- 1Zoology Department, Faculty of Science, South Valley University, Qena, Egypt
- 2Electronic and Nano Devise Lab, Faculty of Science, South Valley University, Qena, Egypt
- 3General Authority for Veterinary Services, Faculty of Veterinary Medicine, South Valley University, Qena, Egypt
- 4Department of Surgery, Anesthesiology, and Radiology, Faculty of Veterinary Medicine, Aswan University, Aswan, Egypt
- 5Department of Nutrition and Animal Husbandry, University of Veterinary Medicine and Pharmacy in Košice, Košice, Slovakia
- 6Department of Biology and Physiology, University of Veterinary Medicine and Pharmacy in Košice, Košice, Slovakia
- 7Department of Husbandry and Development of Animal Wealth, Faculty of Veterinary Medicine, Menoufia University, Shebin Alkom, Egypt
- 8Department of Pathobiochemistry, Faculty of Pharmacy, Meijo University, Nagoya-shi, Japan
The application of metallic nanoparticles poses risks to human and animal health. Titanium dioxide nanoparticles (TiO2NPs) are the most commonly synthesized metallic oxides in the world. Exposure to TiO2NPs can cause toxicity in the target organisms. This study aimed to evaluate the effects of green and chemical TiO2NPs on maternal and embryo-fetal livers. Green TiO2NPs using garlic extract (GTiO2NPs) and chemical TiO2NPs (CHTiO2NPs) were synthesized and characterized by x-ray powder diffraction and high-resolution transmission electron microscopy. The cytotoxicity of both chemical and green TiO2NPs was determined against HepG2 cell lines. Fifty pregnant female Albino rats were equally and randomly divided into five groups. Group 1 was kept as a control. Groups 2 and 3 were orally treated with 100 and 300 mg/kg body weight of CHTiO2NPs, respectively. Groups 4 and 5 were orally treated with 100 and 300 mg/kg of GTiO2NPs, respectively, from day 6 to 19 of gestation. All dams were euthanized on gestation day 20. All live fetuses were weighed and euthanized. Blood and tissue samples were collected for biochemical, histopathological, and Bax-immunohistochemical expression analyses. Our results indicated that garlic could be used as a reducing agent for the synthesis of TiO2NPs, and the produced NPs have no toxic effect against HepG2 cells compared with CHTiO2NPs. The maternal and fetal bodyweights were greatly reduced among the chemically TiO2NPs induced animals. The mean serum level of AST and ALT activities and the total protein level significantly increased when TiO2NPs were administered at high doses. Histologically, the CHTiO2NPs-treated groups revealed vacuolated and necrotized hepatocytes with congested and dilated blood vessels in the fetal and maternal livers. The immunohistochemistry revealed distinct positive staining of Bax expressed in the hepatocytes. Nevertheless, the biosynthesis of TiO2NPs using garlic extract had a minimal effect on the normal architecture of the liver. It could be concluded that the bioactivity of TiO2NPs can be modified by green synthesis using garlic extract. Compared to the CHTiO2NPs, the exposure to GTiO2NPs showed reduced liver damage in maternal and embryo-fetal rats.
