- 1College of Animal Science and Technology, Northeast Agricultural University, Harbin, China
- 2State Key Laboratory for Managing Biotic and Chemical Threats to the Quality and Safety of Agro-Products, Institute of Animal Science & Veterinary, Zhejiang Academy of Agricultural Science, Hangzhou, China
- 3Tropical Crop Genetic Resource Research Institute, Chinese Academy of Tropical Agricultural Sciences, Haikou, China
- 4Wenzhou Vocational College of Science and Technology, Wenzhou, China
- 5Institute of Animal Science & Veterinary, Hainan Academy of Agricultural Science, Haikou, China
The objective of the present study was to evaluate the effects of hydroxylated lecithin on growth performance, serum enzyme activity, hormone levels related to lipid metabolism and meat quality in Jiangnan White goslings. Six hundred 1-day-old goslings were randomly divided into five treatments with six replicates and 20 for each replicate. The control group (CG) was fed the basal diet, while the experimental group was fed the basal diet with 50, 100, 200 mg/kg hydroxylated lecithin and 100 mg/kg soy lecithin (HLG50, HLG100, HLG200, and LG100, respectively) in the form of powder. Feed and water were provided ad libitum for 32 days. Compared with the CG, (a) the average daily feed intake was higher (P < 0.05) in HLG100, the final body weight and average daily gain were higher (P < 0.05), and the feed conversion ratio was lower in the HLG200; (b) the alanine aminotransferase, malate dehydrogenase, leptin, glucagon, thyroid hormone, Triiodothyronine contents in the HLG200 were lower (P < 0.05); (c) The breast muscle water holding capacity was higher (P < 0.05) in groups with hydroxylated lecithin, the breast muscle shear force and fiber diameter were lower (P < 0.05) in the HLG100; (d) the inositic acid, intramuscular fat, phospholipid contents were higher (P < 0.05), the triglyceride content was lower (P < 0.05) in HLG100 of the breast muscle; (e) the relative expression of sterol regulatory element-binding protein-1 genes were higher (P < 0.05) in the treated groups of muscles, the phosphorylase kinase gamma subunit 1 gene expression was shown an opposite trend. In comparison with LG100, (a) the feed conversion ratio was lower (P < 0.05) in HLG200; (b) the alanine aminotransferase and adiponectin contents were higher (P < 0.05), the malondialdehyde and free fatty acid contents were lower (P < 0.05) in HLG200; (c) the water holding capacity and intramuscular fat contents in the breast and leg muscles were higher (P < 0.05) in HLG200. The hydroxylated lecithin concentration of 200 mg/kg improved the growth performance, serum enzyme activity, hormone levels related to lipid metabolism, and the meat quality of Jiangnan White goslings.
Introduction
Hydroxylated lecithin is a substance that introduces hydroxyl groups into the fatty acid double bonds of concentrated and purified soy lecithin (1, 2). It is considered a safe feed additive for its enriched content of phosphatidylcholine, lecithin, unsaturated fatty acids, choline and inositol. Moreover, lecithin is generally recognized as an essential nutrient for maintaining various physiological metabolism of animals (2–4). In livestock production, hydroxylated lecithin can be used as a nutritional supplement, emulsifier, humectant, and thickener. Its emulsification and hydrophilic properties are preferred to those in soy lecithin (5). Both hydroxylated lecithin and soy lecithin contain hydrophobic and hydrophilic groups in their molecular structures, forming stable emulsions of immiscible phases in feeds, which can be acted as surfactants (2, 6). Hydroxylated lecithin and soy lecithin can further disperse lipids entering the intestinal tract of poultry, increase the contact area between intestinal villous membrane and lipids and fat-soluble vitamins, and promote the lipids digestion, absorption and transfer (7, 8). In the initial stage of growth and development, due to the digestive system imperfect development of poultry, the secretion of bile and digestive enzymes is insufficient, making the lipid substances in the feed not be fully digested and absorbed (9–12). The emulsification function of hydroxylated lecithin and soy lecithin contributes to lipid digestion and absorption, and it was found that soy lecithin can improve the utilization of animal oil in feed for poultry (13, 14). Both hydroxylated lecithin and soy lecithin have a fatty flavor and good poultry appetizers (2). Moderate lecithin could increase poultry feed intake and daily weight gain, and decrease the feed conversion ratio of broilers; the daily weight gain was positively correlated with the soy lecithin content (15, 16). The hydroxylated lecithin and soy lecithin are rich in unsaturated fatty acids, which impact the synthesis and metabolism of fatty acids in poultry (2, 15). Lecithin can promote poultry to digest and absorb excess cholesterol under the lecithin cholesterol acyltransferase action increasing the polyunsaturated fatty acids deposition (as a percentage) (17, 18), making poultry meat more recognized by consumers. At present, the application of soy lecithin is still dominant in poultry production, and few hydroxylated lecithin applications have been reported. In this study, we based on comparing the structure and function between soy lecithin and hydroxylated lecithin, and it was hypothesized that hydroxylated lecithin would be superior to soy lecithin in promoting poultry production and was verified in this experiment.
The lipid content has a critical influence on meat quality, especially the flavor and tenderness, in poultry (19, 20). Jiangnan White goose is a fast-growing commercial goose cultivated by Jiangsu Lihua Animal Husbandry Co., Ltd. in China, and its lipid metabolism in the chick stage has a vital influence on its overall production performance. This study intends to (a) study the effects of hydroxylated lecithin on the growth performance, serum enzymes activity, hormone levels related to lipid metabolism, and meat quality of Jiangnan White gosling by adding hydroxylated lecithin to the Jiangnan White gosling diets, (b) discuss the feasibility and appropriate contents of hydroxylated lecithin in the production of Jiangnan White gosling, and (c) preliminarily explore the impact mechanism of the hydroxylated lecithin on production performance of Jiangnan White goslings, to further develop and use the hydroxylated lecithin, and accumulate experience and theoretical basis for safe and reliable additives.
Methods and Materials
The Chinese guidelines for animal welfare conducted this study and with the animal welfare standards of the College of Animal Science and Technology, Northeast Agricultural University (NEAU-2018-0232).
Experimental Material
Jiangnan White goslings: 1-day-old, with an average initial body weight 120.00 ± 5.00 g, provided by Jiangsu Lihua Animal Husbandry Co., Ltd.
Hydroxylated lecithin: EINECS No. 232-307-2, CAS No. 8029-76-3, with purity 99%, in the form of powder added to the basal diets, purchased from Sichuan Huayuan Shengtai Biotechnology Co., Ltd.
Lecithin: EINECS No. 232-307-2, CAS No. 8002-43-5, with purity 99%, in the form of powder added to the basal diets, purchased from Sichuan Huayuan Shengtai Biotechnology Co., Ltd.
Enzyme-linked immunoassay kits were purchased from Shanghai Sangon Biotechnology Co., Ltd.
Experiment Design and Sample Collection
Six hundred 1-day-old female healthy Jiangnan White goslings, with body weight 120.00 ± 5.00 g, were randomly divided into five treatments with six replicates, with 20 goslings for each replicate. The control group (CG) was fed the basal diet (BD), in the granule form, while the treated groups were fed the BD with 50, 100, and 200 mg/kg hydroxylated lecithin and 100 mg/kg lecithin in the form of powder (HLG50, HLG100, HLG200, LG100, respectively). The composition (kg/100 kg) of the BD was shown in Table 1, and the fatty acid composition (g/100 g) of BD and lecithin was shown in Table 2. Feed and water were provided ad libitum for 32 days. The gosling house temperature was kept at 30°C in the first week, then was reduced gradually over the next 9 days to the outside temperature, the humidity at 63%, and the light was applied 24 h a day with 15-watt fluorescent lighting on 1–16 days. Moreover, the house temperature and humidity were kept pace with the outside environment, with natural light during the day and 8-watt fluorescent lighting at night on 17–32 days. The other feeding management and immunization procedures, including ventilation of the birdhouse and vaccine injection for goslings, were carried out following the goose farm guidelines.
