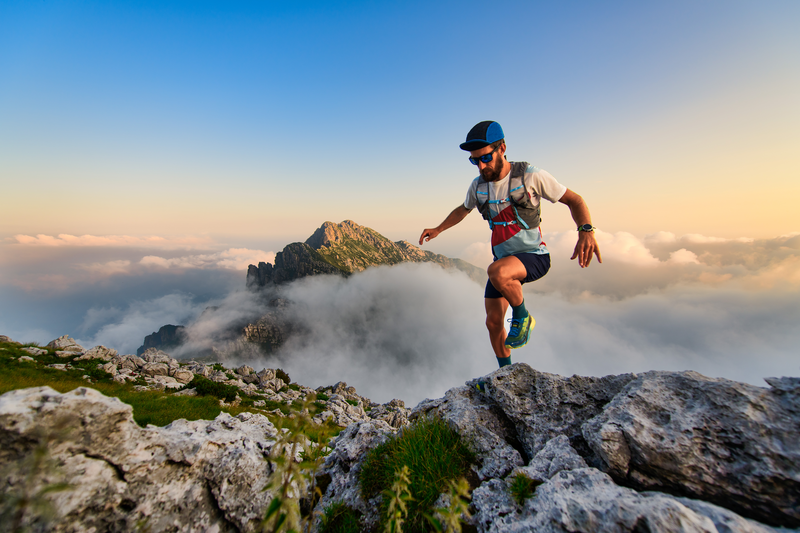
95% of researchers rate our articles as excellent or good
Learn more about the work of our research integrity team to safeguard the quality of each article we publish.
Find out more
ORIGINAL RESEARCH article
Front. Vet. Sci. , 12 May 2022
Sec. Comparative and Clinical Medicine
Volume 9 - 2022 | https://doi.org/10.3389/fvets.2022.891429
This article is part of the Research Topic Rising Stars in Comparative and Clinical Medicine: 2021 View all 21 articles
The overuse of antibiotics in poultry farming causes the accumulation of drug residue in animals' bodies and the occurrence of antibiotic-resistant bacteria, which not only compromise animals' health but ultimately endanger human health. Thus, there is an urgent need for a novel poultry feed additive to substitute for excessive antibiotics. Glycyrrhiza polysaccharides (GPS) derived from Chinese licorice have shown promising immunomodulatory effects in previous studies. The present study investigated the pharmacological effects of GPS on poultry intestines to assess whether it can be used as a feed additive. The results show that GPS can increase production of sIgA, promote the secretion activity of goblet cells, alter the gut microbial composition and lead to changes in short-chain fatty acids. GPS also elevated both Th1 and Th2 immune responses by facilitating the expression of IL-2, IL-4, IL-1β, and IFN-γ while increasing the proportion of both CD4+ and CD8+ cells in the intestine. Moreover, the results of 16S rRNA gene sequencing showed that GPS could significantly change intestinal microbiota composition in the intestine, evidenced by the increased proportion of Bacteroides, Butyricicoccus and Eisenbergiella, as well as a decreased portion of Erysipelatoclostridium, leading to a healthier intestinal microbiota composition for the host. Taken together, it can be concluded that GPS is safe to use as a novel feed additive that can be used as an alternative to prophylactic antibiotics in poultry feeding.
In recent decades, the global poultry farming industry developed rapidly in response to accelerating consumer demand. There are numerous feed additives used in industrial livestock and poultry husbandry to improve productivity, maintain intestinal health, and prevent pathogenic microorganisms (1, 2). Low-cost antibiotics are widely used in industrial farming to prevent bacterial infection and promote animal survival. However, abuse of antibiotics causes a number of unintended consequences, including drug residue in animal products and antibiotic-resistant microbes, both of which can damage human health (3). New research indicates the global death toll from such antimicrobial resistance in 2019 was upward of four million people 47. Therefore, there is an urgent need to find a novel feed additive to substitute for the overuse of antibiotics in industrial poultry farming.
The gastrointestinal tract is the first line of defense against the many considerable challenges posed by harmful pathogens ingested daily. If the health and balance of the intestine is disrupted, the entire body can become infected with pathogens. Therefore, supporting intestinal health is key to maintaining overall animal health. It is well-documented that the intestine has an expansive surface area in which microbiota thrive and interact with the immune system to achieve intestinal homeostasis and maintain host health (4, 5). SIgA, the dominant antibody found in intestinal mucosa, protects the intestine from pathogens and regulates the intestinal microbiota during its development from birth to adulthood (6, 7). In animals, sIgA deficiency can lead to immunodeficiency and incomplete intestinal barrier function, resulting in reduced poultry productivity and even death (7). Based on this, improving the level of sIgA in animals' intestinal mucosa is a useful strategy to maintain the intestine's homeostasis and host health (8).
Licorice, a traditional medicinal and food plant (9), has a long history of application in both diet and pharmacology (10). The edible and medicinal parts of licorice are the root and rhizome. Because of its sweet taste, its soaking liquid is often used as a drinking additive in China, and as a food sweetener in Europe (11). In recent years, more and more active ingredients in licorice such as polysaccharides, flavones, and saponins have been obtained, showing some potential effects in drug development and food industry (12). Glycyrrhiza polysaccharides (GPS) in particular show great potential for use in medical and food industries. GPS consist of a series of biological polysaccharides, mainly glucose, galactose, and mannose (13). Many studies have confirmed that GPS possess various pharmaceutical properties, acting as an antitumor (14), antibacterial (15), antioxidant (16), and immunomodulation (14) agent. It has also been reported that GPS could be used as an immunopotentiator, as it can directly facilitate a robust immune response to inoculation by promoting the proliferation of lymphocytes, especially T lymphocytes (13). Additionally, GPS can activate innate cells, such as macrophages and dendritic cells (DCs), which prime an immune response that can efficiently eliminate pathogens and senescent cells (17, 18). However, the effectiveness of GPS in maintaining intestinal health and regulating intestinal microbiota is still unknown.
