. (2007) 112:2–7. doi: 10.1016/j.livsci.2007.07.005
11. Sigler PB. An analysis of the structure of tRNA. Annu Rev Biophys Bioeng. (1975) 4:477–527. doi: 10.1146/annurev.bb.04.060175.002401
12. Socha MT, Putnam DE, Garthwaite BD, Whitehouse NL, Kierstead NA, Schwab CG, et al. Improving intestinal amino acid supply of pre- and postpartum dairy cows with rumen-protected methionine and lysine. J Dairy Sci. (2005) 88:1113–26. doi: 10.3168/jds.S0022-0302(05)72778-8
13. Fehlberg LK, Guadagnin AR, Thomas BL, Sugimoto Y, Shinzato I, Cardoso FC. Feeding rumen-protected lysine prepartum increases energy-corrected milk and milk component yields in Holstein cows during early lactation. J Dairy Sci. (2020) 103:11386–400. doi: 10.3168/jds.2020-18542
14. Silva GM, Chalk CD, Ranches J, Schulmeister TM, Henry DD, DiLorenzo N, et al. Effect of rumen-protected methionine supplementation to beef cows during the periconception period on performance of cows, calves, and subsequent offspring. Animal. (2021) 15:100055. doi: 10.1016/j.animal.2020.100055
15. Toledo MZ, Stangaferro ML, Gennari RS, Barletta R V, Perez MM, Wijma R, et al. Effects of feeding rumen-protected methionine pre- and postpartum in multiparous Holstein cows: lactation performance and plasma amino acid concentrations. J Dairy Sci. (2021) 104:7583–603. doi: 10.3168/jds.2020-19021
16. Osorio JS Ji P, Drackley JK, Luchini D, Loor JJ. Supplemental Smartamine M or MetaSmart during the transition period benefits postpartal cow performance and blood neutrophil function. J Dairy Sci. (2013) 96:6248–63. doi: 10.3168/jds.2012-5790
17. NRC. Nutrient Requirements of Dairy Cattle. Nutrient Requirements of Dairy Cattle, 7th Revised ed. Washington, DC: National Research Council (2001).
18. Elsaadawy SA, Wu Z, Wang H, Hanigan MD, Bu D. Supplementing ruminally protected lysine, methionine, or combination improved milk production in transition dairy cows. Front Vet Sci. (2022) 9:780637. doi: 10.3389/fvets.2022.780637
19. Ardalan M, Rezayazdi K, Dehghan-Banadaky M. Effect of rumen-protected choline and methionine on physiological and metabolic disorders and reproductive indices of dairy cows. J Anim Physiol Anim Nutr. (2010) 94:e259–65. doi: 10.1111/j.1439-0396.2009.00966.x
20. Rogers JA, Peirce-Sandner SB, Papas AM, Polan CE, Sniffen CJ, Muscato T V, et al. Production responses of dairy cows fed various amounts of rumen-protected methionine and lysine. J Dairy Sci. (1989) 72:1800–17. doi: 10.3168/jds.S0022-0302(89)79297-3
21. Polan CE, Cummins KA, Sniffen CJ, Muscato T V, Vicini JL, Crooker BA, et al. Responses of dairy cows to supplemental rumen-protected forms of methionine and lysine. J Dairy Sci. (1991) 74:2997–3013. doi: 10.3168/jds.S0022-0302(91)78486-5
22. Bonilla L, Luchini D, Devillard E, Hansen PJ. Methionine requirements for the preimplantation bovine embryo. J Reprod Dev. (2010) 56:527–32. doi: 10.1262/jrd.10-037H
