- 1Federal Institute of Amapá, Macapa, Amapá, Brazil
- 2Embrapa Eastern Amazon, Santarém, Pará, Brazil
- 3Embrapa Eastern Amazon, Belém, Pará, Brazil
- 4Federal Rural University of the Amazon, Belém, Pará, Brazil
- 5Federal University of Tocantins, Araguaína, Tocantins, Brazil
- 6Postgraduate Program in Animal Science, Federal University of Pará, Belém, Brazil
- 7Federal University of Pará, Castanhal, Pará, Brazil
- 8Embrapa Southeast Livestock, São Carlos, São Paulo, Brazil
The use of palm kernel cake as an alternative to conventional ingredients, due to the presence of residual fat, can also reduce methane emissions. The objective of the study was to evaluate, in two different experiments, the effects of palm kernel cake supplementation on feed intake, enteric methane production and estimates, and the ingestive behavior of buffaloes in the Amazon biome. In experiment 1, to evaluate feed intake, methane production, and feed efficiency, 20 crossbred females, dry and empty, with a mean age of 34 months and an initial body weight of 514 ± 69 kg, were supplemented with palm kernel cake for 60 days. The supply was calculated in relation to body weight (BW) in four treatments: 0% (control); 0.25, 0.50, and 1% of palm kernel cake, distributed in a completely randomized design. In experiment 2, to evaluate the ingestive behavior, 24 mixed-breed, dry, and non-pregnant buffaloes supplemented with palm kernel cake were evaluated in the less rainy season (LR) and the wettest season (WS) of the eastern Amazon, distributed in a completely randomized in the same treatments as experiment 1. The inclusion of palm kernel cake in the supplementation increased the feed intake of dry matter and components (MM, OM, CP, NDF, ADF, and EE) (P < 0.01), reducing the production of enteric methane intake (P < 0.01), the ratio per kg of meat produced (P < 0.01) and feed efficiency (P < 0.01), and influenced the ingestive behavior (time grazing, rumination, and idleness) during the day. We suggest that further research be carried out to verify the results and improve the use of this co-product as a methanogenesis mitigator.
1. Introduction
Ruminants, due to enteric fermentation, are known as an important emitter of methane (CH4) and contributors to global climate change (1). Methane is one of the main greenhouse gases, and livestock is responsible for about 30% of the anthropogenic production of these gases (2). However, changes in their diet can influence the ruminal microflora, promote better energy use, and reduce methanogenesis and environmental impacts (3).
In this context, agro-industry co-products have been widely studied and present themselves as promising alternatives (4–6). The valorization and use of this material are fundamental for the agri-food sector, as it allows the advancement of the circular economy model with a balance between economic development and environmental and resource protection (7). In addition, they have a low cost compared to conventional ingredients (corn and soy) and an important nutritional composition for ruminant diets.
Palm kernel cake, for example, has ~17% crude protein; 7.7 MJ/kg/MS metabolizable energy; and 60% neutral detergent fiber (8, 9). It is the co-product of palm oil extraction and has between 6 and 16% residual ether extract. In addition to being an important energy source, residual fat promotes the selection of ruminal microorganisms, reducing the population of methanogenic bacteria and energy losses (10, 11).
This is a bioactive function that has been widely studied in animal nutrition because, due to the benefits mentioned, they are possible substitutes for ionophores. The use of these antibiotics in animal feed has been banned in some countries due to the spread of resistance in bacterial communities, which poses some risks to human health (5, 12).
In this context, it is hypothesized that there is a level of palm kernel cake that improves feed intake, does not influence ingestive behavior, but reduces enteric methane emissions. Given the concern about waste disposal, global warming, and the efficiency of diets for ruminants, the objective of this study was to evaluate the effects of palm kernel cake supplementation on methanogenesis, ingestive behavior, and feed efficiency in buffaloes in the Amazon.
2. Materials and methods
2.1. Ethics committee
The experiment was approved by the Ethics Committee for the Use of Animals (CEUA) of the Universidade Federal Rural da Amazônia (UFRA), under Protocol No 007/2015.
2.2. Location
Two experiments were carried out, at the Animal Research Unit “Senador Álvaro Adolfo” (01°25'S e 48°26'W), belonging to Embrapa Eastern Amazon, in the municipality of Belém—PA. According to the Köppen classification, the climate is of type Afi (A—tropical humid climates, without a cold season and with the average temperature of the least hot month above 18°C; F—Tropical forest climate, with relatively abundant rainfall at all times of the year; i—when the amplitude between average temperatures of the hottest month and the coldest month is below 5°C); the average annual rainfall of 3,001 mm is well distributed over the months with the wettest season (WS) from January to June and less rainy (LR) season from July to December. The average annual temperature is 26°C, with relative humidity around 86%.
2.3. Experiment 1
For the evaluation of feed intake, emission of enteric methane and estimates, and feed efficiency, were used 20 buffalo females, without a defined racial pattern, dry and non-pregnant, with age and an initial average weight of 32 ± 05 months and 476 ± 39 kg, respectively. The animals, belonging to the herd of Embrapa Eastern Amazon, were distributed in a completely randomized design with 4 treatments (palm kernel cake levels in relation to body weight of 0, 0.25, 0.50, and 1%) and five replications. The values offered were determined from the literature based on the inclusion of co-products in pasture supplements.
Associated with each diet, in all treatments, 0.15% of body weight (BW) of wheat bran was included in order to provide a better acceptability of the supplement. The experiment took place during the months of September and October and used corn silage (CS) as a fodder source.
