- 1Department of Veterinary Sciences, University of Torino, Grugliasco, Italy
- 2Department of Agricultural, Forest and Food Sciences, University of Torino, Grugliasco, Italy
The use of agro-industrial by-products in animal nutrition is a promising strategy to reduce the food-feed competition, the diet cost at farm level and the environmental impact of animal-derived food production. In this study, the suitability of cocoa bean shell (CBS), a by-product of the cocoa industry, as a feed ingredient in the diet of dairy goats was evaluated, with a focus on the related implications on feed intake, milk yield, milk main constituents, and fatty acid (FA) profile of milk fat. Twenty-two Camosciata delle Alpi goats were divided into two balanced groups. All the goats were fed mixed hay ad libitum. The control group (CTRL; n = 11) also received 1.20 kg/head × day of a commercial concentrate, while in the experimental group (CBS; n = 11) 200 g of the CTRL concentrate were replaced by the same amount of pelleted CBS. The total dry matter intake of the goats was reduced by the dietary inclusion of CBS (P ≤ 0.01). The milk yield, as well as the milk fat, protein, and casein contents and yields were unaffected by the treatment. Milk from the CBS-fed goats showed decreased urea content when compared to the CTRL group (P ≤ 0.001). Milk from the CBS group of goats also showed increased concentrations of total branched-chain FA (both iso and anteiso forms; P ≤ 0.001) and total monounsaturated FA (P ≤ 0.05), as well as a decreased ∑ n6/∑ n3 FA ratio (P ≤ 0.05). De novo saturated FA, total polyunsaturated FA, total conjugated linoleic acids, and the majority of ruminal biohydrogenation intermediates remained unaffected by the dietary treatment. These results suggest that CBS can be strategically used as an alternative non-conventional raw material in diets intended for lactating goats, with no detrimental effects on their milk production performance. The use of CBS in goat nutrition may be hindered by the presence of theobromine, a toxic alkaloid. Special attention is needed by nutritionists to avoid exceeding the theobromine limits imposed by the current legislation. Detheobromination treatments are also suggested in literature to prevent toxic phenomena.
Introduction
The world population is estimated to reach 9.7 billion in 2050 (1). Promoting a more efficient and sustainable use of the available resources has become a priority to satisfy the increasing global food demand (2). Agro-industrial by-products are addressed as innovative raw materials to be used in feed formulations for livestock animals to reduce both the human-animal competition for food supply and the animal feeding costs (3).
Cocoa beans (CB) are the seeds of the cocoa tree (Theobroma cacao L., botanical family: Sterculiaceae). They are composed of an outer shell, two cotyledons and a small germ (4). In 2018/2019, the total worldwide CB production was approximately equal to 4.8 million tons (5), with a forecast of 5% growth for the 2020/2021 period. Africa is the leader cocoa producer worldwide, covering the 76% of total production. Significant contributions to the world cocoa trade are also given by Americas, Indonesia, and Papua New Guinea (18.0, 4.6, and 0.6% of the total production, respectively) (5).
Cocoa beans are destined to the confectionery industry to produce chocolate and other cocoa-derivative foods (e.g., cocoa paste, butter, and liquor) that are largely consumed in the most developed countries, such as Europe and North America (6). Only the 10% of the total weight of the fruit is commercialized and, consequently, different by-products are originated (7). After the harvesting and post-harvesting processes, the removal of CB causes the discarding of the cocoa pod husk, a first by-product representing the 70–75% of the cocoa fruit weight, and of the cocoa mucilage, a white mass surrounding the CB (6, 8). During the winnowing and roasting processes, the cocoa bean shell (CBS), which represents about the 10–17% of the total bean weight (9), is removed from the seeds (4, 7). It can be estimated that about 900,000 tons of CBS waste are produced annually worldwide (10). On a dry matter (DM) basis, the CBS contains 10.3–27.4% of protein, 39.3–66.3% of fiber, and 1.5–8.5% of fat (7). The CBS is also a natural source of bioactive compounds, like polyphenols (total phenolic content ranging from 3.1 to 95.0 mg of gallic acid equivalents/g of dried CBS) (7), with antioxidant and antiradical properties (4, 11).
To reduce waste and maximize the principles of Circular Economy (12), recent studies have been carried out to evaluate the potential uses of CBS. For food purposes, CBS has been mainly proposed as a low-cost cocoa substitute (7), that is as ingredient or additive in baked products (13–15). CBS has also been successfully used to produce alkalized fiber powder (16). In addition, polyphenols extracted from CBS were shown to improve the quality parameters of cooking oils (17) and to reduce lipid oxidation of cooked-refrigerated beef (18). Besides food applications, CBS can also be employed for biofuel production, thanks to its high calorific value (19). Studies were published showing the possibility to produce biomethane (20) and biogas from CBS (21). Thanks to its physical properties (22), antioxidant and biodegradable characteristics, CBS has been also used in food packaging (23, 24), cosmetics and biomedical applications (25), farm bedding material (26), and as fertilizer (7).
In recent years, CBS has also been targeting the livestock sector as a feedstuff (27). The main limitation in the use of CBS as feed ingredient is the presence of theobromine (3,7-dimethylxanthine), an alkaloid prone to cause toxicity and feed intake reduction at high dietary concentrations (28), the levels of which can be decreased by means of hot water, alkali, microbial, or enzymatic detheobromination treatments (28–30). The European Food Safety Authority established a maximum level of theobromine in animal feed equal to 300 mg/kg (31). Currently, exceptions are provided for pigs (200 mg/kg), and for dogs, rabbits, horses, and fur animals (50 mg/kg) (32). Ogunsipe et al. (33) replaced up to 40% maize with CBS in the diet of weaner pigs, showing no deleterious effects on live weight gain and feed conversion at inclusion levels lower than 20 and 10%, respectively. Adeyina et al. (30) and Ayinde et al. (34) demonstrated that untreated CBS and hot water treated CBS can be included in the diet of weaner rabbits at 100 and 200 g/kg, respectively, with no negative effects on animal performance and physiological responses, with the additional benefit of increased farm profitability (34). Studies on poultry nutrition showed that hot water treated CBS and enzyme treated CBS can replace maize up to 20 and 10% in the diet of commercial layers, respectively, without any detrimental effects on the performance, hematology, and egg quality parameters (29, 35). In the aquaculture sector, Bamba et al. (36) demonstrated that CBS, when used in combination with a mixture of plant proteins, is a suitable feed for Nile tilapia, being able to improve the weight gain and the feed conversion ratio of the fish. As far as ruminants are concerned, Amin and Cahyono (37) showed increased daily body weight gain and feed efficiency in young male Bali cattle fed king grass and a protein supplement consisting of 40% cocoa bean waste (a mixture of bean skin, pulp, and placenta) plus 60% rice bran compared to a supplement consisting of 100% rice bran. When included at about 12% in diets for lactating ewes to partially replace corn and barley, CBS did not modify milk yield, milk fat, and protein contents, while it reduced the milk urea content and improved the health indexes of milk fat (38).
No information is currently available on the suitability of CBS as feed ingredient in the diet of dairy goats. Therefore, this study aimed at providing novel information on the effects of the dietary inclusion of CBS on feed intake, milk yield, milk main constituents, and fatty acid (FA) profile of milk fat from lactating goats.
Materials and Methods
The experimental protocol was designed according to the guidelines of the current European Directive 2010/63/EU on the protection of animals used for scientific purposes. All procedures and treatments were in compliance with the European Directive (Council Directive 98/58/EC) on the minimum standards for the protection of animals bred or kept for farming purposes.
Animals and Dietary Treatments
The experiment was carried out in a commercial farm located in None (Turin province, Piedmont, NW Italy, latitude: 44°91′59″ N; longitude: 07°52′57″ E; altitude: 270 m a.s.l.) from May 14 to June 13, 2019. Twenty-two pluriparous Camosciata delle Alpi goats in mid-lactation were selected from a flock of 60 lactating goats and allocated to two balanced groups of 11 animals each, according to their parity (3.9 ± 1.07), stage of lactation (106 ± 4.6 days in milk), milk yield (1.8 ± 0.06 kg/head × day), milk gross composition (fat: 33.8 ± 0.43 g/kg and protein: 32.3 ± 0.42 g/kg) and FA profile of milk fat. The groups were then randomly assigned to a control or an experimental diet, with 55:45 forage to concentrate (F:C) ratio on a DM basis. The experimental diets were formulated following NRC recommendations (39). The control group (CTRL) was fed mixed hay ad libitum and 1.20 kg/head × day of a pelleted commercial concentrate containing corn (34.0%), partially dehulled sunflower meal (15.0%), dehulled soybean meal (12.0%), wheat bran (11.0%), barley (10.0%), soybean hulls (4.0%), roasted soybean (4.0%), dehydrated alfalfa (3.0%), cane molasses (2.5%), calcium carbonate (2.0%), sodium chloride (1.0%), precipitated dicalcium phosphate dihydrate (0.5%), sodium bicarbonate (0.3%), magnesium oxide (0.2%), and mineral-vitamin premix (0.5%). The concentrate was administered in equal amounts during the milking, at 6:30 a.m. and 6:00 p.m. The other group (CBS group, Cocoa Bean Shell) received the same diet but, during the afternoon milking, 200 g of concentrate were replaced by pelleted CBS (which approximately corresponded to the 17% of the daily administered concentrate). The dietary CBS inclusion level was chosen such as to fall within the maximum threshold imposed by the current European regulation on the presence of theobromine in animal feeds (32), in the meantime allowing to formulate isonitrogenous and isoenergetic experimental diets. The goats received increasing quantity of CBS (diet adaptation) starting 10 days before the beginning of the trial: 50 g/head in the 1st day, 100 g in the next 2 days, then 150 g in other 2 days and 200 g/head × day from the 6th day to the end of the trial.
All the selected goats were housed indoors in individual pens and had free access to clean and fresh water.
Feed Intake, Sampling, and Analysis
Feed refusals were weighed daily to estimate feed intake. Representative samples of hay, concentrate and CBS used in the trial were collected at the beginning of the adaptation period. They were ground with a cutting mill to pass a 1 mm screen sieve (Pulverisette 15-Fritsch GmbH, Idar-Oberstein, Germany). AOAC International procedures (40) were used to determine DM (method no. 930.15), ash (method no. 942.05), crude protein (CP, method no. 984.13), acid detergent fiber and acid detergent lignin (ADFom and ADL, method no. 973.18). Ether extract (EE, method no. 2003.05) was analyzed according to AOAC International (41). Neutral detergent fiber (aNDFom) was analyzed according to Mertens (42); α-amylase (Merck, Darmstadt, Germany) and sodium sulphite (Merck, Darmstadt, Germany) were added, and results were corrected for residual ash content.
Rumen degradable protein (RDP) was analyzed according to Licitra et al. (43).
The energetic value of feeds was expressed as net energy for lactation (NEL) and was estimated using National Research Council equations (39).
The FA composition of hay, concentrate and CBS was assessed using a combined direct transesterification and solid-phase extraction method as reported in Dabbou et al. (44). Separation, identification, and quantification of fatty acid methyl esters (FAME) were performed as described by Renna et al. (45). The results were expressed as g/kg DM. The daily intake (g/head) of each individual FA and groups of FA from the diet was estimated considering the daily intake and the analytically determined FA composition of each feedstuff.