Previous studies did not examine the question of GPS as a promoter of intestinal health, nor its potential ability as an immunopotentiator specifically for an intestinal immune response. The present study investigated whether feeding poultry GPS could benefit their overall intestinal health and beneficially regulate their intestinal microbiota. GPS was extracted and purified from Chinese licorice, then fed to male Hy-Line Brown chickens. Next, the effect of GPS on the intestine was explored and its mechanisms investigated.
Male 1-day-old Hy-Line Brown roosters were bought from Hai'an Shuangli Hatchery (Jiangsu, China). All animals were housed in wire cages under 12-h light/dark cycles at 30°C. All animal experiments were conducted in accordance with the guidelines of the Nanjing Agricultural University Institutional Animal Care and Use Committee (IACUC), detailed in the IACUC-approved protocol (No.:2021BAD34B04).
After one week of acclimatization, the roosters were randomly divided into four groups (n = 5) and treated either with isotonic saline (control group), GPS at a high dose (GPS-H, 600 mg/kg), GPS at a medium dose (GPS-M, 450 mg/kg), or GPS at a low dose (GPS-L, 300 mg/kg). The roosters in all groups were given these formulations intragastrically for 14 days. Meanwhile, the body weight of all roosters was measured every day to determine the safety of GPS. On the 15th day, all roosters were sacrificed and their contents, small intestine, bursa of Fabricius, spleen, and thymus were collected for further examination. The contents were directly transferred to −80°C for cryopreservation. The small intestine, bursa of Fabricius, bursa, and spleen were fixed with tissue fixative and stored under normal temperature conditions.
On day 15, 8 cm of the duodenum from each rooster (n = 5 per group) was collected and washed eight times with PBS (phosphate buffer solution, pH = 7.4) containing phenylmethylsulfonyl fluoride (PMSF) protease inhibitor. Next, these solutions were centrifuged at 10,000 rpm for 15 min at 4°C. Afterward, the supernatant was collected and used to determine the level of sIgA in the intestines using an enzyme-linked immunosorbent assay (ELISA).
The jejunums of 5 roosters from each group were collected, homogenized, then centrifuged at 12,000 rpm for 10 min at 4°C. Afterward, the supernatant was collected to analyze IL-2, IL-4, IL-1β, and IFN-γ levels in the intestine using ELISA kits.
On day 15, all jejunums were collected to detect proportions of CD4+ and CD8+ T cells. Jejunums were briefly incubated in primary antibodies CD4+ and CD8+. Then, secondary antibodies CY3 (red) and FITC (green) were incubated with the mixture for 1 h to stain CD4+ and CD8+, respectively. After rinsing, the expression of these markers in the specimen images was observed and captured using confocal microscopy.
The paraffin-embedded bursa of Fabricius, spleen, thymus, liver, kidney, duodenum, jejunum, and ileum were sectioned using a pathology slicer (Leica RM2016), and a hematoxylin and eosin (HE) stain was applied to the sectioned tissue. Then, the stained intestinal segments were observed by using a Nikon Eclipse 80i microscope.
The contents samples from the intestine were collected to measure the level of short-chain fatty acids (SCFAs) using gas chromatography (GC) and then calculate standard curves. The contents of the cecum were vortexed with ultrapure water at a 1:5 ratio. Then, the mixture was centrifuged at 10,000 rpm for 10 min at 4°C and the supernatant was collected. Afterward, each supernatant was mixed 1:1 with 2-methyl butyric acid. The treatment of each sample and the GC analysis were conducted based on the product instructions (19).
The jejunums were embedded in paraffin and sectioned using a Leica RM2016 pathology slicer. Then, the sections were sequentially treated with Periodic Acid Solution, Schiff reagent, and hematoxylin. After rinsing, the stained sections of the jejunums were observed by using a Nikon Eclipse 80i microscope.
Roosters' feces were snap-frozen using liquid nitrogen, followed by storage at −80°C. DNA was extracted using the FastDNA™ Spin Kit for Feces (MP Biomedicals, Santa Ana, CA) following their manual. The purity and quality of the genomic DNA were checked in 0.8% agarose gels. The V3-V4 hypervariable regions of the 16S rRNA gene were amplified with the primers 338F (ACTCCTACGGGAGGCAGCAG) and 806R (GGACTACHVGGGTWTCTAAT) then sequenced using an Illumina NovaSeq 6000 System. The raw data were screened and sequences shorter than 200 bp, those with a low-quality score (≤ 20), those containing ambiguous bases, or those that did not exactly match to primer sequences and barcode tags were removed from consideration. Qualified sequences were separated using the sample-specific barcode sequences and trimmed with the Illumina Sequencing Analysis Pipeline Version 2.6. Afterward, the dataset was analyzed using QIIME. The sequences were clustered into operational taxonomic units (OTUs) at a similarity level of 97%. Taxonomic assignments of operational taxonomic units (OTUs) representative sequences were performed with a confidence threshold of 0.8 by a Naïve Bayes classifier trained on the GreenGenes database (version 13.8).
All statistical analyses were conducted using R (version 3.5.1) and GraphPad Prism (version 8.0), with all results reported as mean ± standard deviation (SD) or box-and-whisker plots. A one-way ANOVA test of multiple comparisons followed by Dunnett's post-hoc test was used to evaluate statistical significance between groups. Differences in the relative abundances of OTUs were assessed using Tukey's honest significant difference (HSD) test in R version 3.4.0. The level of statistical significance for our analyses is p < 0.05.
