- 1Forensic Medicine and Toxicology Department, Faculty of Veterinary Medicine, Zagazig University, Zagazig, Egypt
- 2Poultry Department, Agriculture Faculty, Zagazig University, Zagazig, Egypt
- 3Department of Clinical Laboratory Sciences, Turabah University College, Taif University, Taif, Saudi Arabia
- 4School of Biosciences and Veterinary Medicine, University of Camerino, Matelica, Italy
- 5Department of Poultry and Fish Diseases, Faculty of Veterinary Medicine, Alexandria University, Alexandria, Egypt
This study evaluated the immunotoxic effects of thallium (Tl) in Nile tilapia fingerlings and the recovery role of dietary Astragalus membranaceus polysaccharides (ASs). An 8-week experiment was designed where 180 fishes were randomly and equally assigned in triplicates into the six groups: the control group (CNT) was reared in unpolluted water and fed a commercial diet, two groups were fed a well-balanced commercial diet plus 1.5 and 3.0 g AS/kg diet (AS0.15 and AS0.30), respectively, the fourth group was exposed to a sublethal dose of Tl (41.9 μg l−1) [equal to 1/10 of 96-h lethal concentration 50 (LC50)], and the last two groups were fed 0.15 and 0.3% AS, respectively, and concurrently exposed to Tl (41.9 μg l−1) (AS0.15+Tl and AS0.30+Tl). Fish hematobiochemical parameters, serum immunity [nitric oxide, total immunoglobulin M (IgM) levels, and lysozyme activity], transcription of hepatic interferon-γ (IFN-γ), interleukin-1β (IL-1β), and tumor necrosis factor-α (TNF-α), and resistance to Aeromonas hydrophila (A. hydrophila) were assessed. Hematobiochemical parameters and serum immune indices were significantly decreased in the fish group exposed to sublethal Tl concentration compared to the CNT group. Furthermore, Tl exposure significantly induced overexpression of IL-1β, TNF-α, and IFN-γ genes (4.22-, 5.45-, and 4.57-fold higher, respectively) compared to CNT values. Tl exposure also increased the cumulative mortality (%) in Nile tilapia challenged with A. hydrophila. Remarkably, the groups fed AS0.15+Tl and AS0.30+Tl significantly ameliorated all the aforementioned parameters, but did not reach CNT values. Our findings suggest the possible immunomodulating roles of dietary AS in recovering the immunotoxic effects of Tl in Nile tilapia. We can conclude that dietary AS would be useful for maintaining the immunity of Nile tilapia fingerlings.
Introduction
Thallium (Tl) is a rare-earth element and is regarded as an extremely toxic heavy metal (1). Tl can occur in two forms: monovalent Tl(I) and trivalent Tl(III) forms (2). Currently, Tl gained particular interest among numerous trace metals due to its attractive applications as a product obtained from the mining of coal, glass manufacture, electric materials, and several insecticides (3). Nonetheless, the unhygienic release of Tl over large geographic areas led to increased pollution of the aquatic ecosystems, including water, bottom sediments, and aquatic organisms. The primary Tl sources are closely related to effluents from coal-based industries (processing and mining of coal and coal-firing plants) and refining sulfide-rich natural rocks or sediments (4). As a result, it can be considered an apparent environmental contaminant to aquatic environments.
Several literature reports elucidated that monovalent Tl toxicity in aquatic organisms inhabiting the freshwater environments ranged between μg/l and mg/l (5–11). Importantly, it is crucial to determine the levels of Tl able to result toxic in the exposed organisms. It was found that Tl level ranging between 1 and 60 ppm that slowly killed fish (12). Moreover, the 72-h lethal concentration 50 (LC50) of Tl is 10–15 mg l−1 for Oncorhynchus mykiss, 0.03 mg l−1 for Salmo salar, 2–4 mg l−1 for invertebrates and aquatic insects, and 0.4 mg l−1 for tadpoles. Likewise, the 96-h LC50 for Danio rerio (D. rerio) was 870 μg l−1 (13).
Hou et al. observed that the exposure of D. rerio to environmentally relevant concentrations of Tl considerably increased the expression of heat shock protein 70 (HSP70) and metallothionein 2 (Mt2) genes, elevated superoxide dismutase activity in the liver tissue, and increased the Na+/K+-ATPase in the gill tissues (14). The same authors also found that Tl exposure induced severe histopathological changes in gonads, gills, and liver tissues. Further, Li et al. illustrated that exposure of D. rerio to 0.1 μg l−1 Tl (I) for 15 consecutive days produced physiological alterations in ammonia nitrogen excretion, oxygen consumption rate, and ammonia quotient, indicating rapid increase in protein metabolism and anaerobic energy utilization (15).
Among the wide range of Chinese herbs usually used as traditional medicines, Astragalus membranaceus (AM) is considered to be endowed with immunomodulatory, hepatoprotective, and antioxidant activities (16, 17). Its principal functional bioactive compounds are polysaccharides (PSs), flavonoids, phenolic acids, alkaloids, and saponins (18). PSs are the most crucial bioactive compounds in AM with numerous pharmacological advantages (19, 20). Reports have proven that injection of Astragalus membranaceus polysaccharides (ASs) considerably increased the transcription of immune-related genes in Cyprinus carpio (C. carpio) (21). Furthermore, Sun et al. showed that AS noticeably enhanced the immune responses of sea cucumber (22).
Dietary AS enhanced the growth parameters (initial and final weight, weight gain, feed intake, specific growth rate, and feed conversion ratio) and immune parameters (phagocytic, respiratory burst, and bactericidal and plasma lysozyme activities) of Nile tilapia (Oreochromis niloticus) (23), modulated the gut microbiome of sea cucumber (24, 25), and increased the immunity (phagocytic, respiratory burst, lysozyme and inducible nitric oxide synthase, and content activity) of largemouth bass, enhancing their resistance to Aeromonas hydrophila (A. hydrophila) (26). Furthermore, dietary AS improved the antioxidant status [superoxide dismutase (SOD), glutathione peroxidase, and total antioxidant capacity] of large yellow croaker and turbot (23, 27), enhancing the resistance of juvenile crucian carps (28) and grass carps (29) to A. hydrophila, as well as the antiviral immunity of zebrafish (30).
Several attempts to find natural and safe feed supplements to attenuate the toxicity signs in fishe have been proposed so far. In this sense, it was found that supplementing diets with AS also induced hepatoprotection and increased the antioxidant status of C. carpio exposed to carbon tetrachloride (CCl4) (31). AM has also been regarded as an important natural antistress feed additive for Perca flavescens (32) and Lepomis macrochirus (33). Notably, AM extract powder increased the survivability of Nile tilapia exposed to low-temperature stress (34). Our recently published study elucidated that AS could enhance the growth, hematobiochemical indices (alanine aminotransferase, creatinine, total cholesterol, triglycerides, glucose, and cortisol), hepatic antioxidant status [catalase, SOD enzyme activity, and malondialdehyde (MDA) content], and transcription of apoptosis-related and Hsp70 genes in Nile tilapia fingerlings exposed to sublethal Tl toxicity (35).
Herein, we have assessed the potential effectiveness of dietary AS in the mitigation of hematobiochemical and immune indices, transcription of immune related, and disease resistance of Nile tilapia exposed to sublethal Tl toxicity.
Materials and Methods
Thallium and Astragalus membranaceus Polysaccharide
Thallium (I) nitrate (99.9% purity) was obtained from Sigma-Aldrich (St. Louis, Mosby, USA; CAS Number: 10102-45-1). AS (60% purity) was obtained from the local market (El-Ahmadeya Company, Egypt). The analysis showed that AS was composed of arabinose, α-1,4-glucan, α-1,6-glucan, galacturonic acid, rhamnose, and galactose. Analytical grade chemicals and reagents were utilized in this study.