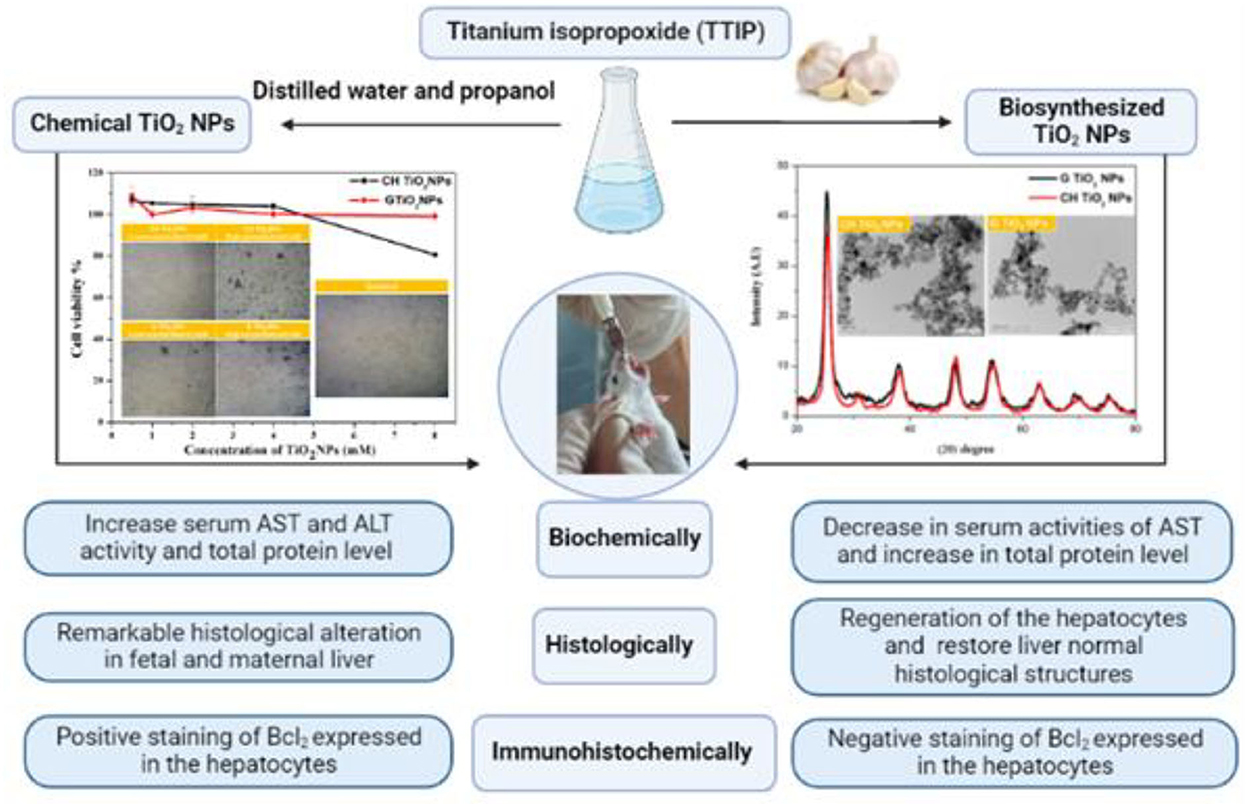
Graphical Abstract. The schematic cartoon of the experimental design – It was created by BioRender.com.
Introduction
There has been a huge increase in the application of nanoparticles in recent years. The development of nanotechnology offers practical benefits in a variety of industries, including the textile, cosmetics, energy, and chemical sectors (1), when compared with larger-scale particles with the same chemical composition (2). Due to their ease of preparation and low cost of production, titanium dioxide nanoparticles (TiO2NPs), among the most well-known metallic oxide NPs, have raised significant concerns (3). Currently, different industrial sectors are using TiO2NPs in a number of scientific fields, including the production of plastics, the use of adjuvants in the formulation of pharmaceutical pills, bleaching agents in the paper industry, and the manufacture of paints, cosmetics, sunscreens, and toothpaste (4). The same study claimed that the usage of nanoparticles as food additives is widespread (5). Ingestion, inhalation, injection into the skin, and penetration were the most common routes of exposure (6). Despite their widespread use and daily exposure, the effects of these particles on animal and human health and the environment remain unknown. Lately, The public health dangers associated with exposure to silica or metallic oxide nanoparticles were the first to suggest the adverse biological response of nano-sized materials (7, 8). We could argue that nanotechnology has become a double-edged sword.
While it has the potential to enhance people's quality of life, it also has some downsides: as environmental contamination increases, it becomes hazardous and poses a serious threat to living organisms. Chemically, NPs are more reactive than heavy metals because of their own physicochemical characteristics (9). In toxicological profiles due to metallic nanoparticle (MNP) exposure, the deteriorations in the levels of the specific enzyme directly reflect the damage in specific organs (6, 10). TiO2NPs were determined to induce hepatocyte apoptosis and increase the inflammatory response (11). An increase in the expression of the genes for reactive oxygen species (ROS) and cytochrome p450 is thought to be the cause of liver damage (CYP1A). Following this process, activated hepatic macrophages produce inflammatory mediators, resulting in lipid peroxidation and protein oxidation, which cause hepatocellular necrosis (12).