To assess the effects of GPS on roosters' intestinal lining, the intestinal juice of roosters was collected to determine if GPS could increase local sIgA concentration. As shown in Figure 1A, sIgA was significantly increased in both the GPS-M and GPS-H treatment groups, relative to the control group. This suggests that GPS were able to boost production of sIgA in roosters' intestine in a dose-dependent manner.
Figure 1. (A) Effects of 14-day oral administration of GPS on intestinal secretion of sIgA in laying hens. (B) Hematoxylin-Eosin (HE) staining was used to observe the effect of GPS on the histology of the small intestine of laying hens after 14 days of oral administration. (C) Glycogen was labeled using PAS staining to show goblet cells (darker granules) in the gut, and the effect of 14-day GPS oral administration on the number of intestinal goblet cells in laying hens. *P < 0.05, **P < 0.01, and ***P < 0.001, all relative to the saline (control) group.
The physical barrier of the intestine is composed of the lamina propria, the intestinal villi, the intestinal epithelium, and various tightly attached proteins in the intestinal cells. As shown in Figure 1B, the intestinal lamina propria was structurally intact and the small intestinal epithelium was healthy across all GPS treatment groups. There was also a significant and dose-dependent increase in the number of villi in the duodenum, jejunum, and ileum of the small intestine in the GPS groups.
The secretion activity of goblet cells in the intestine significantly affects the intestinal barrier and immunity (20). Thus, we further examined whether GPS could regulate goblet cell secretion in the intestine. As shown in Figure 1C, the secretion activity of goblet cells was significantly improved after treatment with GPS, compared to the saline group. This finding suggests that GPS are able to facilitate increased secretion from intestinal goblet cells, which promotes over-all intestinal health and a robust barrier against ingested pathogens.
Short-chain fatty acids (SCFAs) including acetic acid, propionic acid, isobutyric acid, butyric acid, isovaleric acid, and valeric acid help regulate intestinal homeostasis and metabolism, which are key to intestinal health (21). Therefore, the content of these SCFAs in roosters' intestines was also examined. As shown in Figures 2A–F, the quantity of these SCFAs in the intestines of chickens from GPS-L, GPS-M, and GPS-H groups was significantly elevated compared with the control group, which suggests that GPS could promote the secretion of SCFAs. The high dose of GPS (GPS-H) elicited the strongest promotion effect compared to two lower GPS doses. Among them, the content of acetic acid, isobutyric acid, and butyric acid in the high-dose GPS group was significantly different from that in the low-dose group, indicating that this effect is dose- dependent.
Figure 2. Effects of 14-day oral administration of GPS on SCFAs production in chicken intestines. The use of gas chromatography (GC) determined that after GPS treatment, significantly increased intestinal levels of (A) acetic acid, (B) propionic acid, (C) butyric acid, (D) isobutyric acid, (E) valeric acid, and (F) isovaleric acid content. *P < 0.05, **P < 0.01, and ***P < 0.001, all relative to the saline (control) group.
Maintaining intestinal health and homeostasis is inseparable from the regulation of cytokines (22), which influence immune cells, tissue repair, and infection responses (23). As shown in Figures 3A,B, after treatment with GPS, levels of IL-2 and IL-4 were significantly elevated in the intestine compared to control group chickens treated with isotonic saline. After treatment with GPS, the production of IL-1β and IFN-γ was promoted in the intestine (see Figures 3C,D). These results suggest that GPS could enhance intestinal health through facilitation of IL-2, IL-4, IL-1β, and IFN-γ secretion.
Figure 3. After the laying hens were orally administered GPS for 14 days, the changes of cytokines in the serum of laying hens were determined by ELISA.GPS enhances the expression of (A) IL-2, (B) IL-4, (C) IL-1β, and (D) IFN-γ. *P < 0.05, **P < 0.01, and ***P < 0.001, all relative to the saline (control) group.
Having confirmed the promotion effect of GPS on intestinal cytokine expression, we further explored whether GPS would affect activation of CD4+ and CD8+ T cells, which indirectly or directly prevent pathogen invasion and maintain homeostasis in the intestinal immune response (24). The immunofluorescence results in Figure 4 show that different doses of GPS could improve expressions of CD4+ and CD8+ to different extents, compared with saline treatments. Noticeably, the levels of both CD4+ and CD8+ were highest in the GPS-H group, suggesting both that GPS are able to promote the expression of CD4+ and CD8+ in the intestine, and that the effect is dose-dependent.
Figure 4. The proportion of CD4+ and CD8+ T cells in the gut of laying hens after 14 days of oral GPS was analyzed using immunofluorescence. The nuclei were stained with DAPI (blue), the cell surface differentiation antigen CD4+ was stained with CY3 (red), and the cell surface differentiation antigen CD8+ was stained with FITC (green), which is convenient for visual inspection of the fluorescence intensity.
The influence of GPS on the intestinal barrier and local immune response may be attributed to changes in intestinal microbiota. Therefore, the experiment further investigated intestinal microbiota for changes in evenness (uniformity) and richness (the number of species observed in each sample). As shown in Figures 5A,B, Shannon and Chao1 indices, which represent the evenness and total richness of intestinal microbiota, respectively, were both significantly increased in the GPS-H group relative to the control, suggesting that GPS-H could improve the evenness and richness of intestinal microbiota. Additionally, a principal component analysis (PCA) revealed that after treatment with GPS, the intestinal microbiota composition is fundamentally altered from that of the control group, indicating that GPS could dramatically change the intestinal microbial composition (Figures 5C,D). These changes in alpha diversity (Figures 5A,B) and beta diversity (Figures 5C,D) can be attributed to GPS treatment rather a chance variation in this specific sample collection.
Figure 5. Changes in gut microbes in GPS-H-treated and untreated layers. (A) Chao1 and (B) Shannon indices represent microbial richness and evenness, respectively, represents the change in the alpha diversity of gut microbes between the two groups of samples. And (C) principal component analysis (PCA) and (D) heat map relationship (the horizontal and vertical axes are the names of the samples, and the color depth represents the similarity between samples.) The analysis represents the beta diversity between each sample variety.