Animals and Housing
Nile tilapia fingerlings (body weight = 13 g ± 1.00) were purchased from a fish hatchery (Abbassa, Egypt). Fishes were adapted for 15 days in 500 L rearing tanks. During the adaptation period, fisher were fed control (basal) well-balanced diet (Aller Aqua Company, Egypt). This diet contains all the nutritional requirements for better growth of Nile tilapia (36). Fisher were fed three times daily (5% of their biomass). The ingredients and constituents of ration and its chemical composition are given in Table 1.
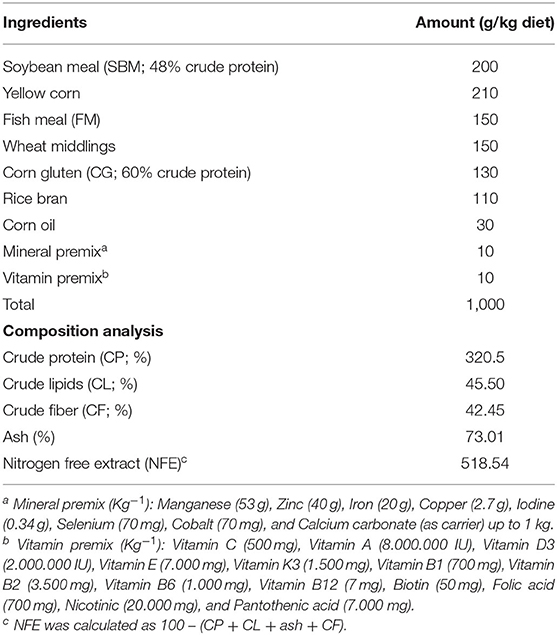
Table 1. Ration constituents (g kg−1 diet) and proximate composition analysis of the control diet (35, 55) used in the experiment.
During the adaptation period, physical and chemical measurements of the rearing water were 26.5–28.5°C for temperature, 6.50–7.5 mg l−1 for dissolved oxygen, 7.45–7.6 for pH, 0.01–0.02 mg l−1 for nitrite, 0.01–0.03 mg l−1 for nitrates, and 0.01–0.02 mg l−1 for unionized ammonia. The photoperiod was stable as 12/12 h as light and dark cycle.
Diets and Experimental Design
Two different inclusion percentages of AS were used with the ingredients of the control diet 1.5 and 3.0 g AS/kg diet and defined as AS0.15 and AS0.30, respectively. All the constituents were pelletized to form 1.5 mm pellets using carboxymethylcellulose (used in fish nutrition as a pelleting agent), allowed to dry in the air for 1 day at 25°C, and then stored in plastic bags at 4°C in the refrigerator. On the other hand, the 96-h LC50 of thallium (Tl) in Nile tilapia fingerlings used during this study was previously calculated as 419 μg l−1 in our recently published study (35).
One hundred eighty fishes were randomly and equally assigned in triplicate to the six experimental groups (n = 30); each group was reared in a glass aquarium (sized 80 × 80 × 100 cm) containing 90 L dechlorinated water. The control group (CNT) was reared in an aquarium filled with clean unpolluted water and fed the basal diet only. The AS0.15 and AS0.30 groups were reared in clean water and fed basal diet supplemented with 0.15 or 0.30% AS. The group exposed to sublethal Tl concentration was fed the basal diet and exposed to 1/10th of the 96-h LC50 of Tl (41.9 μg l−1) and Tl was dispersed and completely dissolved in deionized water to prevent further toxicity to exposed fishes. The last two groups were fed the basal diet supplemented with 0.15 and 0.30% AS and concurrently exposed to Tl (41.9 μg l−1) and were defined as AS0.15+Tl and AS0.30+Tl, respectively. To prevent Tl degradation in the rearing water and keep the exposure level constant, Tl was replenished every 96-h interval. The whole experiment lasted for 8 weeks.
Sampling Procedures (Blood and Liver Tissues)
All the fishes were kept fasted 1 day prior to sampling. After this, they were anesthetized by MS-222 (tricaine methanesulfonate) in a dose rate of 200 mg l−1 to decrease the handling stress (37). Seven fishes per replicate (21 fishes per group) were sampled. Blood was sampled from the fish's caudal vessels (about 1 ml per fish and pooled from three fishes). Blood samples were separated into two parts: three pooled samples mixed with sodium citrate into Eppendorf tubes and used for the hematobiochemical analyses, while the other four pooled samples were left at room temperature and then centrifuged at 3,000 × g for 20 min to collect serum for immunological indices. Serum samples were then stored at −20°C. Liver specimens (100 mg per fish) were sampled after the aseptic opening of the fishes and then quickly frozen in a liquid nitrogen tank. Liver samples were then stored at −80°C for quantitative reverse transcription-PCR (qRT-PCR) analysis.
Hematobiochemical Parameters
Red blood cells (RBCs) and white blood cells (WBCs) were counted by an automatic cell counter (Hospitex Hema Screen 18, Italy). Values of hemoglobin (Hb) and packed cell volume (PCV) were estimated according to (38). The mean corpuscular volume (MCV) was measured according to (39). Total protein (TP) and albumin (ALB) contents were quantified by using the BioMed Diagnostic Colorimetric Kits (Egy Chem for Lab Technology, Egypt) (40, 41). Globulin (GLO) values were calculated by the difference between TP and ALB values.
Immunological Assays
Serum nitric oxide (NO) was evaluated spectrophotometrically using commercial kits (BioChain, Incorporation, USA) following the manufacturer's instructions. Serum myeloperoxidase (MPO) activity was assayed according to the method proposed by Kumari and Sahoo (42). Serum total immunoglobulin M (IgM) was determined by using ELISA kits specific for fish (MyBioSource Corporation, San Diego, USA; Cat. No. MBS035038) (43, 44). Serum lysozyme (LYZ) activity was assessed by the turbidimetric assay using lyophilized Micrococcus lysodeikticus cells (Sigma-Aldrich, St. Louis, Mosby, USA) as a substrate (45). One LYZ activity unit was defined as the decrease of 0.001 of OD520 min−1.
Gene Transcription
Ribonucleic acid extraction was done from 50 mg of the collected liver tissues (100 mg) using TRIzol Reagent (iNtRON Biotechnology, South Korea). The purity and concentration of the extracted RNA were assessed by using a ND-2000 spectrophotometer (NanoDrop 2000, Wilmington, Delaware, USA). 3 μg (0.15 μg/μl) RNA was reverse-transcribed. Complementary DNA (cDNA) synthesis was done by using reverse transcription kits (Quantitect®, Qiagen, Germany). The steps displayed during the processes of RNA extraction and cDNA synthesis were achieved in accordance with the directions obtained from the manufacturer. The primer sequences (forward and reverse sense) and the National Center for Biotechnology Information (NCBI) GenBank accession numbers of interleukin-1β (IL-1β), interferon-γ (INF-γ), tumor necrosis factor-α (TNF-α), and β-actin (β-actin) are given in Table 2.
The qRT-PCR analysis was done by using a Rotor-Gene Q instrument and the QuantiTect® SYBR® Green PCR Kit (Qiagen, Germany). The thermocycling conditions were determined as 95°C for 10 min, followed by 40 cycles of 95°C for 15 s and 60°C for 30 s and 72°C for 30 s. A melt-curve analysis was done to confirm the specificity of PCR products. The relative expression profile of each target gene to β-actin (reference gene) was assessed using the 2-ΔΔCt method (46).
