- 1National Risk Assessment Laboratory for Antimicrobial Resistance of Animal Original Bacteria, College of Veterinary Medicine, South China Agricultural University, Guangzhou, China
- 2Guangdong Laboratory for Lingnan Modern Agriculture, Guangzhou, China
- 3Guangdong Provincial Key Laboratory of Veterinary Pharmaceutics Development and Safety Evaluation, South China Agricultural University, Guangzhou, China
Danofloxacin is a synthetic fluoroquinolone with broad-spectrum activity developed for use in veterinary medicine. The aim of this study was to evaluate the pharmacokinetic/pharmacodynamic (PK/PD) targets, PK/PD cutoff values and the optimum doses of danofloxacin against P. multocida and H. parasuis in piglets. Single dose serum pharmacokinetics was determined in piglets after intravenous and intramuscular administration of 2.5 mg/kg. Danofloxacin was well absorbed and fully bioavailable (95.2%) after intramuscular administration of 2.5 mg/kg. The epidemiological cutoff (ECOFF) values of danofloxacin from 931 P. multocida isolates and 263 H. parasuis isolates were 0.03 and 4 mg/L, respectively. Danofloxacin MICs determined in porcine serum were markedly lower than those measured in artificial broth, with a broth/serum ratio of 4.33 for H. parasuis. Compared to P. multocida, danofloxacin exhibited significantly longer post-antibiotic effects (3.18–6.60 h) and post-antibiotic sub-MIC effects (7.02–9.94 h) against H. parasuis. The mean area under the concentration-time curve/MIC (AUC24h/MIC) targets of danofloxacin in serum associated with the static and bactericidal effects were 32 and 49.8, respectively, for P. multocida, whereas they were 14.6 and 37.8, respectively, for H. parasuis. Danofloxacin AUC24h/MIC targets for the same endpoints for P. multocida were higher than those for H. parasuis. At the current dose of 2.5 mg/kg, the PK/PD cutoff (COPD) values of danofloxacin against P. multocida and H. parasuis were calculated to be 0.125 and 0.5 mg/L, respectively, based on Monte Carlo simulations. The predicted optimum doses of danofloxacin for a probability of target attainment (PTA) of > 90% to cover the overall MIC population distributions of P. multocida and H. parasuis in this study were 2.38 and 13.36 mg/kg, respectively. These PK/PD-based results have potential relevance for the clinical dose optimization and evaluation of susceptibility breakpoints for danofloxacin in the treatment of swine respiratory tract infections involving these pathogens.
Introduction
P. multocida and H. parasuis play important roles in many outbreaks of swine respiratory disease (SRD) and act together to increase the severity and duration of lung damage caused by other symbiotic viruses and bacteria such as porcine circovirus type 2 and Streptococcus suis (1–3). Furthermore, studies indicated that P. multocida type A can act as the primary pathogen of porcine pneumonia and septicemia with a rising prevalence rate reported from 8% to 15.6% in China, Korea and United States (1, 4, 5). Due to the high prevalence of mixed infections with multiple bacterial species, the treatment of SRD generally includes the use of broad-spectrum antibiotics (6, 7). Fluoroquinolones, such as danofloxacin, possess excellent PK characteristics that may contribute to clinical success of treating SRD. Such advantages include high peak concentrations in plasma, extensive distribution to most tissues in animal body and deep penetration into lung fluids (8, 9). Despite these findings from previous studies, the precise pharmacokinetic/pharmacodynamic (PK/PD) targets and cutoff values of danofloxacin in pigs for SRD pathogens, especially for P. multocida have not been fully elucidated.
This study sought to determine and compare the PK/PD relationships of danofloxacin between P. multocida and H. parasuis with the goal to provide a framework for further study and optimization of danofloxacin dosing strategies for the treatment of bacterial respiratory mixed infections in piglets caused by SRD pathogens. By evaluating the drug kinetics, PK/PD targets, post-antibiotic effect (PAE) and postantibiotic sub-MIC effect (PA-SME), the optimum doses and PK/PD cutoffs (COPD) of danofloxacin were estimated for P. multocida and H. parasuis. A comparison of these results provides insights into the accurate antibiotic treatment for SRD.
Materials and Methods
Bacterial Strains and Susceptibility Testing
The MIC distribution of 931 swine-origin P. multocida isolates (Supplementary Table 1) was obtained by merging data from our own laboratory and some previous studies (10, 11). A total of 263 isolates of H. parasuis were gathered during 5-year surveillance study in different provinces of China from 2015 to 2020 (Supplementary Table 1). All the isolates were collected from diseased pigs suffering polyserositis, pneumonia or arthritis, and cultured with Haemophilus test medium (HTM) broth and agar containing 20 mg/L β-NAD and 5% lysed horse blood. Bacterial species was identified using the Axima Assurance MALDI-TOF mass spectrometer (Shimadzu Corp., Kyoto, Japan), as previously described (12). The MICs of danofloxacin for these isolates were determined using the broth microdilution in accordance with CLSI guidelines (13). To determine if there is a potentiation effect of serum on susceptibility, the danofloxacin MICs against P. multocida and H. parasuis were further determined in both broth and porcine serum.
Epidemiological Cutoff Values Determination
The ECOFF defines the upper end of the wild-type MIC distribution devoid of phenotypically detectable acquired resistance mechanisms (14). The isolates carrying plasmid-mediated quinolone resistance (PMQR) genes [qnrA-D, qepA, oqxAB and aac(6')-Ib-cr] were consequently removed (15). The log2-transformed MIC distribution of danofloxacin for P. multocida and H. parasuis was subjected to the statistical goodness-of-fit and non-linear least-squares regression tests to obtain optimum normal distribution (16). The final ECOFF value was calculated as the MIC that captured at least 95% of the optimum MIC distribution using the ECOFFinder program (16, 17).
PAEs and PA-SMEs Determination
Two P. multocida and three H. parasuis strains were selected to expose to danofloxacin at 1 × and 4 × MICs for 1 h. After removal of drug by centrifugation at 3,000 g for 10 min, bacterial cells were resuspended in drug-free broth (PAE) and broth containing 0.1 to 0.3 × MICs of danofloxacin (PA-SME) for continuous measurement of the absorbance at 600 nm. Optical density was converted into bacterial counts using a standard curve, as our previously reported (17). The PAEs and PA-SMEs of danofloxacin against P. multocida and H. parasuis were calculated as follow: PAE/PA-SME = T/TPA-C, where C is the time for 1-log10 control growth and T/TPA is the time for 1-log10 growth after drug removal (T) or in the sub-MIC treated phase (TPA) (18).