The contents of total extractable phenols (TEP) and different polyphenol fractions (non-tannin phenols, NTP; condensed tannins, CT) in CBS, were assessed as detailed in Iussig et al. (46). Briefly, polyphenols were extracted twice with aqueous acetone (70:30 v/v) and subjected to ultrasonic treatment for 20 min at room temperature in an ultrasonic water bath (Bransonic-21, Branson Ultrasonics, Danbury, CT, USA). Polyvinyl-polypyrrolidone was used to separate NTP and total tannins (TT), according to a modified Folin-Ciocalteu method. The butanol-HCl-iron method was used to determine CT. The absorbance was recorded at 725 nm (TEP and NTP, expressed as gallic acid equivalents) and 550 nm (CT, expressed as leucocyanidin equivalents) using a UV–vis spectrophotometer (Shimadzu UVmini-1240, Shimadzu Corporation, Kyoto, Japan). Total tannins (TT) were computed as the difference between TEP and NTP. Hydrolysable tannins (HT) were estimated as the difference between TT and CT (47). The amounts of phenolic compounds daily ingested by the goats belonging to the CBS group was estimated based on the analyzed phenolic composition of CBS and the determined intake of concentrate.
All the analyses were performed in duplicate.
Milk Sampling and Analysis
The goats were milked twice a day (at 6:30 a.m. and 6:00 p.m.) in a 12-unit parallel milking parlor (Milkline®, Piacenza, Italy) with 43 kPa vacuum level combined with 90 cycles/min of pulsation rate. Individual milk yield was recorded and individual composite samples (n = 88) of morning and afternoon milking (proportional to milk production recorded per milking) were collected at 0, 10, 20, and 30 days after the beginning of the trial. Each milk sample was divided into two aliquots, immediately stored at 4°C in a portable refrigerator and transported to the laboratory. The first aliquot (50 mL) was analyzed for fat, protein, lactose, casein, and urea (MilkoScan FT 6000, Foss Electric, Hillerød, Denmark; ISO 9622:2013/IDF 141:2013) and somatic cell count (SCC) (Fossomatic FC, Foss Electric, Hillerød, Denmark; UNI EN ISO 13366-2:2007). The second aliquot (150 mL) was frozen at −80°C and successively analyzed for the FA composition. Milk fat extraction was obtained by centrifugation at 7,300 rpm for 30 min at −4°C. The resulting molten butter was filtered through a hydrophobic filter (Whatman 1, Whatman International Ltd., Maidstone, England). The pure milk fat was then dissolved in 5 mL of internal standard solution (nonanoic acid in heptane) and FAME were obtained by transesterification of glycerides by using a solution of potassium hydroxide in methanol, as reported in Cornale et al. (48). FAME were analyzed by high-resolution gas chromatography (Shimadzu GC 2010 Plus, Shimadzu Corporation Analytical Instruments Division, Kyoto, Japan) equipped with a flame ionization detector. As reported in Kramer et al. (49), FAME were separated on a CP-Sil 88 fused capillary column (100 m × 0.25 mm ID, 0.20 μm film thickness; Varian Inc., Lake Forest, CA, USA). The temperatures of both injector and detector were maintained at 250°C. The temperature program was: 45°C for 4 min, raised to 175°C at 13°C/min for 27 min, then to 215°C at 4°C/min for 35 min. Carrier gas was used at a flow rate of 1 ml/min. The injection volume was 0.1 μL. Peaks were identified by comparing their retention times with pure FAME standards (Matreya Inc., Pleasant Gap, PA, USA and Restek Corporation, Bellefonte, PA, USA) and by comparison with published chromatograms (49).
Energy-corrected milk (ECM) was calculated for each goat using the following formula (50):
Feed efficiency (FE) is defined as a unit of milk produced per unit of DM consumed and was calculated as reported in Renna et al. (51) as the ratio between ECM (kg) and dry matter intake (DMI, kg).
The activity of the enzyme Stearoyl-CoA desaturase (SCD; EC 1.14.19.1) was estimated by the calculation of different desaturase indexes: DI14 (C14:1 c9/C14:0), DI16 (C16:1 c9/C16:0) and DI18 (C18:1 c9/C18:0).
All the analyses were performed in duplicate.
Statistical Analysis
The changes in DMI, milk yield, milk composition, and FE were analyzed using the MIXED procedure of SAS v. 9.4 (SAS Inst. Inc, Cary, NC) for repeated measures over time. The goat was considered as the experimental unit. Compound symmetry, first order autoregressive or unstructured covariance structure, according to the smallest Schwarz Bayesian information criterion, was applied (52). The following model was used:
where: Yijk= mean of response variable, μ= population mean, DTi = fixed effect of dietary treatment, G(i)j = random effect of goat within the treatments, STk= fixed effect of sampling time, (DT × ST)jk= fixed effect of interaction between dietary treatment and sampling time, and εijk= experimental error. Significance was declared at P ≤ 0.05. The results of the statistical analysis are reported as estimate least-squares means.
Results
Chemical Composition of Cocoa Bean Shell and Experimental Diets
The proximate and FA compositions of the feedstuffs and of the experimental diets are shown in Table 1. The CBS used in this trial showed high CP (173 g/kg DM) and fiber (aNDFom: 495 g/kg DM; ADFom: 426.4 g/kg DM) concentrations, while the EE concentration was relatively low (60.6 g/kg DM). The NEL of the by-product was equal to 4.27 MJ/kg DM. The experimental diets were almost isonitrogenous (143 and 141 g/kg DM for the CTRL and CBS diets, respectively) and isoenergetic (5.31 and 5.15 MJ/kg DM for the CTRL and CBS diets, respectively).
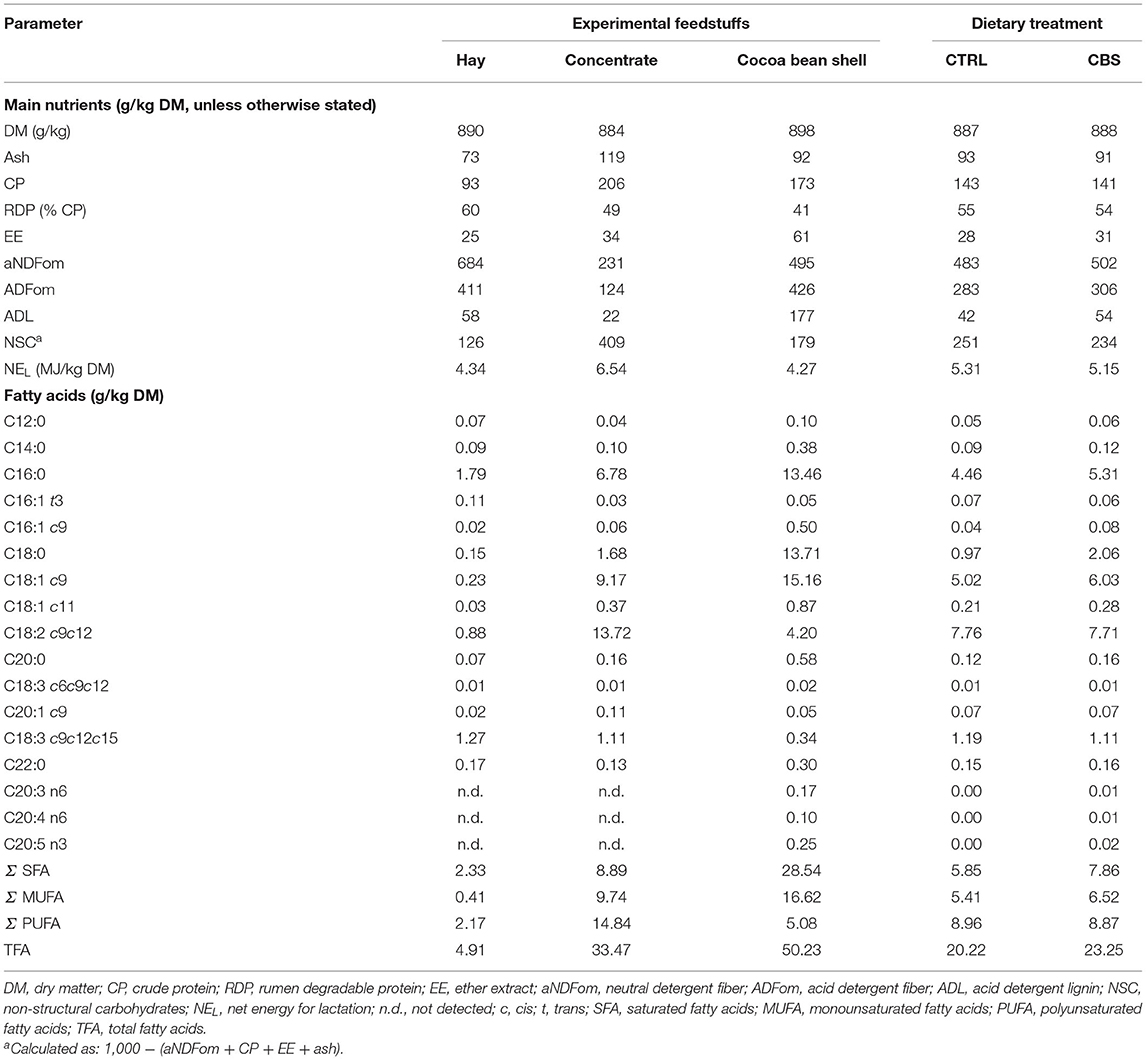
Table 1. Proximate composition (g/kg DM, unless otherwise stated) and fatty acid profile (g/kg DM) of the experimental feedstuffs.
As far as the FA composition is concerned, CBS showed a prevalence of saturated fatty acids [SFA; 28.54 g/kg DM, corresponding to the 56.8% of total detected FA (TFA)] and of monounsaturated fatty acids (MUFA; 16.62 g/kg DM, corresponding to the 33.1% of TFA). Palmitic (C16:0) and stearic (C18:0) acids were the most abundant SFA in CBS, showing approximately the same concentration (13.46 and 13.71 g/kg DM, respectively) in the by-product (representing the 47.2 and 48.0% of total SFA and 26.8 and 27.3% of TFA, respectively). Oleic acid (C18:1 c9) was the most abundant individual FA in CBS (representing the 91.2% of total MUFA and 30.2% of TFA). Polyunsaturated fatty acids (PUFA) only represented a minor component of CBS (5.08 g/kg DM), comprising the 10.1% of TFA. Linoleic acid (C18:2 c9c12) was the most abundant PUFA in the by-product, accounting for the 8.4% of TFA (82.7% of total PUFA). Alpha-linolenic acid (C18:3 c9c12c15) was only detected in very low amounts in CBS (6.7% of PUFA and 0.7% of TFA). The TFA content of the experimental diets was higher for the CBS when compared to the CTRL diet (23.25 and 20.22 g/kg DM, respectively). However, the diets were very similar in terms of their FA composition, with the CBS diet showing a slightly higher concentration of total SFA (7.86 vs. 5.85 g/kg DM, corresponding to 33.8 and 28.9% of TFA) and total MUFA (6.52 vs. 5.41 g/kg DM, corresponding to 28.0 and 26.8% of TFA) and a very similar concentration of total PUFA (8.87 vs. 8.96 g/kg DM, corresponding to 38.2 and 44.3% of TFA) when compared to the CTRL diet.
