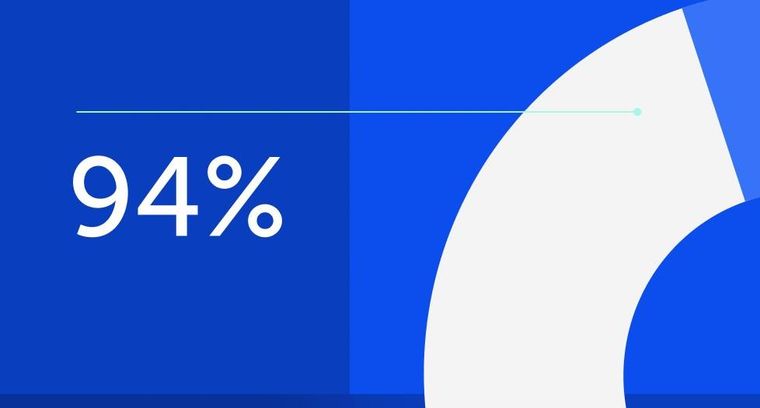
94% of researchers rate our articles as excellent or good
Learn more about the work of our research integrity team to safeguard the quality of each article we publish.
Find out more
ORIGINAL RESEARCH article
Front. Vet. Sci., 01 March 2022
Sec. Veterinary Pharmacology and Toxicology
Volume 9 - 2022 | https://doi.org/10.3389/fvets.2022.815198
We evaluated the pharmacokinetics of silymarin solid dispersion in pigs to determine whether silybin bioavailability would be increased over that of a silymarin premix. In vitro dissolution testing was conducted using dissolution apparatus 1 (baskets) at 100 rpm at 37 ± 0.5°C in pH 1.2 HCl, pH 6.8 phosphate, and pH 4.3 acetate buffers containing 0.5% Tween-80. In vivo pharmacokinetics were studied using 16 healthy pigs (Yorkshire × Landrace) that were randomly assigned to two groups. Silymarin as solid dispersion and premix dosage forms were administered directly by stomach tubes at 50 mg kg−1 silybin. In vitro dissolution of silybin for the premix was 35.02, 35.90, and 38.70% in these buffers, respectively. In contrast, silybin dissolution in solid dispersions was increased to 82.92, 87.48, and 99.70%, respectively. Silymarin solid dispersion administered at a single dose resulted in a peak concentration (Cmax) of 1,190.02 ± 246.97 ng ml−1 with the area under the curve (AUC0−∞) at 1,299.19 ± 67.61 ng ml−1 h. These parameters for the premix groups were 411.35 ± 84.92 ng ml−1 and 586.82 ± 180.99 ng ml−1 h, respectively. The Cmax and AUC0−∞ values for the solid dispersion were about twice that of the premix and were consistent with the in vitro dissolution data.
Silymarin represents a complex polyphenolic mixture extracted from the seeds of the milk thistle Silybum marianum L (1, 2) and is composed of 65–80% flavonolignans silybin, isosilybin, silychristin, and silydianin with trace amounts of flavonoids and 20–35% fatty acids and polyphenolics (3, 4). The primary component of silymarin is silybin (CAS No. 22888-70-6), and this has been identified as its bioactive component (4–6). The main component of silymarin is silybin, synonymous with silibinin and is a mixture of two diastereomers A and B in an ~1:1 proportion (6–8).
Silymarin extracts have antioxidant, radical scavenging, and metal chelating activities in vitro and in vivo that can inhibit neutrophil migration and reduce edema at sites of inflammation (9, 10). Additionally, its flavonolignan and flavonoid compounds have been shown to inhibit JFH-1 virus-induced oxidative stress (11). Interestingly, silymarin has potent effects against hepato-trophic viruses as well as those with other host ranges and is therefore a potential broad-spectrum antiviral (12, 13). Silymarin has an effect that allows its use in all of the most frequent causes of liver damage and possesses three important activities: anti-inflammatory, antioxidant, and pro-apoptotic that can antagonize the onset and the progression of mechanisms of damage that are responsible for the progression of hepatitis to cirrhosis and hepatocellular carcinoma (14).
