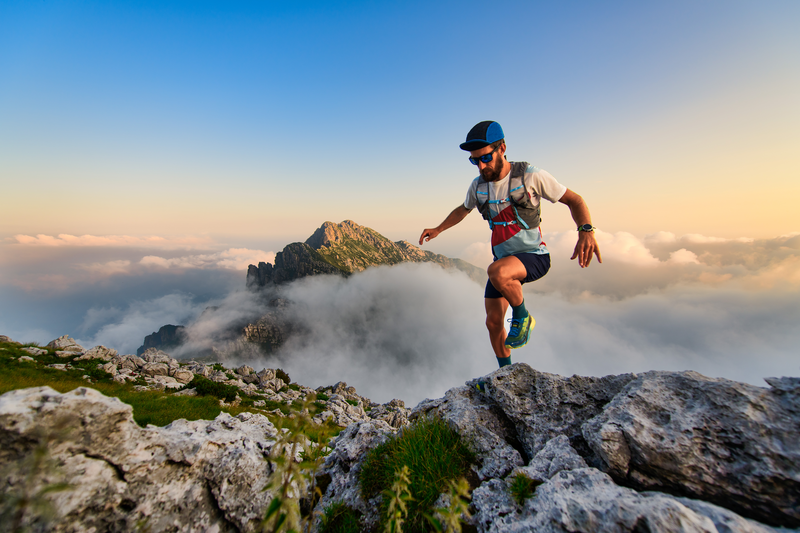
94% of researchers rate our articles as excellent or good
Learn more about the work of our research integrity team to safeguard the quality of each article we publish.
Find out more
ORIGINAL RESEARCH article
Front. Vet. Sci. , 29 April 2022
Sec. Parasitology
Volume 9 - 2022 | https://doi.org/10.3389/fvets.2022.891672
This article is part of the Research Topic Alternatives for the Control of Parasites to Promote Sustainable Livestock View all 9 articles
Ascarid nematodes are the most common and harmful nematodes parasites in animals. By analyzing genetic variation, this study explores the genetic and phylogenetic relationship among ascarids from 11 different hosts. This study collected ascarid samples from the feces of nine animal species in Changsha Ecological Zoo of Hunan Province and two animal kinds in the College of Veterinary Medicine of Hunan Agricultural University. The mitochondrial gene (pcox1) and ribosomal ITS sequences were amplified, sequenced, and analyzed by PCR to identify the species of the samples. The phylogenetic tree was constructed based on two genes (cox1 and ITS) by the Neighbor-joining method, and the phylogenetic relationship was analyzed. The sequencing results showed that the sequence lengths of pcox1 and ITS genes in the samples were 441 bp and 838–1,177 bp, respectively. The difference rates were 0.00–1.70% in pcox1 gene and 0.00–7.30% in ITS gene. Phylogenetic analysis showed that ascarid worms from the white lion, Northeast tiger, South China tiger and cheetah were identified as Toxascaris leonina. Ascarids from the zebra were identified as Parascaris equorum, while those from chicken and peacocks were identified as Ascaridia galli. Ascarids of wolf and dog origin were Toxocara canis, the snake ascarids belonged to Ophidascaris filaria, and the bear ascarids belonged to Baylisascaris transfuga. There was a significant gap between different kinds of ascarid worms. We found that these two mitochondrial genes pcox1 and ITS showed a common characteristic that the intraspecific differences were significantly smaller than the interspecific differences, confirming that these two genes could be used as interspecific genetic markers for molecular identification of different ascarids origins. The intraspecific variation rate of the ITS gene was higher than that of pcox1, indicating that ITS can also be used in the genetic research of Ascaris species development. This study revealed the genetic evolution and phylogeny of ascarids in wild animals, and our results will help prevent and control ascarids in wild animals.