Pharmacokinetics of Danofloxacin in Piglets
Twelve healthy crossbred piglets (Duroc × Landrace × Yorkshire, 9.3 ± 1.9 kg from Jiahe Agricultural Stockbreeding Co., Qingyuan, China) were used for a two-period crossover study. Animal experimental protocols were approved by the Animal Ethics Committee of South China Agricultural University (approval no. 2018014). Each piglet received danofloxacin (Injectable solution; lot no. 190201; Hainan Yuqi Pharmaceutical Co., Dingan, China) at a dose of 2.5 mg/kg b.w. by intravenous (IV) and intramuscular (IM) injections. Feed and water were provided ad libitum. The dose of danofloxacin was chosen based on previous PK studies in pigs and the manufacturer's instruction (19–22). The serums samples for danofloxacin concentration determination were collected from the jugular veins into vacutainers without anticoagulant prior to dosing (0 h) and at 0.08, 0.25, 0.5, 0.75, 1, 2, 4, 6, 8, 12, 24, 36, and 48 h after administrations of danofloxacin.
Danofloxacin concentrations in serum samples were measured by a liquid chromatography-tandem mass spectrometry (LC-MS/MS) method (details are given in Supplementary Data Sheet 1). All PK parameters were calculated using the compartmental models in WinNonlin software (version 5.2; Pharsight, St. Louis, MO, USA). The Akaike information criterion (AIC) was used to guide the selection of the best PK model to describe the observed time-concentration data. Danofloxacin average bioavailability (F%) after intramuscular injection was calculated by dividing each AUCIM value by their respective AUCIV value for each individual piglet according to the following standard equation (23): F% = AUCinfinity(IM) / AUCinfinity(IV) ×100%.
Ex vivo Time-Kill and PK/PD Index Target for Efficacy
The abilities of danofloxacin to kill P. multocida and H. parasuis were assessed ex vivo as previously described (17). Serum samples collected from piglets at different time points were filtered to avoid bacterial contamination. Bacterial cells were subcultured and inoculated to each serum sample, giving an initial inoculum of ~106cfu/mL. The mixtures were serially diluted and plated using a drop-plate technique to enumerate bacterial CFUs after 3, 6, 9 and 24 h of incubation. The limit of detection (LOD) was 40 cfu/mL.
The correlation between antibacterial efficacy and the PK/PD parameter AUC24h/MIC was determined by the non-linear WinNonlin regression program (version 5.2; Pharsight, St. Louis, MO, USA). The AUC/MIC ratio was chosen as the predictive PK/PD parameter as previous studies have demonstrated this index to be predictive for fluoroquinolones (24, 25). The sigmoid Emax model used was derived from the Hill equation: E = E0 + Emax × CN / (EC50N + CN), where E0 is the log10 change of bacterial count in the absence of danofloxacin, Emax is the maximum effect, C is the PK/PD index (AUC24h/MIC), EC50 is the AUC24h/MIC required to achieve 50% of the Emax and N is the slope of the dose-response curve. The coefficient of determination (R2) was used to estimate the variance due to regression with the PK/PD parameter AUC/MIC. The AUC24h/MIC targets in serum required to produce bacteriostatic (E = 0), bactericidal (E = −3) and eradication (E = −4) effects were calculated for each drug-organism combination.
PK/PD Cutoff Determination and Dose Assessment
Based on PK parameters and calculated PK/PD targets (AUC24h/MIC) for bactericidal effect, a 10,000-subject Monte Carlo simulation was conducted to obtain the danofloxacin PK/PD cutoffs (COPD) for P. multocida and H. parasuis using Crystal Ball software (version 11.1.2, Oracle Corporation) (26). The AUC24h/MIC was calculated with the following formula: AUC24h/MIC = Dose / (Cl × MIC). Clearance (Cl) was assumed to be normally distributed in the form of mean ± SD (Table 1). Scenarios were simulated separately at each possible MIC. The COPD was defined as the highest MIC at which the PTA was ≥90% (27).
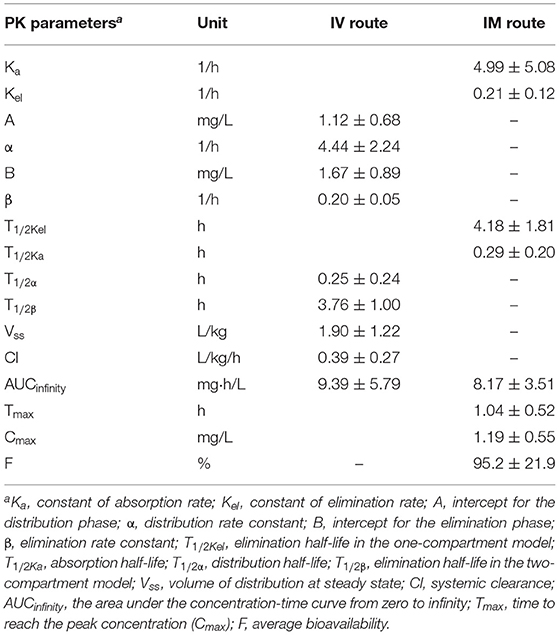
Table 1. The PK parameters of danofloxacin in porcine serum following single dose intravenous (IV) and intramuscular (IM) administrations at 2.5 mg/kg.
In order to ascertain the optimum dose regimens of danofloxacin to cover the overall MIC population distributions in this study (931 P. multocida strains and 263 H. parasuis isolates), the two population distributions of danofloxacin doses were predicted by a 10,000-subject Monte Carlo simulation. The dose was calculated by the equation as follow (17, 28): Dose = (Cl × AUC/MIC × MICdistribution) / (fu × F), where Cl is the body clearance; AUC/MIC is the PK/PD target required for a bactericidal effect, in this case, the AUC24h/MIC of 49.8 and 37.8 for P. multocida and H. parasuis, respectively; a scaling factor of 4.33 was used to bridge the MIC differences between HTM and serum when dose distribution was predicted for H. parasuis; fu is free drug fraction using protein binding rate of 44% in porcine serum (29); F is the bioavailability of IM administration.
Results
MICs and ECOFF Determination
Of 931 P. multocida isolates, the fitted MIC distribution [Log2 mean (−6.06) ± SD (0.27)] contained > 95% that possessed danofloxacin MICs ≤ 0.03 mg/L, and the ECOFF was consequently calculated to be 0.03 mg/L for P. multocida (Figure 1A). No difference in MIC was observed for P. multocida between broth and serum. However, of the 14 H. parasuis isolates tested, geometric mean of danofloxacin MIC in serum was significantly lower than that in HTM, with a HTM/serum ratio of 4.33 (P < 0.05; Supplementary Figure 1). The MICs of danofloxacin against our 263 clinical H. parasuis isolates ranged from 0.004 to 128 mg/L in HTM, with the MIC50 and MIC90 of 0.25 and 4 mg/L, respectively (Supplementary Table 1). In order to obtain a unimodal distribution, the 10 isolates with MICs of ≥64 mg/L were therefore removed. More than 95% of the best fitting normal distribution [Log2 mean (−2.21) ± SD (2.49)] was in the range of 0.004 to 4 mg/L, thus the ECOFF value was determined to be 4 mg/L for H. parasuis (Figure 1B).