Silymarin has been examined for veterinary use because animal production processes in modern animal husbandry places a pathological burden on the liver due to disease and side effects of drugs, especially in the piglet stage before fattening (15). Liver damage is a frequent microscopic finding in cases of postweaning multisystemic wasting syndrome (PMWS), and hepatocytes are a target cell for porcine circovirus type 2 (PCV-2) infection and replication (16). Drug-induced bile duct injury in most affects the biliary epithelium of interlobular ducts, and amoxicillin/clavulanic acid was among the most frequent causes of drug-induced liver injury (17). Some drugs can cause chronic liver damage and even lead to tumor growth (18). Silymarin has been classified as a liver therapeutic agent by the WHO (19), and this has attracted the attention of veterinarians. It has been tested in dairy cows suffering from fatty liver during peripartum, and its effects include reduced ketosis and improved lactation performance (20). S. marianum incorporated into fodder was a successful substitute for fodder antibiotics in pigs, and weight gains increased and were 6.1% greater compared with the group receiving conventional prophylactic antibiotics (21, 22). Silymarin has also been shown to promote bile secretion and has a choleretic effect, but this was species-specific and also linked to dosage (23).
Silymarin has important biological activities and is currently used in veterinary practices to protect the liver, although its pharmacokinetics in pigs and other production animals has not been fully elucidated. In fact, poor solubility and low bioavailability of silybin limits its therapeutic potential (24). Therefore, further optimization and characterization of in vitro dissolution and drug-release profiles of silymarin would be required before it could be used clinically in vivo. In the present study, we examined the in vitro dissolution profiles of silymarin solid dispersions and premix and assessed their pharmacokinetics and bioavailabilities in pigs.
Silybin reference standard (96.3%) was provided by the China Institute of Veterinary Drugs Control (Beijing, China). Silymarin solid dispersion (see Table 1) was developed by the College of Veterinary Medicine, South China Agricultural University (Guangzhou, China), and produced in a pilot project at Jinhe Bio-Tech (Hohhot, China). Silymarin active pharmaceutical ingredient (API) was calculated as silymarin at 60.25%. Silymarin API, silymarin premix, and pharmaceutical excipients of the premix were all provided by Guangzhou Leader Bio-Technology (Guangzhou, China). Assays of silybin in silymarin solid dispersion and silymarin premix were 3.92 and 3.10%, respectively. Pharmaceutical excipients of silymarin solid dispersion were provided by Jinhe Bio-tech. Acetonitrile and methanol [high-performance liquid chromatography (HPLC) grade] were purchased from Fisher Scientific (Pittsburg, PA, USA). Water was purified using a Milli-Q Water Purification system (Milford, MA, USA). Other chemicals used were of analytical grade and purchased from Damao Chemical Reagent Factory (Tianjin, China).
Ethical approval for in vivo experiments in pigs was obtained from the animal ethics committee of South China Agriculture University. Animals used in this study consisted of 16 healthy pigs (Yorkshire × Landrace) weighing 13.68 ± 0.57 kg. The animals were acclimatized in pens for 1 week and given standard commercial feed twice a day with water available ad libitum.
The dissolution study was carried out according to the Chinese Veterinary Pharmacopeia (CVP, 2015) basket method using 900 ml of the following dissolution media: pH 1.2 HCl, pH 4.3 acetate, and pH 6.8 phosphate buffers each containing 0.5% Tween-80. The temperature of the dissolution medium was controlled at 37 ± 0.5°C, and stirring speed was maintained at 100 rpm. Samples (5 ml) were withdrawn at 20, 40 min and 1, 2, 4, and 6 h and replenished immediately with 5 ml dissolution medium. Samples were passed through 0.22 μm filters prior to HPLC quantification. Data at each time point were presented as mean of triplicate samples.