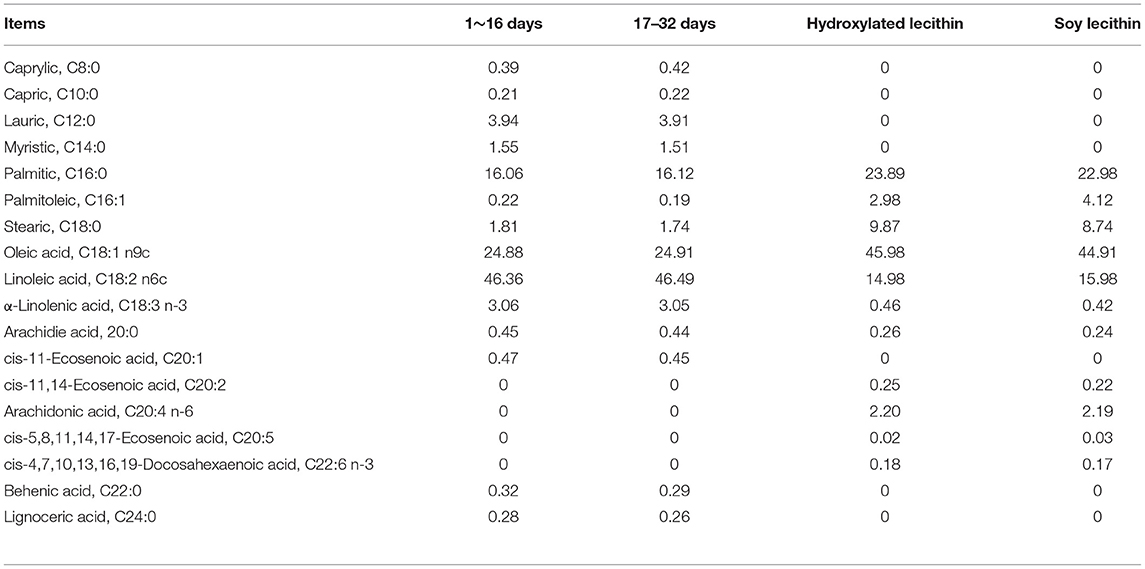
Table 2. The fatty acid composition of the basal experimental diets and lecithin for Jiangnan White goslings (g/100 g).
Feed intake and weight gain of goslings were recorded during the experiment period for calculating the average daily feed intake (ADFI), average daily gain (ADG), and feed conversion ratio (FCR). On the 32nd day, one gosling was randomly chosen from each replicate for sampling. Blood (10 mL) was collected with disposable vacuum blood collection tubes from the neck vein of each gosling. After resting the blood for 15 min, it was centrifuged at 3,000 rpm for 15 min to obtain the serum, divided into Eppendorf tubes and stored at −20°C refrigerators for biochemical indicators testing. Right side breast and leg muscles (10 g) were collected into 15 mL centrifuge tubes filled with formalin and stored at room temperature for section hematoxylin and eosin (HE) staining to determine the muscle fiber diameter and density. In addition, the pH value (Meat Ph direct measuring instrument PH-STAR, Germany), water holding capacity (HP607 meat hydraulic tester, China) and shear force (C-LM4 Muscle Tenderness Meter, China) were determined on breast and leg muscles. Ten gram from each muscle were collected into plastic packaging bags and stored until analyses at −20°C of the inosinic acid (HPLC, Chromaster®, Japan), intramuscular fat (Soxhlet Extractor, China), triglycerides and phospholipid (Gas Chromatograph-Mass Spectrometer, the U.S.A.). The inter-assay variation coefficients of inosinic acid, intramuscular fat, triglycerides and phospholipid were 4.66, 4.98, 4.20, 4.22%, respectively; and the intra-assay variation coefficients were 4.55, 4.66, 4.78, 4.82%, respectively. Two gram of muscles were collected into freezing tubes and stored at −80°C refrigerators for RNA extraction.
Serum Index Determination
The kits information of serum biochemical indexes in this study was shown in Table 3. And all kits were purchased from Shanghai Sangon Biotechnology Co., Ltd.
The total cholesterol (TC), alanine aminotransferase (ALT), glucose (GLU) were measured with a fully automatic biochemical analyzer, CG3040B, Changchun Guangji Medical Instrument Co., Ltd. In addition, the malate dehydrogenase (MDH), adiponectin (ADPN), leptin (LEP), triiodothyronine (T3), thyroid hormone (T4), thyrotropin-releasing hormone (TRH), lipoprotein lipase (LPL), free fatty acid (FFA), glucagon (GLC), insulin (INS), triglycerides (TG), and malondialdehyde (MDA) were determined with the multifunctional marker (SuPerMax 3100, China). The relevant determination operations were carried out according to the kit instructions.
Quantification of Genes With Real-Time PCR
Fifty milligram collected breast and leg muscles with 1 mL TRIzol reagent (Invitrogen, CA) was thoroughly ground in liquid nitrogen and transferred into a 1.5 mL Eppendorf tube for further analysis. Total RNA was extracted with an RNA extraction kit (Vazyme Cat. RC112-01, 50 rxn), and levels of relative expression of sterol regulatory element-binding protein-1 (SREBP-1) and phosphorylase kinase gamma subunit 1 (PHKG1) genes were determined with real-time PCR. Primers for SREBP-1 and PHKG1 were selected according to the geese sequences registered in NCBI and designed by using Beacon Designer 7, glyceraldehyde-3-phosphate dehydrogenase (GAPDH) was used as an internal control. The primer sequences were shown in Table 4. The total real-time PCR system volume was 10 μL, and the reaction system was as follows: SYBR Green Mix 4.4 μL, upstream and downstream primers 0.3 μL each, and cDNA 5 μL. The real-time PCR procedure was as follows: 95°C for 10 min, one cycle, 95°C for 10 s, 60°C for 34 s, and 40 cycles. The Ct values of the target genes and the internal reference genes were measured, and the relative gene expression levels were calculated by the 2−ΔΔCt method. Each test was repeated at least three times.
Statistical Analysis
Statistical analyses were conducted using SAS 9.4 statistics software. Data were expressed as mean ± SEM. Statistical comparisons of different treatments were performed using one-way ANOVA. Each replicate pen served as an experimental unit for the growth performance statistical analyses. One gosling from each replicate served as an experimental unit for the serum biochemistry indexes and meat quality statistical analyses. Duncan's multiple range tests determined significant differences among the treatment means at P < 0.05.
Results
Effects on the Growth Performance
As shown in Table 5, the average initial body weight (AIBW) was no significant difference (P > 0.05) among groups. The final body weight (FBW) and ADG were higher (P < 0.05) in HLG200 than those in CG and HLG50. The ADFI was higher (P < 0.05) in the treated groups with hydroxylated lecithin and soy lecithin than that in CG, it was higher (P < 0.05) in HLG100 than that in HLG200. The FCR in HLG200 was lower (P < 0.05) than that in CG, HLG50 and LG100.
Effects on Serum Biochemistry Indexes Related to Lipid Metabolism
Effects on Serum Enzymes Activity Related to Lipid Metabolism
Enzyme activity related to lipid metabolism data were summarized in Table 6. The ALT contents in treated groups were lower (P < 0.05) than those in CG, and the contents in LG100 were lower (P < 0.05) than those in groups with hydroxylated lecithin. The LPL contents in HLG100, HLG200, and LG100 were higher (P < 0.05) than those in CG and HLG50. The MDH contents in treated groups, especially in HLG200, were lower (P < 0.05) than those in CG, and the contents in HLG200 were lower than those in LG100.

Table 6. Effects of hydroxylated lecithin on enzymes activity related to lipid metabolism in serum of Jiangnan White goslings.