Green synthetic nanoparticles have been offered as new avenues for nanotechnology advancement because they are safer and more environmentally friendly. Biological agents such as bacteria, plant extracts, and fungi are increasingly being used in green synthesis instead of serious chemical agents (13, 14) and algae (15). Cost-effectiveness and a one-step process are advantages of green synthesis (16). Various extracts from plant origins have previously been used in green synthetic nanoparticles due to their easy preparation (17). Scientific reviewers suggest that plant-based extracts may naturally possess hepatoprotective properties. Garlic (Allium sativum) can provide a histo-protective role against organ damage caused by NPs, particularly liver damage (18). Some constituents isolated from garlic, such as steroids, terpenoids, polyphenols, flavonoids, and other phenolic chemicals, have been demonstrated to shield the liver against harmful substances (19).
As a consequence, a diet high in flavonoids can lower the risk of tissue degradation and change caused by specific disorders (20). The possibility of fetal malformations and deaths is dramatically increased by exposure to TiO2NPs. However, research conducted on pregnant mice to examine the possibility of TiO2NPs toxicity on placental growth and development is scant (21). Therefore, this study is a trial to spot the harmful effect of CHTiO2NPs on the liver of the maternal rats and their embryos and how GTiO2NPs could alleviate hepatotoxicity.
Materials and methods
Chemical synthesis of TiO2NPs
According to the previous report (22), the preparation of CHTiO2NPs was performed using the chemical coprecipitation method. Titanium isopropoxide (TTIP) was purchased from Sigma Co., USA; it has been used as a precursor for TiO2, while a mixture of distilled water and propanol was used as a solvent with a 50/1 (V/V). To obtain the solvent solution, 250 ml of water and 5 ml of propanol were combined, and to produce the precursor solution 15 ml of propanol and 5 ml of TTIP were combined. After being heated at 70°-90°C under constant stirring for 2 h, the solvent solution was modified by adding the precursor solution drop by drop. A white precipitate was observed immediately, which indicated the reduction of TiO2. After leaving it to cool overnight at 25°C, this precipitate was washed three times with distilled water and one time with ethanol. The prepared sample was washed, dried for 12 h at 100°C, and then calcinated for 3 h at 400°C.
Green synthesis of TiO2NPs
Garlic cloves were purchased from the local market belonging to Qena City, Qena, Egypt. To make GTiO2NPs, 20 g of dried, finely ground garlic was boiled in 150 ml of distilled water for 1 h. The TiO2 precursor solution was made by vigorously stirring 150 ml of distilled water with 10 ml of TTIP. Then, 60 ml of fresh garlic plant extract was addedto the TiO2 precursor solution with continuous stirring for 2 h. The solution's color changed from white to dark yellow, which signaled the mitigation of TTIP and the formation of GTiO2NPs. The created NPs were dried at 100°C and cooled at 25°C overnight. Finally, the sample was finely crushed into powder using an agate mortar and pestle and then calcinated at 450°C for 2 h (23).
TiO2NPs characterization
The prepared TiO2NPs were characterized by x-ray diffraction (XRD) following X'Pert PRO-PAN analytical diffractometer with Cu-Kα radiation (λ = 1.54056A°), which was produced at 40 kV and 30 mA. Using high-resolution transmission electron microscopy (HRTEM), the samples' morphology was discovered (JEOL, JEM 2100, Japan).
Cytotoxicity of TiO2NPs
Liver hepatocellular cells (HepG2 cell lines) were employed to assess the toxicity of TiO2NPs following an MTT assay. HepG2 cell lines were purchased from Vacsera (Giza, Egypt). In DMEM with 10% heat-inactivated fetal bovine serum and 1% penicillin-streptomycin, HepG2 cells were grown.