The animals, confined in individual pens, were adapted for 21 days to experimental diets, facilities, and management, with access to water and mineral mixtures ad libitum. The diets were offered in two meals: 50% at 07:00 h and the other 50% at 15:00 h. The amount of roughage offered was weighed daily and adjusted according to the animals feed intake to secure 15% leftovers.
2.3.1. Feed intake and chemical composition
The feed intake of nutritional components was determined by the difference between the amount of each component contained in the feed provided and the amount contained in the leftovers. The chemical composition of the diets was performed at the Animal Nutrition Laboratory of the Federal University of Pará (Table 1). The evaluation of the dry matter (DM), organic (OM), ether extract (EE), and mineral (MM) contents of the ingredients was carried out in accordance with the recommendations of the AOAC (13). The sequential method described by Van Soest et al. (14) was used to determine neutral detergent fiber (NDF) and acid detergent fiber (ADF), and the Kjeldahl method was used to determine crude protein (CP).
2.3.2. Methane production
Methane (CH4) emission was estimated by the sulfur hexafluoride (SF6) tracer gas technique (15). A capsule that releases SF6 is implanted in the rumen. The gas release rate is known in advance and assumes that the SF6 emission standard simulates the CH4 emission standard (16). The animals were adapted for 15 days to reduce stress.
A collection and storage diaper in a 60 mm class 20 PVC tube was used, with an internal pressure close to 0 atm, calibrated to reach half an atm pressure at the end of the collection period, using a stainless steel capillary tube with 0.127 mm of inner diameter attached to a halter. The calibration was determined by the length of the capillary tube, which, in this case, was ~4 cm for a period of 24 h.
The diaper was connected to the capillary tube by means of a quick coupling. After the animals had adapted to the sampling apparatus, ruminal gas collections were performed over 5 consecutive days, at 24-h intervals. At 07:00 h 30 min, the animals were removed from the stalls and taken to the management corral, where samples were collected.
The diapers collected were transported to the laboratory, where the samples were diluted and the pressurization was measured until it reached an approximate pressure of 1.2 atm with the injection of nitrogen (the pressure readings were taken on a digital meter ± 0.01). The calibration curves were established using gas standards certified by White Martins/Praxair, with concentrations in ppt (34 ± 9, 91 ± 9, and 978 ± 98 ppt) for SF6 and for measuring CH4 in ppm (4.85 and 20 ppm), according to Westberg et al. (17).
The CH4 and SF6 concentrations were determined in a Model 7890A gas chromatograph, equipped with a flame ionization detector (FID), megabore column (0.53 μm, 30 m), Plot HP-Al/M (for CH4), electron capture (μ-ECD), and HP-MolSiv megabore column (for SF6).
Equivalent emissions were calculated as the ratio between the methane produced and dry matter feed intake, kg of meat produced, feed intake of neutral detergent fiber, feed intake of acid detergent fiber, and feed intake of ether extract.
2.3.3. Feed efficiency
With the total daily dry matter intake (total DMI/kg day) and the average daily gain (ADW), the feed efficiency (FE) of the animals was calculated using the following formula: FE = DWG/total DMI, where total DMI = total daily dry matter intake; DWG = daily weight gain, in kg/day; and FE = feed efficiency.
2.4. Experiment 2
2.4.1. Ingestive behavior
The second experiment was carried out, where the evaluations took place in the months of May (wettest season—WS) and September (season less rain—LR). The evaluation took place visually, by the instantaneous scan method, at 5-min intervals for 24 h (13), in 8 periods of 3 h, to determine the time spent in feeding, rumination, and idleness (18).
It was decided to divide the day into 3-h intervals; thus, 8 periods were obtained (11:00–14:00; 14:00–17:00; 17:00–20:00; 20:00–23:00; 23:00–02:00; 02:00–05:00; 05:00–08:00; and 08:00–11:00 h). The data obtained from each 3-h interval was organized as a percentage of the total time. For rumination time, regurgitation, re-chewing and re-swallowing of the feed bolus were accepted. Grazing time included selection, obtaining and handling of feed, and chewing and swallowing the bolus. For the idleness, the time when they were not feeding, eating, or ruminating was counted. For evaluation according to the Amazonian seasons, two observations were carried out, one for LR and another for WS.
2.5. Statistical analysis
Feed intake, production of methane and equivalents, and feed efficiency were analyzed by means of the analysis of variance and regression, with the degrees of freedom broken down into linear or quadratic effects, with a significance of up to 5%, using the PROC GLIMMIX of the Statistical Analysis System—SAS version 9.1 (19), according to the statistical model below:
where Yij = value observed in the plot that received treatment i in repetition j; μ = overall average; NLi = fixed effect of oil palm inclusion level i (i = 0, 0.25, 0.5, and 1%); and εij = random experimental error associated with each observation assumed NID ~ (0, σ2).
For ingestive behavior: Analysis of variance in a subdivided plot scheme use the model: Yij = μ + αi + βj + αi*βj + εij, where Yij = observed variables (ingestive behavior); μ = overall mean; αi = effect of the i-th level of factor α (TP0, TP0.25, TP0.5, and TP1) observed in Yij; βj = effect of the j-th level of the β factor (11:00–14:00; 14:00–17:00; 17:00–20:00; 20:00–23:00; 23:00–02:00; 02:00–05:00; 05:00–08:00; and 08:00–11:00 h); αi*βj = effect of the interaction α and β; and εi = random error (εij ~ IN (0, σ2)), with t-test at 5% significance level.
