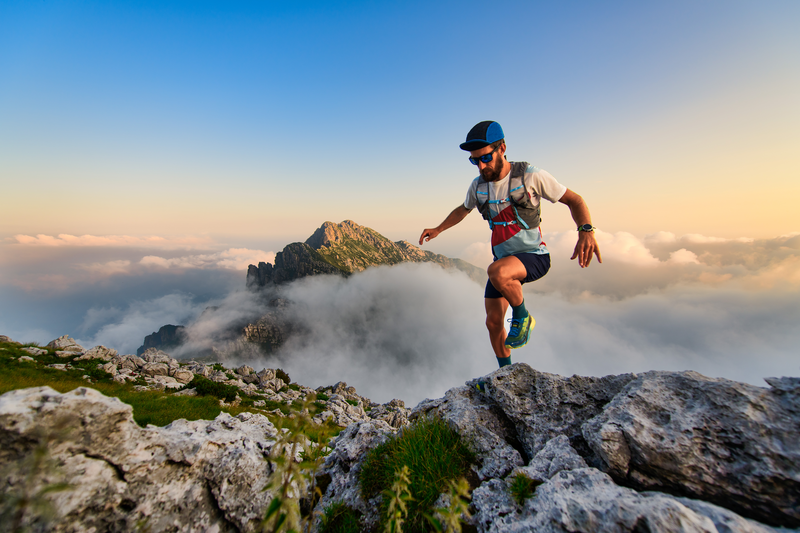
94% of researchers rate our articles as excellent or good
Learn more about the work of our research integrity team to safeguard the quality of each article we publish.
Find out more
ORIGINAL RESEARCH article
Front. Vet. Sci. , 30 March 2022
Sec. Comparative and Clinical Medicine
Volume 9 - 2022 | https://doi.org/10.3389/fvets.2022.846492
This article is part of the Research Topic Novel Insight into the Diagnosis and Treatment of Cardio(thoracic) Diseases in Dogs and Cats View all 10 articles
Myxomatous mitral valve disease (MMVD) is the most common cause of congestive heart failure in dogs, and although complications of MMVD to the lungs and kidneys have been identified, complications to the gut are less well understood. The intestinal microbiota is an important factor in the gut, and although the association between heart disease and the intestinal microbiota has been shown in human medicine, it is unknown in dogs. The study aimed to evaluate the relationship between MMVD and gut microbiota. A total of 69 healthy Chihuahuas and Chihuahuas with MMVD were evaluated for cardiac health by echocardiography and chest radiography and grouped according to ACVIM guidelines. Fecal samples were collected from all cases and 16S rRNA sequencing was used to reveal the intestinal microbiota. There were significant differences in LA/Ao, LVIDd, E vel, VHS, and VLAS with the severity of ACVIM. On the other hand, there were no significant differences in the diversity and composition of gut microbiota among the groups. The present study did not identify the effects of MMVD on the gut microbiota.
Much research has been done on the relationship between heart disease and the gut, and the concept of “Heart-Gut Axis” is well known. An important factor in understanding the function of the gut is the intestinal microbiota. In human medicine, it has been proven that the intestinal microbiota is involved in the pathogenesis of the cardiovascular disease, and it has been reported that lowering the blood concentration of trimethylamine N-oxide, a metabolite of intestinal bacteria, reduces the risk of cardiovascular disease (1–5). In addition, functional and morphological changes in the gut are induced in patients with heart failure, which in turn affects the gut microbiota. In both conditions, heart failure with preserved ejection fraction and heart failure with reduced ejection fraction, there were changes in the gut microbiota (6, 7). It has been reported that changes in the gut microbiota and the effects of heart failure on the intestinal tract can lead to systemic inflammation (8–12). Thus, in human medicine, the relationship between heart disease and intestinal microbiota has been the focus of much research and attention. Although research on the intestinal microbiota in veterinary medicine lags that in human medicine, it has been active in recent years due to the widespread use of 16S rRNA sequencing (13). The relationship between inflammatory bowel disease and intestinal microbiota has been reported, and the relationship between disease and intestinal microbiota has attracted attention in veterinary medicine, but there have been no reports on heart disease and intestinal microbiota (14).