Regarding phenols (data not reported in the table), the recorded amount of TEP in CBS was low (9.12 g/kg DM). More than the 96% of TEP was represented by tannins (TT equal to 8.76 g/kg DM), almost equally represented by hydrolysable (HT) and condensed (CT) forms (55.1 and 44.9% of TT, respectively). Polyphenols were not determined in mixed hay and CTRL concentrate, as their amounts were expected to be negligible.
Intake of Dry Matter, Fatty Acids, and Phenolic Compounds
The effects of the dietary inclusion of CBS on DM and FA intake by the goats are shown in Table 2. The dietary treatment had no significant effect on the individual intake of concentrate, which was always totally ingested by the goats in both experimental groups. However, a significant decrease of the individual intake of hay was observed with the inclusion of CBS in the diet (1.22 kg/head × day and 1.11 kg/head × day for the CTRL and CBS diets, respectively; P ≤ 0.001). Consequently, the individual total DMI of the goats was significantly reduced with the dietary inclusion of CBS (−5%; P ≤ 0.01).
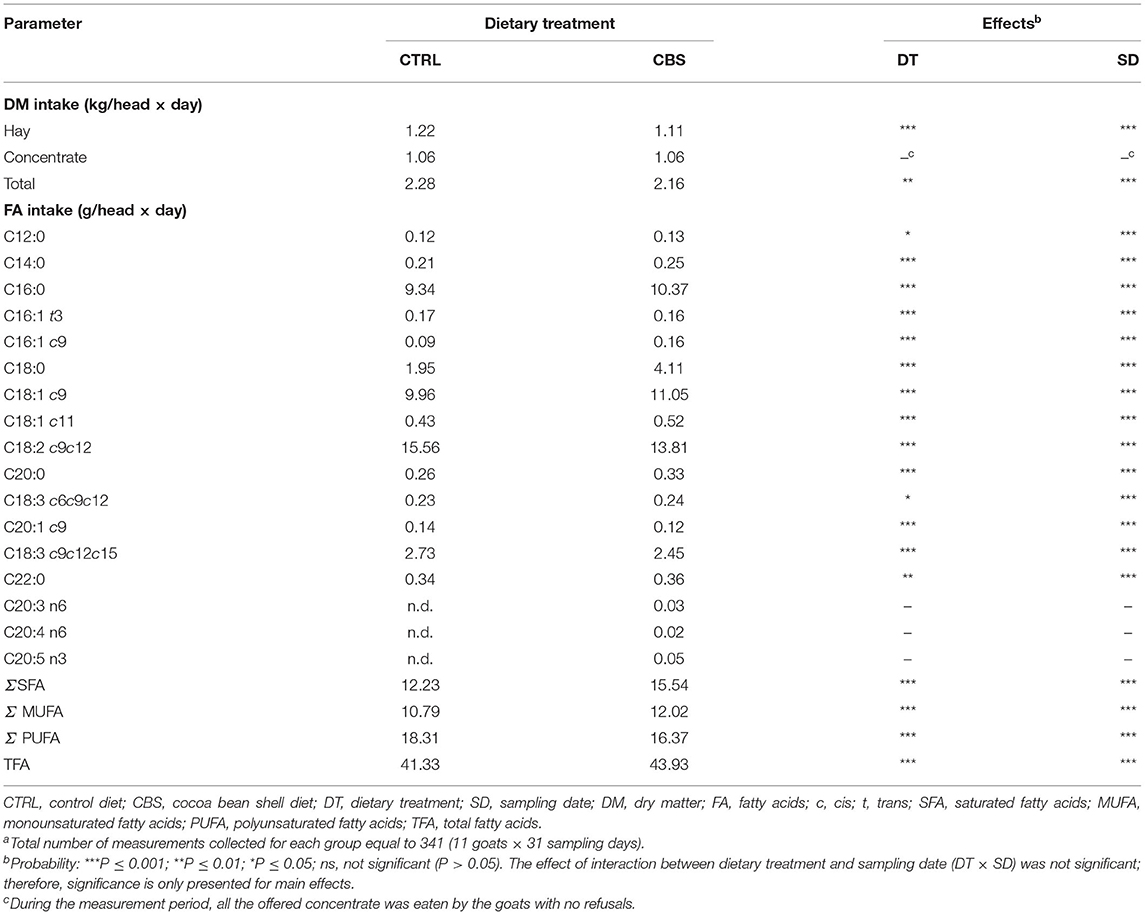
Table 2. Dry matter and main fatty acids intakes of goats fed the control (CTRL) and cocoa bean shell (CBS) dietsa.
Table 2 also shows the differences between the two groups of goats for the intake of all individual FA and groups of FA from the diets. In particular, the CBS group of goats showed a higher intake of palmitic acid (+ 1.03 g/head × day; P ≤ 0.001), stearic acid (+ 2.16 g/head × day; P ≤ 0.001) and oleic acid (+ 1.09 g/head × day; P ≤ 0.001), as well as contemporarily lower intakes of linoleic acid (−1.75 g/head × day; P ≤ 0.001) and α-linolenic acid (−0.28 g/head × day; P ≤ 0.001) when compared to the CTRL group. Overall, the CBS-fed goats ingested higher amounts of total SFA and total MUFA, and lower amounts of total PUFA, that the CTRL-fed goats (in all cases, P ≤ 0.001).
The amounts of TEP, NTP, TT, CT, and HT daily ingested by the goats belonging to the CBS group were equal to 1.64, 0.06, 1.57, 0.61, and 0.87 g/head × day, respectively.
The DT × SD interaction was not significant for the considered parameters.
Milk Yield and Milk Main Constituents
The effects of the dietary inclusion of CBS on milk yield, ECM, milk fat, protein, casein and lactose contents and yields, urea content, SCC, and FE are shown in Table 3. The individual milk yield and ECM were not significantly affected by the treatment. Regarding the main milk constituents, both the content (−3.9%; P < 0.001) and yield (−8.8%; 0.05 < P ≤ 0.10) of lactose as well as the urea content (−20.7%; P < 0.001) were reduced or tended to be reduced with the inclusion of CBS in the diet. The other macro nutrients in milk (fat, protein, and casein), as well as SCC and FE showed no differences while comparing the experimental groups.
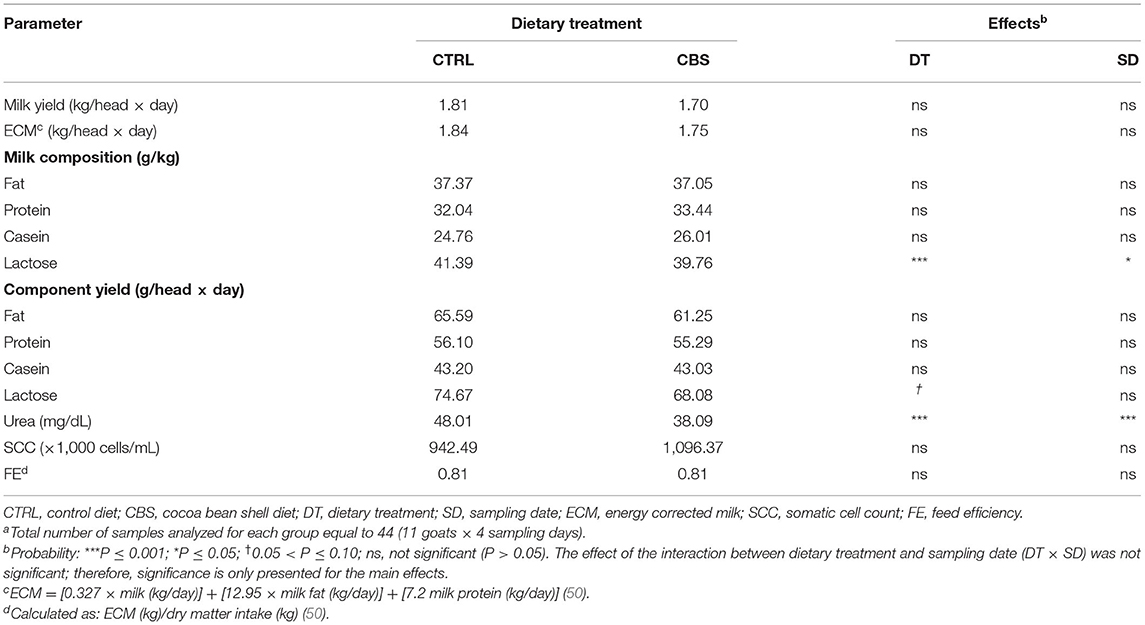
Table 3. Milk yield, milk main constituents, and somatic cell count of milk from goats fed the control (CTRL) and cocoa bean shell (CBS) dietsa.
The DT × SD interaction was not significant for the considered parameters.
Milk Fatty Acid Profile
The inclusion of CBS in the diet significantly affected some individual FA and groups of FA in goat milk fat (Tables 4–6). The concentration of the majority of individual SFA with a linear carbon chain, as well as total SFA, did not significantly vary between the experimental groups, with few exceptions (Table 4). The latter regarded heptanoic acid (C7:0), which was negatively affected by the inclusion of CBS in the goat diet (P ≤ 0.01), and some long chain SFA, such as C18:0, eicosanoic acid (C20:0) and behenic acid (C22:0), which showed significantly higher concentrations in the milk from CBS when compared to the milk from the CTRL group (P ≤ 0.05, P ≤ 0.001, and P ≤ 0.001, respectively). Considering SFA, most changes were instead detected in the milk concentrations of branched-chain fatty acids (BCFA), both iso and anteiso form (Table 4). Total BCFA, total iso BCFA and total anteiso BCFA were, in fact, significantly enhanced (by 10.9, 10.0, and 13.8%, respectively) in the milk from CBS goats when compared to the CTRL group (P ≤ 0.001). Considering individual BCFA, significant increases in CBS milk were observed for C14 iso, C15 iso, C16 iso, C15 anteiso and C17 anteiso; C13 iso showed a tendency toward higher concentrations in CBS than CTRL milk, while C13 anteiso, C17 iso, and C18 iso remained unaffected by the dietary treatment.