The experiment further analyzed which specific intestinal microbes had changed to induce these alterations in alpha and beta diversity. From the phylum analysis shown in Figures 6A,B, it was observed that the proportion of Firmicutes were significantly decreased in the GPS-H group compared to the control. Meanwhile, relative to the control group, the proportion of Bacteroidetes was substantially increased in the GPS-H group; thus, the ratio of Firmicutes to Bacteroidetes was significantly reduced in the GPS-H group compared to the control. Notably, compared with roosters treated with saline alone, the proportion of Actinobacteria was increased in the intestines of roosters treated with GPS-H (Figure 6B), which suggests that GPS-H could not only reduce the ratio of Firmicutes to Bacteroidetes, but also regulate the proportion of specific microbes such as Actinobacteria. Further intestinal microbiota composition on a genus level is displayed in Figure 7. Bacteroides are the dominant flora in the intestines of roosters in the GPS-H group, and many microbes believed to be beneficial to the host were significantly increased in the GPS-H group compared to the control group (Figure 7A). These include Bacteroides, Butyricicoccus, Eisenbergiella, Enterococcus, Ruminococcaceae, and Lactobacillus. In addition, some pathogenic bacteria like Erysipelatoclostridium, Lachnoclostridium, and Escherichia–Shigella were significantly decreased in the GPS-H group compared to the control group (Figure 7B), suggesting that GPS are able to regulate the proportions of some harmful bacteria, which may contribute to the positive effect of GPS on roosters' intestinal health. Furthermore, this study defined which bacteria are primarily influenced by GPS using the method of linear discriminant analysis effect size (LEfSe.) As shown in Figure 8, the quantities of Bacteroidales, Bacteroidia, Lactobacillales, bacilli, Enterococcus, Butyricicoccus, and Eisenbergiella in the GPS-H group were significantly elevated relative to the control group, and the amounts of Clostridiales, Lachnospiraceae, Ruminococcaceae, Anaerotruncus, and Caproiciproducens were significantly decreased within the GPS-H group vs. the control, which accounts for the differences observed in Figure 5. These results taken together suggest that GPS-H could alter specific intestinal microbiota composition, and this change could help shape a better gut barrier and immune system.
Figure 6. Analysis of significant differences between two groups was analyzed using Metastats analysis, which compares multiple samples under two conditions to find the types of microorganisms that are significantly different in the two groups. Analysis of intestinal microbiota composition by phylum. (A) The specific intestinal microbiota composition in individuals in GPS-H and control groups. (B) The relative abundance of intestinal microbes between GPS-H and control groups.
Figure 7. Analysis of significant differences between two groups was analyzed using Metastats analysis, which compares multiple samples under two conditions to find the types of microorganisms that are significantly different in the two groups.The specific composition of intestinal microbiota by genus. (A) The intestinal microbiota structure of each individual in GPS-H and control groups and (B) the average relative abundances of those intestinal microbes.
Figure 8. The LDA Effect Size was used to analyze the comparison between the GPS-H group and the control group, and an intra-subgroup comparison analysis between the two groups was also performed to find substances with significant differences in abundance between the groups.
Commercial feed additives are rightly scrutinized for safety as well as efficacy, thus, this study also examined the toxicity of GPS on roosters. For this purpose, body weight was monitored and the bursa of Fabricius, spleen, thymus, liver, and kidneys were examined to determine the potential toxicity of GPS. As shown in Figure 9B, there are no obvious pathological changes in the bursa of Fabricius, spleen, thymus, liver, or kidneys between the control and GPS-L, GPS-M, or GPS-H groups. Body weights all increased similarly; differences between the control and the three GPS groups (GPS-L, GPS-M, and GPS-H) are negligible, as illustrated in Figure 9A. These results indicate that GPS poses no toxicity as a feed additive for roosters.
Figure 9. Examination of potential toxicity of GPS on roosters. (A) The influence of GPS on body weight. (B) The influence of GPS on immune organs (bursa of Fabricius, spleen, thymus, liver, and kidneys) after 14 days (100 × magnification, 100 μm scale bar).
Maintaining intestinal health and homeostasis is a complex balancing act influenced by many physiological factors in the interaction between the immune system, intestinal barrier, and intestinal microbiota (25). There are enormous quantities of bacteria living in animals' intestines, and differences in the composition of intestinal microbiota may dramatically alter the host's physiological and biochemical reactions, potentially destabilizing host homeostasis and general health (26). An animal's immune system recognizes and responds to the intestinal microbiota, which results in a moderate promotion of innate and adaptive immune pathways that reinforce the intestinal barrier and respond to invasive pathogens while maintaining tolerance to beneficial microbiota and antigens in food (27–29). These immune responses in the intestine help shape the intestinal microbiota composition by changing the nutrient substrates through mucus production or epithelial fucosylation (30). Thus, promoting the immune system, reinforcing the intestinal barrier, and improving intestinal microbiota are keys to enhancing animals' intestinal health. For the poultry farming industry, finding a potent feed additive to enhance animals' intestinal health is a useful strategy which can increase profits while lessening the risk of antibiotic resistant microbes threatening human health.
SIgA possesses many protective functions in the intestine, including as a defender to neutralize bacterial toxins in the gut lumen and disable viruses during transcytosis through the epithelial barrier (31). SIgA also inhibits abnormal epithelial cell translocation and excessive inflammatory responses induced by Shigella lipopolysaccharide (LPS) (32). In addition, sIgA can bind luminal bacteria into Peyer's patches (PPs) (33), which trains the immune system to tolerate beneficial bacteria and eliminate harmful bacteria (34). The present study verifies that GPS possess the ability to increase the sIgA content in the intestine, suggesting that GPS could promote roosters' intestinal health and beneficially alter intestinal microbiota. Goblet cells secrete mucus to protect the intestine and promote the expression of antimicrobial peptides that maintain intestinal homeostasis (20, 35). After treatment with GPS, secretion activity of goblet cells was significantly enhanced. This collective evidence leads to the conclusion that GPS are able to reinforce intestinal barrier health by increasing the content of sIgA and promoting the secretion activity of goblet cells.