A total of 1 × 104 HepG2 cells were seeded into 96-well culture plates, incubating at 37°C and 5% CO2. By dissolving “3-(4,5-Dimethyl-2-thiazolyl)-2,5-diphenyl-2H-tetrazolium bromide” in PBS at a concentration of 5 mg/mL and mixing the solution while stirring for 1 h, the MTT 3-(4,5-dimethylthiazol-2-yl)-2, 5-diphenyltetrazolium reagent was created. The solution was then filtered, serialized, and stored in the dark at 4°C. The MTT assay was performed according to the standardized protocol (11). The developed cells were briefly rinsed with PBS, exposed to TiO2NPs at various concentrations (0, 0.5, 1, 2, 4, and 8 mM), and then incubated for 24 h at 37°C and 5% CO2. The exposed cells were rinsed in PBS the following day, subjected to an MTT solution (80 mL of serum-free medium and 20 mL of MTT), and then incubated for 3 h at 37°C and 5% CO2. We poured MTT solvent (DMSO) into each well to stop the reaction. Plates were then wrapped in foil and shaken on an orbital shaker for 15 min. Finally, the absorbance was recorded at OD = 590 nm using a Tecan infinite F50 absorbance microplate reader. The experiment was carried out three times, and the outcomes are the three replicates' averages with standard deviations normalized to the control. The percent of cell viability was calculated using the following equation (11):
Experimental animals and management
Fifty adult female Sprague–Dawley Albino rats of 5–6 months' age and 180–220 g average body weight were purchased from the animal house of the National Research Center Institute (Cairo, Egypt). The rats were housed in clean, well-ventilated cages under appropriate temperature and humidity laboratory conditions. Throughout the experiment, all rats had access to conventional food and unlimited access to water. Before the experiment, they had an acclimation period of 2 weeks.
Experimental design and treatment
For developmental toxicity studies, every three female rats mated with one male rat in a stainless-steel cage at night. The vaginal smear determined the first day of gestation. On day 6 of gestation, pregnant female rats were immediately introduced into the experimental design.
In this experiment, 50 pregnant female Albino rats were randomly assigned to five groups (n = 10 each):
• Group 1 was utilized as control and supplied with distilled water.
• Group 2 (CH100) orally received 100 mg/kg bwt of CHTiO2NPs.
• Group 3 (CH300) orally received 300 mg/kg bwt of CHTiO2NPs.
• Group 4 (G100) orally received 100 mg/kg bwt of GTiO2NPs.
• Group 5 (G300) orally received 300 mg/kg bwt of GTiO2NPs.
All animals were orally administered fresh suspensions of TiO2NPs by intragastric gavage daily from 6 to 19 days of gestation.
During the experiments, all pregnant females were carefully inspected daily during the gestation period for any clinical signs or abnormalities. Moreover, the maternal body weight was measured on days 6, 9, 12, 15, 17, and 20 of gestation. The body weight of fetuses was also recorded.
Blood samples
On day 20 of gestation, all female rats were euthanized after they were made to inhale a dose of diethyl ether. Using the retro-orbital technique, whole blood samples were drawn, and the serum was obtained in clean, dry tubes, centrifuged at 3,000 rpm for 10 min, and then frozen at −20°C until further biochemical analysis.
Liver biopsy
The livers of mothers and fetuses were excised and preserved in 10% buffered neutral formalin for histopathological and immunohistochemical studies.
Biochemical analysis
Estimation of serum transaminases (IU/l)
Serum transaminase activities (AST and ALT) were estimated with biodiagnostic enzymatic kits purchased from Biodiagnostic (Dokki, Giza, Egypt) using the colorimetric method, as described previously by Sanghavin and Jivani (24).
Estimation of protein profile (g/dl)
Total protein and albumin test kits from Biodiagnostic (Dokki, Giza, Egypt) were used to measure the serum total protein and albumin based on the protocols that have been outlined (25, 26).
Histopathological examination
Systemic autopsies from the livers of each group were dissected and thoroughly immersed in 10% buffered neutral formalin (pH 7.2) for 48 h. Paraffin sections of 4 μm in thickness were stained with hematoxylin and eosin (H&E) stain and inspected under the light microscope (27).
Immunohistochemical reaction
According to the previous report, the cryostat sections of the liver from all groups were prepared at 4 μm thickness, deparaffinized, and then stained with an immunohistochemical reaction for Bax (28).