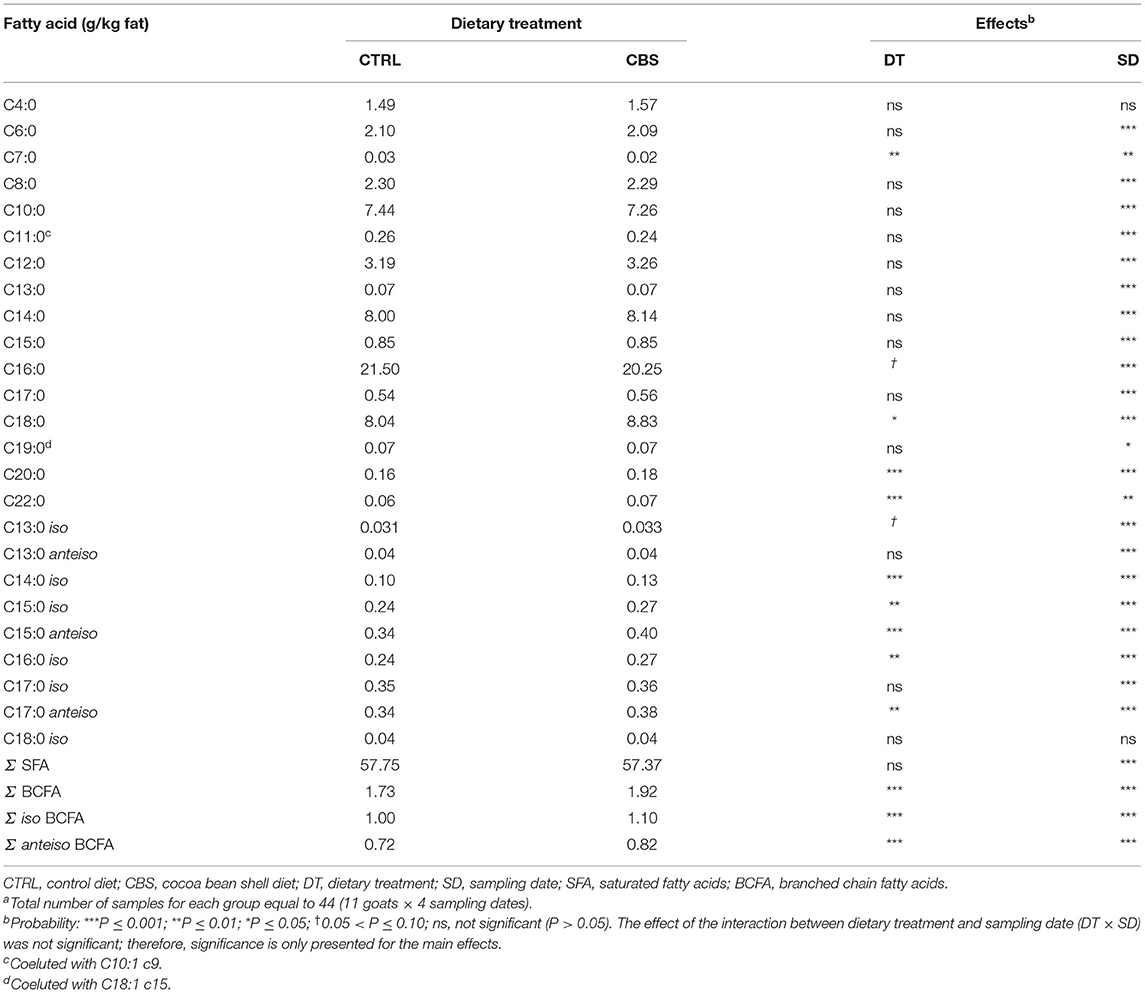
Table 4. Saturated and branched-chain fatty acids (g/kg fat) in milk of goats fed the control (CTRL) and cocoa bean shell (CBS) dietsa.
Differently from what observed for the total SFA, the concentration of the total MUFA significantly increased (+5.1%; P < 0.05) in the milk from CBS-fed goats when compared to the milk from CTRL-fed goats (Table 5). The concentration of total octadecenoic acids tended to increase significantly with the inclusion of CBS in the goat diet (P = 0.08) while the total trans-octadecenoic acids remained unaffected. Significant increases were observed for the concentrations of oleic acid (that with the applied chromatographic conditions coeluted with C18:1 c10 and C18:1 t15, + 5.1%; P ≤ 0.05) and other individual octadecenoic isomers (i.e., C18:1 c11 and C18:1 c14, + 7.1% P ≤ 0.05 and + 16.7% P ≤ 0.01, respectively). Significant increases were also recorded for the concentrations of C16:1 c13, C17:1 t9 and C20:1 t6-11, while C16:1 t4 decreased with the inclusion of CBS in the diet. The other individual detected MUFA were not affected by the treatment. The SCD activity, as estimated by DI14, DI16, and DI18, did not vary between the treatments.
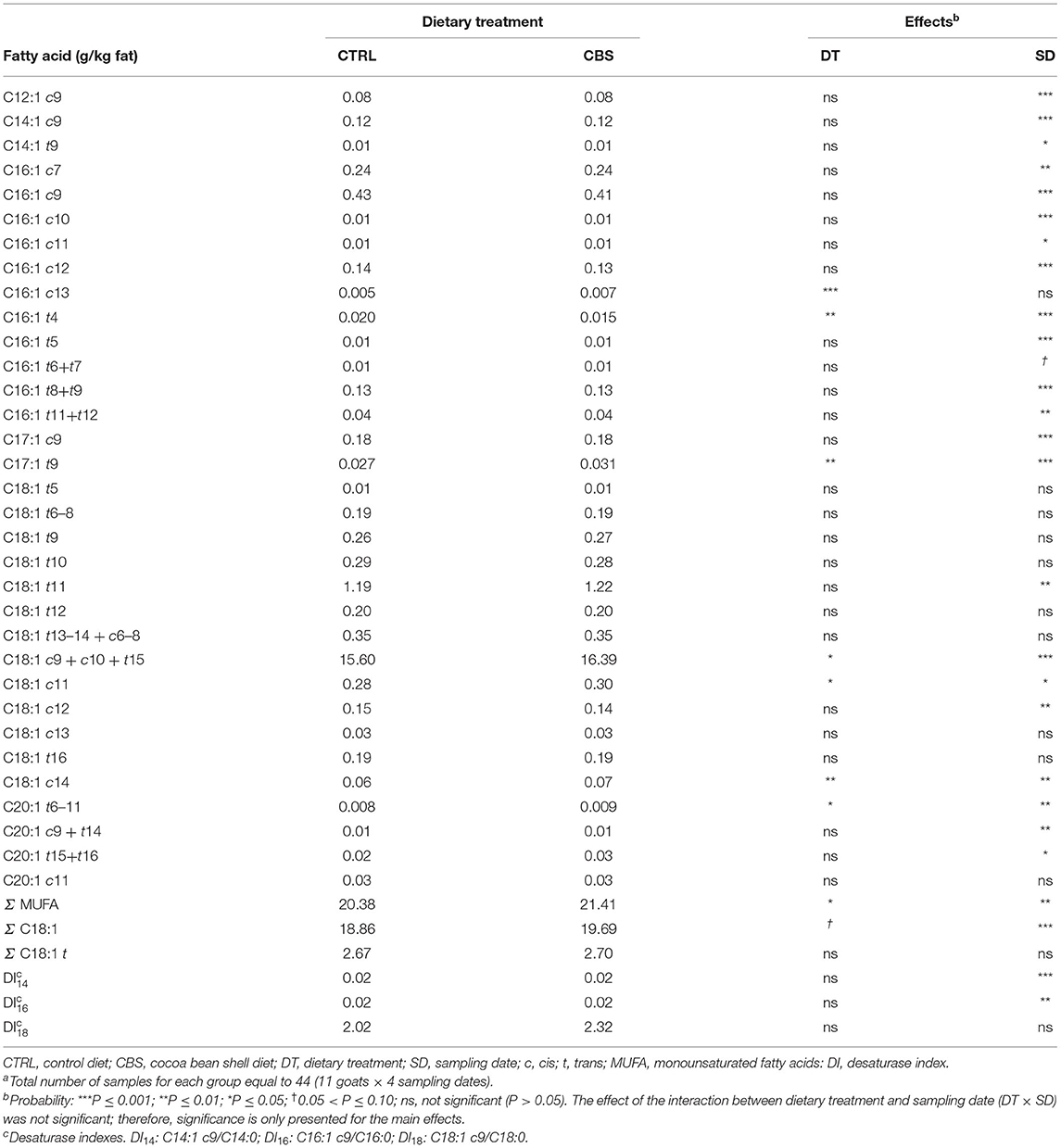
Table 5. Monounsaturated fatty acids (g/kg fat) in milk of goats fed the control (CTRL) and cocoa bean shell (CBS) dietsa.
Very few changes were observed when considering milk PUFA (Table 6). No differences were found for total PUFA, total octadecadienoic acids, total trans-octadecadienoic acids, total conjugated linoleic acid (CLA), and total n6 FA between the experimental groups. The total n3 FA tended to be higher in the milk from CBS-fed goats (P = 0.06), which led to a significant lower n6/n3 FA ratio in the milk (P ≤ 0.05). The majority of individual PUFA remained unaffected by the dietary treatment. Only C18:2 t11c15 and C18:2 t9c11 (which coeluted with C21:0) showed significantly higher concentration in the CBS when comparted to the CTRL group. Linoleic acid tended to be negatively affected by the inclusion of CBS in the goat diet (−4.7%, P = 0.09).
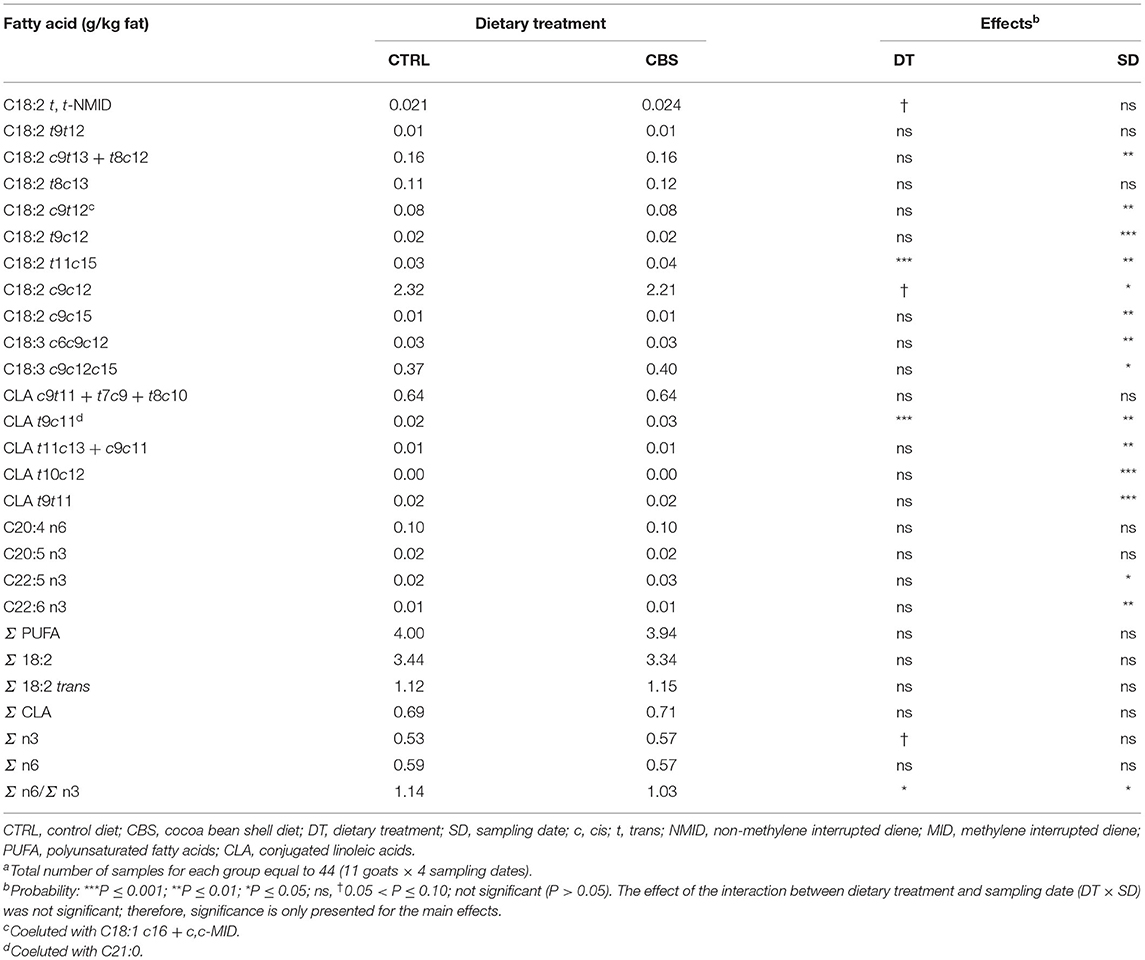
Table 6. Polyunsaturated fatty acids (g/kg fat) in milk of goats fed the control (CTRL) and cocoa bean shell (CBS) dietsa.