Ascarid nematodes are the most common and harmful internal parasites in animals, infecting all kinds of animals. Due to environmental factors and repeated infection, there are many kinds of ascarids in animals, and the infection intensity is high (1). Ascarids nematodes are mainly parasitic in the intestinal tract of animals and can cause symptoms such as weight loss, anemia, anorexia, diarrhea, abdominal pain, or intestinal dysfunction. In severe cases, this roundworm can cause intestinal obstruction or intestinal rupture. Ectopic parasitism can cause liver necrosis, pancreatic hemorrhagic necrosis, and pneumonia, which seriously threaten the host's life. In addition, following ingestion of infectious eggs, larvae can cause tissue damage and inflammatory reaction when they migrate into human and animals tissue. Ascarid larvae of animal origin can also migrate to the eyes and brain tissues of abnormal hosts (human beings), leading to visceral larval migration, ocular larval migration, and neurolarval migration, leading to neurological symptoms, visual impairment, or blindness. Moreover, parasites with neuroinvasive stages, such as Toxocara canis, can cause detrimental damage to the brain of intermediate or paratenic hosts (2). Therefore, ascarid nematodes possess a paramount zoonotic concern (3, 4). Identifying different ascarids is conducive to preventing and treating Ascarids infection in wild animals.
Due to the genetic variation caused by host and geographical environment, the limitations of ascarid morphological classification and identification are becoming more and more apparent. Mitochondrial DNA is an extracellular circular genetic material that, compared with nuclear genes, has a small molecular weight, simple structure, fast evolution rate, and lack of recombination (5). The mitochondrial gene cytochrome C oxidase subunit 1 (cox1) is relatively conservative, suitable for parasite classification, identification, and phylogenetic analysis (6). ITS is an internal transcribed spacer mediated by ribosomal DNA (rDNA) between 18S and 28S, including ITS-1 and ITS-2 sequences. This sequence has a fast evolution speed and small length. Coupled with coevolution, this fragment is consistent among different genome units, suitable for various molecular operations (7). Using genetic markers in rDNA can accurately solve taxonomic problems (8). Blouin (9) believes that using cox1 and nad4 genes in mtDNA is more effective as genetic markers to identify ascarids species, especially hidden species. Zhu et al. (10) amplified and sequenced the rDNA internal transcribed spacer (ITS) of Toxocara canis, Toxocara felis, and a kind of Toxocara lumbricoides from Malaysia by PCR. These ascarids could be classified by using ITS as a genetic marker (10). Presently, some researchers have used mitochondrial genes (cox1, cytb, and nad1) and ribosomal genes (ITS and 5.8S) for species identification and intraspecific genetic variation of Ascarids (11–15). To explore the genetic evolution relationship between ascarids from nine wild animal species in Changsha Ecological Zoo, Hunan Province and ascarids from chicken and dogs in the College of Veterinary Medicine, Hunan Agricultural University. The ascarid worm species were determined by amplifying the pcox1 and ITS genes, and their genetic evolution relationship with other ascarids was analyzed. This study would further support wild animals'molecular epidemiology and population genetics of ascarid worms.
The Ascarid samples in this study were mainly collected from animals of the Changsha Ecological Zoo in Hunan Province, including white lions (No.: WL1, WL2, Wl3), Northeast tigers (No.: ST1, ST2, ST3), South China tigers (No.: SCT1, SCT2, SCT3), cheetahs (No.: AJ1, AJ2, AJ3), zebras (No.: PE1, PE2, PE3), peacocks (No.: P1, P2, P3), wolfs (No.: CL1, CL2, CL3), snakes (No.: S1, S2, S3), and bears (No.: B1, B2, B3). Chicken (No.: A1, A2, A3) and dogs (No.: C1, C2, C3) parasite samples were collected from the College of Veterinary Medicine of Hunan Agricultural University. After washing, the worms were stored in 70% alcohol at −20°C.