Cytokines participate in the activation and derivation of immune cells, and thus are regarded as central to the immune system (36). Different cytokines exhibit diverse functions. They regulate the secretion of physiologically active substances and help maintain homeostasis. IL-2 and IL-4 are cytokines involved in appropriate immune responses and help avoid uncontrolled inflammation while promoting tissue repair (37). IL-1β and IFN-γ cytokines are able to protect the intestine from infection by triggering an immune response and preventing the spread of pathogens, respectively (38, 39). IL-2, a cytokine that can activate Tregs (T) cells and reinforce the inhibitory effect of Tregs on Teff, is also able to prevent the occurrence of spontaneous inflammation, and therefore plays a significant role in intestinal health (40). Our results show that GPS can promote the expression of IL-2, suggesting that GPS may regulate intestinal inflammation. IL-4, a typical Th2-type cytokine, has a potent anti-inflammatory effect (41). IFN-γ, a Th1-type cytokine, also exerts a protective effect on the intestine (42). The ratio of IL-4 to IFN-γ reflects the balance between Th2 and Th1 immune responses. The results show that GPS can increase the production of both IL-4 and IFN-γ, maintaining homeostasis in the intestine while boosting the overall immune response and promoting intestinal health. Having confirmed the effect of GPS on cytokine expression, we further explored whether immune cells in the intestine would be changed, and the proportion of CD4+ and CD8+ T cells were selected for this purpose. CD4+ and CD8+ T cells represent the Th1-type and Th2-type immune responses, respectively (43). The results showed that the numbers of both CD4+ and CD8+ T cells were increased after treatment with GPS, which is in agreement with the promotion effect on cytokine expression. Taken together, these results indicate that GPS can promote intestinal health by regulating the expression of cytokines and activation of CD4+ and CD8+ T cells while maintaining the homeostasis of Th1-type and Th2-type immune responses.
Given the interactions between sIgA, the immune system, and intestinal microbiota, our experiment further examined the effect of GPS on intestinal microbiota. It has been reported that sIgA can coat specific bacteria in the intestine, selectively enriching some members of the microbiota, as microbes vary in their propensity to acquire an sIgA coat when needed (44). Based on the increase of sIgA observed in our roosters treated with high-dose GPS (GPS-H), we further investigated the changes in these rooster's intestinal microbiotas. Many studies have concluded that the evenness and richness of intestinal microbiota are closely related to intestinal homeostasis, and the greater these measures, the more robust the immune response to pathogens (45). The results showed that GPS-H could increase both evenness and richness in the intestinal microbiota, suggesting that GPS-H could improve the host's ability to maintain intestinal homeostasis and improve immune responses. Also of note, the results show that GPS-H altered the composition of the intestinal microbiota, significantly improving the proportion of Bacteroidetes in the intestine, which could be attributed to the increase in sIgA allowing more Bacteroidetes to outcompete other microbes (46). Bacteroidetes break down complex polysaccharides like GPS into components like SCFAs and ferulic acid that they can metabolize, which would explain the jump in SCFAs in the intestine after GPS treatment (47) (Figures 2A–F). From the genus analysis, we found six bacteria significantly increased in the GPS-H group: Bacteroides, Butyricicoccus, Eisenbergiella, Enterococcus, Ruminococcaceae, and Lactobacillus. Butyricicoccus has been reported to promote the accumulation of butyric acid in the intestine (48). Meanwhile, there is evidence that chickens with a higher proportion of Eisenbergiella in their intestines possess a better metabolism and more efficiently use amino acids, nucleotides, and short-chain fatty acids (49). Enterococcus is a beneficial bacterium which plays a role in decomposing food and promoting the absorption of nutrients (50). Ruminococcaceae is the main microorganism that converts primary bile acids into secondary bile acids, which are severely lacking in patients with ulcerative colitis. Supplementation of Ruminococcaceae reduced inflammation and symptoms of colitis in mice (51). Lactobacillus is beneficial in treating irritable bowel syndrome (IBS) and reduces inflammation (51). Conversely, Erysipelatoclostridium, Lachnoclostridium, and Escherichia–Shigella are typical pathogenic bacteria in the intestine, and were seen in lower proportions after GPS-H treatment, compared to the control group. This suggests that GPS-H can effectively reduce the proportion of harmful bacteria in the intestine. Over-all, GPS-H treatment is able to regulate roosters' intestinal microbial composition in a manner beneficial for the host. Furthermore, roosters treated with GPS-H exhibited no signs of drug toxicity, which makes it possible for GPS to be used as a novel feed additive.
GPS can improve intestinal health in three ways: improving the function of the intestinal barrier by increasing levels of sIgA and promoting goblet cell secretion, boosting the activation of the immune system by increasing the expression of cytokines and elevating levels of CD4+ and CD8+ T cells in the intestine while maintaining the homeostasis of Th1 to Th2 responses, and beneficially regulating the intestinal microbiota composition. These findings, combined with no evidence of toxicity, lead us to conclude that GPS shows promise as a novel feed additive and warrants further investigation as a substitute to prophylactic antibiotics in commercial poultry.
The original contributions presented in the study are included in the article/Supplementary Material, further inquiries can be directed to the corresponding author.
The animal study was reviewed and approved by Nanjing Agricultural University No. PZ2020101.