Challenge Test
Aeromonas hydrophila used in the challenge test was previously isolated from diseased Nile tilapia and identified in our previous study (47). The bacterium was cultured in Tryptic Soy Broth (TSB) (Merck, Germany) at 28°C for 24 h (48). The bacterial suspension was then centrifuged at 5,000 × g for 5 min at 25°C. The supernatant was discarded and the pellets were washed 3 times using sterile phosphate-buffered saline (PBS) and then resuspended into the same solution and adjusted to McFarland Standard No. 0.5 at a wavelength of 600 nm, which corresponds to a concentration of 1.5 × 108 cells ml−1. The bacterial concentration of 108 cells ml−1 was previously reported as sufficient to kill more than half of the challenged Nile tilapia (49).
Another set of the experimental groups (30 fishes/group) were received an intraperitoneal (IP) injection of 0.1 ml of cell suspension containing 1.5 × 107 cells ml−1 (50). The mortalities were daily recorded for 2 weeks following the challenge. The relative percentage survival (RPS) and cumulative mortality rate (CMR) of fishes were calculated according to previously published formulas (51).
Statistical Analysis
Results were statistically analyzed by one-way ANOVA using SPSS (SPSS version 16.0, SPSS Incorporation, USA). The Tukey's multiple comparisons were used as post-hoc to compare means between the groups. The statistical significances were approved when P < 0.05. Data were expressed as means ± SEM.
Results
Hematobiochemical Parameters
Erythrogram variables such as RBCs count and PCV values exhibited no significant differences among all the experimental groups. However, Tl exposure caused a significant decline (P < 0.05) in Hb content and increase in MCV value compared with the CNT group. Dietary supplementation with AS in both the AS0.15+Tl and AS0.30+Tl groups induced a significant modulation in Hb and MCV values compared with the fish group exposed to sublethal Tl concentration, but not completely restored to the CNT values (Table 3).
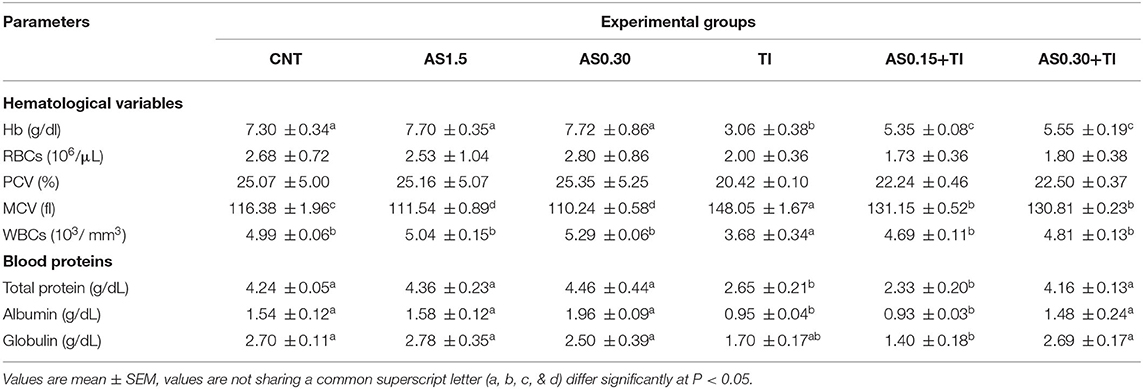
Table 3. Effect of dietary supplementation with Astragalus membranaceus polysaccharides (AS) levels (0.15 and 0.30%) and/or exposure to sublethal thallium (Tl) toxicity [1/10th lethal concentration 50 (LC50); 41.9 μg/l] for 8 weeks on the hematological variables and blood protein profile of Nile tilapia.
When compared with the values of the CNT group, Tl exposure elicited a significant decline (P < 0.05) in the WBCs count (Table 3). In both the AS0.15+Tl and AS0.30+Tl groups, there were significant improvements in WBCs counts that attained the CNT values.
Total protein, ALB, and GLO values were significantly decreased the Tl-exposed group in comparison to the CNT levels. Dietary supplementation with AS in the AS0.30+Tl group induced complete restoration of these values to be non-significantly differed from the CNT values.
Immunological Indexes
Sublethal Tl toxicity showed an alteration of the immunity indexes, including LYZ, IgM, MPO, and NO values (Figure 1). On the other hand, the AS supplemented groups elicited no differences from the CNT group, except a significant enhancement of IgM content was recorded the AS0.30 group. Serum IgM and NO contents were significantly improved the AS0.15+Tl and AS0.30+Tl groups, where they were completely restored to the CNT levels. Serum LYZ activity was restored in the AS0.30+Tl group to reach the CNT levels (Figure 1). On the other hand, the altered MPO levels recorded in the Tl-exposed group were not improved by AS supplementation (Figure 1).
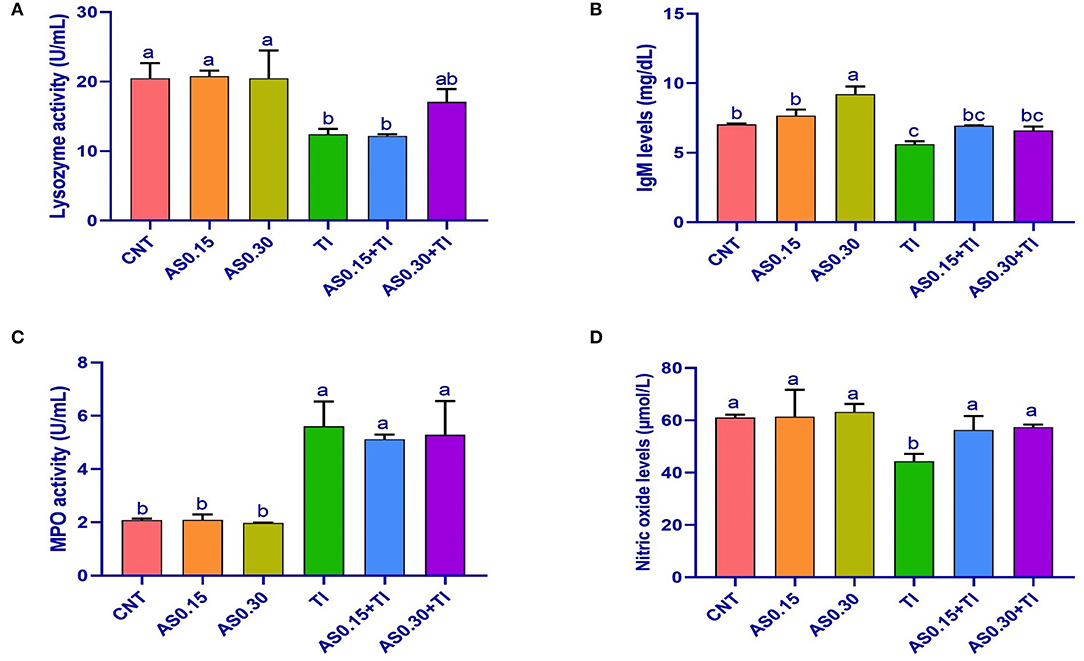
Figure 1. Serum lysozyme activity (A), immunoglobulin M (IgM) levels (B), myeloperoxidase activity (C), and nitric oxide levels (D) in Nile tilapia fed diets supplemented with Astragalus membranaceus polysaccharides (AS) levels (0.15 and 0.30%) and/or exposed to sublethal thallium (Tl) toxicity [1/10th lethal concentration 50 (LC50); 41.9 μg/l] for 8 weeks. Values are mean ± SE and bars not sharing a common superscript letter (a, b, c, and d) differ significantly at P < 0.05.