A two cm part of each sample was cut and repeatedly washed with double distilled water six times for 5 min each time. Sterile tweezers were used to put the moistened sample into a 1.5 mL Eppendorf centrifuge tube, and 200 μL GA buffer solution and 20 μL protease K solution were added and mixed and incubated in a 37°C constant temperature water bath for overnight digestion. The digested worm suspension was used to extract DNA according to Tiangen genomic DNA extraction kit (Beijing, China). The PCR amplification system was 25 μL: 12.5 μL Taq PCR Master Mix (Bao Bioengineering Co., Dalian, China), 9.5 μL ddH2O, upstream and downstream primers (100 pmol /μL, 0.5 μL each), and 2 μL template DNA. At the same time, double distilled water was used as blank control instead of template DNA. Two pairs of primers designed in previous studies (16, 17) were used to amplify pcox1 and ITS genes. PCR amplification conditions were as follows: pre denaturation at 94°C for 5 min; denaturation at 94°C for 30 s, annealing at 50°C for 30 s, and extension at 72°C for 30 s, 35 cycles; and final extension at 72°C for 5 min. After recovery and purification by TaKaRa MiniBEST Agarose Gel DNA Extraction Kit Ver.4.0, the PCR products were sequenced by Beijing Qingke Xinye Biotechnology Co., Ltd.
The sequencing results were analyzed by DNAstar 5.0 software, and the pcox1 and ITS sequences of ascarids from different hosts were compared with those of other Ascaris in GenBank. We used DnaSP V6.12 software to calculate the sequence haplotypes, nucleotide diversity, haplotypes diversity, and the average number of nucleotide differences. Using Mega 7.0 software, Kimura 2-parameter model, NJ method (Neighbor-Joining) was used to draw the phylogenetic tree, and the bootstrap test was calculated based on 1,000 bootstrap replicates.
The sequence lengths of pcox1 and ITS genes of ascarids from different hosts were about 450 bp and 1,000 bp, respectively, which was consistent with the expected fragment length (Supplementary Figures 1, 2), and there was no nonspecific band.
After comparison and correction, 33 different homologous ascarid samples in this study belonged to T. leonina, P. equorum, A. galli, T. canis, O. filaria, and B. transfuga. The amplification results of pcox1 and ITS genes are shown in Table 1. The sequences of pcox1 and ITS genes obtained by sequencing have been uploaded to the GenBank database, and the accession number of pcox1 sequences are OM867278-OM867307 and OM901120-OM901122. The accession numbers of ITS sequences are OM876348-OM876380.
Further nucleotide sequence homology analysis is shown in Table 2. The main differences were base exchange and base deletion.
Phylogenetic tree 1 was constructed by using the pcox1 gene to explore the genetic distance of the pcox1 gene of ascarid worm from different host sources within and between species (Figure 1), which was in the same branch as T. leonina (NC023504) but far away from the branches of other ascarids, such as Baylisascaris schroederi (NC015927), Baylisascaris ailuri (HQ671080), Baylisascaris columnaris (KY580739), Toxocara vitulorum (AJ920062), T. canis (NC010690), Toxocara cati (NC010773), Toxocara malaysiensis (NC010527), Ascaris suum (NC001327), and Ascaris lumbricoides (HQ704900).
Figure 1. Neighbor-Joining estimatesof the phylogenetic relationships of ascarids based on pcox1 gene computed in MEGA version 7.0 under the Kimura 2-parameter model; The confidence levels in each node were assessed with the bootstrap method (1,000 pseudoreplicates) and bootstrap values >50; The genotypes identified in this study are indicated by filled triangle.
Phylogenetic tree 2 was constructed by using the ITS gene to explore the genetic distance of the ITS gene of ascarid worm from different host sources within and between species (Figure 2), which was in the same branch as T. leonina (MN175138) and closely related to B. transfuga (HM594951), Baylisascaris procyonis (MH030596), A. suum (AB571302), and A. lumbricoides (AB571301). The difference between this sequence and T. canis (JN617989) and T. cati (KY0030684) was noticeable.
Figure 2. Neighbor-Joining estimates of the phylogenetic relationships of ascarids based on ITS gene computed in MEGA version 7.0 under the Kimura 2-parameter model; The confidence levels in each node were assessed with the bootstrap method (1,000 pseudoreplicates) and bootstrap values >50; The genotypes identified in this study are indicated by filled triangle.