Effects on Serum Hormone Levels Related to Lipid Metabolism
The effects of hydroxylated lecithin on hormone levels related to lipid metabolism were shown in Table 7. The ADPN contents in treated groups were higher (P < 0.05) than those in CG, and it was higher (P < 0.05) in HLG200 than that in LG100. The LEP contents were lower (P < 0.05) in HLG100, HLG200, and LG100 than those in CG and HLG50. The GLC contents in treated groups were lower (P < 0.05) than those in CG, and it was lower (P < 0.05) in HLG200 than that in LG100. The INS contents in treated groups were higher (P < 0.05) than those in CG, and it was higher (P < 0.05) in LG100 than that in HLG100. The T4 and T3 contents in treated groups were lower (P < 0.05) than those in CG, and they were lower (P < 0.05) in HLG200 than those in LG100. The TRH contents in treated groups were lower (P < 0.05) than those in CG, and it was lower (P < 0.05) in HLG100 than that in LG100.
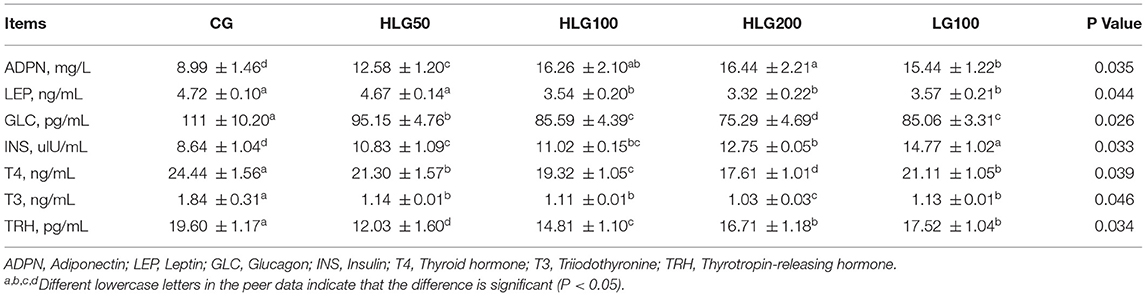
Table 7. Effects of hydroxylated lecithin on hormone levels related to lipid metabolism in serum of Jiangnan White goslings.
Effects on Serum Other Biochemical Indicator Levels Related to Lipid Metabolism
The other biochemical indicator levels related to lipid metabolism data were summarized in Table 8. The GLU contents in treated groups were lower (P < 0.05) than those in CG, and it was lower (P < 0.05) in HLG50 and HLG200 than that in LG100. The TC and TG contents in treated groups were lower (P < 0.05) than those in CG, and the TG content was lower (P < 0.05) in HLG200 than that in LG100. The MDA contents in treated groups were lower (P < 0.05) than those in CG, and it was lower (P < 0.05) in groups with hydroxylated lecithin than that in LG100.The FFA contents in treated groups were higher (P < 0.05) than those in CG, and it was lower (P < 0.05) in groups with hydroxylated lecithin than that in LG100.
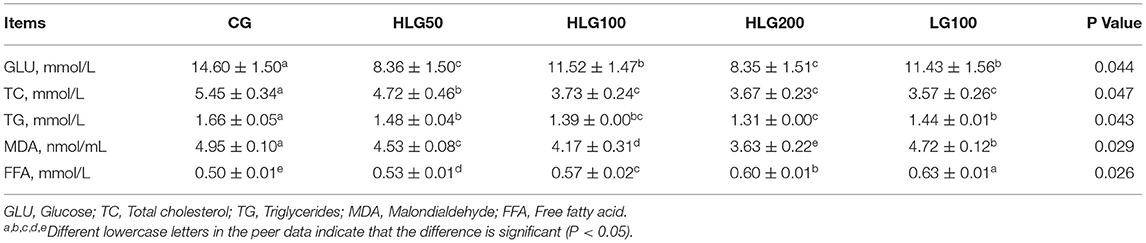
Table 8. Effects of hydroxylated lecithin on other biochemical indicator levels related to lipid metabolism in serum of Jiangnan White goslings.
Effects on Meat Quality
Effects on Meat Physiological Indexes
As shown in Table 9, the breast muscle pH value was higher (P < 0.05) in LG100 than that in HLG100 and HLG200. Leg muscle pH value was lower (P < 0.05) in treated groups than that in CG, and it was lower (P < 0.05) in LG100 than that in HLG100. The breast muscle water holding capacity was higher (P < 0.05) in groups with hydroxylated lecithin than that in CG and LG100. The leg muscle water holding capacity was higher (P < 0.05) in HLG100 than that in CG and LG100. The breast muscle shear force was lower (P < 0.05) in HLG100 than that in CG and LG100, and leg muscle shear force was lower (P < 0.05) in the treated groups than that in CG (P < 0.05). The breast muscle fiber diameter was lower (P < 0.05) in HLG100 than that in CG and LG100 (P < 0.05), while the leg muscle fiber diameter was lower (P < 0.05) in HLG100 than that in CG. The breast muscle fiber density was higher (P < 0.05) in HLG200 and LG100 than that in CG, and the leg muscle fiber density was higher (P < 0.05) in the treated groups than that in CG.
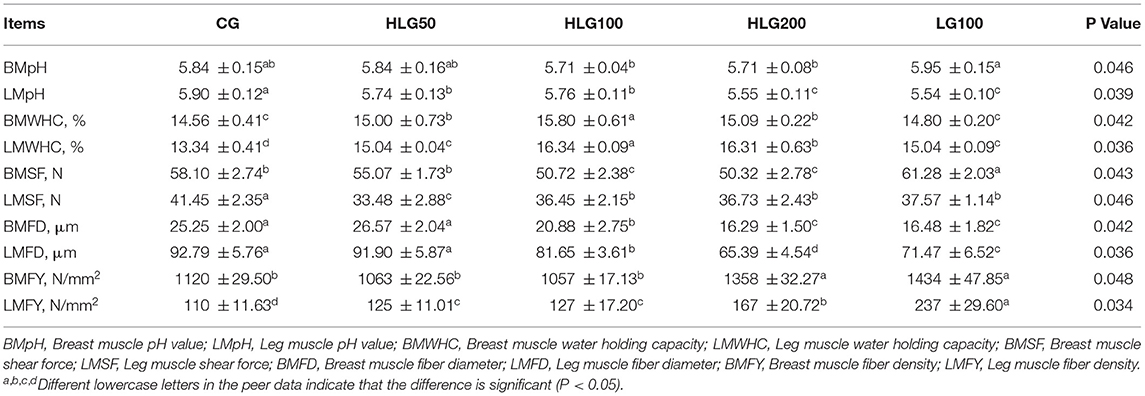
Table 9. Effects of hydroxylated lecithin on meat muscle water holding capacity and shear force of Jiangnan White goslings.
Effects on Meat Biochemistry Indexes
The meat biochemistry indexes were summarized in Table 10. In the breast muscle, the inositic acid and intramuscular fat contents were higher (P < 0.05) in HLG100 than those in CG and LG100. The triglyceride content was lower (P < 0.05) in HLG100 than that in CG and LG100. Phospholipid content was significantly higher (P < 0.05) in the treated groups than that in CG. In the leg muscle, the inositic acid, intramuscular fat and phospholipid contents were higher in HLG100 than those in CG. The triglyceride content was lower (P < 0.05) in HLG100 and LG100 than that in CG.
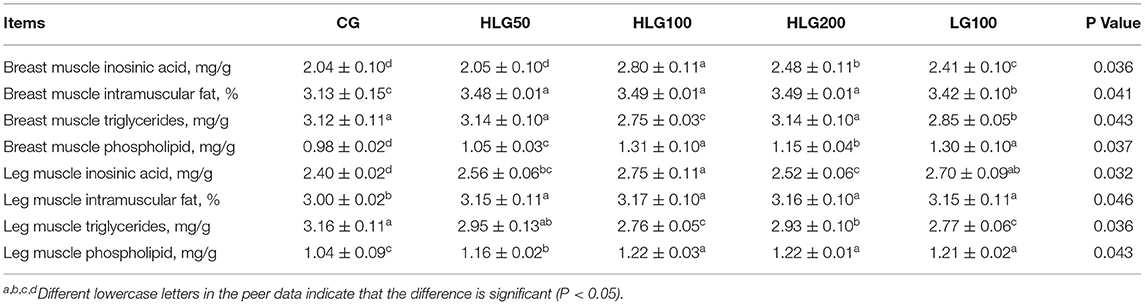
Table 10. Effects of hydroxylated lecithin on muscle inosinic acid and other indicators of Jiangnan White goslings.