23. Acosta DAV, Denicol AC, Tribulo P, Rivelli MI, Skenandore C, Zhou Z, et al. Effects of rumen-protected methionine and choline supplementation on the preimplantation embryo in Holstein cows. Theriogenology. (2016) 85:1669–79. doi: 10.1016/j.theriogenology.2016.01.024
24. Gao H, Wu G, Spencer TE, Johnson GA, Bazer FW. Select nutrients in the ovine uterine lumen. IV Expression of neutral and acidic amino acid transporters in ovine uteri and peri-implantation conceptuses. Biol Reprod. (2009) 80:1196–208. doi: 10.1095/biolreprod.108.075440
25. Gao H, Wu G, Spencer TE, Johnson GA, Bazer FW. Select nutrients in the ovine uterine lumen. V Nitric oxide synthase, GTP cyclohydrolase, and ornithine decarboxylase in ovine uteri and peri-implantation conceptuses. Biol Reprod. (2009) 81:67–76. doi: 10.1095/biolreprod.108.075473
26. Hugentobler SA, Humpherson PG, Leese HJ, Sreenan JM, Morris DG. Energy substrates in bovine oviduct and uterine fluid and blood plasma during the oestrous cycle. Mol Reprod Dev. (2008) 75:496–503. doi: 10.1002/mrd.20760
27. Lee C, Lobos NE, Weiss WP. Effects of supplementing rumen-protected lysine and methionine during prepartum and postpartum periods on performance of dairy cows. J Dairy Sci. (2019) 102:11026–39. doi: 10.3168/jds.2019-17125
28. Zhao K, Liu W, Lin XY, Hu ZY, Yan ZG, Wang Y, et al. Effects of rumen-protected methionine and other essential amino acid supplementation on milk and milk component yields in lactating Holstein cows. J Dairy Sci. (2019) 102:7936–47. doi: 10.3168/jds.2018-15703
29. Elsaadawy S, Wang H, Wu Z, Bu D. Supplementing ruminally protected methionine or lysine improved milk production in transition cows. J Dairy Sci Abstr Annu Meet Am Dairy Sci Assoc. (2021);104(Suppl. 1):97. Available online at: https://www.adsa.org/Portals/0/SiteContent/Docs/Meetings/2021ADSA/ADSA2021_Abstracts.pdf
30. Wang H, Elsaadawy SA, Wu Z, Bu DP. Maternal supply of ruminally-protected lysine and methionine during close-up period enhances immunity and growth rate of neonatal calves. Front Vet Sci. (2021) 8:780731. doi: 10.3389/fvets.2021.780731
31. Faul F, Erdfelder E, Buchner A, Lang AG. Statistical power analyses using G*Power 3. 1: tests for correlation and regression analyses. Behav Res Methods. (2009) 41:1149–60. doi: 10.3758/BRM.41.4.1149
32. NRC. Nutrient Requirements of Domestic Animals. Nutrient Requirements of Domestic Animals. Washington, DC: National Academy of Science, National Research Council (1956).
33. Van Amburgh ME, Collao-Saenz EA, Higgs RJ, Ross DA, Recktenwald EB, Raffrenato E, et al. The cornell net carbohydrate and protein system: updates to the model and evaluation of version 6.5. J Dairy Sci. (2015) 98:6361–80. doi: 10.3168/jds.2015-9378
34. Graulet B, Richard C, Robert JC. Methionine availability in plasma of dairy cows supplemented with methionine hydroxy analog isopropyl ester. J Dairy Sci. (2005) 88:3640–9. doi: 10.3168/jds.S0022-0302(05)73049-6