YiW: conceptualization, funding acquisition, project administration, and supervision. YuW: data curation, writing—original draft preparation, and visualization. CW and YueW: data curation. YC: writing—original draft preparation and methodology. TZ: methodology and editing. CD: methodology. NL: data curation and visualization. KD: reviewing and editing. AN and YH: writing—reviewing and editing. HZ, XW, and HL: data validation. PH: editing. CL: writing—reviewing. All authors contributed to the article and approved the submitted version.
This research was financially supported by the National Natural Science Foundation of China (NSFC, Grant No. 31872514 and 32172900), the Open Project Program of Bei-jing Key Laboratory of Traditional Chinese Veterinary Medicine at Beijing University of Agriculture (No. kf-tcvm202101), Yunnan Provincial Science and Technology Department-Applied Basic Research Joint Special Funds of Yunnan University of Chinese Medicine [2018FF001 (-020), 2019FF002(-012)], and a project funded by the Priority Academic Program Development of Jiangsu Higher Education Institutions (PAPD). We appreciate the assistances from our distinguished colleagues in the Institute of Traditional Chinese Veterinary Medicine of Nanjing Agricultural University.
KD was employed by China Tobacco Henan Industrial Co. Ltd.
The remaining authors declare that the research was conducted in the absence of any commercial or financial relationships that could be construed as a potential conflict of interest.
All claims expressed in this article are solely those of the authors and do not necessarily represent those of their affiliated organizations, or those of the publisher, the editors and the reviewers. Any product that may be evaluated in this article, or claim that may be made by its manufacturer, is not guaranteed or endorsed by the publisher.
The author would like to express our sincere gratitude to Nanjing Agricultural University for providing reasonable experimental conditions and equipment. At the same time, thanks for the support of the above funding.
1. Clavijo V, Florez MJV. The gastrointestinal microbiome and its association with the control of pathogens in broiler chicken production: a review. Poult Sci. (2018) 97:1006–21. doi: 10.3382/ps/pex359
2. Patra AK, Amasheh S, Aschenbach JR. Modulation of gastrointestinal barrier and nutrient transport function in farm animals by natural plant bioactive compounds - A comprehensive review. Crit Rev Food Sci Nutr. (2019) 59:3237–66. doi: 10.1080/10408398.2018.1486284
3. Conradie F, Diacon AH, Ngubane N, Howell P, Everitt D, Crook AM, et al. Treatment of highly drug-resistant pulmonary tuberculosis. N Engl J Med. (2020) 382:893–902. doi: 10.1056/NEJMoa1901814
4. Hooper LV, Macpherson AJ. Immune adaptations that maintain homeostasis with the intestinal microbiota. Nat Rev Immunol. (2010) 10:159–69. doi: 10.1038/nri2710
5. Aureli P, Capurso L, Castellazzi AM, Clerici M, Giovannini M, Morelli L, et al. Probiotics and health: an evidence-based review. Pharmacol Res. (2011) 63:366–76. doi: 10.1016/j.phrs.2011.02.006
6. Hendrickx AP, Top J, Bayjanov JR, Kemperman H, Rogers MR, Paganelli FL, et al. Antibiotic-driven dysbiosis mediates intraluminal agglutination and alternative segregation of Enterococcus faecium from the intestinal epithelium. mBio. (2015) 6:e01346–e01315. doi: 10.1128/mBio.01346-15
7. Bemark M, Boysen P, Lycke NY. Induction of gut IgA production through T cell-dependent and T cell-independent pathways. Ann N Y Acad Sci. (2012) 1247:97–116. doi: 10.1111/j.1749-6632.2011.06378.x
8. Cheng SL, Li X, Lehmler HJ, Phillips B, Shen D, Cui JY. Gut microbiota modulates interactions between polychlorinated biphenyls and bile acid homeostasis. Toxicol Sci. (2018) 166:269–87. doi: 10.1093/toxsci/kfy208
9. Aydin BD. Investigation of antibacterial effects of some medicinal plants and spices on food pathogens. Kafkas Universitesi Veteriner Fakultesi Dergisi. (2008) 14:83–7. doi: 10.9775/kvfd.2008.11-A
10. Chen M, Zhu J, Kang J, Lai X, Gao Y, Gan H, et al. Exploration in the mechanism of action of licorice by network pharmacology. Molecules. (2019) 24:2959. doi: 10.3390/molecules24162959
11. Li Y, Gong Y, Zhang X, Wang J, Cheng Y, Liu F, et al. Exploring the synergistic mechanism of Gegen Qinlian Decoction on the Wnt signaling pathway using an integrated strategy of network pharmacology and RNA-seq. J Ethnopharmacol. (2021) 278:114283. doi: 10.1016/j.jep.2021.114283
12. Hayama K, Takahashi M, Suzuki M, Ezawa K, Yamazaki M, Matsukawa T, et al. [Anti-Candida activity of aroma candy and its protective activity against murine oral candidiasis]. Med Mycol J. (2015) 56:J23–29. doi: 10.3314/mmj.56.J23
13. Wu Y, Yi L, Li E, Li Y, Lu Y, Wang P, et al. Optimization of Glycyrrhiza polysaccharide liposome by response surface methodology and its immune activities. Int J Biol Macromol. (2017) 102:68–75. doi: 10.1016/j.ijbiomac.2017.04.006