Gene Transcription Results
Thallium exposure revealed significant overexpression of IL-1β, TNF-α, and IFN-γ genes to be 4.22-, 5.45-, and 4.57-fold higher than the CNT values, respectively (Figure 2). The observed overexpression of TNF-α was not modulated by AS in the AS0.15+Tl and AS0.30+Tl groups and showed no significant differences compared with the group exposed to sublethal Tl concentration. Alternatively, a significant modulation was observed in the expression pattern of IL-1β in the AS0.15+Tl and AS0.30+Tl groups, particularly in the AS0.30+Tl group as it was completely recovered to attain the normal CNT range. As well, the expression of the IFN-γ gene was not mitigated in the AS0.15+Tl group, while modulated only in the AS0.30+Tl group, but did not attain the normal CNT range (Figure 2).
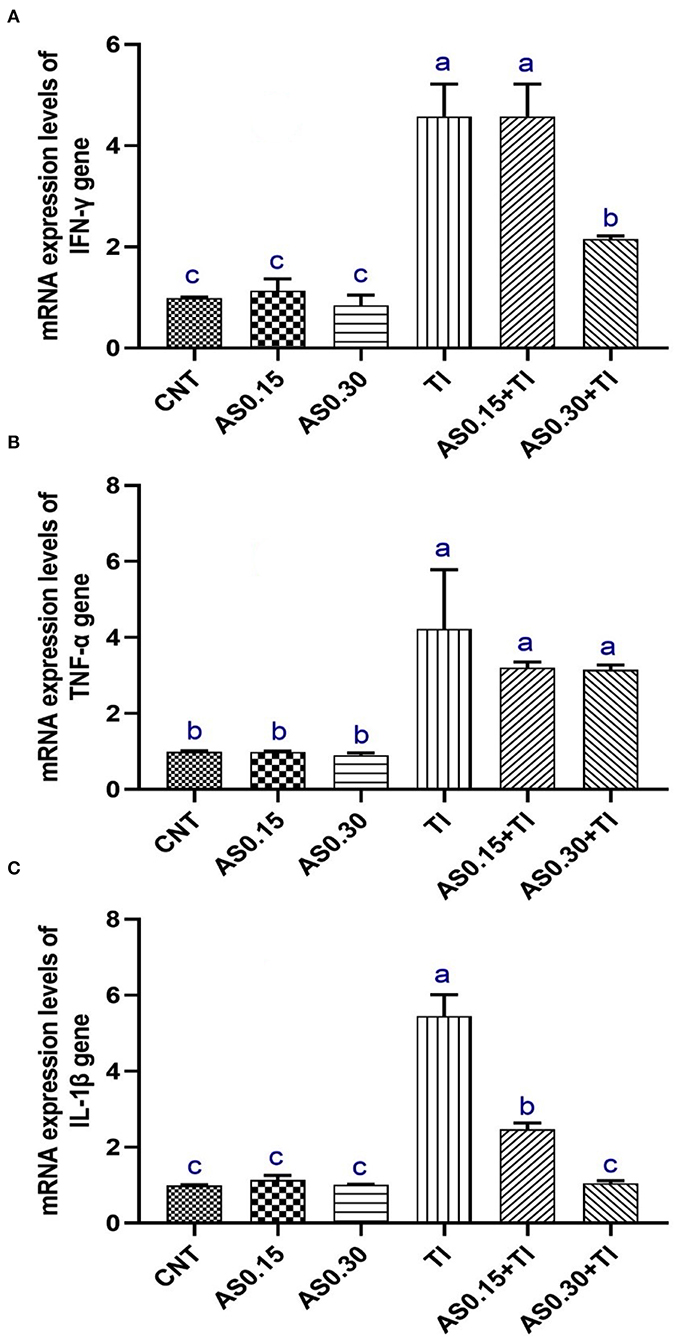
Figure 2. Messenger RNA (mRNA) expression pattern of interferon-γ (INF-γ) (A), tumor necrosis factor-α (TNF-α) (B), and interleukin-1β (IL-1β) (C) in liver of Nile tilapia fed diets supplemented with Astragalus membranaceus polysaccharides (AS) levels (0.15 and 0.30%) and/or exposed to sub-lethal thallium (Tl) toxicity (1/10th LC50; 41.9 μg/l) for 8 weeks. Values are mean ± SE and bars not sharing a common superscript letter (a, b, c, and d) differ significantly at P < 0.05.
Fish Resistance Against Aeromonas hydrophila
The highest CMR (%) after challenge with pathogenic A. hydrophila was observed in the Tl-exposed group (32.33%) followed by the AS0.15+Tl, AS0.30+Tl, and CNT groups (13.33, 10.0, and 6.66%, respectively). On the other hand, no mortalities were recorded in the AS0.15 and AS0.30 groups (Table 4). The challenged fishes exhibited various degrees of escape reflex and abnormal swimming movements. Furthermore, Tl-exposed fishes showed severe signs of infection such as hemorrhagic patches on the abdomen with focal hemorrhage and some ulcerations. The severity of these symptoms was alleviated in the groups whose diets were supplemented with AS.

Table 4. Effect of dietary supplementation with Astragalus membranaceus polysaccharides (AS) levels (0.15 and 0.30%) and/or exposure to thallium (Tl) toxicity (1/10th LC50; 41.9 μg/l) for 8 weeks on the disease resistance of Nile tilapia challenged with Aeromonas hydrophila.
Discussion
Fish hematology is an important tool for monitoring the fish heath condition and biomarkers for diagnosing fish toxicity (52). In this study, Tl exposure significantly decreased Hb content and increased MCV values. These anemic signs may be due to Tl-induced oxidative stress (14), which is characterized by overproduction of reactive oxygen species (ROS) and a consequent negative impact on Hb concentration (53). In addition, Tl exposure significantly decreased the WBCs, which probably elucidate the immunosuppressant effects of Tl exposure. These effects occur due to the toxic effects of Tl on blood constituents. Similar findings were described after fishes' exposure to pollutants (54, 55).
Notably, dietary supplementation with AS in both the AS0.15+Tl and AS0.30+Tl groups partially restored Tl-induced toxicity on the hematobiochemical parameters. It is well known that herbal extracts have a significant number of polyphenols, which might enrich the cells with iron that has a crucial function in improving the functions of RBCs (56, 57). A possible interpretation of the Hb concentrations improvement may result from the antioxidant effect of AS, which could stabilize Hb level and protect the cell membrane of erythrocytes from the oxidative damage of Tl. Moreover, the improvement of WBCs may be due to the preservation of the leukocyte redox state and the immunomodulatory impact of dietary AS, which may increase the proliferation of leukocytes (55). However, the actual mechanisms involved in enhancement of hematobiochemical parameters of fishes fed dietary AS are still unclear to date.
Blood proteins are bioindicators of the nutritional, immunological, and health conditions of fishes. Moreover, they are principally involved during the exposure of fishes to stressors, as these animals require more energy to overwhelm the effects of these stressors (58). Tl exposure significantly decreased TP, ALB, and GLO levels compared to the CNT group. These findings may be attributed to hepatorenal damage after Tl exposure, which may subsequently increase the vascular leakage and worsen renal glomerular filtration or impair liver functions, which ultimately lead to the inability of the liver to synthesize ALB. On the other hand, TP, ALB, and GLO levels were increased in the AS-supplemented groups. In a similar sense, Jia et al. reported that dietary supplementation with AS (1.5 and 3 g/kg) considerably increased the reduced serum TP and ALB values in CCl4-exposed common carps (31). Also, dietary AS increased serum TP and ALB in large yellow croakers (59). Increased serum proteins are closely related to the enhanced immunity by AS feeding.
Lysozyme, MPO, total IgM, and NO are from the immune parameters of fish (60–62). In this study, the fish exposure to sub-lethal Tl toxicity caused a marked decrease in serum LYZ activity and NO and IgM levels in the exposed Nile tilapia, indicating immunosuppressive effects of Tl. Similar findings were also reported after exposure of Nile tilapia to T1 (35) and deltamethrin (35, 63). A recent literature also reported that exposure of fishes to some aquatic toxicants induced immunotoxicity (64).