Phylogenetic tree 3 was constructed using the pcox1 gene to explore the genetic distance of the pcox1 gene of ascarid worm from different host sources within and between species (Figure 3), and sequences PE1, PE2 and PE3 were in the same branch as P. equorum (MK209655). Sequences A1, A3, P1, P2, and P3 were in the same branch as A. galli (KX266856) and were closely related to T. vitulorum (AJ920062). Sequences C1, C2, C3, CL1, CL2 and CL3 were in the same branch with T. canis (AJ920054) and T. canis (AJ920053). Sequences S1, S2 and S3 were in the same branch as O. filaria (MH285590). Sequences B1, B2 and B3 were in the same branch as B. transfuga (MH795154), and closely related to other ascarids, such as B. ailuri (MH795153), B. schroederi (KJ587842), B. columnaris (KY580738), and B. procyonis (MW385526). Therefore, B. transfuga, P. equorum and O. filaria were closely related to T. leonina (AJ920063), A. suum (HQ704901), and A. lumbricoides (KY368764). These sequences were significantly different from other ascarids, such as T. cati (KY003068) and T. malaysiensis (AJ920058).
Figure 3. Neighbor-Joining estimates of the phylogenetic relationships of ascarids based on pcox1 gene computed in MEGA version 7.0 under the Kimura 2-parameter model; The confidence levels in each node were assessed with the bootstrap method (1,000 pseudoreplicates) and bootstrap values >50; The genotypes identified in this study are indicated by filled triangle.
Phylogenetic tree 4 was constructed by using the ITS gene to explore the genetic distance of the ITS gene of ascarid worms from different host sources within and between species (Figure 4), and sequences PE1, PE2 and PE3 were in the same branch as P. equorum (MT579850). Sequences A1, A2, A3, P1, P2 and P3 were in the same branch as A. galli (MW827790). Sequences C1, C2, C3, CL1, CL2 and CL3 were in the same branch with T. canis (JN617989 and JF837169) and closely related to T. cati (KY003083), T. vitulorum (KY442062), and T. leonina (MN175138). Sequences S1, S2 and S3 were in the same branch as O. baylisi (MW837142). Sequences B1, B2 and B3 were in the same branch as B. transfuga (AB571304) and closely related to B. columnaris (MH030595) and B. procyonis (MZ092855). In addition, T. canis, T. cati, T. vitulorum, and O. baylisi were closely related to T. leonina (MN175138). B. transfuga, P. equorum, A. suum (MH030604), A. lumbricoides (LC422643) were closely related. These sequences were significantly different from other ascarids.
Figure 4. Neighbor-Joining estimates of the phylogenetic relationships of ascarids based on ITS gene computed in MEGA version 7.0 under the Kimura 2-parameter model; The confidence levels in each node were assessed with the bootstrap method (1,000 pseudoreplicates) and bootstrap values >50;The genotypes identified in this study are indicated by filled triangle.
In this study, ascarid samples from different 11 hosts were compared and analyzed for the first time. The amplified 33 different homologous ascarid samples belonged to T. leonina, P. equorum, A. galli, T. canis, O. filaria, and B. transfuga. And the two mitochondrial genes pcox1 and ITS showed the commonness that the intraspecific difference is significantly less than the interspecific difference. These results were consistent with those of Jin (18), He et al. (19), He et al. (11), Xie et al. (20), Huang et al. (21), and Sun (22). Our results confirm that the interspecific variation of the pcox1 and ITS genes in ascarids samples from different hosts was more significant than the intraspecific variation.
Nucleotide diversity is an essential indicator of mitochondrial genetic variation in a population. Nucleotide diversity considers the proportion of various mitochondrial haplotypes in the population. Thus, it is more accurate than the simple average genetic distance in reflecting the degree of mitochondrial polymorphism within a population. For most animals, PI values (nucleotide diversity) above 0.01 are considered to be highly variable (23). According to the analysis in Table 3, the two mitochondrial genes pcox1 and ITS showed the commonness that the intraspecific difference is significantly less than the interspecific difference, indicating that these two genes can be used as interspecific genetic markers for the molecular identification of different homologous ascarids. At the same time, the intraspecific variation rate of the ITS gene was higher than that of pcox1, suggesting that it can also be used for genetic research, such as ascarid germline development and molecular identification.