14. Ayeka PA, Bian Y, Githaiga PM, Zhao Y. The immunomodulatory activities of licorice polysaccharides (Glycyrrhiza uralensis Fisch.) in CT 26 tumor-bearing mice. BMC Complement Altern Med. (2017) 17:536. doi: 10.1186/s12906-017-2030-7
15. Wittschier N, Faller G, Hensel A. Aqueous extracts and polysaccharides from liquorice roots (Glycyrrhiza glabra L.) inhibit adhesion of Helicobacter pylori to human gastric mucosa. J Ethnopharmacol. (2009) 125:218–23. doi: 10.1016/j.jep.2009.07.009
16. Rozi P, Abuduwaili A, Ma S, Bao X, Xu H, Zhu J, et al. Isolations, characterizations and bioactivities of polysaccharides from the seeds of three species Glycyrrhiza. Int J Biol Macromol. (2020) 145:364–71. doi: 10.1016/j.ijbiomac.2019.12.107
17. Cheng A, Wan F, Wang J, Jin Z, Xu X. Macrophage immunomodulatory activity of polysaccharides isolated from Glycyrrhiza uralensis Fish. Int Immunopharmacol. (2008) 8:43–50. doi: 10.1016/j.intimp.2007.10.006
18. Aipire A, Yuan P, Aimaier A, Cai S, Mahabati M, Lu J, et al. Preparation, characterization, and immuno-enhancing activity of polysaccharides from Glycyrrhiza uralensis. Biomolecules. (2020) 10:159. doi: 10.3390/biom10010159
19. Rohe I, Vahjen W, Metzger F, Zentek J. Effect of a “diluted” diet containing 10% lignocellulose on the gastrointestinal tract, intestinal microbiota, and excreta characteristics of dual purpose laying hens. Poult Sci. (2020) 99:310–9. doi: 10.3382/ps/pez492
20. Knoop KA, Newberry RD. Goblet cells: multifaceted players in immunity at mucosal surfaces. Mucosal Immunol. (2018) 11:1551–7. doi: 10.1038/s41385-018-0039-y
21. Rauf A, Khalil AA, Rahman UU, Khalid A, Naz S, Shariati MA, et al. Recent advances in the therapeutic application of short-chain fatty acids (SCFAs): an updated review. Crit Rev Food Sci Nutr. (2021) 1−21. doi: 10.1080/10408398.2021.1895064
22. Lassenius MI, Fogarty CL, Blaut M, Haimila K, Riittinen L, Paju A, et al. Intestinal alkaline phosphatase at the crossroad of intestinal health and disease - a putative role in type 1 diabetes. J Intern Med. (2017) 281:586–600. doi: 10.1111/joim.12607
23. Abt MC, Artis D. The intestinal microbiota in health and disease: the influence of microbial products on immune cell homeostasis. Curr Opin Gastroenterol. (2009) 25:496–502. doi: 10.1097/MOG.0b013e328331b6b4
24. Wu C, Sartor RB, Huang K, Tonkonogy SL. Transient activation of mucosal effector immune responses by resident intestinal bacteria in normal hosts is regulated by interleukin-10 signalling. Immunology. (2016) 148:304–14. doi: 10.1111/imm.12612
25. Chen GY, Shaw MH, Redondo G, Nunez G. The innate immune receptor Nod1 protects the intestine from inflammation-induced tumorigenesis. Cancer Res. (2008) 68:10060–7. doi: 10.1158/0008-5472.CAN-08-2061
26. Mach N, Fuster-Botella D. Endurance exercise and gut microbiota: a review. J Sport Health Sci. (2017) 6:179–97. doi: 10.1016/j.jshs.2016.05.001
27. Schnupf P, Gaboriau-Routhiau V, Cerf-Bensussan N. Host interactions with Segmented Filamentous Bacteria: an unusual trade-off that drives the post-natal maturation of the gut immune system. Semin Immunol. (2013) 25:342–51. doi: 10.1016/j.smim.2013.09.001
28. Iweala OI, Nagler CR. The microbiome and food allergy. Annu Rev Immunol. (2019) 37:377–403. doi: 10.1146/annurev-immunol-042718-041621
29. Man AL, Gicheva N, Nicoletti C. The impact of ageing on the intestinal epithelial barrier and immune system. Cell Immunol. (2014) 289:112–8. doi: 10.1016/j.cellimm.2014.04.001
30. Pickard JM, Maurice CF, Kinnebrew MA, Abt MC, Schenten D, Golovkina TV, et al. Rapid fucosylation of intestinal epithelium sustains host-commensal symbiosis in sickness. Nature. (2014) 514:638–41. doi: 10.1038/nature13823
31. Keren DF, Brown JE, McDonald RA, Wassef JS. Secretory immunoglobulin A response to Shiga toxin in rabbits: kinetics of the initial mucosal immune response and inhibition of toxicity in vitro and in vivo. Infect Immun. (1989) 57:1885–9. doi: 10.1128/iai.57.7.1885-1889.1989
32. Boullier S, Tanguy M, Kadaoui KA, Caubet C, Sansonetti P, Corthesy B, et al. Secretory IgA-mediated neutralization of Shigella flexneri prevents intestinal tissue destruction by down-regulating inflammatory circuits. J Immunol. (2009) 183:5879–85. doi: 10.4049/jimmunol.0901838
33. Wang P, Li Y, Xiao H, Shi Y, Le GW, Sun J. Isolation of lactobacillus reuteri from Peyer's patches and their effects on sIgA production and gut microbiota diversity. Mol Nutr Food Res. (2016) 60:2020–30. doi: 10.1002/mnfr.201501065
34. Wu M, Xiao H, Liu G, Chen S, Tan B, Ren W, et al. Glutamine promotes intestinal SIgA secretion through intestinal microbiota and IL-13. Mol Nutr Food Res. (2016) 60:1637–48. doi: 10.1002/mnfr.201600026
35. Yang S, Yu M. Role of goblet cells in intestinal barrier and mucosal immunity. J Inflamm Res. (2021) 14:3171–83. doi: 10.2147/JIR.S318327
36. Ma X, Nakayamada S. Multi-source pathways of T Follicular helper cell differentiation. Front Immunol. (2021) 12:621105. doi: 10.3389/fimmu.2021.621105