The sampling date significantly affected many of the detected individual FA and groups of FA.
The DT × SD interaction was not significant for the considered parameters.
Discussion
Chemical Composition of Cocoa Bean Shell
In accordance with the results of published literature, the major component in CBS was fiber (aNDFom represented almost 500 g/kg DM) (25, 53). As most of the lipids are removed during the industrial process for cocoa butter production, EE is only a minor component of CBS, showing much lower concentration particularly when compared to the EE content of CB (about 50% DM) (7). The CP content of the CBS used in this trial fell within the ranges reported in the literature [10.3 g/100 g to 27.4 g/100 g of the CBS dried weight (7)]. Despite the good CP amount of CBS, almost the 44% of total protein is composed of fraction C according to the Cornell Net Carbohydrate and Protein System (CNCPS) protein fractionation scheme (38). This fraction is represented by protein associated with lignin, tannin-protein complexes, and Maillard products, being characterized by null ruminal degradation rates and intestinal digestibility (54), thus limiting the potential application of CBS as alternative protein source in animal nutrition. High relative proportions of protein fraction C were also reported for other by-products of the agro-industry or biodiesel production chain with potential application in feed formulations, such as the hazelnut skin (55), custard apple and soursop seed cakes (56), and peanut cake (57).
Concerning the FA composition of CBS, few data are currently available in the literature. A detailed FA profile was recently reported by Botella-Martínez et al. (10) for Ghanaian CBS flours of different particle size. The obtained results confirm a prevalence of SFA in CBS, especially stearic and palmitic acids with approximately equal concentrations, followed by MUFA, with a clear prevalence of oleic acid. Similar findings were also reported by Lessa et al. (58) and Soares et al. (59) for Brazilian roasted CBS and CBS fat extract, respectively.
Cocoa bean shell is a good source of phenolic compounds, which are responsible for its bio-functional properties. Several authors investigated the polyphenolic fraction of CBS, and high variability has been shown (i.e., 6.04–94.95 mg of gallic acid equivalents and 1.70–25.30 mg of catechin equivalents/g of dried CBS for total phenols and total tannins, respectively) according to the polyphenolic extraction conditions (7, 53). Because of the use of different extraction methods and/or different units of measure used for the expressions of the obtained results, it is difficult to compare the obtained results with those obtained by other authors. However, with comparable analytical methods used in the current trial, Campione et al. (38) found amounts of TEP in CBS equal to 43.9 g/kg DM, about the 70% of which were tannins. Such values are about 4.8 times higher than those found for the CBS used in the current trial. However, it has been demonstrated that various factors concur to affect the polyphenolic concentration of CBS, such as plant genotype, geographical origin, harvest season, stress conditions for the plant and, finally, cocoa manufacturing processes, the latter being able to cause polyphenol degradation (7). The presence of polyphenolic compounds is typical of many agro-industrial by-products. The TEP concentration of CBS is lower if compared to that of other by-products shown to be potentially used as raw materials in feed formulations, such as hazelnut skin (265 g/kg DM) (51) or pistachio hulls (103.0 g/kg DM) (60).
The theobromine content of the CBS used in this trial (4.3 g/kg DM) is in line with lower-range values reported in the literature for this by-product (31) and slightly lower that the theobromine content of the CBS (7.2 g/kg DM) or cocoa pod husk (6.9 g/kg DM) tested for their suitability in sheep nutrition by Campione et al. (38) and Carta et al. (61), respectively. The above-mentioned theobromine levels are also lower than those reported for hot water treated CBS by other Authors (30).
Dry Matter Intake, Milk Yield, and Milk Main Constituents
The inclusion of CBS in the diet of the goats negatively impaired their total DMI (Table 2). Lots of agro-industrial by-products are characterized by low overall nutritional quality; in addition, some of them contain antinutritional factors that negatively affect palatability (3). However, in the current study, the reduction of the DMI only regarded the roughage part of the diet, as the concentrate was always fully eaten by the animals of both experimental groups (Table 2). This demonstrates that a CBS inclusion level of 17% in the concentrate feed does not cause palatability issues in the caprine species. In cattle and sheep, dietary tannins can create complexes with lignocellulose, inhibiting cellulolytic microorganisms and fibrolytic enzymatic activity, and increasing rumen physical filling, with negative consequences on voluntary DMI and fiber digestion (62, 63). However, goats and other browsing animal species are able to produce tannin-binding saliva and stimulate the proliferation of tannin-tolerant bacteria, mostly overcoming the negative effects of tannins on intake and nutrient digestion (64, 65). da Silva et al. (66) recently demonstrated that a dietary concentration of CT equal to 5% DM did not affect the DMI and nutrient intake of goats from different genotypes. Concerning voluntary DMI, no adverse effects are expected in goats fed diets containing up to 10% DM of CT (65). Having this in mind and given the relatively low concentration of tannins in the CBS used in the current trial trial (both TT and CT lower than 0.1% DM for the CBS diet), it is unlikely that the reduction of the voluntary DMI in the CBS-fed goats may be imputed to the dietary tannin concentration. As a confirmation for such a hypothesis, ewes fed alfalfa hay ad libitum and 800 g of conventional or 11.7% CBS-containing concentrate (dietary TT equal to 0.4% DM) showed no significant alterations of their voluntary DMI (38). However, when feeding growing goats (18–20 months of age) with a diet containing up to 50% CBS or cocoa dust, Aregheore (67) observed in both cases a reduction of the voluntary DMI by the animals, which consequently led to a lower body weight gain, when compared to growing goats of comparable age and initial body weight gain fed dried brewers' grain as the control group. The author attributed the lower production performance of the goats fed CBS and cocoa dust to the presence of theobromine in the cocoa by-products. Unfortunately, Aregheore (67) did not report the theobromine level of the CBS. However, considering an average theobromine concentration in the CBS equal to 13 g/kg, it can be estimated that the theobromine intake in Aregheore (67) was 6.9 g/growing goat per day, corresponding to 290 mg/kg body weight per day (31). As the dietary inclusion level of CBS was much lower in the present study, the theobromine intake was also much lower, corresponding to an average value of about 1.51 g/head × day. From these considerations, an issue arises about the identification of the maximum level of ingested theobromine capable of negatively influence the voluntary DMI in adult goats. Further studies are necessary to clarify this issue.
In this study, milk yield as well as the contents and yields of milk fat, protein and casein were not affected by the dietary inclusion of CBS, confirming the results obtained in CBS-fed and cocoa husks-fed ewes by Campione et al. (38) and Carta et al. (61), respectively. Consistently, Correddu et al. (68) showed that, in general, the dietary inclusion of agro-industrial by products only showed negligible effects on milk yield and composition in small ruminants. The milk urea contents obtained in the current study fell within range values reported in literature for dairy goats (69). However, differently from other milk macro components, but similarly to what observed by Campione et al. (38) in dairy sheep, milk from the CBS-fed goats showed a significantly lower amount of urea when compared to the CTRL group. Campione et al. (38) hypothesized that such a result could be the consequence of the intrinsic characteristics of proteins in CBS. In fact, the high relative proportion of by-pass protein (rumen undegradable protein equal to 59% of total CP in the CBS used in the present study study), and particularly of CNCPS fraction C (unavailable nitrogen) (38), lowers the ratio between available protein and available energy at ruminal level in the CBS-fed animals, even though the CTRL and CBS diets were formulated to be isonitrogenous and isoenergetic. Such results confirm previous findings by Giaccone et al. (70), who showed seasonal variations in the urea content of milk from Girgentana goats, with higher values associated with grazing activities in winter months, when pastures are richer in soluble nitrogen and poorer in NDF and energy.
Furthermore, the observed significant reduction of milk lactose in the CBS-fed group of goats is consistent with the findings of Cobanović et al. (71) who showed a clear positive correlation between urea and lactose contents in goat milk.
Finally, the slight differences observed in yield and quality of milk, and dry matter intake (although the latter statistically significant) did not affect feed efficiency (Table 3). The obtained results are consistent with previous findings in literature: feed efficiency did not vary when different by-products are fed to dairy goats (72, 73). Moreover, the FE results are comparable with data obtained in a previous experimental trial in the same farm (74).
Milk Fatty Acid Profile
Only few changes were detected in the milk FA profile between the two experimental groups. Dietary tannins have been shown to modulate specific metabolic pathways and steps of the rumen biohydrogenation of dietary lipids (75), leading to an improved quality of the lipid fraction of milk in small ruminants (76). However, in the current study, the lack of significant changes between the two experimental groups in the milk concentrations of trans-octadecenoic and trans-octadecadienoic acids, which are intermediate products of ruminal biohydrogenation of dietary unsaturated fatty acids, suggests a lack or only negligible effects of tannins in CBS on the rumen microflora composition and activity. This may be associated to the relatively low amounts of tannins in CBS, when compared to the amounts of tannins reported for other agro-industrial by-products (68). Most probably, the amount of TT in the CBS-containing diet in the current trial was not great enough to significantly affect rates and extents of ruminal biohydrogenation in dairy goats, as already previously suggested by other authors (46, 77). Lignin and other non- or slowly degradable fiber fractions of feeds can significantly slow down the passage rates of both solid and liquid matter in the rumen (78). This led Campione et al. (38) hypothesize that the high concentration of ADL in CBS could have increased the retention time of the feed inside the reticulo-rumen, consequently increasing the time available for the microflora to biohydrogenate the unsaturated fatty acids ingested with the diet, therefore hiding the effects of tannins on rumen biohydrogenation. Such hypothesis may be also coherent to the observed reduced DMI of CBS-fed goats in the current trial, as increasing retention times also lead to increased rumen physical filling (78).