Pigs were randomly divided into two groups, with eight pigs per group, and received solid dispersion and premix by oral gavage at a single dose of silybin 50 mg kg−1, respectively. Blood samples (4 ml) were taken from the superior vena cava by syringe and transferred to polyethylene tubes containing heparin at 0, 5, 10, 15, 20, 30, and 45 min and at 1, 1.5, 2, 3, 4, 6, and 8 h post-administration. Plasma samples were obtained by centrifuging the collected blood samples at 4,000 rpm for 10 min and were stored at −20°C.
Silybin levels in plasma samples were determined using HPLC using an LC-20AT quaternary pump and SPD-20A UV detector (Shimadzu, Kyoto, Japan). Chromatographic separation was achieved on a InertSustain C18 analytical column (250 × 4.6 mm, 5 μm) (Phenomenex, Torrance, CA, USA) using an isocratic mobile phase of 50% methanol in water at 0.9 ml min−1 using a detection wavelength of 288 nm.
Plasma samples were processed according to previously published protocols (25). In brief, 1 ml of plasma in 10 ml centrifuge tubes was combined with 1 ml of tert-butyl methyl ether and vortexed for 2 min and then centrifuged at 5,000 rpm for 5 min. A supernatant sample was evaporated under a nitrogen stream in a heating block at 50°C, the residue was reconstituted with 200 μl methanol and passed through a 0.22-μm filter, and 10 μl was used for HPLC injection.
The cumulative release (%) of the drug at each time point was calculated according to the following formula:
where Cn is the concentration of silybin in the sample taken at time n, V is the total volume of the release medium, Vi is the sampling volume at time i, Ci is the concentration of the sample taken at time i, W is the weight of the solid dispersions, and DL is the drug loaded in the solid dispersions.
Silybin concentration–time data were analyzed using WinNonlin 5.2.1 software (Pharsight, Mountain View, CA, USA) using a non-compartment model with best fitting. The pharmacokinetic parameters are presented as mean ± SD and were compared for statistical significance using independent Student t-tests. A value of p < 0.05 was considered statistically significant. All statistical analyses were performed using SPSS 22.0 statistical software (IBM, Chicago, IL, USA).
The method used in this work was validated by adding silymarin to blank excipients from the solid dispersions and premix to 0.5, 1, and 10 μg ml−1 silybin. The samples were subjected to the extraction process, and the recoveries for silybin from both blank excipients were 98.67–100.93% and 98.26–101.61%, respectively. Correspondingly, the relative standard deviations ranged from 0.26 to 0.74% and 0.07 to 0.79% respectively. Silybin regression data calculated from spiked samples gave recoveries of 94.16–100.00% in plasma at 30, 100, and 1,000 ng ml−1. The intraday and inter-day variations ranged from 2.76 to 9.07% and 6.62 to 8.85%, respectively. The calibration curves were linear in the range of 30–5,000 ng ml−1 (r2 > 0.99). The limit of detection (LOD) was <15 ng ml−1, and the limit of quantification (LOQ) was <30 ng ml−1.
The total accumulated amount of the solid dispersion that was released was >80% silybin at pH 1.2, 4.3, and 6.8 in 0.5% Tween 80 solutions, respectively. In contrast, the maximum released by the silymarin premix was only 38.70% in these three buffers, respectively. Overall, the cumulative release rate of silymarin solid dispersion exceeded that of the premix and the total released per unit time was 2.5-fold higher (Table 2, Figure 1).
Table 2. In vitro silymarin cumulative release for solid dispersion and premix in the indicated buffers.
Figure 1. In vitro cumulative release curves of silymarin solid dispersion and silymarin premix in different pH buffer solutions. SD, solid dispersion; PM, premix; SLY, silymarin.