MMVD is the most common cause of congestive heart failure in dogs. Cavalier King Charles Spaniels, Maltese, and Toy Poodles are reported to be the predominant breeds, and in clinical practice, MMVD is commonly seen in Chihuahuas due to many dogs kept. If MMVD worsens, it can cause fatal complications such as pulmonary edema and arrhythmia. It has been suggested that heart failure due to long-term MMVD can cause decreased blood flow throughout the body, leading to ischemic injury to vital organs such as the kidney and pancreas (15–17). We clarified the intestinal complications of MMVD using intestinal mucosal injury markers (I-FABP and D-Lactate) (18). To further understand the intestinal complications of MMVD, we investigated the fecal samples from MMVD-affected and healthy Chihuahuas using 16S rRNA gene sequencing to reveal the intestinal microbiota.
This study was approved by the Rakuno Gakuen University, School of Veterinary Medicine Institutional Animal Care and Use Committee (approval No. VH19A10). Dogs sampled at two institutions, Yokohama Yamate Dogs and Cats Medical Center and Tokyo Veterinary Cardiology Center, from April 2019 to May 2020 were included in the study. The breed was limited to Chihuahuas only. All Chihuahuas that met the following inclusion criteria and came to the hospital during the study period were included: (1) adult dogs; (2) consented to the study; (3) provided a fecal sample; (4) no cardiac disease not associated with MMVD; (5) no fatal comorbidities. Most of the healthy Chihuahuas came to the hospital for checkups and preventive medical care such as rabies vaccines. Patients with diseases secondary to MMVD (e.g., pulmonary hypertension or chronic kidney disease) were included; however, patients with other cardiac diseases (e.g., cardiac tumor or epicardial disease) were excluded. After obtaining the owner's consent, physical examination (weight and body condition score [BCS]), auscultation (presence and intensity of heart murmur), medical history, current medical history, medications, antibiotics, and the usual diet were recorded in all cases. In all the cases, chest radiography and echocardiography were performed to evaluate the heart.
In all cases, the heart was evaluated by chest X-ray and echocardiography. Echocardiography was performed according to standard techniques, using a Xario (TOSHIBA, probe: PST-50AT, 5MHz) at the Yokohama Yamate Dogs and Cats Medical Center and a LOGIC e9 (GE Health care Japan, probe: 6S) at the Tokyo Veterinary Cardiology Center (19). Data were collected by two examiners, and all examinations were performed in a quiet room. Echocardiography included the subjective evaluation of the valve structure and function; mitral regurgitation (MR) jets on color Doppler examination in four-lumen cross-sectional images, left atrium to aorta ratio (LA/Ao) at diastole, left ventricular septal wall thickness at diastole (IVSd), left ventricular posterior wall thickness at diastole (LVPWd), left ventricular internal diameter at diastole (LVIDd), normalized left ventricular internal diameter (LVIDDN) (20), left ventricular internal diameters in systole (LVIDs), fractional shortening (FS), left ventricular ejection fraction (LVEF), and left ventricular inflow velocity waves (Evel, Avel) were evaluated and recorded.Chest X-rays were taken at maximal right lateral inspiration for the presence of pulmonary edema and a recording of VHS, and VLAS was measured according to previous reports (21, 22). The ACVIM guidelines were used for the severity of MMVD (23).
Fecal samples were collected using a specimen collection kit (Fecal collection container F, Fujifilm Wako Pharmaceutical) and sent to Anicom Insurance Inc. Add 200 μL of fecal samples and 810 μL of the lysis buffer (containing 224 μg/mL ProtenaseK) provided with the schematic kit to a Precellys 2 mL Soft Tissue Homogenizing Ceramic Beads Kit (KT03961-1-003.2, Bertin Instruments, France), and bead fragmentation (6,000 rpm, fragmentation 20 s, interval 30 s, fragmentation 20 s) was performed with a bead homogenizer Precellys Evolution (Bertin Instruments). The specimens were then lysed with Proteinase K by placing them on a heat block at 70°C for 10 min. Subsequently, Proteinase K was inactivated by placing the specimens on a heat block at 95°C for 5 min. The lysed samples were subjected to automated DNA extraction using chemagic 360 (PerkinElmer) and chemagic kit stool protocol to obtain 100 μL of DNA extract.