Statistical analysis
The results were statistically expressed as mean ± standard deviation (SD) by a one-way ANOVA, followed by a post-hoc Tukey's test for multiple comparisons between different groups. The level of significance was set at a p-value of < 0.05 (29).
Results
Characterization of TiO2NPs
The -ray diffraction (XRD) pattern of chemically made and environmentally friendly TiO2NPs is shown in Figure 1. All the characteristic peaks of TiO2NPs were present in the XRD of the two samples, which indicated the successful formation of TiO2NPs. The diffraction peaks at 2θ (25.27, 30.9, 37.79, 48, 55.6, 63.3, 70.5, and 74.2) corresponded to the plane (101, 004, 200, 105, 204, and 220, respectively). HRTEM images of TiO2NPs showed a sort of agglomeration with a spherical or irregular spherical shape in both samples.
Cytotoxicity of TiO2NPs
The toxicity of TiO2NPs against HepG2 cells was determined using the MTT assay. Both samples did not exhibit toxicity at low concentrations (0.5–4 mM). However, at a high concentration (8 mM), CHTiO2NPs showed a decrease in cell viability (from 104 to 80 %). Moreover, an accumulation of TiO2NPs on the surface of the cells was observed at a high concentration, which suggested that the number of NPs internalized in the cells was smaller than the number of adherent NPs, as presented in Figure 2.
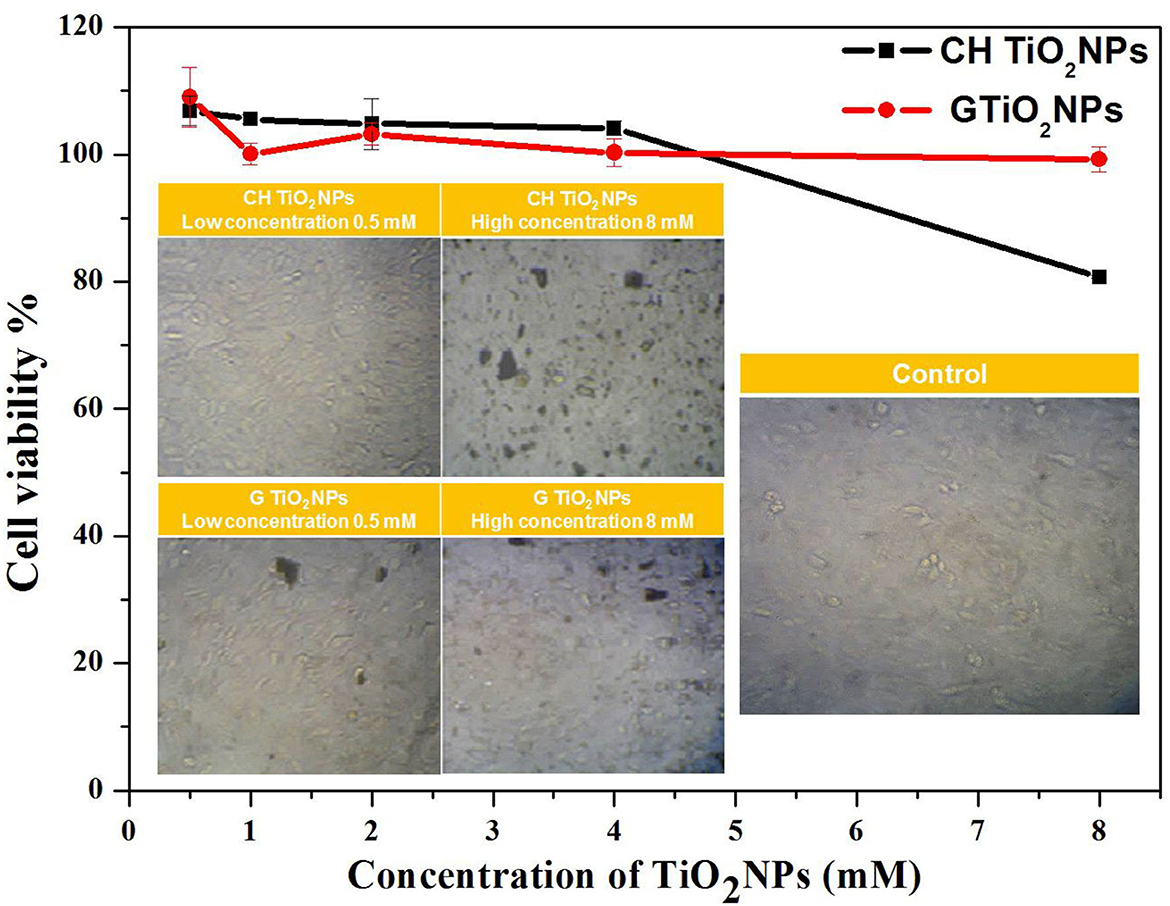
Figure 2. Cell viability of HepG2 cells after 24 h exposure to CHTiO2NPs and GTiO2NPs with different concentrations and microscopic images of HepG2 cells after 24 hrs of exposure to CHTiO2 NPs and GTiO2NPs at concentration 0.5 mM and 8 mM.