On the other hand, dietary AS can modulate the immune parameters in the AS0.15+Tl and AS0.30+Tl groups. Dietary AM can also increase the total immunoglobulins and serum LYZ activities in Nile tilapia (65). Plasma LYZ activity was also elevated in Nile tilapia fishes fed AS-supplemented diets (23). Increased serum LYZ activity was also noticed in sea cucumber-based diets supplemented with AS or AM (25). Furthermore, dietary AS enhanced NO production, increased the phagocytic activity of head kidney macrophages and serum LYZ activities in large yellow croakers (59).
Fish cytokines are molecules involved in initiating and regulating the innate immune responses (66, 67). Tumor necrosis factors (TNFs) are implicated in the inflammation process, apoptosis, and cell proliferation (68). Moreover, TNF-α is the most immunologically significant molecule in the TNF family (66). The pro-inflammatory cytokines such as IL-1β, TNF-α, and IFN-γ are from fish cytokines with pivotal immune functions (69–71).
The pro-inflammatory cytokines are molecules that initiate and regulate the fish innate immunity and their increase is indicative of an inflammatory process (72–74). TNF-α is involved in cell proliferation inflammation apoptosis (66) via promoting the release of other cytokines, activating lymphocytes and neutrophils, increasing the endothelial cells permeability, and controlling the metabolic activities of cells (71). Similar functions were reported for IL-1β in fish, where it can regulate the immune and inflammatory responses (75). INF-γ is a key mediator in enhancing MHC-I and II histocompatibility complexes expressions and is involved in the fish immune response (70). In this study, the expressions of IFN-γ, IL-1β, and TNF-α genes were upregulated in liver of Nile tilapia response to sublethal T1 exposure. Similar results were observed in brain tissue of Nile tilapia exposed to sublethal T1. These genes were also upregulated in response to other contaminants associated with inflammation and immunotoxicity such as clethodim in zebrafish embryos (76) and bifenthrin in zebrafish larvae (77, 78) and Nile tilapia (35).
Reports showed that AS could activate the proliferation of B cells and macrophages and increase cytokine production (18). The effects of AS on the expression of cytokines may be linked to the ability of AS in the modulation of the host immunity via Toll-like receptor 4 (TLR4)-mediated myeloid differentiation factor 88 (MyD88)-dependent signaling pathway (79). Moreover, Sun et al. proposed that dietary AS is involved in regulating the hepatic immune responses in turbot via TLRs/nuclear factor-kappa B (NF-κB) signaling pathway (27). We suggest conducting future study and several experiments to elucidate the actual mechanisms of dietary AS in attenuation of the overexpressed genes after sub-lethal Tl toxicity.
The increased survival rates after challenge with pathogenic A. hydrophila were observed in the AS-supplemented groups compared with Tl-exposed fishes. The immune-suppressive effects after Tl exposure such as leukocytopenia, hypoproteinemia, decreased serum LYZ, NO, and total IgM will also reduce the resistance of fishes against challenged pathogens; therefore, it will increase the mortalities after challenge with A. hydrophila. On the other hand, dietary AS improved the disease resistance of the treated fishes. Dietary AM reduced the mortality rates of Nile tilapia after the challenge with A. hydrophila (65). Moreover, Wang et al. reported that supplementing diets with AM or AS considerably lowered the CMR% in sea cucumber challenged with Vibrio splendidus (25). Besides, Lin et al. also indicated that dietary AS decreased mortalities of largemouth bass challenged with A. hydrophila compared with the CNT fed the basal diet (26). Recently, Shi et al. showed that dietary AS increased the protection of grass carp tissues after a challenge with A. hydrophila (29). These effects may be attributed to the powerful antimicrobial effects of AS (18) and the immunomodulatory effects of AM (80).
Conclusion
Sublethal thallium (Tl) toxicity induced immunosuppression in the exposed Nile tilapia fingerlings. It induced leukocytopenia, hypoproteinemia, and hypoalbuminemia in the exposed fishes. Moreover, a significant decline in the serum immune indices such as LYZ, NO, and total IgM levels was also noticed. Tl exposure induced upregulation of IL-1β, TNF-α, and INF-γ genes in liver tissues, which are cytokines with pivotal immune functions in the fishes. Additionally, Tl increased the CMR (%) of Nile tilapia after challenge with pathogenic A. hydrophila. Collectively, these results draw the overall adverse impacts of sublethal Tl toxicity on fishes' immunity and disease resistance. Oppositely, the immunomodulatory results of AS positively ameliorated the negative impacts of Tl on the exposed fishes. At the same time, the valuable effects of diets supplemented with AS and their effects against the immunosuppressive effects of sub-lethal Tl toxicity partially diminished Tl toxicity compared to the control values. By this way, for better awareness about the mechanisms by which AS could mitigate Tl toxicity in fishes, additional studies are recommended.
Data Availability Statement
The authors acknowledge that the data presented in this study must be deposited and made publicly available in an acceptable repository, prior to publication. Frontiers cannot accept a article that does not adhere to our open data policies.
Ethics Statement
The animal study was reviewed and approved by all experiments in the current study have been done in line with the Local Experimental Animal Care Committee guidelines and approved by the Institutional Ethics Committee of Zagazig University, Egypt.
Author Contributions
MF, MA, SK, WE, and EE-H contributed to the conception and design of the study. MF, MA, SK, and EE-H performed the experiments. MA and CM performed the statistical analyses. ADC, MA, and TI were involved in the project administration and funding acquisition. HA-L and MA wrote the first draft of the manuscript. All the authors have contributed to manuscript revision, read, and approved the submitted version of the manuscript.
Funding
This study was funded by the Taif University Researchers Supporting Project number (TURSP-2020/134), Taif University, Taif, Saudi Arabia.
Conflict of Interest
The authors declare that the research was conducted in the absence of any commercial or financial relationships that could be construed as a potential conflict of interest.
Publisher's Note
All claims expressed in this article are solely those of the authors and do not necessarily represent those of their affiliated organizations, or those of the publisher, the editors and the reviewers. Any product that may be evaluated in this article, or claim that may be made by its manufacturer, is not guaranteed or endorsed by the publisher.
Acknowledgments
The authors would like to acknowledge and thank the Taif University Researchers Supporting Project number (TURSP-2020/134), Taif University, Taif, Saudi Arabia.