The DNAs were subjected to 16S rRNA gene amplicon sequencing. The V3–V4 regions of the 16S rRNA gene were amplified by PCR using the primers described in the Illumina 16S Sample Preparation Guide (Illumina, San Diego, CA, USA) as follows: forward, 5′-TCGTCGGCAGCGTCAGATGTGTATAAGAGACAG–CCTACGGGNGGCWGCAG-3′; reverse, 5′-GTCTCGTGGGCTCGGAGATGTGTATAAGAGACA-GGACTAC- HVGGGTATCTAATCC-3′. PCR amplification was performed using the KAPA HiFi HotStart Library Amplification Kit (Kapa Biosystems, Wilmington, MA, USA). Each PCR product was purified using an Agencourt AMPure XP Beads Kit (Beckman Coulter, Pasadena, CA, USA) and quantified using a Qubit dsDNA BR Assay Kit (Thermo Fisher Scientific, Waltham, MA, USA). One hundred nanograms of each amplicon were subjected to a second PCR round for indexing, using a Nextera XT Index Kit v2 (Illumina). After purification, the PCR products were quantified with a NanoPhotometer (Implen, Westlake Village, CA, USA) and pooled into one tube at a final concentration of 1.6 ng/μl. The concentration of the pooled DNA library was validated using an Agilent 2100 Bioanalyzer (Agilent, Santa Clara, CA, USA). After denaturation with NaOH,850 μl of 9pM DNA library and 150 μl of 9p M PhiX were mixed and subjected to pair-end sequencing using Illumina MiSeq with a MiSeq Reagent Kit v3 (600 cycles; Illumina).
The sequence data were processed using Quantitative Insights into Microbial Ecology 2 (QIIME 2) v2019.4.0 (24). The DADA2 software package v2019.4.0 incorporated in QIIME 2 was used to correct the amplicon sequence errors and to construct an amplicon sequence variant (ASV) table. The ASV table was rarefied. Microbial taxonomy was assigned using a Naïve Bayes classifier trained on the SILVA 132 99 % database.
Metrics of alpha diversity, including Shannon's index (Shannon), and those of beta diversity, including unweighted UniFrac and weighted UniFrac (weighted UniFrac), were examined using QIIME2. These diversity metrics were statistically analyzed by the method described below.
Case data were entered into a spreadsheet, and statistical analysis was performed using SPSS software (SPSS statistics ver 24.0 IBM Japan, Ltd., Tokyo, Japan). Age, weight, and BCS were subjected to a one-way analysis of variance using the Turley-Kramer post-hoc test. For gender and medications, Fisher's exact test was used. Values for each group obtained by echocardiography and chest radiography were subjected to a one-way analysis of variance using the Turley-Kramer post-hoc test.
We collected a sample of 69 cases, ranging in age from 3 to 12 years, which were divided into four groups: Healthy chihuahuas (H) (n = 19), B1 (n = 21), B2 (n = 15), and C/D (n = 14) (Table 1). Of the 14 cases in Group C/D, 3 samples were taken at presentation to the hospital in a hypoxemic crisis of fulminant pulmonary edema. The remaining 11 cases were sampled on stable on medication at a scheduled recheck. There were no cases there that were excluded after sample collection. Group H had a predominantly lower mean age compared to the other groups. One case each in groups B1 and C/D was on antimicrobial medication. They were administered erythromycin and enrofloxacin, respectively. The following comorbidities were observed in each group. Group H: canine atopic dermatitis (CAD) (n = 2), chronic kidney disease (CKD) (n = 1), epileptic seizures (n = 1), and food allergy (n = 1). Group B1: CAD (n = 1), cholelithiasis (n = 1) hypoadrenocorticism (n = 1), and hypothyroidism (n = 2). Group B2: CAD (n = 1), CKD (n = 2), diabetes mellitus (n = 1), tracheal collapse (n = 2), and hypothyroidism (n = 1). Group C/D: CKD (n = 7), tracheal collapse (n = 2). Owing to worsening cardiac disease, many patients were taking cardiac medications, and all the patients in Group C/D were taking angiotensin-converting enzyme inhibitors (ACI) and pimobendan. The three patients in Group B2 on loop diuretics (furosemide: 1.1 ± 0.6 mg/kg/day) had no history of heart failure and, were being prescribed for cough reduction. Nine patients in Group C/D were taking loop diuretics (trasemide: 0.33 ± 0.23 mg/kg/day). The dietary content is summarized in Table 2.