Milk odd-chain FA and BCFA mainly derive from rumen bacteria. Despite their low concentrations in ruminant milk fat, these FA of microbial origin have aroused interest among nutritionists as diagnostic parameters of rumen function (79) and because of their putative beneficial health effects in humans (80). In the current trial, milk from the CBS-fed goats showed increased concentrations for the majority of both iso and anteiso BCFA forms. Ruminant diet is addressed as the main factor affecting the concentration of BCFA in milk fat (81). As dietary tannins negatively affect the concentration of BCFA in milk (51), the increased BCFA concentration in the CBS-fed goats strengthen the hypothesis that the tannin level of the CBS diet was too low to exert detrimental effects on the rumen metabolism in this species. When compared to the CTRL-fed goats, the CBS-fed goats ingested lower amounts of PUFA from the diet (Table 2). As PUFA are known to exert toxic effects on the rumen microflora, particularly on cellulolytic bacteria, a reduced PUFA intake from the diet may have determined a quantitative increase of bacteria biomass inside the rumen (82), and a subsequent higher availability of BCFA for milk fat synthesis in the mammary gland (83). In addition, the higher fiber content of the CBS diet (Table 1) may also partly explain the observed increase of BCFA in the CBS-fed goats, as a positive association has been previously reported between dietary fiber and FA of microbial origin (83). Consistently, Nudda et al. (83) recently reported significant seasonal variations in the BCFA concentrations in goat milk and cheese fat mainly as a consequence of reduced contents of PUFA (mainly ALA) and protein, and increased fiber, in grazed pastures of advanced phenological stages. To the best of our knowledge, to date no studies are available on the effects of the dietary inclusion of cocoa by-products on the compositional variation of rumen microbiome to better understand the biological mechanisms involved in the observed significant BCFA increase in milk fat from the CBS-fed goats.
Being CBS rich in stearic and oleic acids (Table 1), the intake of both these FA was significantly higher in the CBS-fed goats (Table 2), which led to an increase in the concentration of both stearic and oleic acids in milk fat (Tables 4, 5). The presence of stearic acid in milk is a consequence of both preformed forms ingested with the diet and forms deriving from the complete ruminal biohydrogenation of dietary unsaturated fatty acids, including oleic acid (84). Therefore, in this trial it is presumable to assume that both the above-mentioned origin sources were reasons for the increased concentration of stearic acid in milk from the CBS-fed goats. In turn, oleic acid can also derive from desaturation of stearic acid within the mammary gland thanks to the activity of the SCD enzyme (85). The SCD activity is inhibited, through transcriptional or post-transcriptional mechanisms, by the availability of rumen by-pass PUFA and trans intermediates of ruminal biohydrogenation (86), the concentrations of which were not affected by the inclusion of CBS in the goat diet. This led to a similar activity of SCD in both groups of goats, as estimated by the calculation of different desaturase indexes (74, 81, 87). When feeding dairy ewes with diets containing CBS, Campione et al. (38) detected significantly higher rumen contents of both stearic and oleic acids, which led to higher contents of these FA in ovine milk and cheese. The observed increase of oleic acid in milk can be considered a positive effect of the inclusion of CBS in the goats' diet. In fact, oleic acid presents beneficial modulatory effects on various diseases including cancer, autoimmune- and inflammatory-related pathologies (88).
Even if there is no consensus nowadays in the scientific community on what the ∑ n6/∑ n3 FA ratio of the diet should be to obtain optimal health effects in humans (89), it is well-known that it can affect inflammation and other biological processes (90, 91). A recommended value equal or lower than four was suggested in the past for the human diet (92). In the current study, the milk from the CTRL group fell within this range, with a value (∑ n6/∑ n3 FA ratio equal to 1.14) comparable to that of ancestral human diets (93). A further significant improvement (∑ n6/∑ n3 FA ratio equal to 1.03) was obtained when CBS was included in the goat diet, supporting the findings of Campione et al. (38) with the administration of CBS in the diet of dairy ewes.
In conclusion, we showed that a possible utilization to upgrade the value of by-products from the cocoa industry, such as the CBS, is their inclusion in diets for livestock animals. Particular attention by nutritionists must be addressed to the presence of theobromine in CBS. The observed reduction of the DMI by the goats may be associated to the presence of this alkaloid or high amount of lignin in CBS and needs further considerations. However, if CBS is included in diets for lactating goats in amounts that fall within the limits imposed by the current legislation for theobromine, no adverse effects are expected on the milk production performance, particularly when considering milk yield and the contents of milk main constituents. An expected outcome of the high proportion of unavailable nitrogen in CBS is the reduction of the urea content in goat milk. When considering goat milk fatty acids, only minor changes were induced by CBS, including increased concentrations of BCFA, stearic and oleic acids, and a reduced ∑ n6/∑ n3 FA ratio. Despite the presence of tannins in CBS, no impairments of ruminal biohydrogenation pathways or steps were observed.
Data Availability Statement
The raw data supporting the conclusions of this article will be made available by the authors, without undue reservation.
Ethics Statement
According to the National Italian Law (D.L. 26/2014), no specific ethical approval is required for this study, as no pain, suffering, distress or prolonged damage equivalent to or greater than that caused by the insertion of a needle was applied. Written informed consent was obtained from the breeder for the participation of his animals in this study.
Author Contributions
PC, AM, CL, and MR conceived and designed the experiment, analyzed, and interpreted the data. PC, AM, and LC prepared the diets, performed the trial, and collected the experimental data. CL, VM, and LC performed the chemical analyses. PC performed the statistical analysis. MR, PC, AM, and LC analyzed and interpreted the data. MR and LC wrote the first draft of the manuscript. All authors critically reviewed the manuscript for its intellectual content and gave their approval for the final version to be published.
Funding
This research was funded by the University of Turin (Project CORP_RILO_17_01—Valorizzazione di by-products nell'alimentazione animale: da scarto a functional feeds).
Conflict of Interest
The authors declare that the research was conducted in the absence of any commercial or financial relationships that could be construed as a potential conflict of interest.
Publisher's Note
All claims expressed in this article are solely those of the authors and do not necessarily represent those of their affiliated organizations, or those of the publisher, the editors and the reviewers. Any product that may be evaluated in this article, or claim that may be made by its manufacturer, is not guaranteed or endorsed by the publisher.
Acknowledgments
The authors gratefully thank Dr. Aurelio Ceresa for technical assistance and care of animals.
Abbreviations
ADFom, acid detergent fiber; ADL, acid detergent lignin; aNDFom, neutral detergent fiber; BCFA, branched chain fatty acids; c, cis; CB, cocoa beans; CBS, cocoa bean shell; CLA, conjugated linoleic acid; CNCPS, Cornell Net Carbohydrate and Protein System; CP, crude protein; CT, condensed tannins; CTRL, control; DI, desaturase index; DM, dry matter; DMI, dry matter intake; DT, dietary treatment; ECM, energy corrected milk; EE, ether extract; FA, fatty acid; FAME, fatty acid methyl esters; FE, feed efficiency; HT, hydrolysable tannins; MID, methylene interrupted diene; MUFA, monounsaturated fatty acids; n, number of samples; n.d., not detected; NEL, net energy for lactation; NMID, non-methylene interrupted diene; ns, not significant; NSC, non-structural carbohydrates; NTP, non-tannin phenols; PUFA, polyunsaturated fatty acids; RDP, rumen degradable protein; SCC, somatic cell count; SD, sampling date; SFA, saturated fatty acids; t, trans; TEP, total extractable phenols; TFA, total fatty acids; TT, total tannins.
References
1. United Nations. World Population Prospects 2019: Highlights. Available online at: https://www.un.org/development/desa/publications/world-population-prospects-2019-highlights.html (accessed July 22, 2021).
2. European, Union, 2019. Global Food Supply and Demand. Consumer Trends and Trade Challenges. Available online at: https://ec.europa.eu/info/sites/default/files/food-farming-fisheries/farming/documents/market-brief-food-challenges-sep2019_en.pdf (accessed August 11, 2021).
3. Yang K, Qing Y, Yu Q, Tang X, Chen G, Fang R, et al. By-product feeds: current understanding and future perspectives. Agriculture. (2021) 11:207. doi: 10.3390/agriculture11030207
4. Okiyama DCG, Navarro SLB, Rodrigues CEC. Cocoa shell and its compounds: application in the food industry. Trends Food Sci Technol. (2017) 63:103–12. doi: 10.1016/j.tifs.2017.03.007
5. ICCO (International Cocoa Organization). Quarterly Bulletin of Cocoa Statistics, Vol. XLVII, No. 2, Cocoa Year 2020/21.
6. Vásquez ZS, De Carvalho Neto DP, Pereira GVM, Vandenberghe LPS, De Oliveira PZ, Tiburcio PB, et al. Biotechnological approaches for cocoa waste management: a review. Waste Manage. (2019) 90:72–83. doi: 10.1016/j.wasman.2019.04.030
7. Rojo-Poveda O, Barbosa-Pereira L, Zeppa G, Stévigny C. Cocoa bean shell-A by-product with nutritional properties and biofunctional potential. Nutrients. (2020) 12:1123. doi: 10.3390/nu12041123
8. Balladares C, Chóez-Guaranda I, García J, Sosa D, Pérez S, González JE, et al. Physicochemical characterization of Theobroma cacao L. sweatings in Ecuadorian coast. Emir J Food Agric. (2016) 28:741–5. doi: 10.9755/ejfa.2016-02-187
9. Hashimoto JC, Lima JC, Celeghini RM, Nogueira AB, Efraim P, Poppi RJ, et al. Quality control of commercial cocoa beans (Theobroma cacao L.) by near-infrared spectroscopy. Food Anal Methods. (2018) 11:1510–7. doi: 10.1007/s12161-017-1137-2
10. Botella-Martínez C, Lucas-Gonzalez R, Ballester-Costa C, Pérez-Álvarez JA, Fernández-López J, Delgado-Ospina J, et al. Ghanaian cocoa (Theobroma cacao L.) bean shells coproducts: effect of particle size on chemical composition, bioactive compound content and antioxidant activity. Agronomy. (2021) 11:401. doi: 10.3390/agronomy11020401
11. Bordiga M, Locatelli M, Travaglia F, Coisson JD, Mazza G, Arlorio M. Evaluation of the effect of processing on cocoa polyphenols: antiradical activity, anthocyanins and procyanidins profiling from raw beans to chocolate. Int J Food Sci Technol. (2015) 50:840–8. doi: 10.1111/ijfs.12760
12. Valenturf APM, Purnell P. Principles for a sustainable circular economy. Sustain Prod Consum. (2021) 27:1437–57. doi: 10.1016/j.spc.2021.02.018
13. Santana DP, Sanchez JLR, Calle J, de Villavicencio MN, Ortega LD, Llanes LH. Use of cocoa bean shell as a source of dietetic fiber and antioxidants in the production of sweet cookies. Ciência Tecnol Alime. (2018) 28:62–7.