For regression analysis: Y = α + βx + ε, where Y = observed variables (feed intake and performance); x1 = supply level (TP0, TP0.25, TP0.5, and TP1); α and β = regression coefficients; and ε = random error (ε ~ IN (0, σ2)), using the SAS (19) software.
3. Results
3.1. Feed intake
The palm kernel cake supplementation showed a linear increase (P < 0.01) in the nutrient intake (Table 2). Dry matter intake increased linearly, reaching 7.87 kg/day at the maximum supplementation level (TP1), a value 32.8% higher than the control treatment. This increase in dry matter feed intake reflected in the increase, also linear, of the intake of the other fractions, especially the ADF and EE, which were increased by 56 and 300%, respectively.
Crude protein and NDF intakes reached, at the maximum level of cake inclusion, 0.85 and 4.65, against 0.53 and 3.6 kg/day of TP0, respectively. For mineral matter, the increase was 25%, reaching 0.39 kg/day at the maximum level of TP, and organic matter increased from 5.59 (TP0) to 7.49 (TP1).
3.2. Enteric methane production and feed efficiency
Enteric methane production decreased linearly with increased TP inclusion in the supplement (P < 0.01). In the control treatment, the production of CH4 kg/year was 66.04, compared with 26.43 in the supply of 1% of palm kernel cake (Table 3).
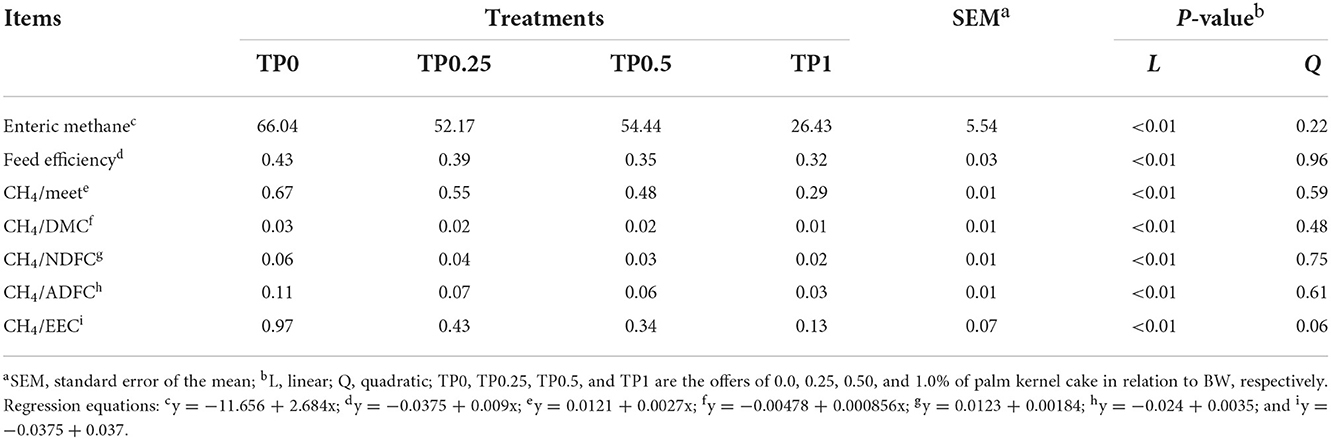
Table 3. Enteric methane production, feed efficiency, and estimates of emissions in female buffaloes supplemented with palm kernel cake.
Feed efficiency and estimates of emissions (methane production/kg of meat produced, production methane/DM feed intake, production methane/DNF feed intake, production methane/ADF feed intake, and production methane/EE feed intake) were also lower when the inclusion level of PT was higher (P < 0.05). As highlighted, the methane emission per kg of meat produced is 131% lower when the inclusion of TP in the supplementation is 1%.
3.3. Ingestive behavior
There was no interaction between the levels of palm kernel cake (P > 0.05); however, the periods of day influenced the rumination and idle time (P < 0.05). The longest feeding times occurred in the periods between 14:00–17:00, 23:00, and 02:00 h, but there was no difference between them (Table 4).