Silybin levels in porcine plasma samples indicated that both formulations possessed the same Tmax while the peak concentrations for the solid dispersion reached 1,189.26 ng ml−1 and were ~3-fold greater than for the premix (Figure 2, Table 3). The solid dispersion raised the AUC0−∞ to 1,299.19 ng ml−1 h, and the relative bioavailability increased more than 2-fold (Table 4).
Figure 2. Plasma concentration–time curves of silymarin after single-dose administration at a dose of 50 mg kg−1. Data represent mean ± SD values for eight pigs.
Table 3. Silymarin concentration–time data at different time points following administration of solid dispersion and premix to pigs.
Table 4. Comparison of pharmacokinetic parameters using a non-compartmental model for solid dispersion and premix administered to pigs.
Silymarin is widely used as a liver protectant in veterinary clinics by virtue of its free radical scavenging, anti-oxidative, and anti-lipid peroxidative activities as well as its antifibrotic and anti-inflammatory activities. However, these positive effects cannot be realized unless the drug is released and generally dissolved in the fluids of the gastrointestinal tract (26). The solubility of silymarin in water is only 0.04 mg ml−1, and it is not lipophilic; this results in low permeability across intestinal epithelial cells (27–29). Therefore, it was particularly important to modify the dosage form of silymarin to enhance its bioavailability.
Solid dispersion is a preparation technology used to disperse poorly soluble drugs in solid carrier materials to a form that enhances dissolution rates and solubility. This thereby improves absorption and bioavailability (30). Silymarin solid dispersions have been prepared by fusion and solution-enhanced dispersion using the supercritical fluids (SEDS) and the “dripping pills” methods (31, 32). However, spray granulation has proven to be easier and more efficient and can be used for continuous production in manufacturing (33). We found a cumulative release from silymarin solid dispersion prepared in HCl, phosphate, and acetate buffers containing Tween-80 that was about 2.5-fold greater than for the silymarin premix. These data also indicated that the in vivo release would also be enhanced, and we verified this in pharmacokinetic experiments using pigs.
Silymarin has been shown to inhibit acute inflammation in a dose-dependent fashion, and the dose that resulted in 50% inhibition of the in vivo inflammatory response (ED50) was 62.42 mg kg−1, calculated as the amount of silybin present (9). Interestingly, silybin (20–50 mg kg−1 day−1) has also shown to be effective against amanitin poisoning (34). We therefore administered a comparable ED50 of 50 mg kg−1 silybin as the single dosage administered orally to pigs. Silybin is isomeric, so we calculated the total amounts using the total areas of peaks that eluted at 14 and 15 min, which was used for pharmacokinetic calculations (see Figure 3) (35).
The rapid and extensive phase II metabolism of silybin has been considered as another reason contributing to its low bioavailability (36, 37). The apparent volumes of distribution we calculated were >1.0 L kg−1 and revealed higher tissue levels than plasma levels (38). Peak levels of silybin were observed at 0.5 h in liver, lung, stomach, and pancreas of mice after oral administration at 50 mg kg−1 (39). Silybin is primarily excreted in conjugated form through the hepato-biliary tract, and bile levels can exceed plasma levels by 100-fold (24, 40, 41). Silybin can be rapidly and readily transported to tissues after administration, and this would also contribute to its short plasma t1/2.
For human volunteers, after oral administration of single doses of 102, 153, 203, and 254 mg, plasma t1/2 were <1 h (42). The plasma t1/2 of 12.2 min was also obtained using a non-compartmental method after oral administration of 500 mg kg−1 to rats (41). A two-compartment model was fitted to silymarin material, and pro-liposome plasma concentrations in beagles generated t1/2β levels of 2.78 and 1.61 h after administration of a dose of 7.7 mg kg−1 (35). Short plasma t1/2 levels were also independent of animal species. Silymarin is eliminated via hepato-enteric circulation, but we did not find double peaks on the drug concentration–time curves (Figure 2).