14. Kārklina D, Gedrovica I, Reca M, Kronberga M. Production of biscuits with higher nutritional value. Proc Latv Acad Sci B Nat Exact Appl Sci. (2012) 66:113–6. doi: 10.2478/v10046-012-0005-0
15. Collar C, Rosell CM, Muguerza B, Moulay L. Breadmaking performance and keeping behavior of cocoa-soluble fiber-enriched wheat breads. Food Sci Technol Int. (2009) 15:79–87. doi: 10.1177/1082013208102643
16. Handojo L, Triharyogi H, Indarto A. Cocoa bean shell waste as potential raw material for dietary fiber powder. Int J Recycl Org Waste Agric. (2019) 8:485–91. doi: 10.1007/s40093-019-0271-9
17. Manzano P, Hernández J, Quijano-Avilés M, Barragán A, Chóez-Guaranda I, Viteri R, et al. Polyphenols extracted from Theobroma cacao waste and its utility as antioxidant. Emir J Food Agric. (2017) 29:45–50. doi: 10.9755/ejfa.2016-04-388
18. Ismail A, Yee CL. Antioxidative effects of extracts of cocoa shell, roselle seeds and a combination of both extracts on the susceptibility of cooked beef to lipid oxidation. J Food Technol. (2006) 4:10–5.
19. Nair KPP. Cocoa (Theobroma cacao L.). In: Nair KPP, editor. The Agronomy and Economy of Important Tree Crops of the Developing World. Amsterdam: Elsevier (2010). p. 131–80.
20. Mancini G, Papirio S, Lens PN, Esposito G. Effect of N-methylmorpholine-N-oxide pretreatment on biogas production from rice straw, cocoa shell, and hazelnut skin. Environ Eng Sci. (2016) 33:843–50. doi: 10.1089/ees.2016.0138
21. Ilham M, Fazil A. Performance and kinetic study of the anaerobic co-digestion of cocoa husk and digested cow manure with high organic loading rate. INMATEH Agric Eng. (2018) 55:131–40.
22. Puglia D, Dominici F, Badalotti M, Santulli C, Kenny J. Tensile, thermal and morphological characterization of cocoa bean shells (CBS)/polycaprolactone-based composites. J Renew Mater. (2016) 4:199–205. doi: 10.7569/JRM.2016.634102
23. Papadopoulou EL, Paul UC, Tran TN, Suarato G, Ceseracciu L, Marras S, et al. Sustainable active food packaging from poly(lactic acid) and cocoa bean shells. ACS Appl Mater Interfaces. (2019) 11:31317–27. doi: 10.1021/acsami.9b09755
24. Tran TN, Heredia-Guerrero JA, Mai BT, Ceseracciu L, Marini L, Athanassiou A, et al. Bioelastomers based on cocoa shell waste with antioxidant ability. Adv Sustain Syst. (2017) 1:1700002. doi: 10.1002/adsu.201700002
25. Panak Balentić J, Ačkar D, Jokić S, Jozinović A, Babić J, Miličević B, et al. Cocoa shell: a by-product with great potential for wide application. Molecules. (2018) 23:1404. doi: 10.3390/molecules23061404
26. Yajima A, Owada H, Kobayashi S, Komatsu N, Takehara K, Ito M, et al. Cacao bean husk: an applicable bedding material in dairy free-stall barns. Asian-Australas J Anim Sci. (2017) 30:1048. doi: 10.5713/ajas.16.0877
27. Makinde OJ, Okunade SA, Opoola E, Sikiru AB, Solomon O, Elaigwu S. Exploration of cocoa (Theobroma cacao) by-products as valuable potential resources in livestock feeds and feeding systems. In: Aikpokpodion P, editor. Theobroma Cacao: Deploying Science for Sustainability of Global Cocoa Economy. London: IntechOpen (2019). p. 1–15.
28. Adamafio NA. Theobromine toxicity and remediation of cocoa by-products: an overview. J Biol Sci. (2013) 13:570–6. doi: 10.3923/jbs.2013.570.576
29. Olumide MD, Hamzat RA, Bamijoko OJ, Akinsoyinu AO. Effects of treated cocoa (Theobroma cacao) bean shell based diets on serum biochemistry and haematological indices of laying hens. Int J Livest Res. (2017) 7:8–20. doi: 10.5455/ijlr.20161218125703
30. Adeyina A, Apata D, Annongu A, Olatunde O, Alli O, Okupke KM. Performance and physiological response of weaner rabbits fed hot water treated cocoa bean shell based diet. Res J Vet Sci. (2010) 5:53–7.
31. EFSA (European Food Safety Authority). Scientific Opinion of the Panel on Contaminants in the Food Chain on a request from the European Commission on theobromine as undesirable substances in animal feed. EFSA J. (2008) 725:1–66. doi: 10.2903/j.efsa.2008.725
32. Commission Directive 2009/141/EC of 23 November 2009 amending Annex I to Directive 2002/32/EC of the European Parliament of the Council as regards maximum levels for arsenic, theobromine Datura sp., Ricinus communis L., Croton tiglium L. and Abrus precatorius L. Off J Eur Union. (2009) 43:21–23.
33. Ogunsipe MH, Ibidapo I, Oloruntola OD, Agbede JO. Growth performance of pigs on dietary cocoa bean shell meal. Livest Res Rural Dev. (2017) 29:18.
34. Ayinde OE, Ojo V, Adeyina AA, Adesoye O. Economics of using cocoa bean shell as feed supplement for rabbits. Pak J Nutr. (2010) 9:195–7. doi: 10.3923/pjn.2010.195.197
35. Olubamiwa O, Ikyo SM, Adebowale BA, Omojola A, Hamzat RA. Effect of boiling time on the utilization of cocoa bean shell in laying hen feeds. Int J Poult Sci. (2006) 5:1137–9. doi: 10.3923/ijps.2006.1137.1139
36. Bamba Y, Ouattara N, Soro Y, Ouattara A, Yao K, Gourène G. Evaluation of production efficiency of Nile tilapia (Oreochromis niloticus L.) fed diets containing crop residues in combination with cocoa bean shell and coconut oil cake in Côte d'Ivoire. Livest Res Rural Dev. (2014) 26:10.
37. Amin M, Cahyono A. The use of cocoa bean waste as a supplement in male Bali cattle feeding. Proc Int Sem LPVT. (2016) 45–9. doi: 10.14334/Proc.Intsem.LPVT-2016-p.45-49
38. Campione A, Pauselli M, Natalello A, Valenti B, Pomente C, Avondo M, et al. Inclusion of cocoa by-product in the diet of dairy sheep: Effect on the fatty acid profile of ruminal content and on the composition of milk and cheese. Animal. (2021) 15:100243. doi: 10.1016/j.animal.2021.100243
39. NRC (National Research Council). Nutrient Requirements of Small Ruminants: Sheep, Goats, Cervids, and New World Camelids. Washington, DC: The National Academy Press (2007).
40. AOAC International. Official Methods of Analysis of AOAC International, 16th Edn. Gaithersburg: Association of Official Analytical Chemists (2000).
41. AOAC International. Official Methods of Analysis of AOAC International, 17th Edn. Gaithersburg: Association of Official Analytical Chemists (2003).
42. Mertens RD. Gravimetric determination of amylase-treated neutral detergent fiber in feeds with refluxing in beakers or crucibles: collaborative study. J AOAC Int. (2002) 85:1217–40.
43. Licitra G, Hernandez TM, Van Soest PJ. Standardization of procedures for nitrogen fractionation of ruminant feeds. Anim Feed Sci Technol. (1996) 57:347–58. doi: 10.1016/0377-8401(95)00837-3
44. Dabbou S, Gai F, Renna M, Rotolo L, Dabbou S, Lussiana C, et al. Inclusion of bilberry pomace in rabbit diets: effects on carcass characteristics and meat quality. Meat Sci. (2017) 124:77–83. doi: 10.1016/j.meatsci.2016.10.013
45. Renna M, Cornale P, Lussiana C, Battaglini LM, Turille G, Mimosi A. Milk yield, gross composition and fatty acid profile of dual-purpose Aosta Red Pied cows fed separate concentrate-forage versus total mixed ration. Anim Sci J. (2014) 85:37–45. doi: 10.1111/asj.12083
46. Iussig G, Renna M, Gorlier A, Lonati M, Lussiana C, Battaglini LM, et al. Browsing ratio, species intake, and milk fatty acid composition of goats foraging on alpine open grassland and grazable forestland. Small Rumin Res. (2015) 132:12–24. doi: 10.1016/j.smallrumres.2015.09.013
47. Ravetto Enri S, Probo M, Renna M, Caro E, Lussiana C, Battaglini LM, et al. Temporal variations in leaf traits, chemical composition and in vitro true digestibility of four temperate fodder tree species. Anim Prod Sci. (2020) 60:643–58. doi: 10.1071/AN18771
48. Cornale P, Renna M, Lussiana C, Bigi D, Chessa S, Mimosi A. The Grey Goat of Lanzo Valleys (Fiurinà): breed characteristics, genetic diversity, and quantitative-qualitative milk traits. Small Rumin Res. (2014) 116:1–13. doi: 10.1016/j.smallrumres.2013.10.006
49. Kramer JKG, Hernandez M, Cruz-Hernandez C, Kraft J, Dugan MER. Combining results of two GC separations partly achieves determination of all cis and trans 16:1, 18:1, 18:2 and 18:3 except CLA Isomers of milk fat as demonstrated using Ag-Ion SPE fractionation. Lipids. (2008) 4:259–73. doi: 10.1007/s11745-007-3143-4
50. Orth R. Sample Day and Lactation Report, DHIA 200. Fact Sheet A-2. Ames, IA: Mid-States Dairy Records Processing Center (DRPC) (1992).