Effects on Genes Relative Expression in Breast and Leg Muscles
As shown in Figure 1, the relative expression levels of the SREBP-1 gene in the treated groups were higher (P < 0.05) than those in the CG of breast and leg muscles. The PHKG 1 gene relative expression in HLG100, HLG200, and LG100 was lower than that in the CG of breast and leg muscles.
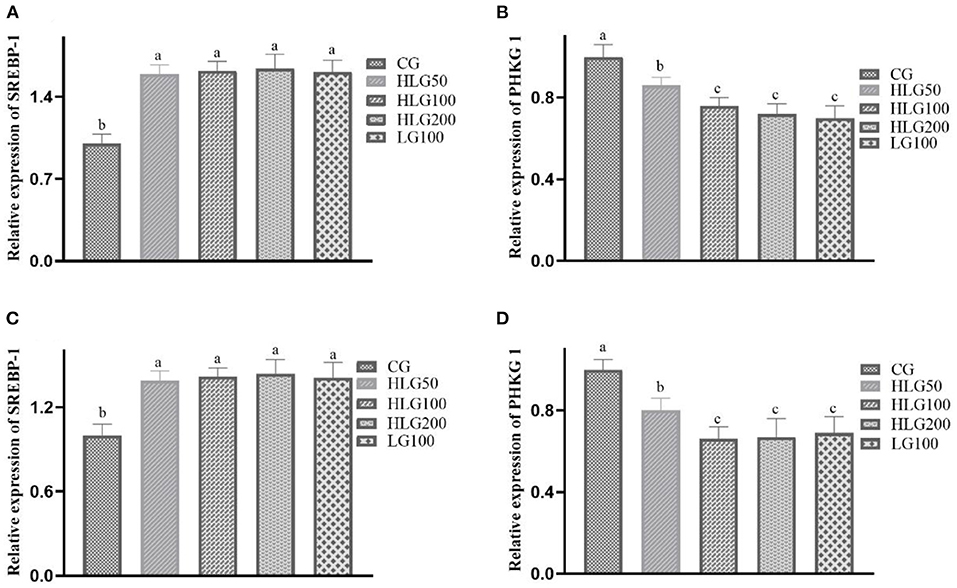
Figure 1. The relative expression levels of genes relative to meat quality in breast and leg muscle of Jiangnan White goslings. The data in CG, HLG50, HLG100, HLG200, and LG100 in breast muscle were (A) SREBP-1 gene: 1.00 ± 0.10b, 1.59 ± 0.08a, 1.62 ± 0.08a, 1.64 ± 0.12a, 1.61 ± 0.10a, respectively; (B) PHKG 1 gene: 1.00 ± 0.10a, 0.86 ± 0.04b, 0.76 ± 0.04c, 0.72 ± 0.05c, 0.70 ± 0.06c, respectively; The data in CG, HLG50, HLG100, HLG200, and LG100 in leg muscle were (C) SREBP-1 gene: 1.00 ± 0.10b, 1.39 ± 0.07a, 1.42 ± 0.06a, 1.44 ± 0.10a, 1.41 ± 0.11a, respectively; (D) PHKG 1 gene: 1.00 ± 0.10a, 0.80 ± 0.06b, 0.66 ± 0.06c, 0.67 ± 0.09c, 0.69 ± 0.08c, respectively.
Discussion
Effects on Growth Performance
Growth performance is an indispensable indicator of poultry growth status under different conditions (22, 23). The utilization of unsaturated fatty acids in poultry is much higher than that of saturated fatty acids (24, 25). Hydroxylated lecithin and soy lecithin provide nutrients and many unsaturated fatty acids to poultry (15).
In this study, the results of adding 50, 100, and 200 mg/kg of hydroxylated lecithin and 100 mg/kg of soy lecithin to the basal diet of goslings showed that the FBW was significantly higher in HLG200 than that in the CG and HLG50. The ADFI in the treated groups with hydroxylated lecithin and soy lecithin was considerably higher, the ADG in HLG200 was substantially higher than that in the CG, but the FCR was shown an opposite trend. Ren et al. (26) found that hydroxylated lecithin could significantly improve the average daily weight gain and average daily feed intake of three-yellow chickens. Gu and Li (27) found that soy lecithin had a significant effect on the average daily weight gain and the feed-to-weight ratio of piglets and could significantly reduce the feeding costs. Wang et al. (28) found that phosphatidylcholine, one of the main components of soy lecithin and hydroxylated lecithin, could dramatically improve the production performance of broiler chicks. Mandalawi et al. (29) found that soy lecithin could significantly improve the feed-to-egg ratio of laying hens. Furthermore, the results in this study were consistent with the above results, indicating that both hydroxylated lecithin and soy lecithin could improve the growth performance of goslings and the effect of hydroxylated lecithin is better than that of soy lecithin. Huang et al. (30) found no significant effect of soy lecithin on the performance of broiler chicks, which is not consistent with the results of this experiment, which may be related to the experimental animal species, conditions, soy lecithin ingredients and supplementation.
The hydroxylated lecithin and soy lecithin are effective emulsifiers, and both can promote the absorption of fat in feed for poultry (2, 15). Sibbald and Kramer (31) found that soy lecithin could improve the animal fats utilization in poultry, and Dei et al. (32) found that soy oil could improve the fats digestibility coefficient of broiler chickens. In this study, the hydroxylated lecithin significantly reduced goslings' FCR, which may be associated with the increasing cholecystokinin secretion stimulated by the hydroxylated lecithin in the small intestine that can delay the gastrointestinal emptying. This physiological process can extend the feed residence time in the gastrointestinal tract so that nutrients can be fully digested and absorbed in goslings.
Effects on Serum Biochemistry Indexes Related to Lipid Metabolism
Effects on Serum Enzymes Activity Related to Lipid Metabolism
The ALT activity can indirectly reflect the fatty acid synthesis state, and it is the most sensitive indicator of damage to hepatocytes. Liver damage can directly cause lipid metabolism disorders in animals (33). Liang et al. (34) found that the ALT activity increase in crucian carp indicated that the hepatocytes oxidative damage, which would cause the lipid metabolism disorder in the body of crucian carp. Song et al. (35) found that the ALT activity decrease in cells was accompanied by a reduction of intracellular fatty acid deposition, which indicates that ALT can effectively respond to lipid metabolism in the body and signal the formation or treatment for fatty liver and other pathologies. In this study, the serum ALT activity was significantly decreased, indicating that the hydroxylated lecithin and soy lecithin can effectively maintain the healthy state of liver cells and reduce the ALT activity, thus enabling the lipid metabolism process to proceed smoothly. In addition, the decrease of triglycerides and increase of free fatty acids in the serum of goslings in this study also verified the effect of alanine aminotransferase on the body. Further, it reflected that a healthy liver is an indispensable guarantee for lipid metabolism in the body.
LPL is one of the lipid metabolism limiting enzymes in poultry and has a vital influence on body fat deposition and intramuscular fat content (36). LPL catalyses the breakdown of TG into fatty acids and monoglycerides in the poultry, providing the organism with the energy needed for various metabolism (37). He et al. (38) found that increasing LPL mRNA and protein levels in hepatocytes could accelerate intracellular lipid metabolism. Kaneko et al. (39) found that LPL in the body of lean juvenile red seabream showed an increasing trend followed by a decreasing trend with the fat level increase in the diet. However, if the fat level in the diet were too high, the LPL activity would be inhibited, and the accumulation of fat deposition in the liver would harm the health of lean juvenile red seabream. In this study, the serum LPL activity in treated groups except for HLG50 was significantly increased, indicating that hydroxylated lecithin and soy lecithin could improve the LPL activity in goslings, which played a regulatory role in the fatty acids synthesis and metabolism. Furthermore, the serum GLU, TG and TC of goslings in this study were lower than those in the CG, which also verified to a certain extent the regulatory role of LPL on lipid metabolism in the organism.