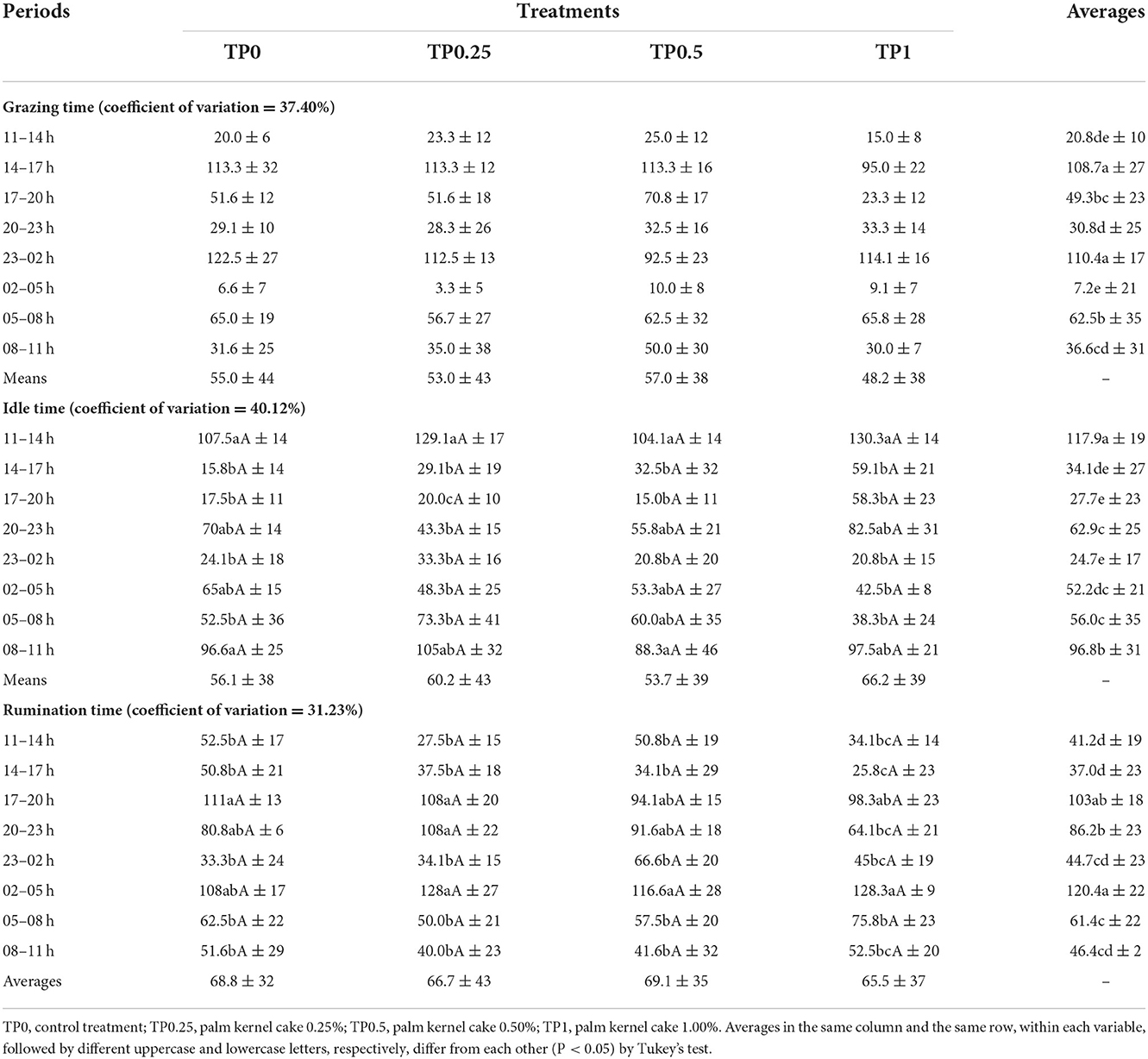
Table 4. Grazing, idleness, and ruminating time in female buffaloes supplemented with palm kernel cake during the less rainy season (LR).
The time spent for idleness was influenced by the periods of the day, being higher overnight, with an average of 56.04, 96.88, and 117.91 for the periods 05:00–08:00, 08:00–11:00, and 11:00–14:00 h, respectively (P < 0.05). Rumination time was also influenced only in the periods of the day (P < 0.05), with a shorter time observed in TP1 animals, from 11:00 to 17:00 h.
The highest means of rumination occurred from 02:00 to 05:00 h, with no differences between treatments and an average of 120.42, reducing at times of higher feeding frequency. The times of lowest rumination occurred between 11:00 and 17:00 h, time of intense feeding or idleness.
At dawn and early morning, there was an effect for the grazing time variable (P < 0.05). The longest feeding times occurred between 14:00–17:00 (rainy time), 20:00–23:00, and 2:00–5:00 h (milder temperature) (Table 5). During the WS period, there was an effect for idleness between 11:00 and 14:00 h, reaching an average of 126.66 (P < 0.05).
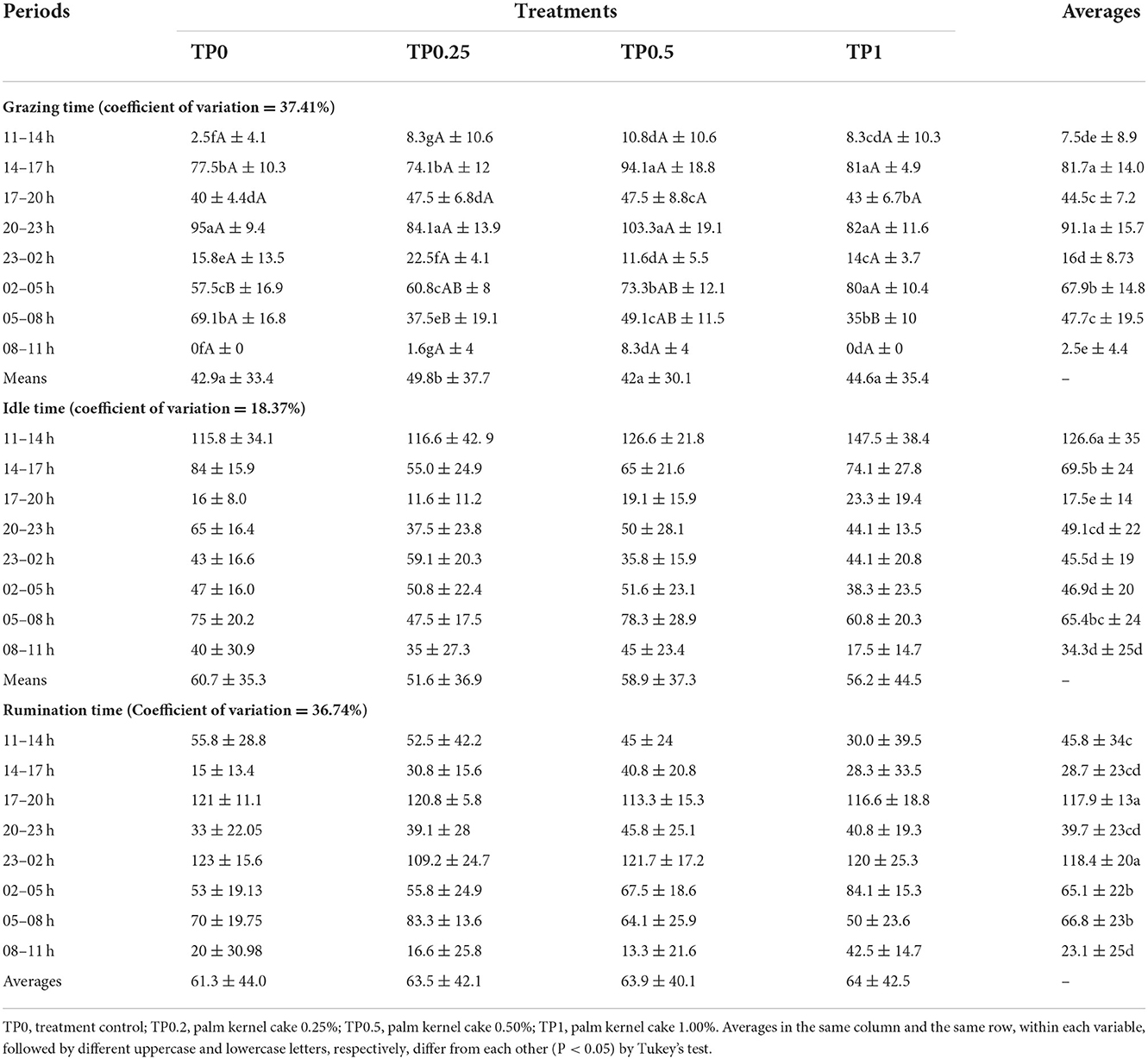
Table 5. Grazing, idleness, and ruminating time in female buffaloes supplemented with palm kernel cake during the less rainy season (MAC).
Except between 23:00 and 02:00 h, there was also an effect of the periods on the rumination time (P < 0.05), being the period 17:00–20:00 h different from the others. The lowest rumination averages occurred from 8:00 to 11:00 h and from 14:00 to 17:00 h, but there was no difference between them.
4. Discussion
4.1. Feed intake
It is recommended not to exceed 7% lipid in ruminant diets, as despite the benefits, it can reduce fiber digestibility and feed intake (20, 21). Supplementation with up to 1% of palm kernel cake increased the lipid content of the diets and feed intake. This was possibly due to an increase in ADF and lignin levels and, consequently, reduced digestibility (6, 22). Thus, to meet the nutritional requirements, animals are compensated with increases in DMI and dietary nutrients (23). However, it is worth mentioning that the increase in ether extract, ADF, and lignin in the supplement was not enough to limit feed intake.
4.2. Methane production
Alternative ingredients such as co-products, secondary plant compounds, essential oils, and lipids still show inconsistent results in mitigating methanogenesis, ranging from substantial reduction (24–26), to stability or a modest increase in enteric emissions (27–29).