Table 1. Patient characteristics of healthy Chihuahuas (H) and Chihuahuas with myxomatous mitral valve disease divided into three groups (B1, B2, and C/D).
Table 2. Patient diet data in healthy Chihuahuas (H) and Chihuahuas with myxomatous mitral valve disease divided into three groups (B1, B2, and C/D).
Echocardiography, VHS, and VLAS findings are presented in Table 2. LVIDd was higher in groups B2 and C/D than in groups H and B1; however, there was no significant difference between groups B2 and C/D. The E wave also increased with severity, with group C/D having the highest increase. VHS and VLAS were also higher with worsening cardiac disease, with Group C/D having higher values than the other groups.
The ACVIM stage classification showed no significant difference in alpha diversity between the groups (Figure 1). In terms of the composition of the intestinal microbiota at the phylum level, most cases were dominated by bacterial species in the following order: Firmicutes, Bacteroides, Proteobacteria, Fusobacterium, Actinobacteria (Figure 2). There was no significant difference in the composition of the intestinal microbiota at the phylum level between the groups. Firmicutes/Bacteroidetes ratio was 82.6 ± 383.7.
Figure 1. α-diversity: Shannon index based on operational taxonomic unit abundance of healthy Chihuahuas (H) and Chihuahuas with myxomatous mitral valve disease divided into three groups (B1, B2, and C/D).
Figure 2. Abundances of taxa in the intestinal microbiota of healthy Chihuahuas (H) and Chihuahuas with myxomatous mitral valve disease divided into three groups (B1, B2, and C/D) (phylum level).
As shown in Table 3, there was a tendency for the measurements obtained by echocardiography and chest radiography to worsen with increasing severity of MMVD. LA, LA/Ao, E vel, VHS, and VLAS were significantly increased in heart failure dogs compared with other groups, suggesting that they are important items indicating the severity of MMVD. LA/Ao and E vel have been reported to be important parameters that can be poor prognostic factors in MMVD, and the results of this study are consistent with previous reports (25). FS and LVEF did not differ significantly, although there was a trend toward increased values due to worsening MMVD. Worsening of MMVD has been reported in the past to increase FS, which was also true in this study, although the difference was not significant (26). FS is influenced by a variety of factors, including age, sex, breed, concomitant disease, preload, post load, and wall stress (20, 27–29). Also, many of the patients in this study were taking pimobendan internally. Pimobendan has been reported to increase FS and may have influenced this study (30). It has also been reported that the more severe the myocardial failure, the less FS that was elevated in MMVD (26). This study is difficult to evaluate because no histological examination of the myocardium was performed, but the influence of such a condition on the results should be considered.
Table 3. Echocardiographic and radiographic data in healthy Chihuahuas (H) and Chihuahuas with myxomatous mitral valve disease divided into three groups (B1, B2, and C/D).
As shown in Figures 1, 2, there was no difference in α diversity or composition of the gut microbiota among the groups classified by the ACVIM guidelines in the gut microbiota analysis. In a study by Sandri et al. (31), the gut microbiota of dog was found to be composed of Firmicutes (43%), Bacteroidetes (19.8–26.9%), Fusobacteria (4.7–11%), and Proteobacteria (1.3–4.3%); in another study, it was found to be composed of Firmicutes (84% of all sequences), Bacteroidetes (2.9%), Fusobacteria (3.2%), Proteobacteria (7.8%) and Actinobacteria (1.7%) (32). These differences are thought to be due to individual and animal differences in intestinal microbiota (33). The intestinal microbiota of each case obtained by this study was consistent with previous reports in dogs. Human medicine has reported changes in gut microbiota composition in heart failure patients (6, 7). In this study, the FS and LVEF of heart failure dogs were normal and there was no obvious decrease in cardiac output. Decreased cardiac output has been reported to reduce blood flow to the intestinal tract and enteric membrane arteries, resulting in changes in the composition of the intestinal microbiota (6, 10). Considering the results of this study, it is possible that there is no obvious change in the composition of the gut microbiota in dogs with heart failure that do not have a reduced cardiac output. Next, we need to study the composition of the gut microbiota in dogs with heart failure due to MMVD with reduced cardiac output.