37. Bartemes KR, Kephart GM, Fox SJ, Kita H. Enhanced innate type 2 immune response in peripheral blood from patients with asthma. J Allergy Clin Immunol. (2014) 134:671–8. doi: 10.1016/j.jaci.2014.06.024
38. Andoh A, Bamba S, Brittan M, Fujiyama Y, Wright NA. Role of intestinal subepithelial myofibroblasts in inflammation and regenerative response in the gut. Pharmacol Ther. (2007) 114:94–106. doi: 10.1016/j.pharmthera.2006.12.004
39. Monteleone G, Pender SL, Alstead E, Hauer AC, Lionetti P, McKenzie C, et al. Role of interferon alpha in promoting T helper cell type 1 responses in the small intestine in coeliac disease. Gut. (2001) 48:425–9. doi: 10.1136/gut.48.3.425
40. Shin MH, Kim J, Lim SA, Kim J, Kim SJ, Lee KM. NK Cell-based immunotherapies in cancer. Immune Netw. (2020) 20:e14. doi: 10.4110/in.2020.20.e14
41. Kucharzik T, Lugering N, Pauels HG, Domschke W, Stoll R. IL-4, IL-10 and IL-13 down-regulate monocyte-chemoattracting protein-1 (MCP-1) production in activated intestinal epithelial cells. Clin Exp Immunol. (1998) 111:152–7. doi: 10.1046/j.1365-2249.1998.00481.x
42. Jakobi V, Petry F. Humoral immune response in IL-12 and IFN-gamma deficient mice after infection with Cryptosporidium parvum. Parasite Immunol. (2008) 30:151–61. doi: 10.1111/j.1365-3024.2007.01013.x
43. Lee SK, Choi BK, Kim YH, Kang WJ, Kim KH, Sakaguchi S, et al. Glucocorticoid-induced tumour necrosis factor receptor family-related receptor signalling exacerbates hapten-induced colitis by CD4+ T cells. Immunology. (2006) 119:479–87. doi: 10.1111/j.1365-2567.2006.02459.x
44. Nagatake T, Hirata SI, Koga T, Kuroda E, Kobari S, Suzuki H, et al. BLT1 mediates commensal bacteria-dependent innate immune signals to enhance antigen-specific intestinal IgA responses. Mucosal Immunol. (2019) 12:1082–91. doi: 10.1038/s41385-019-0175-z
45. Carbone EA, D'Amato P, Vicchio G, De Fazio P, Segura-Garcia C. A systematic review on the role of microbiota in the pathogenesis and treatment of eating disorders. Eur Psychiatry. (2020) 64:e2. doi: 10.1192/j.eurpsy.2020.109
46. Zhu W, Zhou S, Liu J, McLean RJC, Chu W. Prebiotic, immuno-stimulating and gut microbiota-modulating effects of Lycium barbarum polysaccharide. Biomed Pharmacother. (2020) 121:109591. doi: 10.1016/j.biopha.2019.109591
47. Sack EL, van der Wielen PW, van der Kooij D. Polysaccharides and proteins added to flowing drinking water at microgram-per-liter levels promote the formation of biofilms predominated by bacteroidetes and proteobacteria. Appl Environ Microbiol. (2014) 80:2360–71. doi: 10.1128/AEM.04105-13
48. Geirnaert A, Wang J, Tinck M, Steyaert A, Van den Abbeele P, Eeckhaut V, et al. Interindividual differences in response to treatment with butyrate-producing Butyricicoccus pullicaecorum 25-3T studied in an in vitro gut model. FEMS Microbiol Ecol. (2015) 91:fiv054. doi: 10.1093/femsec/fiv054
49. Zhang Y, Liu Y, Li J, Xing T, Jiang Y, Zhang L, et al. Dietary resistant starch modifies the composition and function of caecal microbiota of broilers. J Sci Food Agric. (2020) 100:1274–84. doi: 10.1002/jsfa.10139
50. Yehia HM, Hassanein WA, Ibraheim SM. Purification and characterisation of the extracellular cholesterol oxidase enzyme from Enterococcus hirae. BMC Microbiol. (2015) 15:178. doi: 10.1186/s12866-015-0517-2
Keywords: glycyrrhiza polysaccharides, gut immunity, intestinal barrier, gut microbes, feed additives
Citation: Wu Y, Wu C, Che Y, Zhang T, Dai C, Nguyễn AD, Duan K, Huang Y, Li N, Zhou H, Wan X, Wang Y, Lei H, Hao P, Li C and Wu Y (2022) Effects of Glycyrrhiza Polysaccharides on Chickens' Intestinal Health and Homeostasis. Front. Vet. Sci. 9:891429. doi: 10.3389/fvets.2022.891429
Received: 07 March 2022; Accepted: 05 April 2022;
Published: 12 May 2022.
Edited by:
Fazul Nabi, Water and Marine Sciences, PakistanReviewed by:
Khalid Mehmood, Islamia University of Bahawalpur, PakistanCopyright © 2022 Wu, Wu, Che, Zhang, Dai, Nguyễn, Duan, Huang, Li, Zhou, Wan, Wang, Lei, Hao, Li and Wu. This is an open-access article distributed under the terms of the Creative Commons Attribution License (CC BY). The use, distribution or reproduction in other forums is permitted, provided the original author(s) and the copyright owner(s) are credited and that the original publication in this journal is cited, in accordance with accepted academic practice. No use, distribution or reproduction is permitted which does not comply with these terms.
*Correspondence: Yi Wu, wuyi2001cn@163.com; wuyi2001cn@njau.edu.cn
†These authors have contributed equally to this work
Disclaimer: All claims expressed in this article are solely those of the authors and do not necessarily represent those of their affiliated organizations, or those of the publisher, the editors and the reviewers. Any product that may be evaluated in this article or claim that may be made by its manufacturer is not guaranteed or endorsed by the publisher.
Research integrity at Frontiers
Learn more about the work of our research integrity team to safeguard the quality of each article we publish.