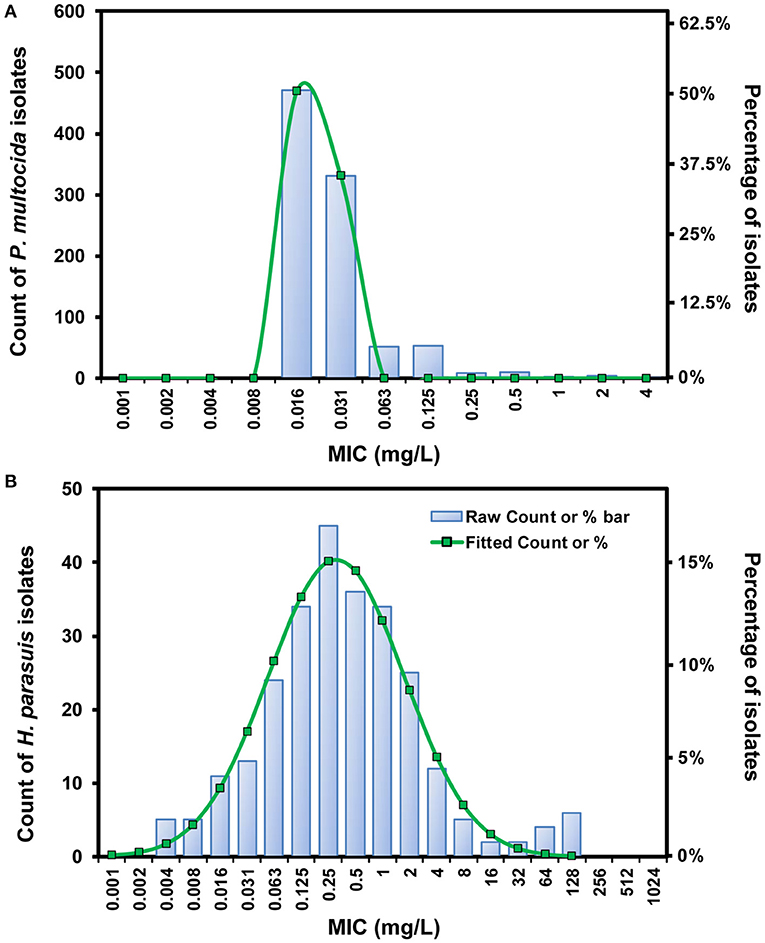
Figure 1. The log2-transformed MIC distribution of danofloxacin against swine-origin P. multocida (A) and H. parasuis (B). The MIC distribution of P. multocida isolates (n = 931) was created by merging data from our laboratory and some previous studies (10, 11). H. parasuis isolates (n = 263) and MIC data were obtained in our own laboratory from 2015 to 2020. The number of isolates and the observed frequency corresponding to each MIC value are shown along the y-axes. The lines represent predicted frequency based on the best fitting log2-normal distribution [log2 mean (−6.06) ± SD (0.27) for P. multocida and log2 mean (−2.21) ± SD (2.49) for H. parasuis, respectively].
PAEs and PA-SMEs
PAEs were calculated after removal of bacterial cells from danofloxacin exposures at 1 × and 4 × MICs. Persistent regrowth inhibition was observed in a concentration-dependent manner, resulting in PAE values of 0.96–4.46 h for P. multocida and 2.42–6.92 h for H. parasuis, respectively (Figures 2A,B). The addition of sub-MIC danofloxacin during the post-antibiotic phase substantially delayed bacterial regrowth, producing PA-SMEs of 4.30–6.86 h for P. multocida and 7.02–9.94 h for H. parasuis, respectively (Figure 2C). Despite this fact, the mean bacterial densities in the presence of sub-MIC danofloxacin remained at a lower level than their respective growth controls until at least 12 h (Figure 2; Supplementary Figure 2). Of note, the time suppression of regrowth (PAE and PA-SME) for H. parasuis was significantly longer relative to P. multocida (P < 0.05; two-tailed unpaired Students t-test; Figure 2C).
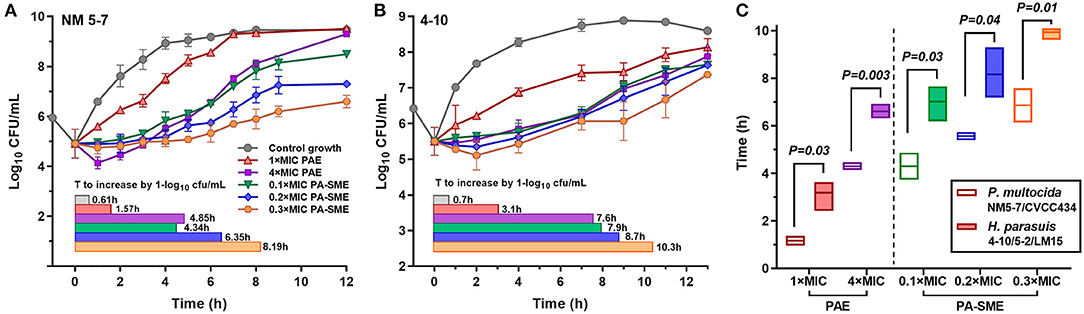
Figure 2. The postantibiotic effects (PAEs) and post-antibiotic sub-MIC effects (PA-SMEs) of danofloxacin. (A,B) The PAEs were measured after initial exposure to danofloxacin at 1× and 4× MICs against P. multocida (A; strain NM5-7) and H. parasuis (B; strain 4–10). The PA-SMEs were measured after initial exposure to danofloxacin at 4 × MICs. The color horizontal bars represent the time that required bacterial counts to increase by 1.0-log10cfu/mL after drug removal (PAE) or at the sub-MIC phase (PA-SME). (C) Comparison of danofloxacin PAE and PA-SME between P. multocida and H. parasuis. Two P. multocida isolates and three H. parasuis isolates were tested and included. Statistical significance was determined using the two-tailed unpaired Student t-test (P < 0.05).
Danofloxacin PKs in Piglets
A two-compartmental model fit was shown for time-concentration profile of danofloxacin after IV injection (Figure 3), which was consistent with previous results observed in both healthy and infected pigs (29). Notably, the decline in serum danofloxacin concentrations was bi-exponential with half-lives of (T1/2α) 0.25 h and (T1/2β) 3.76 h for distribution and elimination phases, respectively (Table 1). After IM dosing of danofloxacin, the mean peak concentration (Cmax; 1.19 mg/L) was reached in serum within 1.04 h. While the prolonged terminal half-life (T1/2Kel; 4.18 h) was observed in serum following IM dosing, AUCinfinity values were comparable regardless of administration routes, indicating a high bioavailability of 95.2% after IM administration (Table 1).