These results are probably due to the composition of the diet and, mainly, to the differences in the lipid source of the ingredient (28). There are fatty acid profiles that are more effective in reducing CH4, making studies with alternative feeds even more important (2, 30).
Thus, the inclusion of palm oil cake, whose main fatty acids are oleic, myristic and palmitic (9), and which have an inhibitory action on GHG production (31, 32), endorse the results obtained.
At levels above 0.50% (PC), the inclusion of palm kernel cake in the diet of female buffaloes had a bioactive function and reduced the enteric methane production of the animals, with values below the average emission in buffaloes estimated at 55.00 kg/year (33).
This result, when compared to the effectiveness of ionophores, once again shows promise, since the reduction between the most distant treatments was 40.02%, higher than most studies with antibiotics (34). Ionophores are intended for the control of proteolytic rumen bacteria; thus, they present more modest reductions in terms of methane mitigation, appearing in the results only as a co-benefit of their use (34, 35). Furthermore, the mitigation of CH4 by these antibiotics, unlike lipid sources, is reduced in the short term by the selection of more resistant microorganisms, posing a threat to public health (36, 37).
4.3. Feed efficiency and estimates of productions
The reduction in feed efficiency was probably due to the increase in ADF and lignin in diets with PT, allied to the increase in DMC. Acid detergent fiber intake among the most distant treatments was 1.56 times higher, reducing digestibility and causing a compensatory increase in feed intake. Furthermore, the increase in EE intake can lead to a reduction in NDF digestibility, reflecting a 34% drop in feed efficiency.
However, the increase in EE intake with the inclusion of PT had a bioactive effect on methanogenic rumen microorganisms, reducing not only the production of enteric methane but also the amount emitted per kilogram of meat produced. This is an important indicator of the efficiency of PT inclusion for the production of more environmentally friendly meat (38, 39), and it has been widely studied (40–42).
The inclusion of the cake in the supplementation, through modulations in the rumen environment, also allowed lower CH4 emission per kilogram of DM, NDF, ADF, and EE consumed, demonstrating its efficiency as a bioactive compound in ruminant diets in the mitigation of greenhouse gases (GHG).