MDH is a vital enzyme in the malate shuttle system of poultry to ensure that NADH generated by glycolysis in the cytosol enters the mitochondria for complete oxidation to produce energy, which plays an indispensable role in the complete oxidation or mutual transformation of nutrients in poultry (40, 41). In this study, the MDH activity in goslings was significantly decreased, indicating that the hydroxylated lecithin and soy lecithin reduced the oxaloacetate content, a raw material in the tricarboxylic acid cycle, thus inhibiting the fatty acid oxidation process and enhancing the deposition of lipids in poultry.
Effects on Serum Hormone Levels Related to Lipid Metabolism
ADPN is an endogenous bioactive cytokine and hormone secreted by adipocytes in poultry (42). After binding to the receptor, ADPN has a vital role in regulating the sugars and lipids metabolism in the organism, and it can improve insulin sensitivity, accelerate glucose metabolism and promote lipids metabolism in poultry (43). De Koster et al. (44) found that the ADPN could reduce the levels of β-hydroxybutyric acid, non-lipidated fatty acids and triacylglycerol in periparturient cows, i.e., ADPN could promote the lipids metabolism. Tahmoorespur et al. (45) found that the expression levels of adiponectin and its receptors were significantly increased when broilers needed much energy for body growth and development underfeeding restriction and other conditions, i.e., ADPN has an essential regulatory role in lipid metabolism and energy metabolism in poultry. In this study, the serum lipocalin content in treated groups was significantly increased due to the vigorous growth of goslings requiring more energy to prompt the lipids metabolism. The results in this study are consistent with the above studies.
LEP in poultry can regulate lipid metabolism in the organism by exciting the sympathetic nervous system (46, 47). Li et al. (48) found that LEP could inhibit lipid synthesis in hepatocytes, promote hepatocyte lipolysis and lipid deposition in Siniperca chuatsi under a high-fat feeding environment; Lin et al. (49) found that LEP could improve insulin resistance and increase insulin sensitivity in rats, and Steinberg Greg and Dyck (50) found that the LEP content decrease accelerated the lipid metabolism in obese rats caused by high-fat diets. In this study, except for HLG50, the LEP contents in the treated groups were significantly decreased, indicating that the hydroxylated lecithin and soy lecithin can reduce LEP content to promote lipid metabolism, making the goslings produce foraging behavior, which was consistent with the above study results.
GLC has a decisive role in promoting glycogenolysis and gluconeogenesis, which can cause a significant increase in blood GLC. In contrast, INS promotes the GLC uptake and utilization by tissue cells and stimulates glycogen synthesis inhibiting gluconeogenesis, causing the blood GLU decrease in poultry (51–53). Gallagher et al. (54) found that improving INS sensitivity and reducing INS resistance can regulate the metabolic level of adipocytokines in patients with metabolic syndrome. Adeva-Andany et al. (55) found that fat oxidation metabolism was inhibited when INS level increased, and fat anabolism was inhibited if GLC level increased in animals. In this study, the GLC contents in the groups treated with hydroxylated lecithin and soy lecithin were significantly lower than those in CG. In contrast, the INS contents were showed an opposite trend, indicating that the hydroxylated lecithin and soy lecithin regulated the lipid anabolism by increasing the INS content and decreasing the GLC content in goslings.
The TRH, a 3-peptide hormone secreted by the hypothalamus, can stimulate the pituitary gland thyrotropin-secreting cells to secrete the thyrotropic hormone (56). Thyroid hormones mainly include T4 and T3, which promote gluconeogenesis and hepatic glycogen synthesis in poultry (57). Sinha et al. (58) found that excessive T4 caused more fat breakdown than fat synthesis, leading to decreased cholesterol levels and weight loss in mice. Schering et al. (59) found that T4 in beef cattle could promote fatty acid oxidation and accelerate TC degradation if the level of T4 in beef cattle was within a reasonable range, the lipid synthesis and decomposition were in a dynamic balance, and the blood lipids were maintained at an average level. Ge et al. (60) found that the high energy level diets would reduce T3 in broiler breeders and thus promote lipid metabolism. In this study, the treated groups' TRH, T3, and T4 contents were significantly decreased, resulting from hydroxylated lecithin and soy lecithin regulating the TRH content, handling the T4 and T3 contents to complete the lipid synthesis and metabolism regulation in goslings.
Effects on Meat Quality
The poultry meat quality can generally be evaluated by pH value, water holding capacity and shear force (61). Various aspects influence muscle pH value changes, and the direct influence factor is muscle glycolysis. The pH value visualizes muscle acidity and alkalinity and can directly impact muscle tenderness and drip loss (61). In this study, the BMpH in HLG100 and HLG200 was lower, the LMpH in the treated groups with hydroxylated lecithin and soy lecithin was lower, indicating that hydroxylated lecithin could reduce the pre-growth muscles pH value of geese. Poultry muscle water holding capacity has a critical impact on meat processing. If the muscle water holding capacity is strong, the muscle itself can be well-preserved nutrients and flavor components in the production and processing to enhance the muscle quality. Lee et al. (62) found that the higher muscle water holding capacity can increase the muscle flavor of broiler breasts. In this study, the BMWHC was higher in HLG100 and HLG200, and the LMWHC was significantly higher in the treated groups, indicating that hydroxylated lecithin and soy lecithin can improve the breast and leg muscle water holding capacity of goslings, thus improving its meat quality. Stefania et al. (63) found that muscle tenderness improved with reduced muscle shear force in pigs. In this study, the BMSF in HLG200 was lower, and LMSF in the treated groups was more downward, indicating that the hydroxylated lecithin and soy lecithin could improve the breast and leg muscle tenderness of goslings.
The muscle fiber small-diameter and large-density can improve the meat tenderness in poultry (64). In this study, the BMFD and LMFD in HLG100, HLG200, and LG100 were lower, the BMFY and LMFY were higher in HLG200 and LG100, indicating that the hydroxylated lecithin and soy lecithin could improve the meat tenderness goslings, which echoes the decrease in breast and leg muscle shear force above to increase the meat tenderness.
The inosinic acid has a vital influence on the meat flavor in poultry (65). Zhang et al. (66) found that the inosinic acid was correlated with the meat flavor of broilers, and Wang et al. (65) found that different feed additives could determine the meat freshness grade by affecting the muscle inosinic acid content. In this study, the higher breast muscle inosinic acid content in HLG100, HLG200, and LG100, and the higher leg muscle inosinic acid content in treated groups, indicating that the hydroxylated lecithin and soy lecithin could improve the meat flavor of goslings. Intramuscular fat includes lipid droplets within muscle fibers and fat deposited between muscle fibers and fiber bundles (67). The intramuscular fat content in muscle critically influences the meat flavor and tenderness. Zhang et al. (68) found that the expression of the SREBP-1 gene, PHKG 1 gene, fatty acid synthase gene, hormone-sensitive lipase gene, and lipoprotein lipase gene could regulate intramuscular fat deposition, thus improving the pork flavor and tenderness. Yang et al. (69) found that the meat tenderness of Peking duck could be enhanced with the intramuscular fat content increase. In this study, the intramuscular fat content in goslings' breast and leg muscles was increased, indicating that the hydroxylated lecithin affects the intramuscular fat deposition in the muscles, improving the meat tenderness flavor of goslings. And the results of SREBP-1 gene and PHKG 1 gene relative expression in HLG100 also validated the above results at the RNA levels. TG, the most abundant lipids, can be broken down to provide energy for poultry (70). The phospholipid is an indispensable part of the biological membrane structure (70). Therefore, TG and phospholipids can affect the meat quality of poultry in different ways. In this study, the breast and leg muscle TG contents in HLG100 and LG100 were lower; and the phospholipids content in treated groups was higher, indicating that the hydroxylated lecithin could improve the meat quality by decreasing the triglycerides content and increasing the phospholipids content.