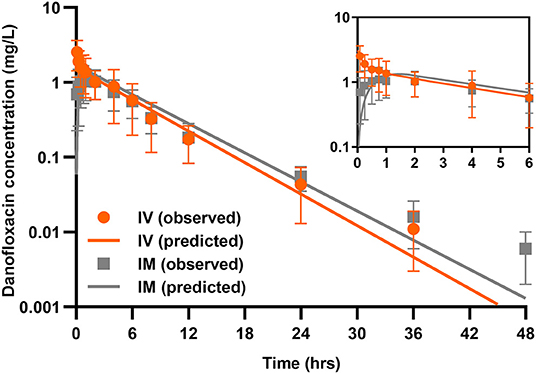
Figure 3. Danofloxacin pharmacokinetics in piglets. Serum concentrations of danofloxacin following single dose intravenous (IV) and intramuscular (IM) administrations at 2.5 mg/kg in piglets (n = 12 per time point). Upper right panel shows details from 0 to 6 h. The lines drawn through the time points indicated the best-fitting lines based on the compartment models.
Ex vivo Antimicrobial Activities and PK/PD Targets
Rapid activity against P. multocida strain NM5-7 (MICserum = 0.13 mg/L) was demonstrated with porcine serum collected up to 12 h at concentrations of 0.22 to 1.12 mg/L (Figure 4A). Notably, a concentration-dependent trend toward a greater level of P. multocida killing was observed with increasing danofloxacin concentrations in serums. Bacterial densities of P. multocida were driven below detectable limits by serums collected up to 6 h after 9 h of incubation (Figure 4A). Concentration-dependent killing activity was similarly observed for all H. parasuis strains tested (Figure 4B; Supplementary Figure 3). Accordingly, ex vivo activity was negligible for serums at 36 and 48 h, while sustained bactericidal activity was attained within 9 h of exposure to serums containing danofloxacin > 0.5 mg/L (Supplementary Figure 3). For H. parasuis 4–10 (MICserum = 0.06 mg/L), complete bactericidal activities reaching undetectable limits of eradication were noted within 24 h in response to serums collected up to 12 h (Figure 4B).
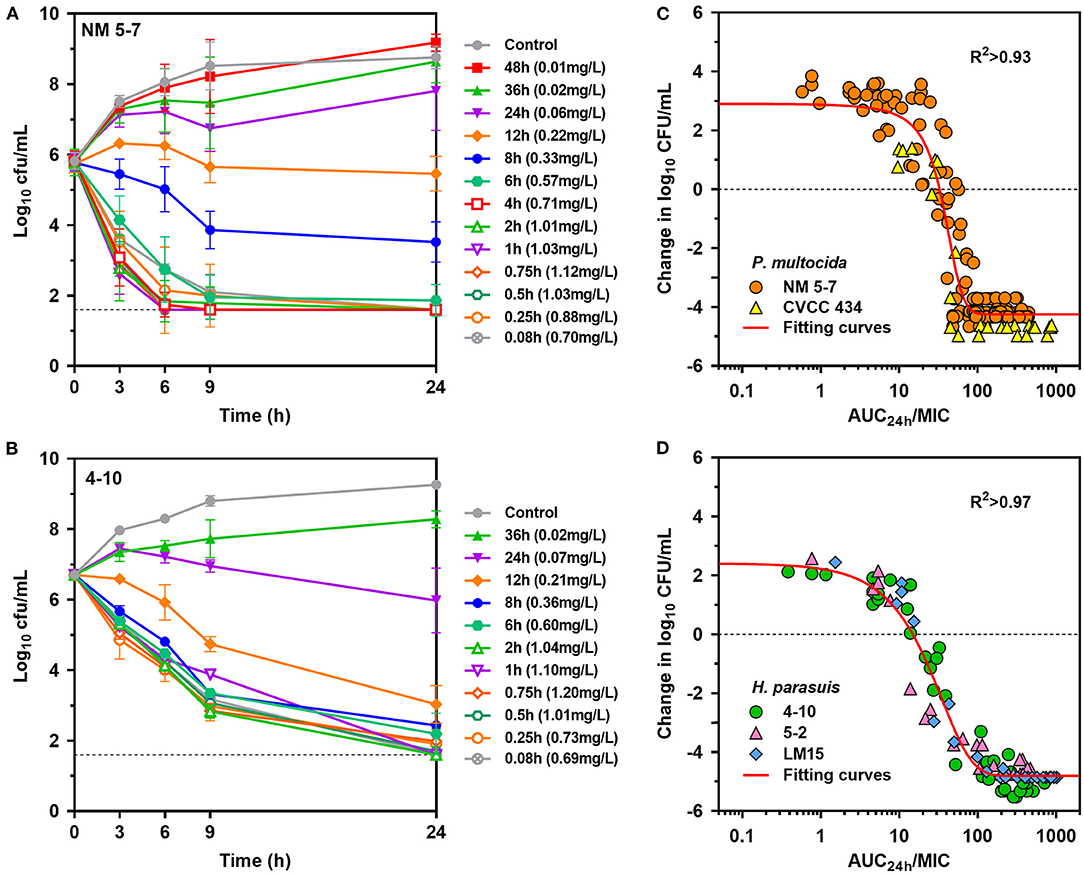
Figure 4. Ex vivo activity and PK/PD relationships of danofloxacin. (A,B) Ex vivo time-kill curves of danofloxacin against P. multocida [(A) strain NM5-7; MICserum = 0.13 mg/L] and H. parasuis [(B) strain 4-10; MICserum = 0.06 mg/L] in serums of piglets receiving intramuscular injection of danofloxacin (2.5 mg/kg b.w.). Numerical values on right brackets represent the mean concentrations of danofloxacin in serums collected from different time points post-dosing. (C,D) Correlation plots between ex vivo activity and AUC24h/MIC of danofloxacin using the sigmoid Emax equation. The fitting curves represent predicted values (two P. multocida and three H. parasuis included), and the points represent values of individual serum samples collected from 0 to 48 h.
PK/PD analyses of the ex vivo time-kill data were performed to determine AUC/MIC targets of danofloxacin associated with the optimal activity. PK/PD relationships between AUC/MIC and ex vivo activity were strong, with an R2 of > 0.93 (Figures 4C,D). For P. multocida, the mean AUC/MIC targets in serum for bacteriostatic, bactericidal and eradication effects were 32, 49.8 and 66.9, respectively. Of note, the PK/PD targets for H. parasuis were much lower than those for P. multocida (P < 0.05, two-tailed unpaired Student's t-test). Serum AUC/MIC targets for the same endpoints were 14.6, 37.8 and 62.9 (Table 2).