The phylogenetic trees constructed based on the pcox1 and ITS genes showed that 32 sample strains of ascarid worms from different host sources (except A2) can cluster with their corresponding species and genera to form a branch. Nematodes in the genus Toxocara are distant relatives of the free-living, soil (and laboratory)-dwelling roundworm Caenorhabditis elegans (24). T. leonina of Amur tiger and other felines clustered into one clade, showing a closer relationship than canines, which is identical to the result of Peng et al. (25). P. equorum was closely related to A. suum and B. transfuga, which is a similar result to Gao et al. (26). Interestingly, when building a tree based on the ITS gene, T. leonina is closely related to T. canis, T. cati and T. vitulorum, which is different from building a tree based on the pcox1 gene. Scholars have found that it is limited to reconstructing the genetic relationship between species based on a single or a few genes. Due to horizontal gene transfer (HGT), nucleotide substitution saturation, interspecific hybridization and paralog, the inconsistency between a gene tree and a species tree will appear when using a single gene to build trees (27).
In summary, both pcox1 and ITS genes can be used as molecular markers to study the genetic variation of ascarids from wild animals. In addition, the ascarid worm samples were collected from white lions, Amur tigers, South China tigers, cheetahs, zebras, peacocks, wolfs, snakes and bears from Changsha Ecological Zoo in Hunan Province, suggesting that other wild animals raised in this area also have the risk of ascarid infection. Thus, it is necessary to formulate a perfect anthelmintic regime to prevent repeated and recessive infections and reduce the loss caused in wild animals due to ascarid worms.
The datasets presented in this study can be found in online repositories. The names of the repository/repositories and accession number(s) can be found in the article/Supplementary Material.
WL, S-YC, Q-GQ, and T-FG: conceptualization. WL, S-YC, Q-GQ, T-FG, and H-LM: data curation, visualization, and validation. S-YC, T-FG, and H-LM: formal analysis. WL, S-YC, Q-GQ, T-FG, H-LM, J-LH, X-RX, W-CL, and FL: investigation and methodology. S-YC, T-FG, H-LM, and WL: writing original draft preparation. WL and Q-GQ: funding. All authors have read and agreed to the published version of the final manuscript.
This project was supported by the Natural Science Foundation of Hunan Province, China (2021JJ30335) and the Horizontal Scientific Research Project of Hunan Province, China (202105000152).
The authors declare that the research was conducted in the absence of any commercial or financial relationships that could be construed as a potential conflict of interest.
All claims expressed in this article are solely those of the authors and do not necessarily represent those of their affiliated organizations, or those of the publisher, the editors and the reviewers. Any product that may be evaluated in this article, or claim that may be made by its manufacturer, is not guaranteed or endorsed by the publisher.
The Supplementary Material for this article can be found online at: https://www.frontiersin.org/articles/10.3389/fvets.2022.891672/full#supplementary-material
Supplementary Figure 1. PCR amplification products of mitochondrial pcox1 gene of Ascaris. M: DL500 DNA Marker; 1-33: Samples of different hosts ascarids; +: Positive; -: Negative.
Supplementary Figure 2. PCR amplification products of mitochondrial ITS gene of Ascaris. M: DL500 DNA Marker; 1-33: Samples of different hosts ascarids; +: Positive; -: Negative.
Supplementary Figure 3. Sequence Distance of 33 samples based on pcox1 gene.
Supplementary Figure 4. Sequence Distance of 33 samples based on ITS gene.
1. Qiu QG. The parasite investigation and animal Ascaris pcox1 sequencing analysis of changsha ecological zoo. Hunan Agricultural University. (2015).