Conclusion
The effects of hydroxylated lecithin on growth performance, serum enzyme activity, hormone levels related to lipid metabolism and meat quality of Jiangnan White goslings in the different treatment groups were assessed. The final body weight, average daily gain, feed conversion ratio, alanine aminotransferase, malate dehydrogenase, leptin, glucose, triiodothyronine, thyroid hormone, malondialdehyde, free fatty acid, breast muscle water holding capacity, leg muscle water holding capacity, intramuscular fat contents, relative expression levels of the sterol regulatory element-binding protein-1 and phosphorylase kinase gamma subunit 1 genes in HLG200 were improved compared with the CG and/or LG100. Overall, the hydroxylated lecithin concentration of 200 mg/kg improved the growth performance, serum enzyme activity, hormone levels related to lipid metabolism, and the meat quality of Jiangnan White goslings. Therefore, hydroxylated lecithin can be widely used as a safe and reliable additive in livestock production.
Data Availability Statement
The original contributions presented in the study are included in the article/supplementary material, further inquiries can be directed to the corresponding authors.
Ethics Statement
The animal study was reviewed and approved by the Chinese guideline for animal welfare and with the animal welfare standards of the College of Animal Science and Technology, Northeast Agricultural University (NEAU-2018-0232).
Author Contributions
HW and SW prepared the manuscript and collected some data. YT, NZ, CW, RL, WX, TX, LG, and FJ collected the samples. LL and LX were responsible for the design and direction of the experiment. All authors have read and agreed to the published version of the manuscript. All authors contributed to the article and approved the submitted version.
Funding
This work was supported by the China Agriculture Research System of MOF and MARA, China Scholarship Council (CSC) National Public Graduate Program for Building Highly Qualified Universities, and Wenzhou New poultry variety breeding cooperation group project (2019ZX005).
Conflict of Interest
The authors declare that the research was conducted in the absence of any commercial or financial relationships that could be construed as a potential conflict of interest.
Publisher's Note
All claims expressed in this article are solely those of the authors and do not necessarily represent those of their affiliated organizations, or those of the publisher, the editors and the reviewers. Any product that may be evaluated in this article, or claim that may be made by its manufacturer, is not guaranteed or endorsed by the publisher.
References
1. Hugo E-a, Gladys P-h. Optimization of ultrasonication curcumin-hydroxylated lecithin nanoemulsions using response surface methodology. J Food Sci Technol. (2020) 57:549–56. doi: 10.1007/s13197-019-04086-w
2. Marina B. Hydroxylated lecithin. In: Springer Lexikon Kosmetik und Körperpflege. Berlin; Heidelberg: Springer (2007). p. 272. doi: 10.1007/978-3-540-71095-0_5066
3. Sally T, Chung RWS, Kamili A, Wat E, Weir JM, Meikle PJ, et al. Hydrogenated phosphatidylcholine supplementation reduces hepatic lipid levels in mice fed a high-fat diet. Atherosclerosis. (2010) 213:142–7. doi: 10.1016/j.atherosclerosis.2010.07.050
4. Kamili A, Wat E, Chung RWS, Tandy S, Weir JM, Meikle PJ, et al. Hepatic accumulation of intestinal cholesterol is decreased and fecal cholesterol excretion is increased in mice fed a high-fat diet supplemented with milk phospholipids. Nutr Metab. (2010) 31:90. doi: 10.1186/1743-7075-7-90
5. Huang J, Yang D, Gao S, Wang T. Effects of soy-lecithin on lipid metabolism and hepatic expression of lipo-genic genes in broiler chickens. Livest Sci. (2008) 118:53–60. doi: 10.1016/j.livsci.2008.01.014
6. Polichetti E, Janisson A, Porte C. Dietary polyenyl phosphatidyl choline decreases cholesterolemia in hypercholesterolemic rabbits: role of the hepato-biliary axis. Life Sci. (2000) 16:2563–76. doi: 10.1016/S0024-3205(00)00840-7
7. Polichetti E, Diaconescu N. Cholesterol-lowering effect of soy-bean lecithin in normal lipid aemic rats by stimulation of biliary lipid secretion. Br J Nutr. (1996) 75:471–8. doi: 10.1079/BJN19960148
8. Beccaglia M, Anastasi P, Chigioni S, Luvoni GC. TRIS-lecithin extender supplemented with antioxidant catalase for chilling of canine semen. Reprod Domest Anim. (2010) 44:345–9. doi: 10.1111/j.1439-0531.2009.01410.x
9. Jha R, Singh AK, Yadav S, Berrocoso JFD, Mishra B. Early nutrition programming (in ovo and post-hatch feeding) as a strategy to modulate gut health of poultry. Front Vet Sci. (2019) 6:82. doi: 10.3389/fvets.2019.00082
10. Kumar S, Chen C, Indugu N, Werlang GO, Singh M, Kim WK, et al. Effect of antibiotic withdrawal in feed on chicken gut microbial dynamics, immunity, growth performance and prevalence of foodborne pathogens. PLoS ONE. (2018) 13:e0192450. doi: 10.1371/journal.pone.0192450
11. Zhang B, Zhang X, Schilling MW, Tabler GT, Peebles ED, Zhai W. Effects of broiler genetic strain and dietary amino acid reduction on (part I) growth performance and internal organ development. Poult Sci. (2020) 99:3266–79. doi: 10.1016/j.psj.2020.03.024
12. Roto SM, Kwon YM, Ricke SC. Applications of in-ovo technique for the optimal development of the gastrointestinal tract and the potential influence on the establishment of its microbiome in poultry. Front Vet Sci. (2016) 3:63. doi: 10.3389/fvets.2016.00063
13. Shen Y, Zhang S, Zhao X, Shi S. Evaluation of a lecithin supplementation on growth performance, meat quality, lipid metabolism, and cecum microbiota of broilers. Animals. (2021) 11:2537. doi: 10.3390/ani11092537
14. Liu X, Yoon SB, Kim IH. Growth performance, nutrient digestibility, blood profiles, excreta microbial counts, meat quality and organ weight on broilers fed with de-oiled lecithin emulsifier. Animals. (2020) 10:478. doi: 10.3390/ani10030478
15. Mortensen A, Aguilar F, Crebelli R, Di Domenico A, Frutos MJ, Galtier P, et al. Re-evaluation of lecithins (E 322) as a food additive. EFSA J. (2017) 15:e04742. doi: 10.2903/j.efsa.2017.4742
16. Viñado A, Castillejos L, Barroeta AC. Soybean lecithin as an alternative energy source for grower and finisher broiler chickens: impact on performance, fatty acid digestibility, gut health, and abdominal fat saturation degree. Poult Sci. (2020) 99:5653–62. doi: 10.1016/j.psj.2020.06.050
17. El-Katcha MI, Soltan MA, Shewita R, Abdo SE, Sanad AS, Tufarelli V, et al. Dietary fiber and lysolecithin supplementation in growing ducks: effect on performance, immune response, intestinal morphology and lipid metabolism-regulating genes. Animals. (2021) 11:2873. doi: 10.3390/ani11102873
18. Nutautaite M, Racevičiute-Stupeliene A, Andalibizadeh L, Šašyte V, Bliznikas S, Pockevičius A, et al. Improving broiler chickens' health by using lecithin and lysophosphatidylcholine emulsifiers: a comparative analysis of physiological indicators. Iran J Vet Res. (2021) 22:33–9. doi: 10.22099/ijvr.2021.37028.5411
19. Domínguez R, Pateiro M, Gagaoua M, Barba FJ, Zhang W, Lorenzo JMA. Comprehensive review on lipid oxidation in meat and meat products. Antioxidants. (2019) 8:429. doi: 10.3390/antiox8100429
20. Alfaia CM, Pestana JM, Rodrigues M, Coelho D, Aires MJ, Ribeiro DM, et al. Influence of dietary Chlorella vulgaris and carbohydrate-active enzymes on growth performance, meat quality and lipid composition of broiler chickens. Poult Sci. (2021) 100:926–37. doi: 10.1016/j.psj.2020.11.034
21. National Research Council (NRC). Nutrient Requirements of Poultry (Geese). 9th rev ed. Washington, DC: National Research Council, National Academy Press (1994).