51. Renna M, Lussiana C, Malfatto V, Gerbelle M, Turille G, Medana C, et al. Evaluating the suitability of hazelnut skin as a feed ingredient in the diet of dairy cows. Animals. (2020) 10:1653. doi: 10.3390/ani10091653
52. Littell RC, Henry PR, Ammerman CB. Statistical analysis of repeated measures data using SAS procedures. J Anim Sci. (1998) 76:1216–31. doi: 10.2527/1998.7641216x
53. Nsor-Atindana J, Zhong F, Kebitsamang MJ, Mothibe ML, Bangoura C, Lagnika C. Quantification of total polyphenolic content and antimicrobial activity of cocoa (Theobroma cacao L.) bean shells. Pak J Nutr. (2012) 11:672–7. doi: 10.3923/pjn.2012.672.677
54. Lanzas C, Broderick GA, Fox DG. Improved feed protein fractionation schemes for formulating rations with the Cornell Net Carbohydrate and Protein System. J Dairy Sci. (2008) 91:4881–91. doi: 10.3168/jds.2008-1440
55. Campione A, Natalello A, Valenti B, Luciano G, Rufino-Moya PJ, Avondo M, et al. Effect of feeding hazelnut skin on animal performance, milk quality, and rumen fatty acids in lactating ewes. Animals. (2020) 10:588. doi: 10.3390/ani10040588
56. Pinto CS, Magalhães ALR, Teodoro AL, Gois GC, Véras RML, Campos FS, et al. Potential alternative feed sources for ruminant feeding from the biodiesel production chain by-products. S Afr J Anim Sci. (2020) 50:69–77. doi: 10.4314/sajas.v50i1.8
57. Mahesh MS, Thakur SS. Rice gluten meal, an agro-industrial by-product, supports performance attributes in lactating Murrah buffaloes (Bubalus bubalis). J Clean Prod. (2018) 177:655–64. doi: 10.1016/j.jclepro.2017.12.206
58. Lessa OA, Reis NS, Leite SGF, Gutarra MLE, Souza AO, Gualberto SA, et al. Effect of the solid state fermentation of cocoa shell on the secondary metabolites, antioxidant activity, and fatty acids. Food Sci Biotechnol. (2018) 27:107–13. doi: 10.1007/s10068-017-0196-x
59. Soares ID, Okiyama DCG, da Costa Rodrigues CE. Simultaneous green extraction of fat and bioactive compounds of cocoa shell and protein fraction functionalities evaluation. Food Res Int. (2020) 137:109622. doi: 10.1016/j.foodres.2020.109622
60. Razzaghi A, Naserian AA, Valizadeh R, Ebrahimi SH, Khorrami B, Malekkahahi M, et al. Pomegranate seed pulp, pistachio hulls, and tomato pomace as replacement of wheat bran increased milk conjugated linoleic acid concentrations without adverse effects on ruminal fermentation and performance of Saanen dairy goats. Anim Feed Sci Technol. (2015) 210:46–55. doi: 10.1016/j.anifeedsci.2015.09.014
61. Carta S, Nudda A, Cappai MG, Lunesu MF, Atzori AS, Battacone G, et al. Cocoa husks can effectively replace soybean hulls in dairy sheep diets-Effects on milk production traits and hematological parameters. J Dairy Sci. (2020) 103:1553–8. doi: 10.3168/jds.2019-17550
62. Tseu RJ, Junior FP, Carvalho RF, Sene GA, Tropaldi CB, Peres AH, et al. Effect of tannins and monensin on feeding behaviour, feed intake, digestive parameters and microbial efficiency of nellore cows. Ital J Anim Sci. (2020) 19:262–73. doi: 10.1080/1828051X.2020.1729667
63. Yisehak K, Kibreab Y, Taye T, Ribeiro Alves Lourenço M, Janssens GPJ. Response to dietary tannin challenges in view of the browser/grazer dichotomy in an Ethiopian setting: Bonga sheep versus Kaffa goats. Trop Anim Health Prod. (2016) 48:125–31. doi: 10.1007/s11250-015-0931-3
64. Giger-Reverdin S, Domange C, Broudiscou LP, Sauvant D, Berthelot V. Rumen function in goats, an example of adaptive capacity. J Dairy Res. (2020) 87:45–51. doi: 10.1017/S0022029920000060
65. Min BR, Solaiman S. Comparative aspects of plant tannins on digestive physiology, nutrition and microbial community changes in sheep and goats: a review. J Anim Physiol Anim Nutr. (2018) 102:1181–93. doi: 10.1111/jpn.12938
66. da Silva APR, de Sant'ana AS, do Nascimento SPO, Barbosa SN, Miranda ALA, Luna FS, et al. Tannins in the diet for lactating goats from different genetic groups in the Brazilian semiarid: Nitrogen, energy and water balance. Anim Feed Sci Technol. (2021) 279:115023. doi: 10.1016/j.anifeedsci.2021.115023
67. Aregheore EM. Chemical evaluation and digestibility of cocoa (Theobroma cacao) byproducts fed to goats. Trop Anim Health Prod. (2002) 34:339–48. doi: 10.1023/a:1015638903740
68. Correddu F, Lunesu MF, Buffa G, Atzori AS, Nudda A, Battacone G et al. Can agro-industrial by-products rich in polyphenols be advantageously used in the feeding and nutrition of dairy small ruminants? Animals. (2020) 10:131. doi: 10.3390/ani10010131
69. Bendelja Ljoljić DB, Špehar ID, Prpić Z, Vnučec I, SamarŽija D. Urea concentration in goat milk: importance of determination and factors of variability. J Cent Eur Agric. (2020) 21:707–21. doi: 10.5513/JCEA01/21.4.3058
70. Giaccone P, Todaro M, Scatassa ML. Factors associated with milk urea concentrations in Girgentana goats. Ital J Anim Sci. (2007) 6(Suppl. 1):622–4. doi: 10.4081/ijas.2007.1s.622
71. Cobanović K, Krstović S, Štrbac L, Šaran M, Kasalica A, Popović M. Relationship between milk urea level and milk parameters in the Saanen dairy goat. Contemp Agric. (2019) 68:88–91. doi: 10.2478/contagri-2019-0014
72. Arco-Pérez A, Ramos-Morales E, Yáñez-Ruiz DR, Abecia L, Martín-García AI. Nutritive evaluation and milk quality of including of tomato or olive by-products silages with sunflower oil in the diet of dairy goats. Anim Feed Sci Technol. (2017) 232:57–70. doi: 10.1016/j.anifeedsci.2017.08.008
73. Marcos CN, Carro MD, Fernández Yepes JE, Haro A, Romero-Huelva M, Molina-Alcaide E. Effects of agroindustrial by-product supplementation on dairy goat milk characteristics, nutrient utilization, ruminal fermentation, and methane production. J Dairy Sci. (2020) 103:1472–83. doi: 10.3168/jds.2019-17386
74. Renna M, Lussiana C, Cornale P, Battaglini LM, Fortina R, Mimosi A. Effect of ruminally unprotected Echium oil on milk yield, composition and fatty acid profile in mid-lactation goats. J Dairy Res. (2016) 83:28–34. doi: 10.1017/S0022029915000655
75. Frutos P, Hervas G, Natalello A, Luciano G, Fondevila M, Priolo A, et al. Ability of tannins to modulate ruminal lipid metabolism and milk and meat fatty acid profiles. Anim Feed Sci Technol. (2020) 269:114623. doi: 10.1016/j.anifeedsci.2020.114623
76. Nudda A, Cannas A, Correddu F, Atzori AS, Lunesu MF, Battacone G, et al. Sheep and goats respond differently to feeding strategies directed to improve the fatty acid profile of milk fat. Animals. (2020) 10:1290. doi: 10.3390/ani10081290
77. Mancilla-Leytón JM, Vicente AM, Delgado-Pertíñez M. Summer diet selection of dairy goats grazing in a Mediterranean shrubland and the quality of secreted fat. Small Rumin Res. (2013) 113:437–45. doi: 10.1016/j.smallrumres.2013.04.010
78. Moyo M, Nsahlai IV. Rate of passage of digesta in ruminants; are goats different? In: Kukovics S, editor. Goat Science. London: InTechOpen (2018). p. 39–74. Available online at: https://www.intechopen.com/chapters/56672
79. Cívico A, Núñez Sánchez N, Gómez-Cortés P, de la Fuente MA, Peña Blanco F, Juárez M, et al. Odd- and branched-chain fatty acids in goat milk as indicators of the diet composition. Ital J Anim Sci. (2017) 16:68–74. doi: 10.1080/1828051X.2016.1263547
80. Taormina VM, Unger AL, Schiksnis MR, Torres-Gonzalez M, Kraft J. Branched-chain fatty acids - an underexplored class of dairy-derived fatty acids. Nutrients. (2020) 12:2875. doi: 10.3390/nu12092875
81. Hanuš O, Samková E, KríŽová L, Hasonová L, Kala R. Role of fatty acids in milk fat and the influence of selected factors on their variability - a review. Molecules. (2018) 23:1636. doi: 10.3390/molecules23071636
82. Enjalbert F, Combes S, Zened A, Meynadier A. Rumen microbiota and dietary fat: a mutual shaping. J Appl Microbiol. (2017) 123:782–97. doi: 10.1111/jam.13501
83. Nudda A, Correddu F, Cesarani A, Pulina G, Battacone G. Functional odd- and branched-chain fatty acid in sheep and goat milk and cheeses. Dairy. (2021) 2:79–89. doi: 10.3390/dairy2010008
84. Schettino B, Vega S, Gutiérrez R, Escobar A, Romero J, Domínguez E, et al. Fatty acid profile of goat milk in diets supplemented with chia seed (Salvia hispanica L.). J Dairy Sci. (2017) 100:6256–65. doi: 10.3168/jds.2017-12785
85. Toral PG, Frutos P, Carreño D, Hervás G. Endogenous synthesis of milk oleic acid in dairy ewes: in vivo measurement using 13C-labeled stearic acid. J Dairy Sci. (2017) 100:5880–7. doi: 10.3168/jds.2016-12097
86. Tudisco R, Chiofalo B, Lo Presti V, Morittu VM, Moniello G, Grossi M, et al. Influence of feeding linseed on SCD activity in grazing goat mammary glands. Animals. (2019) 9:786. doi: 10.3390/ani9100786
87. Renna M, Lussiana C, D'Agostino M, Mimosi A, Fortina R. Extruded linseed supplementation in dairy goat diet: effects on productive performance and fatty acid profile of bulk milk, fresh and ripened cheese. J Anim Vet Adv. (2013) 12:1550–64. doi: 10.3923/javaa.2013.1550.1564
88. Sales-Campos H, de Souza PR, Peghini BC, da Silva JS, Cardoso CR. An overview of the modulatory effects of oleic acid in health and disease. Mini-Rev Med Chem. (2013) 13:201–10. doi: 10.2174/1389557511313020003
89. Djuricic I, Calder PC. Beneficial outcomes of Omega-6 and Omega-3 polyunsaturated fatty acids on human health: an update for 2021. Nutrients. (2021) 13:2421. doi: 10.3390/nu13072421
90. D'Angelo S, Motti ML, Meccariello R. ω-3 and ω-6 polyunsaturated fatty acids, obesity and cancer. Nutrients. (2020) 12:2751. doi: 10.3390/nu12092751
91. DiNicolantonio JJ, O'Keefe JH. Importance of maintaining a low omega-6/omega-3 ratio for reducing inflammation. Open Heart. (2018) 5:e000946. doi: 10.1136/openhrt-2018-000946
92. Sheppard KW, Cheatham CL. Omega-6/omega-3 fatty acid intake of children and older adults in the U.S.: dietary intake in comparison to current dietary recommendations and the Healthy Eating Index. Lipids Health Dis. (2018) 17:43. doi: 10.1186/s12944-018-0693-9
Keywords: agro-industrial by-products, caprine milk, lipids, production performance, Theobroma cacao (L.)
Citation: Renna M, Lussiana C, Colonna L, Malfatto VM, Mimosi A and Cornale P (2022) Inclusion of Cocoa Bean Shell in the Diet of Dairy Goats: Effects on Milk Production Performance and Milk Fatty Acid Profile. Front. Vet. Sci. 9:848452. doi: 10.3389/fvets.2022.848452
Received: 04 January 2022; Accepted: 25 January 2022;
Published: 18 February 2022.
Edited by:
Amlan Kumar Patra, West Bengal University of Animal and Fishery Sciences, IndiaReviewed by:
Carlos Fernández, Universitat Politècnica de València, SpainTarek Abdelfattah Morsy, National Research Centre, Egypt
Copyright © 2022 Renna, Lussiana, Colonna, Malfatto, Mimosi and Cornale. This is an open-access article distributed under the terms of the Creative Commons Attribution License (CC BY). The use, distribution or reproduction in other forums is permitted, provided the original author(s) and the copyright owner(s) are credited and that the original publication in this journal is cited, in accordance with accepted academic practice. No use, distribution or reproduction is permitted which does not comply with these terms.
*Correspondence: Manuela Renna, bWFudWVsYS5yZW5uYSYjeDAwMDQwO3VuaXRvLml0