References
1. Kazantzis G. Thallium in the environment and health effects. Environ Geochem Health. (2000) 22:275–80. doi: 10.1023/A:1006791514080
2. Peter ALJ, Viraraghavan T. Thallium: a review of public health and environmental concerns. Environ Int. (2005) 31:493–501. doi: 10.1016/j.envint.2004.09.003
3. Zhang Z, Zhang B, Long J, Zhang X, Chen G. Thallium pollution associated with mining of thallium deposits. Sci China: Earth Sci. (1998) 41:75–81. doi: 10.1007/bf02932424
4. Cheam V, Garbai G, Lechner J, Rajkumar J. Local impacts of coal mines and power plants across Canada. I Thallium Waters Sediments Water Quality Res J. (2000) 35:581–608. doi: 10.2166/wqrj.2000.034
5. Borgmann U, Cheam V, Norwood WP, Lechner J. Toxicity and bioaccumulation of thallium in Hyalella Azteca, with comparison to other metals and prediction of environmental impact. Environ Pollut. (1998) 99:105–14. doi: 10.1016/S0269-7491(97)00181-4
6. Lapointe D, Couture P. Accumulation and effects of nickel and thallium in early-life stages of fathead minnows (Pimephales Promelas). Ecotoxicol Environ Saf. (2010) 73:572–8. doi: 10.1016/j.ecoenv.2010.01.004
7. Lin TS, Meier P, Nriagu J. Acute toxicity of thallium to Daphnia Magna and Ceriodaphnia Dubia. Bull Environ Contam Toxicol. (2005) 75:350–5. doi: 10.1007/s00128-005-0760-6
8. Pickard J, Yang R, Duncan B, McDevitt CA, Eickhoff C. Acute and sublethal toxicity of thallium to aquatic organisms. Bull Environ Contam Toxicol. (2001) 66:94–101. doi: 10.1007/s001280000210
9. Rickwood CJ, King M, Huntsman-Mapila P. Assessing the fate and toxicity of thallium I and thallium iii to three aquatic organisms. Ecotoxicol Environ Saf. (2015) 115:300–8. doi: 10.1016/j.ecoenv.2014.12.024
10. Song J, Qiao L, Ji L, Ren B, Hu Y, Zhao R, et al. Toxic Responses of Zebrafish (Danio Rerio) to Thallium and Deltamethrin Characterized in the Electrocardiogram. Chemosphere. (2018) 212:1085–94. doi: 10.1016/j.chemosphere.2018.09.014
11. Zitko V, Carson WV, Carson WG. Thallium: occurrence in the environment and toxicity to fish. Bull Environ Contam Toxicol. (1975) 13:23–30. doi: 10.1007/BF01684859
12. Zitko V, Carson WV. Accumulation of thallium in clams and mussels. Bull Environ Contam Toxicol. (1975) 14:530–3. doi: 10.1007/BF01683366
13. Tatsi K, Turner A, Handy RD, Shaw BJ. The acute toxicity of thallium to freshwater organisms: implications for risk assessment. Sci Total Environ. (2015) 536:382–90. doi: 10.1016/j.scitotenv.2015.06.069
14. Hou LP, Yang Y, Shu H, Ying GG, Zhao JL, Chen YB, et al. Changes in histopathology, enzyme activities, and the expression of relevant genes in Zebrafish (Danio Rerio) following long-term exposure to environmental levels of thallium. Bull Environ Contam Toxicol. (2017) 99:574–81. doi: 10.1007/s00128-017-2176-5
15. Li B, Zhang J, Ma J, Qiao L, Ren B, Chen M, et al. The continuous physiological changes of Zebrafish (Danio Rerio) based on metabolism under controlled thallium stress. Chemosphere. (2020) 240:124974. doi: 10.1016/j.chemosphere.2019.124974
16. Fu J, Wang Z, Huang L, Zheng S, Wang D, Chen S, et al. Review of the botanical characteristics, phytochemistry, and pharmacology of Astragalus Membranaceus (Huangqi). Phytother Res. (2014) 28:1275–83. doi: 10.1002/ptr.5188
17. Farag MR, Elhady WM, Ahmed SYA, Taha HSA, Alagawany M. Astragalus polysaccharides alleviate tilmicosin-induced toxicity in rats by inhibiting oxidative damage and modulating the expressions of Hsp70, Nf-Kb and Nrf2/Ho-1 pathway. Res Vet Sci. (2019) 124:137–48. doi: 10.1016/j.rvsc.2019.03.010
18. Zheng Y, Ren W, Zhang L, Zhang Y, Liu D, Liu Y, et al. Review of the pharmacological action of astragalus polysaccharide. Front Pharmacol. (2020) 11:349. doi: 10.3389/fphar.2020.00349
19. Jin M, Zhao K, Huang Q, Shang P. Structural features and biological activities of the polysaccharides from Astragalus Membranaceus. Int J Biol Macromol. (2014) 64:257–66. doi: 10.1016/j.ijbiomac.2013.12.002
20. Li S, Qi Y, Ren D, Qu D, Sun Y. The structure features and improving effects of polysaccharide from Astragalus Membranaceus on antibiotic-associated diarrhea. Antibiotics. (2019) 9:8. doi: 10.3390/antibiotics9010008
21. Yuan C, Pan X, Gong Y, Xia A, Wu G, Tang J, et al. Effects of astragalus polysaccharides (Aps) on the expression of immune response genes in head kidney, gill and spleen of the common carp, Cyprinus Carpio L. Int Immunopharmacol. (2008) 8:51–8. doi: 10.1016/j.intimp.2007.10.009
22. Sun Y, Jin L, Wang T, Xue J, Liu G, Li X, et al. Polysaccharides from Astragalus Membranaceus promote phagocytosis and superoxide anion (O2-) production by coelomocytes from sea cucumber Apostichopus Japonicus in Vitro. Comp Biochem Physiol C Toxicol Pharmacol. (2008) 147:293–8. doi: 10.1016/j.cbpc.2007.11.003
23. Zahran E, Risha E, Abdelhamid F, Mahgoub HA, Ibrahim T. Effects of dietary astragalus polysaccharides (Aps) on growth performance, immunological parameters, digestive enzymes, and intestinal morphology of Nile Tilapia (Oreochromis Niloticus). Fish Shellfish Immunol. (2014) 38:149–57. doi: 10.1016/j.fsi.2014.03.002
24. Song X, Feng Z, Zhang Y, Zhu W. Regulation of dietary astragalus polysaccharide (Aps) supplementation on the non-specific immune response and intestinal microbiota of sea cucumber Apostichopus Japonicus. Fish Shellfish Immunol. (2019) 94:517–24. doi: 10.1016/j.fsi.2019.09.049
25. Wang T, Sun Y, Jin L, Xu Y, Wang L, Ren T, et al. Enhancement of non-specific immune response in sea cucumber (Apostichopus Japonicus) by Astragalus Membranaceus and its polysaccharides. Fish Shellfish Immunol. (2009) 27:757–62. doi: 10.1016/j.fsi.2009.09.002
26. Lin SM, Jiang Y, Chen YJ, Luo L, Doolgindachbaporn S, Yuangsoi B. Effects of astragalus polysaccharides (Aps) and chitooligosaccharides (Cos) on growth, immune response and disease resistance of juvenile largemouth bass, Micropterus Salmoides. Fish Shellfish Immunol. (2017) 70:40–7. doi: 10.1016/j.fsi.2017.08.035
27. Sun Y, Wang X, Zhou H, Mai K. He G. Dietary Astragalus polysaccharides ameliorates the growth performance, antioxidant capacity and immune responses in turbot (Scophthalmus Maximus L) fish. Shellfish Immunol. (2020) 99:603–8. doi: 10.1016/j.fsi.2020.02.056
28. Wu S. Dietary Astragalus Membranaceus polysaccharide ameliorates the growth performance and innate immunity of juvenile crucian carp (Carassius Auratus). Int J Biol Macromol. (2020) 149:877–81. doi: 10.1016/j.ijbiomac.2020.02.005
29. Fei S, Zhijie L, Minxuan Y, Feng L, Fanbin Z, Lijuan Z, et al. Astragalus polysaccharides mediate the immune response and intestinal microbiota in grass carp (Ctenopharyngodon Idellus). Aquaculture. (2021) 2021: 736205. doi: 10.1016/j.aquaculture.2020.736205
30. Li Y, Ran C, Wei K, Xie Y, Xie M, Zhou W, et al. The effect of astragalus polysaccharide on growth, gut and liver health, and anti-viral immunity of Zebrafish. Aquaculture. (2021) 540:736677. doi: 10.1016/j.aquaculture.2021.736677
31. Jia R, Cao L, Xu P, Jeney G, Yin G. In Vitro and in Vivo hepatoprotective and antioxidant effects of astragalus polysaccharides against carbon tetrachloride-induced hepatocyte damage in common carp (Cyprinus Carpio). Fish Physiol Biochem. (2012) 38:871–81. doi: 10.1007/s10695-011-9575-z
32. Elabd H, Wang H-P, Shaheen A, Yao H, Abbass A. Feeding Glycyrrhiza Glabra (Liquorice) and Astragalus Membranaceus (Am) alters innate immune and physiological responses in yellow perch (Perca Flavescens). Fish Shellfish Immunol. (2016) 54:374–84. doi: 10.1016/j.fsi.2016.04.024
33. Elabd H, Wang HP, Shaheen A, Yao H, Abbass A. Astragalus Membranaceus (Am) enhances growth performance and antioxidant stress profiles in bluegill sunfish (Lepomis Macrochirus). Fish Physiol Biochem. (2016) 42:955–66. doi: 10.1007/s10695-015-0188-9
34. Wu F, Yang C, Wen H, Zhang C, Jiang M, Liu W, et al. Improving low-temperature stress tolerance of tilapia, Oreochromis Niloticus: a functional analysis of Astragalus Membranaceus. J World Aquac Soc. (2019) 50:749–62. doi: 10.1111/jwas.12586
35. Farag MR, Alagawany M, Khalil SR, Moustafa AA, Mahmoud HK, Abdel-Latif HMR. Astragalus Membranaceus polysaccharides modulate growth, hemato-biochemical indices, hepatic antioxidants, and expression of Hsp70 and apoptosis-related genes in Oreochromis Niloticus exposed to sub-lethal thallium toxicity. Fish Shellfish Immunol. (2021) 118:251–60. doi: 10.1016/j.fsi.2021.09.009
36. Jobling M. Fish in aquaculture environments. In: Huntingford F, Jobling M, Kadri S, editors Aquaculture and Behavior. Oxford: Wiley-Blackwell. (2012). doi: 10.1002/9781444354614.ch2
37. Smith DA, Smith SA, Holladay SD. Effect of Previous Exposure to tricaine methanesulfonate on time to anesthesia in hybrid tilapias. J Aquat Anim Health. (1999) 11:183–6. doi: 10.1577/1548-8667(1999)011 <0183:EOPETT>2.0.CO;2.2.0.CO;2