35. AOAC International. Official Methods of Analysis. 17th ed. AOAC International, Gaithersburg, MD. J AOAC Int. (2000).
36. Van Soest PJ, Robertson JB, Lewis BA. Methods for dietary fiber, neutral detergent fiber, and nonstarch polysaccharides in relation to animal nutrition. J Dairy Sci. (1991) 74:3583–97. doi: 10.3168/jds.S0022-0302(91)78551-2
37. Tyrrell HF, Reid JT. Prediction of the energy value of cow's milk. J Dairy Sci. (1965) 48:1215–23. doi: 10.3168/jds.S0022-0302(65)88430-2
38. Kononoff PJ, Heinrichs AJ, Buckmaster DR. Modification of the penn state forage and total mixed ration particle separator and the effects of moisture content on its measurements. J Dairy Sci. (2003) 86:1858–63. doi: 10.3168/jds.S0022-0302(03)73773-4
39. Shook GE. Major advances in determining appropriate selection goals. J Dairy Sci. (2006) 89:1349–61. doi: 10.3168/jds.S0022-0302(06)72202-0
40. Souza AH, Ayres H, Ferreira RM, Wiltbank MC. A new presynchronization system (Double-Ovsynch) increases fertility at first postpartum timed AI in lactating dairy cows. Theriogenology. (2008) 70:208–15. doi: 10.1016/j.theriogenology.2008.03.014
41. Littell RC. Analysis of unbalanced mixed model data: a case study comparison of ANOVA versus REML/GLS. J Agric Biol Environ Stat. (2002) 7:472–90. doi: 10.1198/108571102816
42. Xu S, Harrison JH, Chalupa W, Sniffen C, Julien W, Sato H, et al. The effect of ruminai bypass lysine and methionine on milk yield and composition of lactating cows. J Dairy Sci. (1998) 81:1062–77. doi: 10.3168/jds.S0022-0302(98)75668-1
43. Girma DD, Ma L, Wang F, Jiang QR, Callaway TR, Drackley JK, et al. Effects of close-up dietary energy level and supplementing rumen-protected lysine on energy metabolites and milk production in transition cows. J Dairy Sci. (2019) 102:7059–72. doi: 10.3168/jds.2018-15962
44. Swanepoel N, Robinson PH, Erasmus LJ. Amino acid needs of lactating dairy cows: Impact of feeding lysine in a ruminally protected form on productivity of lactating dairy cows. Anim Feed Sci Technol. (2010) 157:79–94. doi: 10.1016/j.anifeedsci.2010.02.008
45. Alharthi AS, Batistel F, Abdelmegeid MK, Lascano G, Parys C, Helmbrecht A, et al. Maternal supply of methionine during late-pregnancy enhances rate of Holstein calf development in utero and postnatal growth to a greater extent than colostrum source. J Anim Sci Biotechnol. (2018) 9:83. doi: 10.1186/s40104-018-0298-1
46. Weiss WP. Effects of feeding diets composed of corn silage and a corn milling product with and without supplemental lysine and methionine to dairy cows. J Dairy Sci. (2019) 102:2075–84. doi: 10.3168/jds.2018-15535
47. Morris DL, Kononoff PJ. Effects of rumen-protected lysine and histidine on milk production and energy and nitrogen utilization in diets containing hydrolyzed feather meal fed to lactating Jersey cows. J Dairy Sci. (2020) 103:7110–23. doi: 10.3168/jds.2020-18368
48. Giallongo F, Harper MT, Oh J, Lopes JC, Lapierre H, Patton RA, et al. Effects of rumen-protected methionine, lysine, and histidine on lactation performance of dairy cows. J Dairy Sci. (2016) 99:4437–52. doi: 10.3168/jds.2015-10822
49. Misciattelli L, Kristensen VF, Vestergaard M, Weisbjerg MR, Sejrsen K, Hvelplund T. Milk production, nutrient utilization, and endocrine responses to increased postruminal lysine and methionine supply in dairy cows. J Dairy Sci. (2003) 86:275–86. doi: 10.3168/jds.S0022-0302(03)73606-6
50. Chen ZH, Broderick GA, Luchini ND, Sloan BK, Devillard E. Effect of feeding different sources of rumen-protected methionine on milk production and N-utilization in lactating dairy cows. J Dairy Sci. (2011) 94:1978–88. doi: 10.3168/jds.2010-3578
51. Gietzen DW, Leung PMB, Rogers QR. Norepinephrine and amino acids in prepyriform cortex of rats fed imbalanced amino acid diets. Physiol Behav. (1986) 36:1071–80. doi: 10.1016/0031-9384(86)90482-8
52. Gietzen DW, Hao S, Anthony TG. Mechanisms of food intake repression in indispensable amino acid deficiency. Annu Rev Nutr. (2007) 27:63–78. doi: 10.1146/annurev.nutr.27.061406.093726
53. Patton RA. Effect of rumen-protected methionine on feed intake, milk production, true milk protein concentration, and true milk protein yield, and the factors that influence these effects: a meta-analysis. J Dairy Sci. (2010) 93:2105–18. doi: 10.3168/jds.2009-2693
54. Ordway RS, Boucher SE, Whitehouse NL, Schwab CG, Sloan BK. Effects of providing two forms of supplemental methionine to periparturient Holstein dairy cows on feed intake and lactational performance. J Dairy Sci. (2009) 92:5154–66. doi: 10.3168/jds.2009-2259
55. Robinson PH, Swanepoel N, Shinzato I, Juchem SO. Productive responses of lactating dairy cattle to supplementing high levels of ruminally protected lysine using a rumen protection technology. Anim Feed Sci Technol. (2011) 168:30–41. doi: 10.1016/j.anifeedsci.2011.03.019
56. Bauchart D, Durand D, Gruffat D, Chilliard Y. Mechanisms of liver steatosis in early lactation cows - Effects of hepatoreceptor agents. In: Proceedings of the Cornell Nutrition Conference for Feed Manufactures [Internet]. (1998), p. 27–37. Available online at: http://agris.fao.org/agris-search/search.do?recordID=US201302906454 (accessed December 23, 2019).