2. Waindok P, Özbakiş-Beceriklisoy G, Janecek-Erfurth E, Springer A, Pfeffer M, Leschnik M, et al. Parasites in brains of wild rodents (Arvicolinae and Murinae) in the city of Leipzig, Germany. Int J Parasitol Parasites Wildl. (2019) 10:211–7. doi: 10.1016/j.ijppaw.2019.09.004
3. Klion AD, Nutman TB. The role of eosinophils in host defense against helminth parasites. J Allergy Clin Immunol. (2004) 113:30–7. doi: 10.1016/j.jaci.2003.10.050
5. Sadaow L, Sanpool O, Phosuk I, Rodpai R, Thanchomnang T, Wijit A, et al. Molecular identification of Ascaris lumbricoides and Ascaris suum recovered from humans and pigs in Thailand, Lao PDR, and Myanmar. Parasitol Res. (2018) 117:2427–36. doi: 10.1007/s00436-018-5931-6
6. Hao GY, Wang ZW. Sequencing and phylogenetic analysis of mitochondrial cox1 and cytb gene of Trichuris ovis isolates from Liangshan. Chin J Prev Vet Med. (2018) 38:2327–33. doi: 10.16303/j.cnki.1005-4545.2018.12.17
7. Weng YB, Xie DH, Lin RQ, Li HW, Zhang DL, Wu SQ et al. PCR amplification XQ, cloning and sequencing analysis of ITS and 5.8S of rDNA from Toxoplasma gondii. Acta Veterinaria et Zootechnica Sinica. (2005) 36:70–3.
8. Hust J, Frydenberg J, Sauriau PG, Le Gall P, Mouritsen KN, Jensen KT. Use of ITS rDNA for discriminating of larval stages of two microphallid (Digenea) species using Hydrobia ulvae (Pennant, 1777) and Corophium volutator (Pallas, 1766) as intermediate hosts. Parasitol Res. (2004) 93:304–10. doi: 10.1007/s00436-004-1136-2
9. Blouin MS. Molecular prospecting for cryptic species of nematodes: mitochondrial DNA versus internal transcribed spacer. Int J Parasitol. (2002) 32:527–31. doi: 10.1016/S0020-7519(01)00357-5
10. Zhu XQ, Jacobs DE, Chilton NB, Sani RA, Cheng NA. Gasser RB. Molecular characterization of a Toxocara variant from cats in Kuala Lumpur, Malaysia. Parasitology. (1998) 117:155–64. doi: 10.1017/S0031182098002856
11. He JL, Gong TF, Tan L, Li F, Liu W. Molecular identification and genetic diversity analysis of peacock-derived Ascarid. Chin J Prev Vet Med. (2021) 1–8. doi: 10.19958/j.cnki.cn31-2031/s.20210615.005
12. Hao GY, He XQ, Zhou RK. Genetic variation in mitochondrial cytb and nad1 genes among the isolates of Ascaridia galli from Xichang city. Vet Anim Sci. (2017) 49:85–90.
13. Liang X, Liu Y. Analysis of sequence variation in mitochondrial cox1 gene of Toxocara canis isolates in China. China Animal Health Inspection. (2015) 32:66–9.
14. Wu HL, Tan MY, Liu GH Li F, Xu PY, Luo DS. Liu, Sequence Y, and phylogenetic analysis of mitochondrial cox1 and nad1 genes for Ascaridia galli isolates from Hunan Province. Acta Veterinaria et Zootechnica Sinica. (2010) 41:1592−7.
15. Jia WZ, Yan HB Ni XW, Cao P, Lou ZZ, Fu BQ, Shi WZ. Research Progress on analysis of complete mitochondrial genome for nematodes. Scientia Agricultura Sinica. (2011) 44:1255–65.
16. Gasser RB, Zhu X, McManus DP. Display of sequence variation in PCR-amplified mitochondrial DNA regions of Echinococcus by single-strand conformation polymorphism. Acta Trop. (1998) 71:107–15. doi: 10.1016/S0001-706X(98)00052-7
17. Hao GY, He XQ. Sequencing and phylogenetic analysis of ITS and 5.8S rDNA Ascaridia galli isolated from Liangshan. J Anim Sci. (2015) 42:3167–72. doi: 10.16431/j.cnki.1671-7236.2015.12.009
18. Jin YC. Sequencing and analysis of the complete mitochondrial genome of Toxascaris leonina from the Cheetah. Changsha: Hunan Agricultural University (2019).