The silymarin solid dispersion achieved relatively higher peak concentrations and AUC in pigs compared with the premix. Administration of 50 mg kg−1 silybin premix generated a Cmax of 411 ± 85 ng ml−1, and the AUC0−∞ was 587 ± 181 ng ml−1 h in pigs, while the solid dispersion increased these numbers to 1,190 ± 247 ng ml−1 and 1,299 ± 68 ng ml−1 h. The Cmax and AUC0−∞ for the solid dispersion group were both statistically different (p < 0.05) from the premix group. However, no significant statistical difference was observed for pharmacokinetic parameters of solid dispersion such as elimination rate constant (Kel), elimination half-time (t1/2), and the peak time (Tmax) when compared to the premix. Meanwhile, the solid dispersion showed higher Cmax and AUC values than those of premix at the same time point, denoting that the in vivo dissolution is the rate-limiting step of in vivo absorption and bioavailability due to its high dependency on the drug solubility, namely, the amount of drug in the absorption site (43). The increase in AUC indicated that the developed silymarin solid dispersion improved the absorption of silymarin in vivo and was consistent with the in vitro dissolution profiles for both these preparations; both increased bioavailability and is a positive factor for reducing costs while increasing efficacy.
New drug development often relies on in vitro dissolution experiments because they can predict in vivo results based on in vitro data. This reduces development time and optimizes the formulation during pharmaceutical development (44). Silymarin is categorized as a class IV compound according to the Biopharmaceutical Classification System (BCS), so the likelihood for in vivo–in vitro correlations (IVIVC) would be small (45, 46). Nevertheless, we observed that the total amount of silymarin released in dissolution of the solid dispersion in pH 6.8 phosphate buffer solution was × 2.5 that of the premix, and Cmax and AUC in pharmacokinetics were about × 2.9 and × 2.2 that of the premix group. These proportional changes between pharmacokinetic parameters and amount dissolved in vitro indicated that in vitro dissolution data may be used to select pilot formulations and quality controls for production.
This study first showed the pharmacokinetics of silymarin in pigs with rapid absorption and elimination after oral administration. The pharmacokinetic parameters in pigs obtained in the study can be a theoretical foundation for establishing the clinically effective dose regimens of silymarin. The solid dispersion exhibited the higher Cmax and AUC compared to the premix, and that is consistent with in vitro dissolution data. This study provides a basis for further testing of silymarin solid dispersion.
The original contributions presented in the study are included in the article/supplementary material, further inquiries can be directed to the corresponding author/s.
The animal study was reviewed and approved by the Animal Research Committee of the South China Agriculture University.
XH and YX designed and coordinated the project and agreed to be accountable for all aspects of work ensuring integrity and accuracy. JL and TF performed the animal experiment and sample detection by HPLC. BH and LL contributed data processing analysis and chart drawing. The manuscript was drafted and critically revised by YX. All authors read and approved the final manuscript.
The study was financially supported by the National Key Research and Development Program of China (Grant No. 2016YFD0501306).
The authors declare that the research was conducted in the absence of any commercial or financial relationships that could be construed as a potential conflict of interest.
All claims expressed in this article are solely those of the authors and do not necessarily represent those of their affiliated organizations, or those of the publisher, the editors and the reviewers. Any product that may be evaluated in this article, or claim that may be made by its manufacturer, is not guaranteed or endorsed by the publisher.
1. Karimi G, Vahabzadeh M, Lari P, Rashedinia M, Moshiri M. “Silymarin”, a promising pharmacological agent for treatment of diseases. Iran J Basic Med Sci. (2011) 14:308–17.