57. Hanigan MD, Crompton LA, Reynolds CK, Wray-Cahen D, Lomax MA, France J. An integrative model of amino acid metabolism in the liver of the lactating dairy cow. J Theor Biol. (2004) 228:271–89. doi: 10.1016/j.jtbi.2004.01.010
58. KríŽová L, Richter M, Trináctý J, Ríha J, Kumprechtová D. The effect of feeding live yeast cultures on ruminal pH and redox potential in dry cows as continuously measured by a new wireless device. Czech J Anim Sci. (2011) 56:37–45. doi: 10.17221/39/2010-CJAS
59. Allen MS, Piantoni P. Metabolic control of feed intake. Vet Clin North Am Food Anim Pract. (2013) 29:279–97. doi: 10.1016/j.cvfa.2013.04.001
60. Waterman R, Schultz LH. Methionine hydroxy analog treatment of bovine ketosis: effects on circulating metabolites and interrelationships. J Dairy Sci. (1972) 55:1513–6. doi: 10.3168/jds.S0022-0302(72)85707-2
61. Junior VC, Lopes F, Schwab CG, Toledo MZ, Collao-Saenz EA. Effects of rumen-protected methionine supplementation on the performance of high production dairy cows in the tropics. PLoS ONE. (2021) 16:e0243953. doi: 10.1371/journal.pone.0243953
62. Trináctý J, KríŽová L, Richter M, Cerný V, Ríha J. Effect of rumen-protected methionine, lysine or both on milk production and plasma amino acids of high-yielding dairy cows. Czech J Anim Sci. (2009) 54:239–48. doi: 10.17221/1730-CJAS
63. Kudrna V, Illek J, Marounek M, Nguyen Ngoc A. Feeding ruminally protected methionine to pre- and postpartum dairy cows: effect on milk performance, milk composition and blood parameters. Czech J Anim Sci. (2009) 54:395–402. doi: 10.17221/1684-CJAS
64. Davidson S, Hopkins BA, Odle J, Brownie C, Fellner V, Whitlow LW. Supplementing limited methionine diets with rumen-protected methionine, betaine, and choline in early lactation holstein cows. J Dairy Sci. (2008) 91:1552–9. doi: 10.3168/jds.2007-0721
65. Batistel F, Arroyo JM, Bellingeri A, Wang L, Saremi B, Parys C, et al. Ethyl-cellulose rumen-protected methionine enhances performance during the periparturient period and early lactation in Holstein dairy cows. J Dairy Sci. (2017) 100:7455–67. doi: 10.3168/jds.2017-12689
66. Zhou Z, Bulgari O, Vailati-Riboni M, Trevisi E, Ballou MA, Cardoso FC, et al. Rumen-protected methionine compared with rumen-protected choline improves immunometabolic status in dairy cows during the peripartal period. J Dairy Sci. (2016) 99:8956–69. doi: 10.3168/jds.2016-10986
67. Robinson PH, Swanepoel N, Evans E. Effects of feeding a ruminally protected lysine product, with or without isoleucine, valine and histidine, to lactating dairy cows on their productive performance and plasma amino acid profiles. Anim Feed Sci Technol. (2010) 161:75–84. doi: 10.1016/j.anifeedsci.2010.07.017
68. Clements AR, Ireland FA, Freitas T, Tucker H, Shike DW. Effects of supplementing methionine hydroxy analog on beef cow performance, milk production, reproduction, and preweaning calf performance1. J Anim Sci. (2017) 95:5597–605. doi: 10.2527/jas2017.1828