The intestinal microbiota is said to be affected by various factors such as individual differences and living environment, and it has been reported in dogs that it also changes with age. The age of Group H was lower in this study, and the difference in age between the groups may have affected the results. Chihuahuas are the preferred breed for MMVD, and the Chihuahuas that did not have MMVD tended to be sampled at a relatively young age (34–37). On the other hand, there is no significant age difference between the groups affected by MMVD (B1, B2, C/D), and comorbidities and medications administered need to be considered. The major difference was the presence of diuretics and the presence of CKD. In Group C/D, 9/14 patients were taking diuretics due to worsening MMVD, and in Group B2, 3/15 patients were taking diuretics. Diuretics in Group B2 were used to reduce cough and were used at lower volumes than in Groups C/D. In human medicine, chronic kidney disease has been reported to affect the intestinal microbiota (38). The accumulation of urea in the blood due to decreased renal function increases urea efflux in the intestine, and urease-containing intestinal bacteria increase ammonia concentration in the intestine, affecting the intestinal microbiota (39). The possibility of intestinal ischemia and acidosis due to uremia has also been suggested. We need to consider the possibility that the dogs in this study had various comorbidities (e.g., CAD, hypothyroidism, etc.) that may have affected the results.
Two cases were taking antibiotics and many cases were taking ACE-I internally. It has been reported that antibiotics and ACE-I affect the intestinal microbiota, which may have influenced the results of this study (40). In addition, the number of cases in group C/D was less than the other groups (H, B1, B2) in this study. It is necessary to consider the possibility that this may have affected the results.
The effect of diet on intestinal microbiota has been reported in dogs (33, 41). Specifically, dogs fed a natural diet have more diverse and abundant microbial composition in the gut microbiota than dogs fed a commercial feed. In this study, there was no uniformity in the diets, as shown in Table 2. In addition, the treatment diet contained several dietary fibers, including prebiotics. It is highly possible that the lack of uniformity in diet content and composition affected the gut microbiota, and the results of this study should be discussed in this light.
In this study, we were not able to confirm changes in the gut microbiota according to ACVIM stage. However, because this study was a clinical trial, there were a variety of factors in each case, and the influence of these factors on the results of this study cannot be measured. In the future, it is necessary to conduct a study with uniform conditions.
The original contributions presented in the study are included in the article/supplementary material, further inquiries can be directed to the corresponding author/s.
The animal study was reviewed and approved by the Rakuno Gakuen University, School of Veterinary Medicine Institutional Animal Care and Use Committee (approval No. VH19A10). Written informed consent was obtained from the owners for the participation of their animals in this study.
RA: sample collection, data analysis, and writing the draft. KU and KI: sample collection and data analysis. AS, GI, MA, and TF: data analysis for gut microbiota. MI: study design and data analysis. All authors contributed to the article and approved the submitted version.
AS, GI, MA, and TF were employed by Anicom Inc.
The remaining authors declare that the research was conducted in the absence of any commercial or financial relationships that could be construed as a potential conflict of interest.
All claims expressed in this article are solely those of the authors and do not necessarily represent those of their affiliated organizations, or those of the publisher, the editors and the reviewers. Any product that may be evaluated in this article, or claim that may be made by its manufacturer, is not guaranteed or endorsed by the publisher.
We would like to thank Editage (www.editage.com) for English language editing.