4.4. Ingestive behavior
The time spent on rumination, feeding, and idleness is directly influenced by diet (43). The nature of the diet, especially the fiber quality, is a determining factor in the oscillation of rumination time and, consequently, feeding, and idleness (44).
Supplementation with concentrates, in turn, as well as weather conditions, also influence the times chosen to perform each activity. Thus, as the availability and quality of the pasture and the inclusion of supplements were common to all, the ingestive behavior of the animals was not influenced. However, the evaluated climatic conditions (LR and WS) influenced the distribution of activities in the periods of the day.
Possibly, this is due to the supply of supplementation and the strategies adopted by the animals to overcome situations of intense heat or rain (45). Immediately after the offer, the animals start feed intake, and, in reflex, the rumination activity is also influenced since it occurs soon after ingestion.
5. Conclusion
Supplementation with palm kernel cake is a promising alternative. Its inclusion in the diet increases feed intake, reduces enteric methane production, and improves the proportion of methane per kg of meat produced. We suggest that further research be carried out to verify the results and improve the use of this co-product as a methanogenesis mitigator.
Data availability statement
The raw data supporting the conclusions of this article will be made available by the authors, without undue reservation.
Ethics statement
The animal study was reviewed and approved by the Ethics Committee for the Use of Animals (CEUA) of the Universidade Federal Rural da Amazônia (UFRA), under Protocol N° 007/2015. Written informed consent was obtained from the owners for the participation of their animals in this study.
Author contributions
Experiment design: ALS, AB, JL, and AS. Experiment performed: VC, JA, and LS. Data curation: LM, BN, TR, AS, and JL. Formal analysis: ALS, JS, AS, and AB. Writing-original draft: AS, JL, TR, and JS. All authors contributed to the article and approved the submitted version.
Funding
Thanks to Embrapa Eastern Amazon, Rede PECUS, and collaborators of the Animal Research Unit; Marborges Agroindústria S.A. for donating the co-product; Coordination for the Improvement of Higher Education Personnel (CAPES) and also financial support for the publication fee from the Pro-Rectory of Research and Graduate Studies (PROPESP/UFPA).
Conflict of interest
The authors declare that the research was conducted in the absence of any commercial or financial relationships that could be construed as a potential conflict of interest.
Publisher's note
All claims expressed in this article are solely those of the authors and do not necessarily represent those of their affiliated organizations, or those of the publisher, the editors and the reviewers. Any product that may be evaluated in this article, or claim that may be made by its manufacturer, is not guaranteed or endorsed by the publisher.
References
1. Zhao Y, Nan X, Yang L, Zheng S, Jiang L, Xiong B. A review of enteric methane emission measurement techniques in ruminants. Animals. (2020) 10:1004. doi: 10.3390/ani10061004
2. Beauchemin KA, Ungerfeld EM, Abdalla AL, Alvarez C, Arndt C, Becquet P, et al. Invited review: current enteric methane mitigation options. J Dairy Sci. (2022) 105:9297–326. doi: 10.3168/jds.2022-22091
3. Pratama SM, Wajizah S, Jayanegara A. Evaluation of agro-industrial by products as potential local feed for ruminant animals: volatile fatty acid and NH3 concentration, gas production and methane emission. In: IOP Conference Series: Earth and Environmental Science, Vol. 425. Bristol: IOP Publishing (2020). p. 012010. doi: 10.1088/1755-1315/425/1/012010
4. Halmemies-Beauchet-Filleau A, Rinne M, Lamminen M, Mapato C, Ampapon T, Wanapat M, et al. Alternative and novel feeds for ruminants: nutritive value, product quality and environmental aspects. Animal. (2018) 12:s295–309. doi: 10.1017/S1751731118002252
5. Marcos CN, Carro MD, Yepes JF, Haro A, Romero-Huelva M, Molina-Alcaide E. Effects of agroindustrial by-product supplementation on dairy goat milk characteristics, nutrient utilization, ruminal fermentation, and methane production. J Dairy Sci. (2020) 103:1472–83. doi: 10.3168/jds.2019-17386
6. Rodrigues TCGC, Santos SA, Cirne LGA, dos Santos Pina D, Alba HDR, de Araújo MLGML, et al. Palm kernel cake in high-concentrate diets for feedlot goat kids: nutrient intake, digestibility, feeding behavior, nitrogen balance, blood metabolites, and performance. Trop Animal Health Prod. (2021) 53:1–11. doi: 10.1007/s11250-021-02893-y
7. Serrapica F, Masucci F, Raffrenato E, Sannino M, Vastolo A, Barone CMA, et al. High fiber cakes from mediterranean multipurpose oilseeds as protein sources for ruminants. Animals. (2019) 9:918. doi: 10.3390/ani9110918
8. Ribeiro RDX, Medeiros AN, Oliveira RL, de Araújo GGL, Queiroga RDCDE, Ribeiro MD, et al. Palm kernel cake from the biodiesel industry in goat kid diets. Part 2: physicochemical composition, fatty acid profile and sensory attributes of meat. Small Ruminant Res. (2018) 165:1–7. doi: 10.1016/j.smallrumres.2018.05.014
9. Rodrigues TCGC, Santos SA, Cirne LGA, Pina DDS, Alba HDR, de Araújo MLGML, et al. Palm kernel cake in high-concentrate diets improves animal performance without affecting the meat quality of goat kids. Anim Prod Sci. (2022) 62:78–89. doi: 10.1071/AN21129
10. Sun J, Xu J, Shen Y, Wang M, Yu L, Wang H. Effects of different dietary ratio of physically effective neutral detergent fiber and metabolizable glucose on rumen fermentation, blood metabolites and growth performance of 8–10-month-old heifers. Asian Aust J Anim Sci. (2018) 31:1230–7. doi: 10.5713/ajas.17.0885
11. Saeed OA, Sazili AQ, Akit H, Alimon AR, Samsudin AA. Effects of corn supplementation into PKC-urea treated rice straw basal diet on hematological, biochemical indices and serum mineral level in Lambs. Animals. (2019) 9:2–13. doi: 10.3390/ani9100781
12. Silva WP, Santos SA, Cirne LGA, dos Santos Pina D, Alba HDR, de Carvalho Rodrigues TCG, et al. Nutrient intake, digestibility, feeding behavior, nitrogen balance, and performance of feedlot goat kids fed high-concentrate diets containing licury cake (Syagrus coronata). Trop Anim Health Prod. (2022) 54:1–10. doi: 10.1007/s11250-022-03131-9