69. Redifer C, Tucker H, Loy D, Gunn P. 266 Evaluation of peri-partum supplementation of methionine hydroxy analog on cow-calf performance. J Anim Sci. (2018) 96(suppl_3):90–1. doi: 10.1093/jas/sky404.200
70. Larsen M, Lapierre H, Kristensen NB. Abomasal protein infusion in postpartum transition dairy cows: effect on performance and mammary metabolism. J Dairy Sci. (2014) 97:5608–22. doi: 10.3168/jds.2013-7247
71. Lapierre H, Berthiaume R, Raggio G, Thivierge MC, Doepel L, Pacheco D, et al. The route of absorbed nitrogen into milk protein. Anim Sci. (2005) 80:11–22. doi: 10.1079/ASC41330011
72. Giallongo F, Harper MT, Oh J, Parys C, Shinzato I, Hristov AN. Histidine deficiency has a negative effect on lactational performance of dairy cows. J Dairy Sci. (2017) 100:2784–800. doi: 10.3168/jds.2016-11992
73. Lee C, Hristov AN, Cassidy TW, Heyler KS, Lapierre H, Varga GA, et al. Rumen-protected lysine, methionine, and histidine increase milk protein yield in dairy cows fed a metabolizable protein-deficient diet. J Dairy Sci. (2012) 95:6042–56. doi: 10.3168/jds.2012-5581
74. Yoder PS, Huang X, Teixeira IA, Cant JP, Hanigan MD. Effects of jugular infused methionine, lysine, and histidine as a group or leucine and isoleucine as a group on production and metabolism in lactating dairy cows. J Dairy Sci. (2020) 103:2387–404. doi: 10.3168/jds.2019-17082
75. Lapierre H, Martineau R, Hanigan MD, Van Lingen HJ, Kebreab E, Spek JW, et al. Review: Impact of protein and energy supply on the fate of amino acids from absorption to milk protein in dairy cows. Animal. (2020) 14:S87–102. doi: 10.1017/S1751731119003173
76. Guliński P, Salamończyk E, Młynek K. Improving nitrogen use efficiency of dairy cows in relation to urea in milk - a review. Anim Sci Pap Rep. (2016) 34:5–24.
77. Calsamiglia S, Ferret A, Reynolds CK, Kristensen NB, van Vuuren AM. Strategies for optimizing nitrogen use by ruminants. Animal. (2010) 4:1184–96. doi: 10.1017/S1751731110000911
78. Arriola Apelo SI, Bell AL, Estes K, Ropelewski J, de Veth MJ, Hanigan MD. Effects of reduced dietary protein and supplemental rumen-protected essential amino acids on the nitrogen efficiency of dairy cows. J Dairy Sci. (2014) 97:5688–99. doi: 10.3168/jds.2013-7833
79. Schukken YH, Wilson DJ, Welcome F, Garrison-Tikofsky L, Gonzalez RN. Monitoring udder health and milk quality using somatic cell counts. Vet Res. (2003) 34:579–96. doi: 10.1051/vetres:2003028
80. Alhussien MN, Dang AK. Milk somatic cells, factors influencing their release, future prospects, and practical utility in dairy animals: an overview. Vet World. (2018) 11:562–77. doi: 10.14202/vetworld.2018.562-577
81. Chase LE. Environmental considerations in developing dairy rations. In: Cornell Nutrition Conference for Feed Manufacturers (Ithaca, New York, USA). (1994).