1. Andersen LW, Landow L, Baek L, Jansen E, Baker S. Association between gastric intramucosal pH and splanchnic endotoxin, antibody to endotoxin, and tumor necrosis factor-alpha concentrations in patients undergoing cardiopulmonary bypass. Crit Care Med. (1993) 21:210–7. doi: 10.1097/00003246-199302000-00011
2. Emoto T, Yamashita T, Sasaki N, Hirota Y, Hayashi T, So A, et al. Analysis of gut microbiota in coronary artery disease patients: a possible link between gut microbiota and coronary artery disease. J Atheroscler Thromb. (2016) 23:908–21. doi: 10.5551/jat.32672
3. Tang WH, Wang Z, Fan Y, Levison B, Hazen JE, Donahue LM, et al. Prognostic value of elevated levels of intestinal microbe-generated metabolite trimethylamine-N-oxide in patients with heart failure: refining the gut hypothesis. J Am Coll Cardiol. (2014) 64:1908–14. doi: 10.1016/j.jacc.2014.02.617
4. Wang Z, Klipfell E, Bennett BJ, Koeth R, Levison BS, Dugar B, et al. Gut flora metabolism of phosphatidylcholine promotes cardiovascular disease. Nature. (2011) 472:57–63. doi: 10.1038/nature09922
5. Wang Z, Roberts AB, Buffa JA, Levison BS, Zhu W, Org E, et al. Non-lethal inhibition of gut microbial trimethylamine production for the treatment of atherosclerosis. Cell. (2015) 163:1585–95. doi: 10.1016/j.cell.2015.11.055
6. Luedde M, Winkler T, Heinsen FA, Ruhlemann MC, Spehlmann ME, Bajrovic A, et al. Heart failure is associated with depletion of core intestinal microbiota. ESC Heart Fail. (2017) 4:282–90. doi: 10.1002/ehf2.12155
7. Huang Z, Mei X, Jiang Y, Chen T, Zhou Y. Gut Microbiota in heart failure patients with preserved ejection fraction (GUMPTION Study). Front Cardiovasc Med. (2021) 8:803744. doi: 10.3389/fcvm.2021.803744
8. Sandek A, Bauditz J, Swidsinski A, Buhner S, Weber-Eibel J, von Haehling S, et al. Altered intestinal function in patients with chronic heart failure. J Am Coll Cardiol. (2007) 50:1561–9. doi: 10.1016/j.jacc.2007.07.016
9. Sandek A, Bjarnason I, Volk HD, Crane R, Meddings JB, Niebauer J, et al. Studies on bacterial endotoxin and intestinal absorption function in patients with chronic heart failure. Int J Cardiol. (2012) 157:80–5. doi: 10.1016/j.ijcard.2010.12.016
10. Sandek A, Swidsinski A, Schroedl W, Watson A, Valentova M, Herrmann R, et al. Intestinal blood flow in patients with chronic heart failure: a link with bacterial growth, gastrointestinal symptoms, and cachexia. J Am Coll Cardiol. (2014) 64:1092–102. doi: 10.1016/j.jacc.2014.06.1179
11. Pasini E, Aquilani R, Testa C, Baiardi P, Angioletti S, Boschi F, et al. Pathogenic gut flora in patients with chronic heart failure. JACC Heart Fail. (2016) 4:220–7. doi: 10.1016/j.jchf.2015.10.009
12. Kamo T, Akazawa H, Suda W, Saga-Kamo A, Shimizu Y, Yagi H, et al. Dysbiosis and compositional alterations with aging in the gut microbiota of patients with heart failure. PLoS ONE. (2017) 12:e0174099. doi: 10.1371/journal.pone.0174099
13. Handl S, Dowd SE, Garcia-Mazcorro JF, Steiner JM, Suchodolski JS. Massive parallel 16S rRNA gene pyrosequencing reveals highly diverse fecal bacterial and fungal communities in healthy dogs and cats. FEMS Microbiol Ecol. (2011) 76:301–10. doi: 10.1111/j.1574-6941.2011.01058.x
14. Xenoulis PG, Palculict B, Allenspach K, Steiner JM, Van House AM, Suchodolski JS. Molecular-phylogenetic characterization of microbial communities imbalances in the small intestine of dogs with inflammatory bowel disease. FEMS Microbiol Ecol. (2008) 66:579–89. doi: 10.1111/j.1574-6941.2008.00556.x
15. Han D, Choi R, Hyun C. Canine pancreatic-specific lipase concentrations in dogs with heart failure and chronic mitral valvular insufficiency. J Vet Intern Med. (2015) 29:180–3. doi: 10.1111/jvim.12521