22. Wu H, Yang J, Wang S, Zhang X, Hou J, Xu F, et al. Effects of soybean isoflavone and astragalus polysaccharide mixture on immune and antioxidant activity of lactating sows. Animals. (2021) 11:132. doi: 10.3390/ani11010132
23. Baker JT, Duarte ME, Holanda DM, Kim SW. Friend or foe? Impacts of dietary xylans, xylooligosaccharides, and xylanases on intestinal health and growth performance of monogastric animals. Animals. (2021) 11:609. doi: 10.3390/ani11030609
24. Hunter JE, Zhang J, Kris-Etherton PM. Cardiovascular disease risk of dietary stearic acid compared with trans, other saturated, and unsaturated fatty acids: a systematic review. Am J Clin Nutr. (2010) 91:46–63. doi: 10.3945/ajcn.2009.27661
25. Kochikuzhyil BM, Devi K, Fattepur SR. Effect of saturated fatty acid-rich dietary vegetable oils on lipid profile, antioxidant enzymes and glucose tolerance in diabetic rats. Indian J Pharmacol. (2010) 42:142–5. doi: 10.4103/0253-7613.66835
26. Ren JD, Zhao XL, Zhan HQ. Effect of hydroxylated lecithin on the production performance and meat quality of three-yellow chickens. China Poult. (2019) 41:70–2. doi: 10.16372/j.issn.1004-6364.2019.01.017
27. Gu X, Li D. Fat nutrition and metabolism in piglets: a review. Anim Feed Sci Technol. (2003) 109:151–70. doi: 10.1016/S0377-8401(03)00171-8
28. Wang H, Zhang HJ, Wang XC, Wu SG, Wang J, Xu L, et al. Dietary choline and phospholipid supplementation enhanced docosahexaenoic acid enrichment in egg yolk of laying hens fed a 2% Schizochytrium powder-added diet. Poult Sci. (2017) 96:2786–94. doi: 10.3382/ps/pex095
29. Mandalawi HA, Lázaro R, Redón M, Herrera J, Menoyo D, Mateos GG. Glycerin and lecithin inclusion in diets for brown egg-laying hens: effects on egg production and nutrient digestibility. Anim Feed Sci Technol. (2015) 209:145–56. doi: 10.1016/j.anifeedsci.2015.07.019
30. Huang J, Yang D, Wang T. Effects of replacing soy-oil with soy-lecithin on growth performance, nutrient utilization and serum parameters of broilers fed corn-based diets. Asian Austral J Anim. (2007) 20:1880–6. doi: 10.5713/ajas.2007.1880
31. Sibbald IR, Kramer JKG. The effect of basal diet on the utilization of fat as a source of true metabolizable energy, lipid and fatty acids. Poult Sci. (1980) 59:316–24. doi: 10.3382/ps.0590316
32. Dei HK, Rose SP, Mackenzie AM. Apparent metabolizable energy and digestibility of shea (Vitellaria paradoxa) fat, cocoa (Theobroma cacao) fat and soybean oil in broiler chicks. Br Poult Sci. (2006) 47:607–12. doi: 10.1080/00071660600939701
33. Liu X, Hamnvik OPR, Chamberland JP, Petrou M, Gong H, Christophi CA, et al. Circulating alanine transaminase (ALT) and γ-glutamyl transferase (GGT), but not fetuin-A, are associated with metabolic risk factors, at baseline and at two-year follow-up: the prospective cyprus metabolism study. Metabolism. (2014) 63:773–82. doi: 10.1016/j.metabol.2014.03.008
34. Liang H, Zhou W, Zhang Y, Qiao Q, Zhang X. Are fish fed with cyanobacteria safe, nutritious and delicious? A laboratory study. Sci Rep. (2015) 5:15166. doi: 10.1038/srep15166
35. Song H, Han W, Yan F, Xu D, Chu Q, Zheng X. Dietary Phaseolus vulgaris extract alleviated diet-induced obesity, insulin resistance and hepatic steatosis and alters gut microbiota composition in mice. J Funct Foods. (2016) 20:236–44. doi: 10.1016/j.jff.2015.10.022
36. Loving BA, Tang M, Neal MC, Gorkhali S, Murphy R, Eckel RH, et al. Lipoprotein lipase regulates microglial lipid droplet accumulation. Cells. (2021) 10:198. doi: 10.3390/cells10020198
37. Mellado JM, Calmet J, Olona M, Esteve C, Camins A, Perez Del Palomar L, et al. Surgically repaired massive rotator cuff tears: MRI of tendon integrity, muscle fatty degeneration, and muscle atrophy correlated with intraoperative and clinical findings. Am J Roentgenol. (2005) 184:1456–63. doi: 10.2214/ajr.184.5.01841456
38. He AY, Ning LJ, Chen LQ, Chen YL, Xing Q, Li JM, et al. Systemic adaptation of lipid metabolism in response to low-and high-fat diet in Nile tilapia (Oreochromis niloticus). Physiol Rep. (2015) 3:e12485. doi: 10.14814/phy2.12485
39. Kaneko G, Shirakami H, Yamada T, Ide SI, Haga Y, Satoh S, et al. Short-term fasting increases skeletal muscle lipid content in association with enhanced mRNA levels of lipoprotein lipase 1 in lean juvenile red seabream (Pagrus major). Aquaculture. (2016) 452:160–8. doi: 10.1016/j.aquaculture.2015.10.030
40. Molenaar D, van der Rest ME, Drysch A, Yücel R. Functions of the membrane-associated and cytoplasmic malate dehydrogenases in the citric acid cycle of corynebacterium glutamicum. J Bacteriol. (2000) 182:6884–91. doi: 10.1128/JB.182.24.6884-6891.2000
41. Dong YW, Liao ML, Meng XL, Somero GN. Structural flexibility protein adaptation to temperature: molecular dynamics analysis of malate dehydrogenases of marine molluscs. Proc Natl Acad Sci USA. (2018)115:1274–9. doi: 10.1073/pnas.1718910115
42. Hidekatsu Y, Yoshida H. Beneficial effects of adiponectin on glucose and lipid metabolism and atherosclerotic progression: mechanisms and perspectives. Int J Mol Sci. (2019) 20:1190. doi: 10.3390/ijms20051190
43. Katsiki N, Mantzoros C, Mikhailidis DP. Adiponectin, lipids and atherosclerosis. Curr Opin Lipidol. (2017) 28:347–54. doi: 10.1097/MOL.0000000000000431
44. De Koster J, Urh C, Hostens M, Van Den Broeck W, Sauerwein H, Opsomer G. Relationship between serum adiponectin concentration, body condition score, and peripheral tissue insulin response of dairy cows during the dry period. Domest Anim Endocrinol. (2017) 59:100–4. doi: 10.1016/j.domaniend.2016.12.004
45. Tahmoorespur M, Ghazanfari S, Nobari K. Evaluation of adiponectin gene expression in the abdominal adipose tissue of broiler chickens: feed restriction, dietary energy, and protein influences adiponectin messenger ribonucleic acid expression. Poult Sci. (2010) 89:2092–100. doi: 10.3382/ps.2010-00772
46. Izquierdo AG, Crujeiras AB, Casanueva FF, Carreira MC. Leptin, obesity, and leptin resistance: where are we 25 years later? Nutrients. (2019) 11:2704. doi: 10.3390/nu11112704
47. Gruzdeva O, Borodkina D, Uchasova E, Dyleva Y, Barbarash O. Leptin resistance: underlying mechanisms and diagnosis. Diabetes Metab Syndr Obes. (2019) 12:191–8. doi: 10.2147/DMSO.S182406