82. Yan T, Frost JP, Agnew RE, Binnie RC, Mayne CS. Relationships among manure nitrogen output and dietary and animal factors in lactating dairy cows. J Dairy Sci. (2006) 89:3981–91. doi: 10.3168/jds.S0022-0302(06)72441-9
83. Wang C, Liu HY, Wang YM, Yang ZQ, Liu JX, Wu YM, et al. Effects of dietary supplementation of methionine and lysine on milk production and nitrogen utilization in dairy cows. J Dairy Sci. (2010) 93:3661–70. doi: 10.3168/jds.2009-2750
84. Vargas-Rodriguez CF, Yuan K, Titgemeyer EC, Mamedova LK, Griswold KE, Bradford BJ. Effects of supplemental chromium propionate and rumen-protected amino acids on productivity, diet digestibility, and energy balance of peak-lactation dairy cattle. J Dairy Sci. (2014) 97:3815–21. doi: 10.3168/jds.2013-7767
85. Noftsger S, St-Pierre NR. Supplementation of methionine and selection of highly digestible rumen undegradable protein to improve nitrogen efficiency for milk production. J Dairy Sci. (2003) 86:958–69. doi: 10.3168/jds.S0022-0302(03)73679-0
86. Baez GM, Barletta R V, Guenther JN, Gaska JM, Wiltbank MC. Effect of uterine size on fertility of lactating dairy cows. Theriogenology. (2016) 85:1357–66. doi: 10.1016/j.theriogenology.2015.04.022
87. Peñagaricano F, Souza AH, Carvalho PD, Driver AM, Gambra R, Kropp J, et al. Effect of maternal methionine supplementation on the transcriptome of bovine preimplantation embryos. PLoS ONE. (2013) 8:e72302. doi: 10.1371/journal.pone.0072302
88. Souza AH, Carvalho PD, Dresch AR, Vieira LM, Hackbart KS, Luchini D, et al. Effect of methionine supplementation during postpartum period in dairy cows II: embryo quality. J Dairy Sci. (2012) 95(E-suppl. 1):T181.
89. Sun L, Zhang H, Wang Z, Fan Y, Guo Y, Wang F. Dietary rumen-protected arginine and N-carbamylglutamate supplementation enhances fetal growth in underfed ewes. Reprod Fertil Dev. (2018) 30:1116. doi: 10.1071/RD17164
90. Ikeda S, Sugimoto M, Kume S. Importance of methionine metabolism in morula-to-blastocyst transition in bovine preimplantation embryos. J Reprod Dev. (2012) 58:91–7. doi: 10.1262/jrd.11-096H
91. Wallace HM, Fraser A V, Hughes A. A perspective of polyamine metabolism. Biochem J. (2003) 376:1–14. doi: 10.1042/bj20031327
92. Bistulfi G, Diegelman P, Foster BA, Kramer DL, Porter CW, Smiraglia DJ. Polyamine biosynthesis impacts cellular folate requirements necessary to maintain S -adenosylmethionine and nucleotide pools. FASEB J. (2009) 23:2888–97. doi: 10.1096/fj.09-130708
93. Lefèvre PLC, Palin M-F, Murphy BD. Polyamines on the Reproductive Landscape. Endocr Rev. (2011) 32:694–712. doi: 10.1210/er.2011-0012
94. Gilmore HS, Young FJ, Patterson DC, Wylie ARG, Law RA, Kilpatrick DJ, et al. An evaluation of the effect of altering nutrition and nutritional strategies in early lactation on reproductive performance and estrous behavior of high-yielding Holstein-Friesian dairy cows. J Dairy Sci. (2011) 94:3510–26. doi: 10.3168/jds.2010-3547
Keywords: amino acids, peak of milk, metabolizable protein, pregnancy rate, β-hydroxybutyrate, energy balance, milk production, body condition score
Citation: Elsaadawy SA, Wu Z and Bu D (2022) Feasibility of Supplying Ruminally Protected Lysine and Methionine to Periparturient Dairy Cows on the Efficiency of Subsequent Lactation. Front. Vet. Sci. 9:892709. doi: 10.3389/fvets.2022.892709
Received: 09 March 2022; Accepted: 09 May 2022;
Published: 14 June 2022.
Edited by:
Ahmed Elolimy, National Research Centre, EgyptReviewed by:
Kyle McLean, The University of Tennessee, Knoxville, United StatesAudino Melgar Moreno, Agricultural Innovation Institute of Panama (IDIAP), Panama
Copyright © 2022 Elsaadawy, Wu and Bu. This is an open-access article distributed under the terms of the Creative Commons Attribution License (CC BY). The use, distribution or reproduction in other forums is permitted, provided the original author(s) and the copyright owner(s) are credited and that the original publication in this journal is cited, in accordance with accepted academic practice. No use, distribution or reproduction is permitted which does not comply with these terms.
*Correspondence: Dengpan Bu, YnVkZW5ncGFuQGNhYXMuY24=