13. AOAC. Official Methods of Analysis. 18th ed. Gaithersburgs, MD: Association of Official Analytical Chemists (2006).
14. Van Soest PV, Robertson JB, Lewis BA. Methods for dietary fiber, neutral detergent fiber, and non-starch polysaccharides in relation to animal nutrition. J Dairy Sci. (1991) 74:3583–97.
15. Zimmerman PR. System for measuring metabolic gas emissions from animals. In: U.S. Patent No. 5,265,618. Washington, DC: U.S. Patent and Trademark Office (1993).
16. Johnson K, Huyler M, Westberg H, Lamb B, Zimmerman P. Measurement of methane emissions from ruminant livestock using a SF6 tracer technique. Environ Sci Technol. (1994) 28:359–62.
17. Westberg HH, Johnson KA, Cossalman MW, Michal JJ. A SF6 Tracer Technique: Methane Measurement from Ruminants. Pullman: Washington State University (1998), 40.
18. Johnson TR, Combs DK. Effects of prepartum diet, inert rumen bulk, and dietary polythyleneglicol on dry matter intake of lactating dairy cows. J Dairy Sci. (1991) 74:933–44.
19. Statistical Analysis System (SAS). Statistical Analysis System. Version 9.1. Cary: SAS Institute (2009).
20. National Research Council. Nutrient Requirements of Dairy Cattle: 2001. Washington, DC: National Academies Press (2001).
21. Lynch JP, Jin L, Lara EC, Baah J, Beauchemin KA. The effect of exogenous fibrolytic enzymes and a ferulic acid esterase-producing inoculant on the fibre degradability, chemical composition and conservation characteristics of alfalfa silage. Animal Feed Sci Technol. (2014) 193:21–31. doi: 10.1016/j.anifeedsci.2014.03.013
22. da Silva LO, de Carvalho GGP, Tosto MSL, Lima VGO, Cirne LGA, dos Santos Pina D, et al. Digestibility, nitrogen metabolism, ingestive behavior and performance of feedlot goats fed high-concentrate diets with palm kernel cake. Livestock Sci. (2020) 241:104226. doi: 10.1016/j.livsci.2020.104226
23. Jung HG, Allen MS. Characteristics of plant cell walls affecting intake and digestibility of forages by ruminants. J Anim Sci. (1995) 73:2774–90.
24. Carrazco AV, Peterson CB, Zhao Y, Pan Y, McGlone JJ, DePeters EJ, et al. The impact of essential oil feed supplementation on enteric gas emissions and production parameters from dairy cattle. Sustainability. (2020) 12:10347. doi: 10.3390/su122410347
25. Dey A, Paul SS, Lailer PC, Dahiya SS. Reducing enteric methane production from buffalo (Bubalus bubalis) by garlic oil supplementation in in vitro rumen fermentation system. SN Appl Sci. (2021) 3:1–7. doi: 10.1007/s42452-021-04264-6
26. Bharanidharan R, Thirugnanasambantham K, Ibidhi R, Baik M, Kim TH, Lee Y, et al. Perfil Metabólico, Redução de Metano Ruminal e Potencial Modulador do Microbioma de Sementes de Pharbitis nil. Front Microbiol. (2022) 13:9125194. doi: 10.3389/fmicb.2022.892605
27. Alemu AW, Romero-Pérez A, Araujo RC, Beauchemin KA. Effect of encapsulated nitrate and microencapsulated blend of essential oils on growth performance and methane emissions from beef steers fed backgrounding diets. Animals. (2019) 9:21. doi: 10.3390/ani9010021
28. Honan M, Feng X, Tricarico JM, Kebreab E. Feed additives as a strategic approach to reduce enteric methane production in cattle: modes of action, effectiveness and safety. Animal Prod Sci. (2021) 71:295. doi: 10.1071/AN20295
29. de Araújo TLDR, da Silva WL, Berça AS, Cardoso ADS, Barbero RP, Romanzini EP, et al. Effects of replacing cottonseed meal with corn dried distillers' grain on ruminal parameters, performance, and enteric methane emissions in young nellore bulls reared in tropical pastures. Animals. (2021) 11:2959. doi: 10.3390/ani11102959
30. Rasmussen J, Harrison A. The benefits of supplementary fat in feed rations for ruminants with particular focus on reducing levels of methane production. Int Scholarly Res Not. (2011) 2011:10. doi: 10.5402/2011/613172
31. Yanza YR, Szumacher-Strabel M, Jayanegara A, Kasenta AM, Gao M, Huang H, et al. The effects of dietary medium-chain fatty acids on ruminal methanogenesis and fermentation in vitro and in vivo: a meta-analysis. J Anim Physiol Anim Nutr. (2021) 105:874–89. doi: 10.1111/jpn.13367
32. Flores-Santiago EDJ, Arceo-Castillo JI, Vaquera-Huerta H, Aguilar-Pérez CF, Cadena-Villegas S, González-Garduño R, et al. Reduction of enteric methane production with palm oil: responses in dry matter intake, rumen fermentation and apparent digestibility in sheep. Anim Feed Sci Technol. (2022) 291:15396. doi: 10.1016/j.anifeedsci.2022.115396
33. IPCC. Intergovernmental Panel on Climate Change, IPCC Guidelines for National Greenhouse Gas Inventories, Agriculture, Forestry and Other Land Use. Hayama: IPCC (2006). p. 1–10.