38. Collier HB. Standardization of blood haemoglobin determinations. Can Med Assoc J. (1944) 50:550–2.
40. Doumas BT, Bayse DD, Borner K, Carter RJ, Elevitch F, Garber CC, et al. A candidate reference method for determination of total protein in serum. Ii Test for Transferability Clin Chem. (1981) 27:1651–4. doi: 10.1093/clinchem/27.10.1651
41. Doumas BT, Watson WA, Biggs HG. Albumin standards and the measurement of serum albumin with bromcresol green. Clin Chim Acta. (1971) 31:87–96. doi: 10.1016/0009-8981(71)90365-2
42. Kumari J. Sahoo PK. Dietary beta-1,3 glucan potentiates innate immunity and disease resistance of Asian catfish, Clarias Batrachus (L) J Fish Dis. (2006) 29:95–101. doi: 10.1111/j.1365-2761.2006.00691.x
43. Siwicki A, Anderson D. An easy spectrophotometric assay for determining total protein and immunoglobulin levels in fish sera: correlation to fish health. Tech Fish Immunol. (1993) 3:23–30.
44. Cuesta A, Meseguer J. Esteban MA. Total serum immunoglobulin M levels are affected by immunomodulators in Seabream (Sparus Aurata L). Veterinary Immunol Immunopathol. (2004) 101:203–10. doi: 10.1016/j.vetimm.2004.04.021
45. Stolen JS, Fletcher TC, Anderson DP, Roberson BS, van Muiswinkel WB. Techniques in Fish Immunology. Fair Haven, NJ: SOS Publications (1990). 220 p.
46. Livak KJ, Schmittgen TD. Analysis of relative gene expression data using real-time quantitative Pcr and the 2(-Delta Delta C(T)) method. Methods. (2001) 25:402–8. doi: 10.1006/meth.2001.1262
47. Abdel-Latif HMR, Khafaga AF. Natural co-infection of cultured nile tilapia Oreochromis Niloticus with Aeromonas Hydrophila and Gyrodactylus Cichlidarum experiencing high mortality during summer. Aquac Res. (2020) 51:1880–92. doi: 10.1111/are.14538
48. El-Son MAM, Nofal MI, Abdel-Latif HMR. Co-infection of Aeromonas Hydrophila and Vibrio Parahaemolyticus isolated from diseased farmed striped mullet (Mugil Cephalus) in Manzala, Egypt – a case report. Aquaculture. (2021) 530:735738. doi: 10.1016/j.aquaculture.2020.735738
49. Mohammadi G, Rafiee G, El Basuini MF, Van Doan H, Ahmed HA, Dawood MAO, et al. Oregano (Origanum Vulgare), St John's-Wort (Hypericum Perforatum), and Lemon Balm (Melissa Officinalis) extracts improved the growth rate, antioxidative, and immunological responses in Nile Tilapia (Oreochromis Niloticus) infected with Aeromonas Hydrophila. Aquacult Rep. (2020) 18:100445. doi: 10.1016/j.aqrep.2020.100445
50. Mohammadi G, Rafiee G, El Basuini MF, Abdel-Latif HMR, Dawood MAO. The Growth performance, antioxidant capacity, immunological responses, and the resistance against Aeromonas Hydrophila in Nile Tilapia (Oreochromis Niloticus) fed pistacia vera hulls derived polysaccharide. Fish Shellfish Immunol. (2020) 106:36–43. doi: 10.1016/j.fsi.2020.07.064
51. Abdel-Latif HMR, Abdel-Tawwab M, Khafaga AF. Dawood MAO. Dietary origanum essential oil improved antioxidative status, immune-related genes, and resistance of common carp (Cyprinus Carpio L) to aeromonas hydrophila infection. Fish Shellfish Immunol. (2020) 104:1–7. doi: 10.1016/j.fsi.2020.05.056
52. Fazio F. Fish hematology analysis as an important tool of aquaculture: a review. Aquaculture. (2019) 500:237–42. doi: 10.1016/j.aquaculture.2018.10.030
53. Bloom JC, Schade AE, Brandt JT. Toxic Responses of the Blood. Casarett and Doull's Toxicology: The Basic Science of Poisons. New York, NY: McGraw-Hill Education. (2012).