Body weight
Maternal body weight
CHTiO2NPs 100 and CHTiO2NPs 300 significantly decreased maternal body weight from 6 to 20 days of gestation compared to the control group, while GTiO2NPs 100 significantly decreased maternal body weight from 6 to 17 days of gestation compared to the control group. Moreover, GTiO2NPs 300 considerably decreased at 9, 17, and 20 days of gestation compared to the control group. Additionally, CHTiO2NPs 300 significantly increased at 6, 9, 17, and 20 of gestation compared with CHTiO2NPs 100. At 6–20 days of gestation, the maternal body weight was considerably higher in GTiO2NPs 100 and GTiO2NPs 300 than in CHTiO2NPs 100, and at 6–15 days of gestation, it was significantly higher in GTiO2NPs 100 and GTiO2NPs 300 than in CHTiO2NPs 300. Furthermore, the maternal body weight of the GTiO2NPs 100 treated group significantly increased compared with CHTiO2NPs 300 on the 20th day of gestation, while GTiO2NPs 300 significantly increased on the 17th day compared with CHTiO2NPs 300. Moreover, compared to GTiO2NPs 100, GTiO2NPs 300 showed a considerable increase between days 6 and 20 of gestation (Table 1).
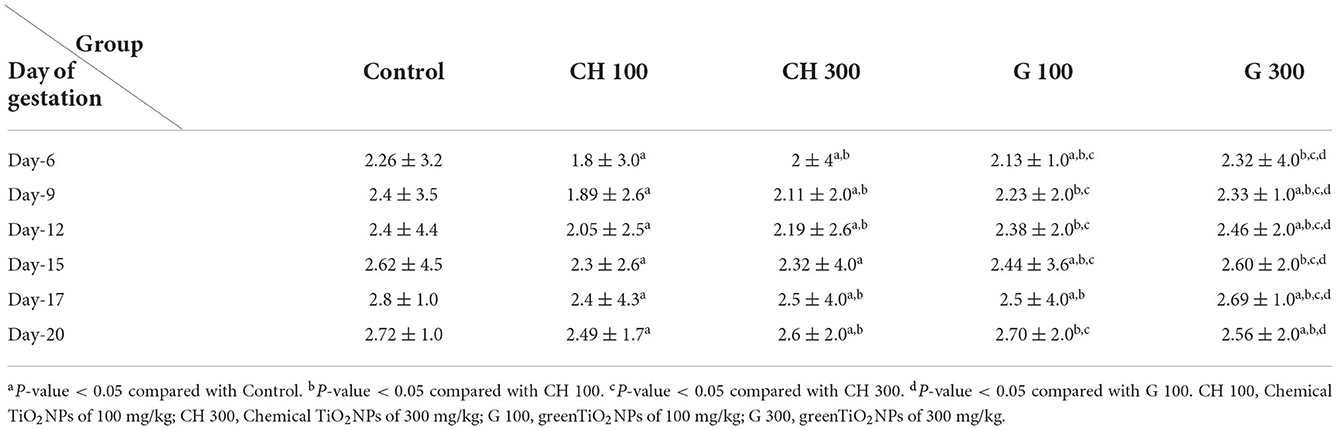
Table 1. Effect of chemical and green TiO2NPs on maternal body weight (g/kg) of control and experimental groups.