2. Chambers CS, Holečková V, Petrásková L, Biedermann D, Valentová K, Buchta M, Kren V.. The silymarin composition and why does it matter??? Food Res Int. (2017) 100:339–53. doi: 10.1016/j.foodres.2017.07.017
3. Surai P. Silymarin as a natural antioxidant: an overview of the current evidence and perspectives. Antioxidants. (2015) 4:204–47. doi: 10.3390/antiox4010204
4. Comelli MC, Mengs U, Schneider C, Prosdocimi M. Toward the definition of the mechanism of action of silymarin: activities related to cellular protection from toxic damage induced by chemotherapy. Integr Cancer Ther. (2007) 6:120–9. doi: 10.1177/1534735407302349
5. Saller R, Melzer J, Reichling J, Brignoli R, Meier R. An updated systematic review of the pharmacology of silymarin. Complement Med Res. (2007) 14:70–80. doi: 10.1159/000100581
6. Vladimír Krena BDW. Silybin and silymarin – new effects and applications. Biomedical Papers. (2005) 149:29–41. doi: 10.5507/bp.2005.002
7. Kvasnička F, Biba B, Ševčik R, Voldrich M, Krátká J. Analysis of the active components of silymarin. J Chromatogr A. (2003) 990:239–45. doi: 10.1016/S0021-9673(02)01971-4
8. Esmaeil N, Anaraki SB, Gharagozloo M, Moayedi B. Silymarin impacts on immune system as an immunomodulator: One key for many locks. Int Immunopharmacol. (2017) 50:194–201. doi: 10.1016/j.intimp.2017.06.030
9. De La Puerta R, Martinez E, Bravo L, Ahumada MC. Effect of silymarin on different acute inflammation models and on leukocyte migration. J Pharm Pharmacol. (1996) 48:968–70. doi: 10.1111/j.2042-7158.1996.tb06014.x
10. KÖksal E, GÜLÇ NL, Beyza S, Sarikaya Ö, Bursal E. In vitro antioxidant activity of silymarin. J Enzym Inhib Med Chem. (2009) 24:395–405. doi: 10.1080/14756360802188081
11. Polyak SJ, Morishima C, Lohmann V, Pal S, Lee DYW, Liu Y, et al. Identification of hepatoprotective flavonolignans from silymarin. Proc Nat Acad Sci. (2010) 107:5995–9. doi: 10.1073/pnas.0914009107
12. Liu C, Jassey A, Hsu H, Lin L. Antiviral activities of silymarin and derivatives. Molecules. (2019) 24:1552. doi: 10.3390/molecules24081552
13. Wagoner J, Negash A, Kane OJ, Martinez LE, Nahmias Y, Bourne N, et al. Multiple effects of silymarin on the hepatitis C virus lifecycle. Hepatology. (2010) 51:1912–21. doi: 10.1002/hep.23587
14. Federico A, Dallio M, Loguercio C. Silymarin/silybin and chronic liver disease: a marriage of many years. Molecules. (2017) 22:191. doi: 10.3390/molecules22020191
15. Echtermann T, Muentener C, Sidler X, Kümmerlen D. Antimicrobial drug consumption on swiss pig farms: a comparison of swiss and european defined daily and course doses in the field. Front Vet Sci. (2019) 6:240. doi: 10.3389/fvets.2019.00240
16. Rosell C, Segalés J, Domingo M. Hepatitis and staging of hepatic damage in pigs naturally infected with porcine circovirus type 2. Vet Pathol. (2000) 37:687–92. doi: 10.1354/vp.37-6-687
17. Visentin M, Lenggenhager D, Gai Z, Kullak-Ublick GA. Drug-induced bile duct injury. Biochim Biophys Acta Mol Basis Dis. (2018) 1864:1498–506. doi: 10.1016/j.bbadis.2017.08.033
18. Singh D, Cho WC, Upadhyay G. Drug-induced liver toxicity and prevention by herbal antioxidants: an overview. Front Physiol. (2015) 6:363. doi: 10.3389/fphys.2015.00363
19. Hellerbrand C, Schattenberg JM, Peterburs P, Lechner A, Brignoli R. The potential of silymarin for the treatment of hepatic disorders. Clin Phytosci. (2016) 2:7. doi: 10.1186/s40816-016-0019-2