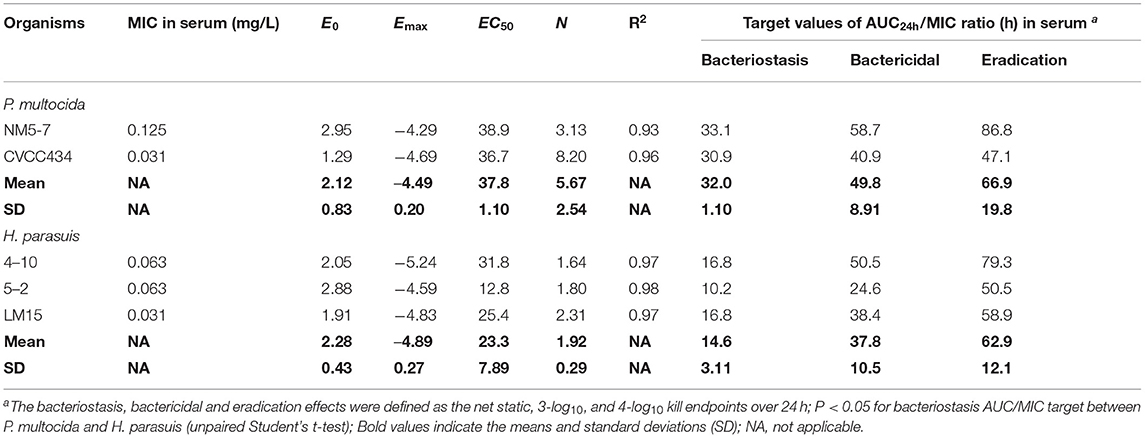
Table 2. PK/PD targets of danofloxacin in serum (AUC24h/MIC) necessary to achieve the bacteriostasis, bactericidal, and eradication effects for the study organisms in piglets.
PK/PD Cutoff Determination and Dose Prediction
The probabilities of the current dose regimen (2.5 mg/kg) achieving typical AUC/MIC targets at each possible MIC were determined by a 10,000-iteration Monte Carlo simulation, from which PTAs were estimated (Figure 5). With a target AUC/MIC ratio of 49.8 (i.e., bactericidal action for P. multocida), the PTA was still 87.3% at a MIC of 0.125 mg/L. The COPD value of danofloxacin for P. multocida was consequently determined to be 0.125 mg/L (Figure 5A). In view of the significant potentiation effect of serum on activity of danofloxacin for H. parasuis (Supplementary Figure 1), a scaling factor of 4.33 was created to bridge the MIC variation between HTM and serum when calculating the COPD for H. parasuis. The PTA for AUC/MIC ratio of 37.8 was only 26.1% at a MIC of 1.0 mg/L and reached 99.7% when the MIC was 0.5 mg/L. The COPD of danofloxacin against H. parasuis was therefore defined as a MIC of 0.5 mg/L (Figure 5B).
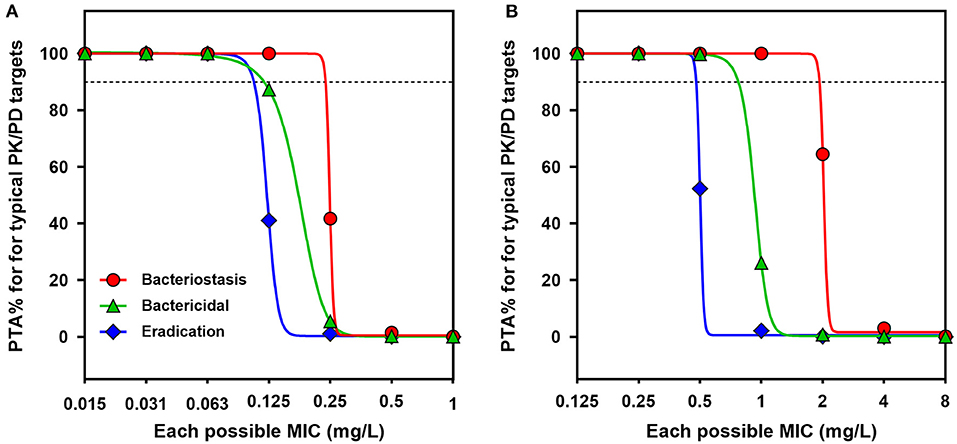
Figure 5. PK/PD cutoff values for danofloxacin against P. multocida and H. parasuis. (A,B) Probability of target attainment (PTA) for typical AUC24h/MIC targets (bacteriostatic, bactericidal and eradication effects) at each possible MIC when treated with danofloxacin at dose of 2.5 mg/kg against P. multocida (A) and H. parasuis (B) infections. Dotted lines denote the PTA of 90%.
Based on the results of the current PK parameters, PK/PD targets and the MIC distribution, if danofloxacin was given once daily intramuscularly in piglets, the predicted dosages for a PTA of 90% to cover the overall MIC population distributions in this study were 2.38 and 13.36 mg/kg (Figure 6), which were estimated to be effective achieving a bactericidal effect against P. multocida and H. parasuis, respectively.
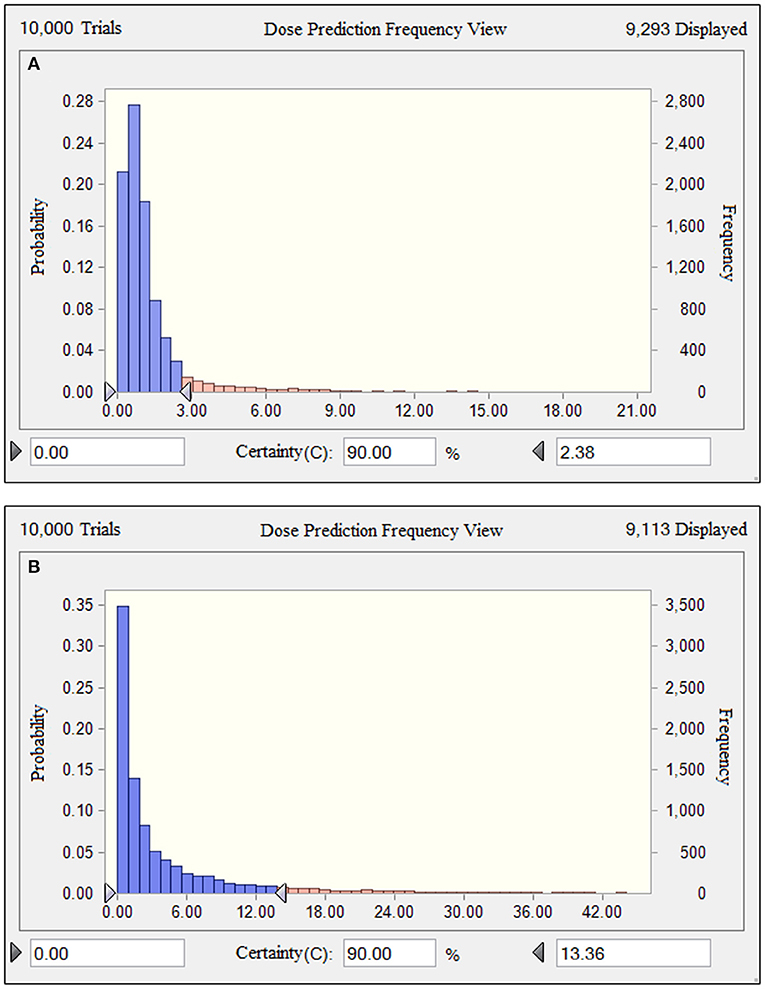
Figure 6. Comparison of population distributions of danofloxacin doses for P. multocida (A) and H. parasuis (B). The right triangle depicted the calculated target doses for a PTA of > 90% to cover the overall MIC population distributions in this study.