34. Almeida AK, Hegarty RS, Cowie A. Meta-analysis quantifying the potential of dietary additives and rumen modifiers for methane mitigation in ruminant production systems. Anim Nutr. (2021) 7:1219–30. doi: 10.1016/j.aninu.2021.09.005
35. Andrew Selaledi L, Mohammed Hassan Z, Manyelo TG, Mabelebele M. The current status of the alternative use to antibiotics in poultry production: an African perspective. Antibiotics. (2020) 9:594. doi: 10.3390/antibiotics9090594
36. Omar JA. Effect of different ionophore treatments on some rumen metabolic measures of steers. Dirasat Agric Sci. (2004) 31:178–84. Available online at: https://staff-beta.najah.edu/media/sites/default/files/Effect_of_Different_Ionophore_Treatments_on_Some_Rumen_Metabolic_Measures_of_Steers.pdf
37. Broucek J. Options to methane production abatement in ruminants: a review. J Anim Plant Sci. (2018) 28:348–64. Available online at: http://www.vuzv.sk/pdf/broucek/Met%C3%B3dy%20zni%C5%BEovania%20produkcie%20met%C3%A1nu%20u%20pre%C5%BE%C3%BAvavcov%20(%C3%BApravy%20prostredia%20bachoru,%20bioch%C3%A9mia).pdf
38. Thomas DT, Beletse YG, Dominik S, Lehnert SA. Net protein contribution and enteric methane production of pasture and grain-finished beef cattle supply chains. Animal. (2021) 15:00392. doi: 10.1016/j.animal.2021.100392
39. Ryan CV, Pabiou T, Purfield DC, Conroy S, Kirwan SF, Crowley JJ, et al. Phenotypic relationship and repeatability of methane emissions and performance traits in beef cattle using a GreenFeed system. J Anim Sci. (2022) skac349. doi: 10.1093/jas/skac349
40. Haque MN. Dietary manipulation: a sustainable way to mitigate methane emissions from ruminants. J Anim Sci Technol. (2018) 60:1–10. doi: 10.1186/s40781-018-0175-7
41. Maciel ICDF, Barbosa FA, Tomich TR, Ribeiro LGP, Alvarenga RC, Lopes LS, et al. Could the breed composition improve performance and change the enteric methane emissions from beef cattle in a tropical intensive production system? PLoS ONE. (2019) 14:e0220247. doi: 10.1371/journal.pone.0220247
42. Moot DJ, Davison R. Changes in New Zealand red meat production over the past 30 year. Anim Front. (2021) 11:26–31. doi: 10.1093/af/vfab027
43. Polli VA, Restle J, Senna DB, Almeida SRS. Aspectos relativos à ruminação de bovinos e bubalinos em regime de confinamento. Rev Brasil Zoot. (1996) 25:987–93.
Keywords: by-products, greenhouse gases, methane, nutrition, ruminants
Citation: Amaral Júnior JMd, Martorano LG, Nahúm BdS, de Castro VCG, Sousa LF, Rodrigues TCGdC, da Silva JAR, da Costa Silva AL, Lourenço Júnior JdB, Berndt A and e Silva AGM (2022) Feed intake, emission of enteric methane and estimates, feed efficiency, and ingestive behavior in buffaloes supplemented with palm kernel cake in the Amazon biome. Front. Vet. Sci. 9:1053005. doi: 10.3389/fvets.2022.1053005
Received: 24 September 2022; Accepted: 23 November 2022;
Published: 21 December 2022.
Edited by:
Melissa Duplessis, Agriculture and Agri-Food Canada (AAFC), CanadaReviewed by:
Anusorn Cherdthong, Khon Kaen University, ThailandFrancesco Serra, University of Naples Federico II, Italy
Copyright © 2022 Amaral Júnior, Martorano, Nahúm, de Castro, Sousa, Rodrigues, da Silva, da Costa Silva, Lourenço Júnior, Berndt and e Silva. This is an open-access article distributed under the terms of the Creative Commons Attribution License (CC BY). The use, distribution or reproduction in other forums is permitted, provided the original author(s) and the copyright owner(s) are credited and that the original publication in this journal is cited, in accordance with accepted academic practice. No use, distribution or reproduction is permitted which does not comply with these terms.
*Correspondence: André Guimarães Maciele e Silva, YW5kcmVnbXNAdWZwYS5icg==