48. Li J, Liang XF, Alam MS, Luo H, Zhang Y, Peng B, et al. Adaptation of AMPK-mTOR-signal pathways lipid metabolism in response to low-and high-level rapeseed meal diet in Chinese perch (Siniperca chuatsi). J Comp Physiol B. (2021)191:881–94. doi: 10.1007/s00360-021-01393-7
49. Lin CY, Higginbotham DA, Judd RL, White BD. Central leptin increases insulin sensitivity in streptozotocin-induced diabetic rats. Am J Physiol Endocrinol Metab. (2002) 282:E1084–91. doi: 10.1152/ajpendo.00489.2001
50. Steinberg Greg R, Dyck DJ. Development of leptin resistance in rat soleus muscle in response to high-fat diets. Am J Physiol Endocrinol Metab. (2000) 279:E1374–82. doi: 10.1152/ajpendo.2000.279.6.E1374
51. Glass CK, Olefsky JM. Inflammation and lipid signaling in the etiology of insulin resistance. Cell Metab. (2012) 15:635–45. doi: 10.1016/j.cmet.2012.04.001
52. Holland WL, Knotts TA, Chavez JA, Wang LP, Hoehn KL, Summers SA. Lipid mediators of insulin resistance. Nutr Rev. (2007) 65:S39–46. doi: 10.1111/j.1753-4887.2007.tb00327.x
53. Galsgaard KD, Pedersen J, Knop FK. Glucagon receptor signaling and lipid metabolism. Front Physiol. (2019) 10:413. doi: 10.3389/fphys.2019.00413
54. Gallagher EJ, LeRoith D, Karnieli E. Insulin resistance in obesity as the underlying cause for the metabolic syndrome. Mount Sinai J Med. (2010) 77:511–23. doi: 10.1002/msj.20212
55. Adeva-Andany MM, Funcasta-Calderón R, Fernández-Fernández C, Castro-Quintela E, Carneiro-Freire N. Metabolic effects of glucagon in humans. J Clin Trans Endocinol. (2019) 15:45–53. doi: 10.1016/j.jcte.2018.12.005
56. Sinha R, Singh B, Yen P. Direct effects of thyroid hormones on hepatic lipid metabolism. Nat Rev Endocrinol. (2018) 14:259–69. doi: 10.1038/nrendo.2018.10
57. Duntas LH, Brenta G. A renewed focus on the association between thyroid hormones and lipid metabolism. Front Endocrinol. (2018) 9:511. doi: 10.3389/fendo.2018.00511
58. Sinha RA, Singh BK, Yen PM. Thyroid hormone regulation of hepatic lipid and carbohydrate metabolism. Trends Endocrinol Metab. (2014) 25:538–45. doi: 10.1016/j.tem.2014.07.001
59. Schering L, Albrecht E, Komolka K, Kühn C, Maak S. Increased expression of thyroid hormone responsive protein (THRSP) is the result but not the cause of higher intramuscular fat content in cattle. Int J Biol Sci. (2017) 13:532. doi: 10.7150/ijbs.18775
60. Ge XK, Wang AA, Ying ZX, Zhang LG, Su WP, Cheng K, et al. Effects of diets with different energy and bile acids levels on growth performance and lipid metabolism in broilers. Poult Sci. (2019) 98:887–95. doi: 10.3382/ps/pey434
61. Mohammed AA, Zaki RS, Negm EA, Mahmoud MA, Cheng HW. Effects of dietary supplementation of a probiotic (Bacillus subtilis) on bone mass and meat quality of broiler chickens. Poult Sci. (2021) 100:100906. doi: 10.1016/j.psj.2020.11.073
62. Lee YS, Zhekov ZG, Owens CM, Kim M, Meullenet JF. Effects of partial and complete replacement of sodium chloride with potassium chloride on the texture, flavor and water-holding capacity of marinated broiler breast fillets. J Texture Stu. (2012) 43:124–32. doi: 10.1111/j.1745-4603.2011.00322.x
63. Stefania D, Schiavo G, Gallo M, Bovo S, Bertolini F, Buttazzoni L, et al. Candidate gene markers associated with production, carcass and meat quality traits in Italian Large White pigs identified using a selective genotyping approach. Livest Sci. (2020) 240:104145. doi: 10.1016/j.livsci.2020.104145
64. Klont RE, Brocks L, Eikelenboom G. Muscle fibre type and meat quality. Meat Sci. (1998) 49:S219–29. doi: 10.1016/S0309-1740(98)90050-X
65. Wang XF, Liu GH, Cai HY, Chang WH, Ma JS, Zheng AJ, et al. Attempts to increase inosinic acid in broiler meat by using feed additives. Poult Sci. (2014) 93:2802–8. doi: 10.3382/ps.2013-03815
66. Zhang GQ, Ma QG, Ji C. Effects of dietary inosinic acid on carcass characteristics, meat quality, and deposition of inosinic acid in broilers. Poult Sci. (2008) 87:1364–9. doi: 10.3382/ps.2007-00193
67. Rahemi H, Nigam N, Wakeling JM. The effect of intramuscular fat on skeletal muscle mechanics: implications for the elderly and obese. J R Soc Interface. (2015) 12:20150365. doi: 10.1098/rsif.2015.0365
68. Zhang H, Zhang X, Wang Z, Dong X, Tan C, Zou H, et al. Effects of dietary energy level on lipid metabolism-related gene expression in subcutaneous adipose tissue of Yellow breed × Simmental cattle. Anim Sci J. (2015) 86:392–400. doi: 10.1111/asj.12316
69. Yang T, Wang X, Wen M, Zhao H, Liu G, Chen X, et al. Effect of manganese supplementation on the carcass traits, meat quality, intramuscular fat, and tissue manganese accumulation of Pekin duck. Poult Sci. (2021) 100:101064. doi: 10.1016/j.psj.2021.101064
70. Feingold KR, Grunfeld C. Introduction to lipids and lipoproteins. In: Endotext. MDText.com, Inc, South Dartmouth (MA) (2000). Available online at: https://europepmc.org/article/NBK/nbk305896
Keywords: hydroxylated lecithin, Jiangnan White goslings, enzymes activity, hormone levels, lipid metabolism, meat quality
Citation: Wu H, Wang S, Tian Y, Zhou N, Wu C, Li R, Xu W, Xu T, Gu L, Ji F, Xu L and Lu L (2022) Effects of Hydroxylated Lecithin on Growth Performance, Serum Enzyme Activity, Hormone Levels Related to Lipid Metabolism and Meat Quality in Jiangnan White Goslings. Front. Vet. Sci. 9:829338. doi: 10.3389/fvets.2022.829338
Received: 05 December 2021; Accepted: 21 January 2022;
Published: 22 February 2022.
Edited by:
Iveta Placha, Centre of Biosciences, Slovak Academy of Sciences (SAS), SlovakiaReviewed by:
Abdel-Moneim Eid Abdel-Moneim, Egyptian Atomic Energy Authority, EgyptShourong Shi, Poultry Institute (CAAS), China
Copyright © 2022 Wu, Wang, Tian, Zhou, Wu, Li, Xu, Xu, Gu, Ji, Xu and Lu. This is an open-access article distributed under the terms of the Creative Commons Attribution License (CC BY). The use, distribution or reproduction in other forums is permitted, provided the original author(s) and the copyright owner(s) are credited and that the original publication in this journal is cited, in accordance with accepted academic practice. No use, distribution or reproduction is permitted which does not comply with these terms.
*Correspondence: Lizhi Lu, bHVsaXpoaWJveEAxNjMuY29t; Li Xu, eHVsaV8xOTYyMTk5MUAxNjMuY29t
†These authors have contributed equally to this work