20. Tedesco D, Tava A, Galletti S, Tameni M, Varisco G, Costa A, et al. Effects of silymarin, a natural hepatoprotector, in periparturient dairy cows. J Dairy Sci. (2004) 87:2239–47. doi: 10.3168/jds.S0022-0302(04)70044-2
21. Grela E.R., Świa̧tkiewicz M., Florek M., Wojtaszewska I. Impact of milk thistle (Silybum marianum L.) seeds in fattener diets on pig performance and carcass traits and fatty acid profile and cholesterol of meat, backfat and liver. LIVEST SCI. (2020) 239. doi: 10.1016/j.livsci.2020.104180
22. Gazak R, Walterova D, Kren V. Silybin and silymarin–new and emerging applications in medicine. Curr Med Chem. (2007) 14:315–38. doi: 10.2174/092986707779941159
23. Martínez G, Diéguez SN, Rodríguez E, Decundo JM, Romanelli A, Fernández Paggi MB, et al. Effect of Cynara scolymus and Silybum marianum extracts on bile production in pigs. J Appl Anim Res. (2018) 46:1059–63. doi: 10.1080/09712119.2018.1456441
24. Wu J, Lin L, Tsai T. Drug–drug interactions of silymarin on the perspective of pharmacokinetics. J Ethnopharmacol. (2009) 121:185–93. doi: 10.1016/j.jep.2008.10.036
25. Qiuhong L, Liu Y, Ying W, Yue G, Tianying L. Pharmacokinetics and Tissue Distribution in Rats by Injection Use of Silybin Meglumine Salt. (2012) 29:58–61. doi: 10.3969/j.issn.1002-2406.2012.02.023
26. Gowthamarajan K, Singh SK. Dissolution testing for poorly soluble drugs: a continuing perspective. Dissolut Technol. (2010) 17:24–32. doi: 10.14227/DT170310P24
27. Woo JS, Kim TS, Park JH, Chi SC. Formulation and biopharmaceutical evaluation of silymarin using SMEDDS. Arch Pharm Res. (2007) 30:82–9. doi: 10.1007/BF02977782
28. Gažák R, Svobodová A, Psotová J, Sedmera P, Přikrylová V, Walterová D, et al. Oxidised derivatives of silybin and their antiradical and antioxidant activity. Bioorgan Med Chem. (2004) 12:5677–87. doi: 10.1016/j.bmc.2004.07.064
29. Pérez-Sánchez A, Cuyàs E, Ruiz-Torres V, Agulló-Chazarra L, Verdura S, González-Álvarez I, et al. Intestinal permeability study of clinically relevant formulations of silibinin in Caco-2 cell monolayers. Int J Mol Sci. (2019) 20:1606. doi: 10.3390/ijms20071606
31. Yang G, Zhao Y, Feng N, Zhang Y, Liu Y, Dang B. Improved dissolution and bioavailability of silymarin delivered by a solid dispersion prepared using supercritical fluids. Asian J Pharm Sci. (2015) 10:194–202. doi: 10.1016/j.ajps.2014.12.001
32. Theodosiou E, Purchartova K, Stamatis H, Kolisis F, R?en VRK. Bioavailability of silymarin flavonolignans: drug formulations and biotransformation. Phytochem Rev. (2014) 13:1–18. doi: 10.1007/s11101-013-9285-5
34. Saller R, Brignoli R, Melzer J, Meier R. An updated systematic review with meta-analysis for the clinical evidence of silymarin. Forsch Komplementärmed. (2008) 15:9–20. doi: 10.1159/000113648
35. Yanyu X, Yunmei S, Zhipeng C, Qineng P. Preparation of silymarin proliposome: a new way to increase oral bioavailability of silymarin in beagle dogs. INT J Pharmaceut. (2006) 319:162–8. doi: 10.1016/j.ijpharm.2006.03.037
36. Xie Y, Zhang D, Zhang J, Yuan J. Metabolism, transport and drug-drug interactions of silymarin. Molecules. (2019) 24:3693. doi: 10.3390/molecules24203693