Discussion
In this study, we observed a marked potentiation effect of porcine serum on danofloxacin activities for H. parasuis but not for P. multocida. This finding was in agreement with the previous studies showing that incorporation of the increasing amounts of serum to broth progressively reduced macrolides MICs for seven bacterial species harvested from pigs (28, 30). On the contrary, reduced antimicrobial activity in serum was observed for moxifloxacin against Staphylococcus aureus (31). It is therefore likely that serum potentiation effect is strain- and drug-codependent (32). The complement and specific antibody are considered as the key factors responsible for increased serum activity (33). In general, a prolong PAE has a high likelihood of predicting favorable outcomes (34). The findings of our study exhibiting the notable PAEs from 0.96 to 6.92 h in a concentration-dependent manner for both P. multocida and H. parasuis, point to clinical treatment with fluoroquinolones that cloud be administered at a longer dosing interval without loss of efficacy. It is well known that fluoroquinolones disrupt DNA synthesis by binding bacterial gyrase and topoisomerase, the PAEs induced by danofloxacin may represent the lag time for drug to dissociate from binding sites and to diffuse out of bacteria (35).
Similar to other fluoroquinolones, danofloxacin have good penetration into pulmonary epithelial lining fluid (ELF). A previous bronchopulmonary PK study with danofloxacin demonstrated higher peak concentration in ELF compared to plasma in pigs, with a mean ELF/plasma AUC ratio of 5.4 (9). Of note, the pharmacokinetic profiles of danofloxacin were linear and proportional in piglets as described by the result of linear regression analysis (R2 = 0.951 for AUC24h) (36). The similarity and proportionality of PK profiles potentially reflects passive diffusion of danofloxacin from plasma to ELF. In this case, serum could be used as a predictive surrogate for PD target assessment, although the value of PD target could be relatively high (37). The PD targets associated with bactericidal action in previous fluoroquinolone studies has been a total AUC24h/MIC of 88 for H. parasuis, and values 1.5- to 5-fold higher (121–451) for gram-negative pathogens such as Escherichia coli and Salmonella typhimurium (38–40). In our study with danofloxacin, the PD targets were lower for each of the bacteria tested. This difference was most profound for H. parasuis with a mean bactericidal AUC24h/MIC of 37.8. Similarly, the AUC24h/MIC target identified for P. multocida (49.8) was modestly lower than marbofloxacin with a bactericidal AUC24h/MIC of 64.9 (41). These endpoints were roughly 2- to 9-fold lower than comparative PD studies for veterinary fluoroquinolones. The notable PK/PD efficacies for both H. parasuis and P. multocida provide a fairly robust option for treating SRD, especially in situations of bacterial coinfections due to mixed species.
At the current clinical dose of 2.5 mg/kg, the ECOFF and COPD values of danofloxacin against P. multocida were determined to be 0.03 and 0.125 mg/L, respectively. This is similar with the EUCAST MIC breakpoint (0.06 mg/L) used for levofloxacin and ciprofloxacin against P. multocida (42). The clinical trial has been previously conducted to investigate danofloxacin efficacy in Danish swine herds with a naturally occurring outbreak of acute Pasteurella pneumonia. A satisfactory response to treatment with 1.25 mg/kg danofloxacin was observed in 87% of the diseased pigs (43). For H. parasuis, danofloxacin COPD value (0.5 mg/L) was 8-fold greater than ciprofloxacin MIC breakpoint against Haemophilus influenzae (0.06 mg/L), but was equivalent to PK/PD breakpoints (0.25–0.5 mg/L) of other fluoroquinolones such as ofloxacin moxifloxacin (42). Of note, danofloxacin ECOFF for H. parasuis (4 mg/L) was higher compared to the corresponding COPD (0.5 mg/L) in this study. The over-estimated ECOFF value could be due to other unknown resistance mechanisms and the limited number of strains collected. A similar cutoff value (≥2 mg/L) was observed for enrofloxacin against Haemophilus somnus (13). This result suggested that danofloxacin at 2.5 mg/kg may be insufficient to combat swine respiratory infections due to H. parasuis with high-level MICs of > 4 mg/L. Indeed, a higher danofloxacin dosage of 13.36 mg/kg is required to achieve a PTA of > 90% for bactericidal effect against the overall H. parasuis isolates collected in this study.
Conclusion
In summary, we have demonstrated a large potentiation effect of serum on the potency of danofloxacin for H. parasuis. Compared with P. multocida, the PAEs and PA-SMEs of danofloxacin were substantially longer for H. parasuis. The PK/PD targets and cutoff values identified in this study will be useful in guiding the optimum dosing regimen design for danofloxacin in the context of specific PK exposure and MIC distribution, and in the development of clinical breakpoints for the treatment of SRD involving these pathogens.
Data Availability Statement
The original contributions presented in the study are included in the article/Supplementary Material, further inquiries can be directed to the corresponding author/s.
Ethics Statement
The animal study was reviewed and approved by the South China Agricultural University (SCAU) Institutional Ethics Committee (Approval No. 2018014). Written informed consent was obtained from the owners for the participation of their animals in this study.
Author Contributions
Y-FZ and X-PL designed this study. Y-FZ and ZS wrote the manuscript. ZS, R-LW, J-GL, C-YN, X-AL, and Y-YF carried out the experiments. JS and Y-HL analyzed the data. All authors read and approved the final manuscript.
Funding
This work was supported by the Laboratory of Lingnan Modern Agriculture Project (NT2021006), the Foundation for Innovative Research Groups of the National Natural Science Foundation of China (32121004), National Natural Science Foundation of China (31902318), the Local Innovative and Research Teams Project of Guangdong Pearl River Talents Program (2019BT02N054), Program for Changjiang Scholars and Innovative Research Team in University of Ministry of Education of China (IRT_17R39), the Found for Fostering Talents of College of Veterinary Medicine of South China Agricultural University (5500A17003), and the Innovative Team Project of Guangdong University (2019KCXTD001).
Conflict of Interest
The authors declare that the research was conducted in the absence of any commercial or financial relationships that could be construed as a potential conflict of interest.
Publisher's Note
All claims expressed in this article are solely those of the authors and do not necessarily represent those of their affiliated organizations, or those of the publisher, the editors and the reviewers. Any product that may be evaluated in this article, or claim that may be made by its manufacturer, is not guaranteed or endorsed by the publisher.