19. He MY, Xie Y, Tian SC, Zhang ZY, Liu W. Polymorphism of Mitochondrial cox1 and nad5 genes sequence Parascaris isolate from Zebra Chinese Journal of Veterinary Medicine. (2021) 57:39–42.
20. Xie WQ, Tang W, Wang XK, Qiu QG, Gong TF. Liu, W. Genetic analysis of mitochondrial pcox1 and pnad1 genes of Toxocara canis isolated from different hosts in Hunan Province. Chin J Prev Vet Med. (2021) 43:1223–7.
21. Huang XH, Wang SC, Li SY, He JY, Zhang L, Song ZC, et al. Amplification of cox1 gene of Ascaris in Snake and analysis on phylogenetic development in Fujian Province China. Animal Health Inspection. (2021) 38:99–103.
22. Sun Y. Population genetic structure based on mitochondrial nad1 and cox1 in Baylisacaris schroederi. Chengdu: Sichuan Agricultural University (2013).
23. Hao GY, He XQ, Wan R. Genetic variation analysis of mitochondrial cox1 and nad4 genes of Ascaris galli China. Vet Anim Sci. (2017) 44:113–22. doi: 10.16431/j.cnki.1671-7236.2017.01.016
24. Despommier D. Toxocariasis: clinical aspects, epidemiology, medical ecology, molecular aspects. Clin Microbiol Rev. (2003) 16:265–72. doi: 10.1128/CMR.16.2.265-272.2003
25. Peng ZW, Ning Y, Liu D, Sun Y, Wang LX, Zhai QA, et al. Ascarid infection in wild Amur tigers (Panthera tigris altaica) in China. BMC Vet Res. (2020) 16:86. doi: 10.1186/s12917-020-02296-5
26. Gao JF, Zhang XX, Wang XX Li Q, Li Y, Xu WW, et al. According to mitochondrial DNA evidence, Parascaris equorum and Parascaris univalens may represent the same species. J Helminthol. (2019) 93:383–8. doi: 10.1017/S0022149X18000330
Keywords: Ascarids, wild animals, mitochondrial DNA, ribosomal ITS, sequence analysis, phylogenetic relationship
Citation: Chen S-Y, Qiu Q-G, Mo H-L, Gong T-F, Li F, He J-L, Li W-C, Xie X-R and Liu W (2022) Molecular Identification and Phylogenetic Analysis of Ascarids in Wild Animals. Front. Vet. Sci. 9:891672. doi: 10.3389/fvets.2022.891672
Received: 08 March 2022; Accepted: 05 April 2022;
Published: 29 April 2022.
Edited by:
Vinícius Longo Ribeiro Vilela, Instituto Federal de Educação, Ciência e Tecnologia da Paraíba, BrazilReviewed by:
Serena Cavallero, Sapienza University of Rome, ItalyCopyright © 2022 Chen, Qiu, Mo, Gong, Li, He, Li, Xie and Liu. This is an open-access article distributed under the terms of the Creative Commons Attribution License (CC BY). The use, distribution or reproduction in other forums is permitted, provided the original author(s) and the copyright owner(s) are credited and that the original publication in this journal is cited, in accordance with accepted academic practice. No use, distribution or reproduction is permitted which does not comply with these terms.
*Correspondence: Wei Liu, d2VpbGl1cHJvQGh1bmF1LmVkdS5jbg==
†These authors have contributed equally to this work
Disclaimer: All claims expressed in this article are solely those of the authors and do not necessarily represent those of their affiliated organizations, or those of the publisher, the editors and the reviewers. Any product that may be evaluated in this article or claim that may be made by its manufacturer is not guaranteed or endorsed by the publisher.
Research integrity at Frontiers
Learn more about the work of our research integrity team to safeguard the quality of each article we publish.