37. Wen Z, Dumas TE, Schrieber SJ, Hawke RL, Fried MW, Smith PC. Pharmacokinetics and metabolic profile of free, conjugated, and total silymarin flavonolignans in human plasma after oral administration of milk thistle extract. Drug Metab Dispos. (2007) 36:65–72. doi: 10.1124/dmd.107.017566
39. Zhao J, Agarwal R. Tissue distribution of silibinin, the major active constituent of silymarin, in mice and its association with enhancement of phase II enzymes: implications in cancer chemoprevention. Carcinogenesis. (1999) 20:2101–8. doi: 10.1093/carcin/20.11.2101
40. Wu JW, Lin LC, Hung SC, Lin CH, Chi CW, Tsai TH. Hepatobiliary excretion of silibinin in normal and liver cirrhotic rats. Drug Metab Dispos. (2008) 36:589–96. doi: 10.1124/dmd.107.017004
41. Wu J, Lin L, Hung S, Chi C, Tsai T. Analysis of silibinin in rat plasma and bile for hepatobiliary excretion and oral bioavailability application. J Pharmaceut Biomed. (2007) 45:635–41. doi: 10.1016/j.jpba.2007.06.026
42. Weyhenmeyer R, Mascher H, Birkmayer J. Study on dose-linearity of the pharmacokinetics of silibinin diastereomers using a new stereospecific assay. Int J Clin Pharmacol Ther Toxicol. (1992) 30:134.
43. Takano R, Furumoto K, Shiraki K, Takata N, Hayashi Y, Aso Y, et al. Rate-limiting steps of oral absorption for poorly water-soluble drugs in dogs; prediction from a miniscale dissolution test and a physiologically-based computer simulation. Pharm Res-Dordr. (2008) 25:2334–44. doi: 10.1007/s11095-008-9637-9
44. Cardot J, Beyssac E, Alric M. In vitro–in vivo correlation: importance of dissolution in IVIVC. Dissolut Technol. (2007) 14:15–9. doi: 10.14227/DT140107P15
45. Gonzalez-Garcia I, Mangas-Sanjuan V, Merino-Sanjuan M, Bermejo M. In vitro-in vivo correlations: general concepts, methodologies and regulatory applications. Drug Dev Ind Pharm. (2015) 41:1935–47. doi: 10.3109/03639045.2015.1054833
Keywords: silymarin, solid dispersion, dissolution, pharmacokinetic, pigs
Citation: Xu Y, Li J, He B, Feng TS, Liang LJ and Huang XH (2022) In vitro Dissolution Testing and Pharmacokinetic Studies of Silymarin Solid Dispersion After Oral Administration to Healthy Pigs. Front. Vet. Sci. 9:815198. doi: 10.3389/fvets.2022.815198
Received: 15 November 2021; Accepted: 03 January 2022;
Published: 01 March 2022.
Edited by:
Perumal Subramanian, Annamalai University, IndiaReviewed by:
Fan Yang, Henan University of Science and Technology, ChinaCopyright © 2022 Xu, Li, He, Feng, Liang and Huang. This is an open-access article distributed under the terms of the Creative Commons Attribution License (CC BY). The use, distribution or reproduction in other forums is permitted, provided the original author(s) and the copyright owner(s) are credited and that the original publication in this journal is cited, in accordance with accepted academic practice. No use, distribution or reproduction is permitted which does not comply with these terms.
*Correspondence: Xianhui Huang, eGhodWFuZ0BzY2F1LmVkdS5jbg==
Disclaimer: All claims expressed in this article are solely those of the authors and do not necessarily represent those of their affiliated organizations, or those of the publisher, the editors and the reviewers. Any product that may be evaluated in this article or claim that may be made by its manufacturer is not guaranteed or endorsed by the publisher.
Research integrity at Frontiers
Learn more about the work of our research integrity team to safeguard the quality of each article we publish.