Supplementary Material
The Supplementary Material for this article can be found online at: https://www.frontiersin.org/articles/10.3389/fvets.2022.811967/full#supplementary-material
References
1. Liu H, Zhao Z, Xi X, Xue Q, Long T, Xue Y. Occurrence of Pasteurella multocida among pigs with respiratory disease in China between 2011 and 2015. Ir Vet J. (2017) 70:2. doi: 10.1186/s13620-016-0080-7
2. Liu S, Li W, Wang Y, Gu C, Liu X, Charreyre C, et al. Coinfection with Haemophilus parasuis serovar 4 increases the virulence of porcine circovirus type 2 in piglets. Virol J. (2017) 14:227. doi: 10.1186/s12985-017-0890-6
3. Zhu H, Chang X, Zhou J, Wang D, Zhou J, Fan B, et al. Co-infection analysis of bacterial and viral respiratory pathogens from clinically healthy swine in Eastern China. Vet Med Sci. (2021) 7:1815–9. doi: 10.1002/vms3.533
4. Oliveira JXD, Morés MA, Rebelatto R, Agnol A, Plieski CL, Klein CS, et al. Pasteurella multocida type A as the primary agent of pneumonia and septicaemia in pigs. Pesquisa Veterinária Brasileira. (2015) 35:716–24. doi: 10.1590/S0100-736X2015000800003
5. Kim J, Kim JW, Oh SI, So B, Kim WI, Kim HY. Characterisation of Pasteurella multocida isolates from pigs with pneumonia in Korea. BMC Vet Res. (2019) 15:119. doi: 10.1186/s12917-019-1861-5
6. Cheong Y, Oh C, Lee K, Cho KH. Survey of porcine respiratory disease complex-associated pathogens among commercial pig farms in Korea via oral fluid method. J Vet Sci. (2017) 18:283–9. doi: 10.4142/jvs.2017.18.3.283
7. Sargeant JM, Bergevin MD, Churchill K, Dawkins K, Deb B, Dunn J, et al. A systematic review of the efficacy of antibiotics for the prevention of swine respiratory disease. Anim Health Res Rev. (2019) 20:291–304. doi: 10.1017/S1466252319000185
8. Friis C. Penetration of danofloxacin into the respiratory tract tissues and secretions in calves. Am J Vet Res. (1993) 54:1122–7.
9. Xu Z, Huang A, Luo X, Zhang P, Huang L, Wang X, et al. Exploration of Clinical Breakpoint of Danofloxacin for Glaesserella parasuis in Plasma and in PELF. Antibiotics. (2021) 10:808. doi: 10.3390/antibiotics10070808
10. Sweeney MT, Lindeman C, Johansen L, Mullins L, Murray R, Senn MK, et al. Antimicrobial susceptibility of Actinobacillus pleuropneumoniae, Pasteurella multocida, Streptococcus suis, and Bordetella bronchiseptica isolated from pigs in the United States and Canada, 2011 to 2015. J Swine Health Product. (2017) 25:106–20. doi: 10.3389/fmicb.2019.02430
11. Cid D, Fernández-Garayzábal JF, Pinto C, Domínguez L, Vela AI. Antimicrobial susceptibility of Pasteurella multocida isolated from sheep and pigs in Spain. Acta Vet Hung. (2019) 67:489–98. doi: 10.1556/004.2019.048
12. Moreno LZ, Silva GF, Gomes VT, Matajira CE, Silva AP, Mesquita RE, et al. Application of protein profiling of virulent Haemophilus parasuis by MALDI-TOF mass spectrometry. J Infect Dev Ctries. (2016) 10:678–81. doi: 10.3855/jidc.7787
13. CLSI. (2020). Performance Standards for Antimicrobial Disk and Dilution Susceptibility Tests for Bacteria Isolated From Animals: Approved Standard, 5th ed. Wayne: CLSI supplement VET01S.
14. Toutain PL, Bousquet-Melou A, Damborg P, Ferran AA, Mevius D, Pelligand L, et al. En Route towards European clinical breakpoints for veterinary antimicrobial susceptibility testing: a position paper explaining the VetCAST Approach. Front Microbiol. (2017) 8:2344. doi: 10.3389/fmicb.2017.02344
15. Guo L, Zhang J, Xu C, Zhao Y, Ren T, Zhang B, et al. Molecular characterization of fluoroquinolone resistance in Haemophilus parasuis isolated from pigs in South China. J Antimicrob Chemother. (2011) 66:539–42. doi: 10.1093/jac/dkq497
16. Turnidge J, Kahlmeter G, Kronvall G. Statistical characterisation of bacterial wild-type MIC value distributions and the determination of epidemiological cut-off values. Clin Microbiol Infect. (2006) 12:418–25. doi: 10.1111/j.1469-0691.2006.01377.x
17. Zhou YF, Bu MX, Liu P, Sun J, Liu YH, Liao XP. Epidemiological and PK/PD cutoff values determination and PK/PD-based dose assessment of gamithromycin against Haemophilus parasuis in piglets. BMC Vet Res. (2020) 16:81. doi: 10.1186/s12917-020-02300-y
18. Zhou YF, Yu Y, Sun J, Tao MT, Zhou WJ, Li X, et al. Ex vivo pharmacokinetic/pharmacodynamic relationship of valnemulin against Clostridium perfringens in plasma, the small intestinal and caecal contents of rabbits. Anaerobe. (2016) 39:150–7. doi: 10.1016/j.anaerobe.2016.04.005
19. Lauritzen B, Lykkesfeldt J, Friis C. Evaluation of a single dose a divided dose regimen of danofloxacin in treatment of Actinobacillus pleuropneumoniae infection in pigs. Res Vet Sci. (2003) 74:271–7. doi: 10.1016/S0034-5288(03)00029-8
20. Wang C, Ai D, Chen C, Lin H, Li J, Shen H, et al. Preparation and evaluation of danofloxacin mesylate microspheres and its pharmacokinetics in pigs. Vet Res Commun. (2009) 33:1013–22. doi: 10.1007/s11259-009-9320-6
21. Yang Y, Zhang Y, Li J, Cheng P, Xiao T, Muhammad I, et al. Susceptibility breakpoint for Danofloxacin against swine Escherichia coli. BMC Vet Res. (2019) 15:51. doi: 10.1186/s12917-019-1783-2
22. Yang Y, Cheng P, Xiao T, Ulziikhutag J, Yu H, Li J, et al. Pharmacokinetics and pharmacodynamics integration of danofloxacin against Eschrichia coli in piglet ileum ultrafiltration probe model. Sci Rep. (2021) 11:681. doi: 10.1038/s41598-020-80272-7
23. Toutain PL, Bousquet-Melou A. Bioavailability and its assessment. J Vet Pharmacol Ther. (2004) 27:455–66. doi: 10.1111/j.1365-2885.2004.00604.x
24. Lepak AJ, Andes DR. In Vivo Pharmacodynamic Target Assessment of Delafloxacin against Staphylococcus aureus, Streptococcus pneumoniae, and Klebsiella pneumoniae in a murine lung infection model. Antimicrob Agents Chemother. (2016) 60:4764–9. doi: 10.1128/AAC.00647-16