16. Pouchelon JL, Atkins CE, Bussadori C, Oyama MA, Vaden SL, Bonagura JD, et al. Cardiovascular-renal axis disorders in the domestic dog and cat: a veterinary consensus statement. J Small Anim Pract. (2015) 56:537–52. doi: 10.1111/jsap.12387
17. Borgarelli M, Haggstrom J. Canine degenerative myxomatous mitral valve disease: natural history, clinical presentation and therapy. Vet Clin North Am Small Anim Pract. (2010) 40:651–63. doi: 10.1016/j.cvsm.2010.03.008
18. Araki R, Iwanaga K, Ueda K, Isaka M. Intestinal complication with myxomatous mitral valve diseases in Chihuahuas. Front Vet Sci. (2021) 8:777579. doi: 10.3389/fvets.2021.777579
19. Thomas WP, Gaber CE, Jacobs GJ, Kaplen PM, Lombard CW, Mioise NS, et al. Recommendations for standards in transthoracic two-dimentional echocardiography in the dogb and cat. J Vet Intern Med. (1993) 7:247–52. doi: 10.1111/j.1939-1676.1993.tb01015.x
20. Cornell CC, Kittleson MD, Torre PD, Haggstorm J, Lombard CW, Pedersen HD, et al. Allometric scaling of M-Mode cardiac measurements in normal adult dogs. J Vet Intern Med. (2004) 18:311–21. doi: 10.1111/j.1939-1676.2004.tb02551.x
21. Malcolm EL, Visser LC, Phillips KL, Johnson LR. Diagnostic value of vertebral left atrial size as determined from thoracic radiographs for assessment of left atrial size in dogs with myxomatous mitral valve disease. J Am Vet Med Assoc. (2018) 253:1038–45. doi: 10.2460/javma.253.8.1038
22. Buchanan JW, Bucheler J. Vertebral scale system to measure canine heart size in radiographs. J Am Vet Med Assoc. (1995) 206:194–9.
23. Keene BW, Atkins CE, Bonagura JD, Fox PR, Haggstrom J, Fuentes VL, et al. ACVIM consensus guidelines for the diagnosis and treatment of myxomatous mitral valve disease in dogs. J Vet Intern Med. (2019) 33:1127–40. doi: 10.1111/jvim.15488
24. Bolyen E, Rideout JR, Dillon MR, Bokulich NA, Abnet CC, Al-Ghalith GA, et al. Reproducible, interactive, scalable and extensible microbiome data science using QIIME 2. Nat Biotechnol. (2019) 37:852–7. doi: 10.1038/s41587-019-0209-9
25. Borgarelli M, Savarino P, Crosara S, Santilli RA, Chiavegato D, Poggi M, et al. Survival characteristics and prognostic variables of dogs with mitral regurgitation attributable to myxomatous valve disease. J Vet Intern Med. (2008) 22:120–8. doi: 10.1111/j.1939-1676.2007.0008.x
26. Borgarelli M, Tarducci A, Zanatta R, Haggstorm J. Decreased systolic function and inadequate hypertrophy in large and small breed dogs with chronic mitral valve insufficiency. J Vet Intern Med. (2007) 21:61–7. doi: 10.1111/j.1939-1676.2007.tb02929.x
27. Moise NS, Moise NS, Scarlett J, Mohammed H, Yeager AE. Effect of breed and body weight on echocardiographic values in four breeds of dogs of differing somatotype. J Vet Intern Med. (1992) 6:220–4. doi: 10.1111/j.1939-1676.1992.tb00342.x
28. Urabe Y, Mann DL, Kent RL, Nakano K, Tomanek RJ, Carabello BA, et al. Cellular and ventricular contractile dysfunction in experimental canine mitral regurgitation. Circ Res. (1992) 70:131–47. doi: 10.1161/01.RES.70.1.131
29. della Torre PK, Kirby AC, Church DB, Malik R. Echocardiographic measurements in greyhounds, whippets and Italian greyhounds–dogs with a similar conformation but different size. Aust Vet J. (2000) 78:49–55. doi: 10.1111/j.1751-0813.2000.tb10361.x
30. Kanno N, Kuse H, Kawasaki M, Hara A, Kano R, Sasaki Y. Effects of pimobendan for mitral valve regurgitation in dogs. J Vet Med Sci. (2007) 69:373–7. doi: 10.1292/jvms.69.373
31. Sandri M, Dal Monego S, Conte G, Sgorlon S, Stefanon B. Raw meat based diet influences faecal microbiome and end products of fermentation in healthy dogs. BMC Vet Res. (2017) 13:65. doi: 10.1186/s12917-017-0981-z