54. Dawood M, El-Salam Metwally A, Elkomy AH, Gewaily MS, Abdo SE, Abdel-Razek MAS, et al. The impact of menthol essential oil against inflammation, immunosuppression, and histopathological alterations induced by chlorpyrifos in Nile Tilapia. Fish Shellfish Immunol. (2020) 102:316–25. doi: 10.1016/j.fsi.2020.04.059
55. Farag MR, Alagawany M, Taha HSA, Ismail TA, Khalil SR, Abou-Zeid SM. Immune response and susceptibility of Nile Tilapia fish to Aeromonas Hydrophila infection following the exposure to bifenthrin and/or supplementation with Petroselinum Crispum essential oil. Ecotoxicol Environ Saf. (2021) 216:112205. doi: 10.1016/j.ecoenv.2021.112205
56. Ahmadifar E, Yousefi M, Karimi M, Fadaei Raieni R, Dadar M, Yilmaz S, et al. Benefits of dietary polyphenols and polyphenol-rich additives to aquatic animal health: an overview. Rev Fish Sci Aquacult. (2021) 29:478–511. doi: 10.1080/23308249.2020.1818689
57. Dawood MAO, Abdel-Tawwab M, Abdel-Latif HMR. Lycopene reduces the impacts of aquatic environmental pollutants and physical stressors in fish. Rev Aquacult. (2020) 12:2511–26. doi: 10.1111/raq.12455
58. Ismael NEM, Abd El-Hameed SAA, Salama AM, Naiel MAE, Abdel-Latif HMR. The effects of dietary clinoptilolite and chitosan nanoparticles on growth, body composition, haemato-biochemical parameters, immune responses, and antioxidative status of Nile Tilapia exposed to imidacloprid. Environ Sci Pollut Res Int. (2021) 28:29535–50. doi: 10.1007/s11356-021-12693-4
59. Zhang W, Zhang M, Cheng A, Hao E, Huang X, Chen X. Immunomodulatory and antioxidant effects of astragalus polysaccharide liposome in large yellow croaker (Larimichthys Crocea). Fish Shellfish Immunol. (2020) 100:126–36. doi: 10.1016/j.fsi.2020.03.004
60. Magnadottir B. Innate immunity of fish (overview). Fish Shellfish Immunol. (2006) 20:137–51. doi: 10.1016/j.fsi.2004.09.006
61. Mashoof S, Criscitiello MF. Fish immunoglobulins. Biology. (2016) 5:45. doi: 10.3390/biology5040045
62. Saurabh S, Sahoo PK. Lysozyme: an important defence molecule of fish innate immune system. Aquac Res. (2008) 39:223–39. doi: 10.1111/j.1365-2109.2007.01883.x
63. Mahmoud EA, El-Sayed BM, Mahsoub YH, El-Murr AEI, Neamat-Allah ANF. Effect of Chlorella Vulgaris enriched diet on growth performance, hemato-immunological responses, antioxidant and transcriptomics profile disorders caused by deltamethrin toxicity in Nile Tilapia (Oreochromis Niloticus). Fish Shellfish Immunol. (2020) 102:422–9. doi: 10.1016/j.fsi.2020.04.061
64. Bols NC, Brubacher JL, Ganassin RC, Lee LE. Ecotoxicology and innate immunity in fish. Dev Comp Immunol. (2001) 25:853–73. doi: 10.1016/S0145-305X(01)00040-4
65. Ardó L, Yin G, Xu P, Váradi L, Szigeti G, Jeney Z, et al. Chinese herbs (Astragalus Membranaceus and Lonicera Japonica) and boron enhance the non-specific immune response of Nile Tilapia (Oreochromis Niloticus) and resistance against Aeromonas Hydrophila. Aquaculture. (2008) 2008:26–33. doi: 10.1016/j.aquaculture.2007.12.022
66. Sakai M, Hikima J-i, Kono T. Fish cytokines: current research and applications. Fisheries Science. (2021) 87:1–9. doi: 10.1007/s12562-020-01476-4
67. Secombes CJ, Wang T, Hong S, Peddie S, Crampe M, Laing KJ, et al. Cytokines and innate immunity of fish. Dev Comp Immunol. (2001) 25:713–23. doi: 10.1016/S0145-305X(01)00032-5
68. Goetz FW, Planas JV, MacKenzie S. Tumor necrosis factors. Dev Comp Immunol. (2004) 28:487–97. doi: 10.1016/j.dci.2003.09.008
69. Savan R, Sakai M. Genomics of fish cytokines. Comp Biochem Physiol Part D Genomics Proteom. (2006) 1:89–101. doi: 10.1016/j.cbd.2005.08.005
70. Robertsen B. The interferon system of teleost fish. Fish Shellfish Immunol. (2006) 20:172–91. doi: 10.1016/j.fsi.2005.01.010
71. Zou J, Secombes CJ. The Function of Fish Cytokines. Biology (Basel). (2016) 5:23. doi: 10.3390/biology5020023
72. Galeotti M, Manzano M, Beraldo P, Bulfon C, Rossi G, Volpatti D, et al. Ultrastructural and biomolecular detection of rickettsiales-like organisms in tissues of rainbow trout with red mark syndrome. J Fish Dis. (2017) 40:907–17. doi: 10.1111/jfd.12571
73. Galeotti M, Ronza P, Beraldo P, Bulfon C, Magi GE, Manzano M, et al. First report of red mark syndrome (Rms) in farmed rainbow trout in Slovenia. J Fish Dis. (2017) 40:1935–9. doi: 10.1111/jfd.12652
74. Melotti P, Roncarati A, Angellotti L, Dees A, Magi GE, Mazzini C, et al. Effects of rearing density on rainbow trout welfare, determined by plasmatic and tissue parameters. Ital J Anim Sci. (2004) 3:393–400. doi: 10.4081/ijas.2004.393
75. Buonocore F, Forlenza M, Randelli E, Benedetti S, Bossu P, Meloni S, et al. Biological activity of sea bass (Dicentrarchus Labrax L) recombinant interleukin-1beta. Mar Biotechnol. (2005) 7:609–17. doi: 10.1007/s10126-004-5131-5
76. Xiong G, Zou L, Deng Y, Meng Y, Liao X, Lu H. Clethodim exposure induces developmental immunotoxicity and neurobehavioral dysfunction in zebrafish embryos. Fish Shellfish Immunol. (2019) 86:549–58. doi: 10.1016/j.fsi.2018.12.002
77. Park J, Kim C, Jeon HJ, Kim K, Kim M-J, Moon J-K, et al. Developmental toxicity of 3-Phenoxybenzoic Acid (3-Pba) and endosulfan sulfate derived from insecticidal active ingredients: abnormal heart formation by 3-Pba in zebrafish embryos. Ecotoxicol Environ Saf. (2021) 224:112689. doi: 10.1016/j.ecoenv.2021.112689
78. Jin M, Zhang X, Wang L, Huang C, Zhang Y, Zhao M. Developmental toxicity of bifenthrin in embryo-larval stages of zebrafish. Aquatic Toxicology. (2009) 95:347–54. doi: 10.1016/j.aquatox.2009.10.003
79. Zhou L, Liu Z, Wang Z, Yu S, Long T, Zhou X, et al. Astragalus polysaccharides exerts immunomodulatory effects via Tlr4-mediated Myd88-dependent signaling pathway in vitro and in vivo. Sci Rep. (2017) 7:44822. doi: 10.1038/srep44822
Keywords: toxicity, immunity, hematology, attenuation, herbal medicines, diseases
Citation: Farag MR, Alagawany M, Khalil SR, El-Hady EW, Elhady WM, Ismail TA, Marini C, Di Cerbo A and Abdel-Latif HMR (2022) Immunosuppressive Effects of Thallium Toxicity in Nile Tilapia Fingerlings: Elucidating the Rescue Role of Astragalus membranaceus Polysaccharides. Front. Vet. Sci. 9:843031. doi: 10.3389/fvets.2022.843031
Received: 24 December 2021; Accepted: 20 April 2022;
Published: 09 June 2022.
Edited by:
Arturo Anadón, Complutense University of Madrid, SpainReviewed by:
Huaping Zhu, Chinese Academy of Fishery Sciences, ChinaGuihong Fu, Hunan Agricultural University, China
Copyright © 2022 Farag, Alagawany, Khalil, El-Hady, Elhady, Ismail, Marini, Di Cerbo and Abdel-Latif. This is an open-access article distributed under the terms of the Creative Commons Attribution License (CC BY). The use, distribution or reproduction in other forums is permitted, provided the original author(s) and the copyright owner(s) are credited and that the original publication in this journal is cited, in accordance with accepted academic practice. No use, distribution or reproduction is permitted which does not comply with these terms.
*Correspondence: Mayada R. Farag, ZHIubWF5YWRhcmYmI3gwMDA0MDtnbWFpbC5jb20=; Mahmoud Alagawany, ZHIubWFobW91ZC5hbGFnd2FueSYjeDAwMDQwO2dtYWlsLmNvbQ==; Alessandro Di Cerbo, YWxlc3NhbmRyby5kaWNlcmJvJiN4MDAwNDA7dW5pY2FtLml0