25. Zhou YF, Tao MT, Huo W, Liao XP, Sun J, Liu YH. In Vivo pharmacokinetic and pharmacodynamic profiles of antofloxacin against Klebsiella pneumoniae in a neutropenic murine lung infection model. Antimicrob Agents Chemother. (2017) 61:e02691–e02616. doi: 10.1128/AAC.02691-16
26. Tao MT, Zhou YF, Sun J, Liu YH, Liao XP. Establishment of valnemulin susceptibility breakpoint against Clostridium perfringens in rabbits. Anaerobe. (2017) 48:118–20. doi: 10.1016/j.anaerobe.2017.08.006
27. Turnidge J, Paterson DL. Setting and revising antibacterial susceptibility breakpoints. Clin Microbiol Rev. (2007) 20:391–408. doi: 10.1128/CMR.00047-06
28. Zhou YF, Peng HM, Bu MX, Liu YH, Sun J, Liao XP. Pharmacodynamic evaluation and pk/pd-based dose prediction of tulathromycin: a potential new indication for Streptococcus suis infection. Front Pharmacol. (2017) 8:684. doi: 10.3389/fphar.2017.00684
29. Lindecrona RH, Friis C, Nielsen JP. Pharmacokinetics and penetration of danofloxacin into the gastrointestinal tract in healthy and in Salmonella typhimurium infected pigs. Res Vet Sci. (2000) 68:211–6. doi: 10.1053/rvsc.1999.0361
30. Rose M, Menge M, Bohland C, Zschiesche E, Wilhelm C, Kilp S, et al. Pharmacokinetics of tildipirosin in porcine plasma, lung tissue, and bronchial fluid and effects of test conditions on in vitro activity against reference strains and field isolates of Actinobacillus pleuropneumoniae. J Vet Pharmacol Ther. (2013) 36:140–53. doi: 10.1111/j.1365-2885.2012.01397.x
31. Zeitlinger M, Sauermann R, Fille M, Hausdorfer J, Leitner I, Muller M. Plasma protein binding of fluoroquinolones affects antimicrobial activity. J Antimicrob Chemother. (2008) 61:561–7. doi: 10.1093/jac/dkm524
32. Lees P, Illambas J, Potter TJ, Pelligand L, Rycroft A, Toutain PL. A large potentiation effect of serum on the in vitro potency of tulathromycin against Mannheimia haemolytica and Pasteurella multocida. J Vet Pharmacol Ther. (2017) 40:419–28. doi: 10.1111/jvp.12372
33. Ramos-Sevillano E, Rodríguez-Sosa C, Cafini F, Giménez MJ, Navarro A, Sevillano D, et al. Cefditoren and ceftriaxone enhance complement-mediated immunity in the presence of specific antibodies against antibiotic-resistant pneumococcal strains. PLoS One. (2012) 7:e44135. doi: 10.1371/journal.pone.0044135
34. Craig WA. Post-antibiotic effects in experimental infection models: relationship to in-vitro phenomena and to treatment of infections in man. J Antimicrob Chemother 31 Suppl D. (1993) 149–58. doi: 10.1093/jac/31.suppl_D.149
35. Carbone M, Pennisi MG, Masucci M, De Sarro A, Giannone M, Fera MT. Activity and postantibiotic effect of marbofloxacin, enrofloxacin, difloxacin and ciprofloxacin against feline Bordetella bronchiseptica isolates. Vet Microbiol. (2001) 81:79–84. doi: 10.1016/S0378-1135(01)00349-2
36. Zhang L, Kang Z, Yao L, Gu X, Huang Z, Cai Q, et al. Pharmacokinetic/pharmacodynamic integration to evaluate the changes in susceptibility of Actinobacillus pleuropneumoniae after repeated administration of danofloxacin. Front Microbiol. (2018) 9:2445. doi: 10.3389/fmicb.2018.02445
37. Berkhout J, Melchers MJ, Van Mil AC, Seyedmousavi S, Lagarde CM, Nichols WW, et al. Pharmacokinetics and penetration of ceftazidime and avibactam into epithelial lining fluid in thigh- and lung-infected mice. Antimicrob Agents Chemother. (2015) 59:2299–304. doi: 10.1128/AAC.04627-14
38. Sang K, Hao H, Huang L, Wang X, Yuan Z. Pharmacokinetic-Pharmacodynamic Modeling of Enrofloxacin Against Escherichia coli in Broilers. Front Vet Sci. (2015) 2:80. doi: 10.3389/fvets.2015.00080
39. Sun J, Xiao X, Huang RJ, Yang T, Chen Y, Fang X, et al. In vitro Dynamic Pharmacokinetic/Pharmacodynamic (PK/PD) study and COPD of Marbofloxacin against Haemophilus parasuis. BMC Vet Res. (2015) 11:293. doi: 10.1186/s12917-015-0604-5
40. Xiao X, Pei L, Jiang LJ, Lan WX, Xiao JY, Jiang YJ, et al. In Vivo Pharmacokinetic/Pharmacodynamic Profiles of Danofloxacin in Rabbits Infected With Salmonella typhimurium After Oral Administration. Front Pharmacol. (2018) 9:391. doi: 10.3389/fphar.2018.00391
41. Potter T, Illambas J, Pelligand L, Rycroft A, Lees P. Pharmacokinetic and pharmacodynamic integration and modelling of marbofloxacin in calves for Mannheimia haemolytica and Pasteurella multocida. Vet J. (2013) 195:53–8. doi: 10.1016/j.tvjl.2012.08.027
42. EUCAST. (2021). The European Committee on Antimicrobial Susceptibility Testing. Breakpoint tables for interpretation of MICs and zone diameters. Version 11.0, 2021. (2021). Available online at: http://www.eucast.org (accessed January 19, 2022).
Keywords: PK/PD, cutoff, danofloxacin, P. multocida, H. parasuis
Citation: Zhou Y-F, Sun Z, Wang R-L, Li J-G, Niu C-Y, Li X-A, Feng Y-Y, Sun J, Liu Y-H and Liao X-P (2022) Comparison of PK/PD Targets and Cutoff Values for Danofloxacin Against Pasteurella multocida and Haemophilus parasuis in Piglets. Front. Vet. Sci. 9:811967. doi: 10.3389/fvets.2022.811967
Received: 09 November 2021; Accepted: 12 January 2022;
Published: 02 February 2022.
Edited by:
Moussa Sory Diarra, Agriculture and Agri-Food Canada (AAFC), CanadaReviewed by:
Ji Yu Zhang, Lanzhou Institute of Husbandry and Pharmaceutical Sciences, Chinese Academy of Agricultural Sciences (CAAS), ChinaKun Li, Nanjing Agricultural University, China
Copyright © 2022 Zhou, Sun, Wang, Li, Niu, Li, Feng, Sun, Liu and Liao. This is an open-access article distributed under the terms of the Creative Commons Attribution License (CC BY). The use, distribution or reproduction in other forums is permitted, provided the original author(s) and the copyright owner(s) are credited and that the original publication in this journal is cited, in accordance with accepted academic practice. No use, distribution or reproduction is permitted which does not comply with these terms.
*Correspondence: Xiao-Ping Liao, eHBsaWFvJiN4MDAwNDA7c2NhdS5lZHUuY24=; Yu-Feng Zhou, enlmJiN4MDAwNDA7c2NhdS5lZHUuY24=