32. Igarashi H, Maeda S, Ohno K, Horigome A, Odamaki T, Tsujimoto H. Effect of oral administration of metronidazole or prednisolone on fecal microbiota in dogs. PLoS ONE. (2014) 9:e107909. doi: 10.1371/journal.pone.0107909
33. Kim J, An JU, Kim W, Lee S, Cho S. Differences in the gut microbiota of dogs (Canis lupus familiaris) fed a natural diet or a commercial feed revealed by the Illumina MiSeq platform. Gut Pathog. (2017) 9:68. doi: 10.1186/s13099-017-0218-5
34. Guard BC, Mila H, Steiner JM, Mariani C, Suchodolski JS, Chastant-Maillard S. Characterization of the fecal microbiome during neonatal and early pediatric development in puppies. PLoS ONE. (2017) 12:e0175718. doi: 10.1371/journal.pone.0175718
35. Masuoka H, Shimada K, Kiyosue-Yasuda T, Kiyosue M, Oishi Y, Kimura S, et al. Transition of the intestinal microbiota of dogs with age. Biosci Microbiota Food Health. (2017) 36:27–31. doi: 10.12938/bmfh.BMFH-2016-021
36. Matumoto H, Baba E, Ishikawa H, Hodate Y. Studies in bacterial flora in the alimentary canal of dogs II. Development of the fecal bacterial flora in puppies. J Vet Med Sci. (1976) 38:485–94. doi: 10.1292/jvms1939.38.485
37. Mizukami K, Uchiyama J, Igarashi H, Murakami H, Osumi T, Shima A, et al. Age-related analysis of the gut microbiome in a purebred dog colony. FEMS Microbiol Lett. (2019) 366:8. doi: 10.1093/femsle/fnz095
38. Gao H, Liu S. Role of uremic toxin indoxyl sulfate in the progression of cardiovascular disease. Life Sci. (2017) 185:23–9. doi: 10.1016/j.lfs.2017.07.027
39. Ramezani A, Raj DS. The gut microbiome, kidney disease, and targeted interventions. J Am Soc Nephrol. (2014) 25:657–70. doi: 10.1681/ASN.2013080905
40. Doig CJ, Sutherland LR, Sandham JD, Fick GH, Verhoef M, Meddings JB. Increased intestinal permeability is associated with the development of multiple organ dysfunction syndrome in critically ill ICU patients. Am J Respir Crit Care Med. (1998) 158:444–51. doi: 10.1164/ajrccm.158.2.9710092
Keywords: Chihuahua, fractional shortening, intestinal complication, intestinal microbiota, myxomatous mitral valve disease
Citation: Araki R, Iwanaga K, Ueda K, Shima A, Ishihara G, Aizu M, Fukayama T and Isaka M (2022) Comparison of Intestinal Microbiota Between Healthy and MMVD Chihuahuas Using 16S rRNA Gene Amplicon Sequencing. Front. Vet. Sci. 9:846492. doi: 10.3389/fvets.2022.846492
Received: 31 December 2021; Accepted: 07 February 2022;
Published: 30 March 2022.
Edited by:
Zeki Yilmaz, Faculty of Veterinary Medicine, TurkeyReviewed by:
Kerem Ural, Adnan Menderes University, TurkeyCopyright © 2022 Araki, Iwanaga, Ueda, Shima, Ishihara, Aizu, Fukayama and Isaka. This is an open-access article distributed under the terms of the Creative Commons Attribution License (CC BY). The use, distribution or reproduction in other forums is permitted, provided the original author(s) and the copyright owner(s) are credited and that the original publication in this journal is cited, in accordance with accepted academic practice. No use, distribution or reproduction is permitted which does not comply with these terms.
*Correspondence: Mitsuhiro Isaka, bS1pc2FrYUByYWt1bm8uYWMuanA=
Disclaimer: All claims expressed in this article are solely those of the authors and do not necessarily represent those of their affiliated organizations, or those of the publisher, the editors and the reviewers. Any product that may be evaluated in this article or claim that may be made by its manufacturer is not guaranteed or endorsed by the publisher.
Research integrity at Frontiers
Learn more about the work of our research integrity team to safeguard the quality of each article we publish.