Fetal body weight
Compared with the control, fetal body weight significantly decreased with 300 mg/kg of CHTiO2NP, 100 mg/kg of CHTiO2NP, 100 mg/kg of GTiO2NP, and 300 mg/kg of GTiO2NP, as shown in Table 2. Additionally, the number of fetuses significantly decreased CHTiO2NP by 300 mg/kg compared with the control.
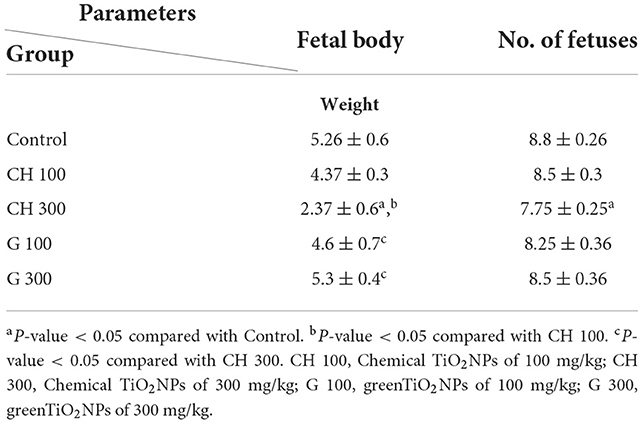
Table 2. Effect of chemical and green TiO2NPs on fetal body weight (g/kg) and the number of fetuses of control and experimental groups (mean ± SD).
Biochemical findings
The group treated with 300 mg/kg of CHTiO2NPs showed significantly (P < 0.05) increased serum AST and ALT activities compared with the control group (Table 3). In contrast, the groups treated with 100 and 300 mg/kg of GTiO2NPs showed a significant decrease (P < 0.05) in serum AST activity compared with the control group.
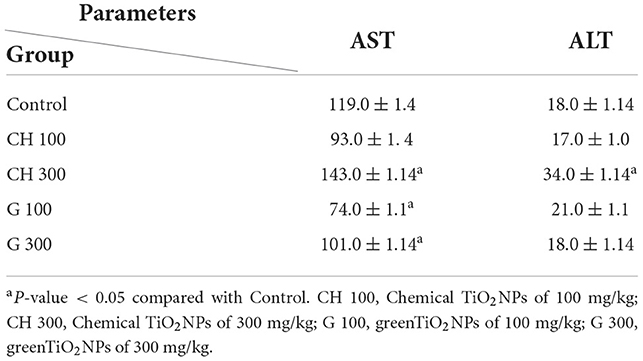
Table 3. Effect of chemical and green TiO2NPs on liver transaminases of control and experimental groups (mean ± SD).
Regarding the protein profile, total protein exhibited a significant increase (P < 0.05) in all exposed groups among the chemical and green TiO2NPs compared with the control group. Albumin levels significantly (P < 0.05) outperformed the mean values of the treated group with 300 mg/kg of CHTiO2NPs in comparison with the control group (Table 4).
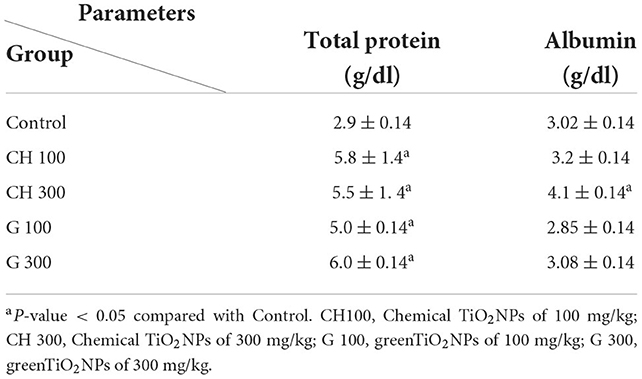
Table 4. Effect of chemical and green TiO2NPs on protein profile of control and experimental groups (mean ± SD).
Histopathological findings
Liver of rat fetuses
The fetal livers in the control group showed primitive hepatocytes with prominent infiltration of hematopoietic cells, mostly composed of red blood cells (RBCs) and lymphocytes in the blood sinusoids (Table 5, Figures 3A,B).
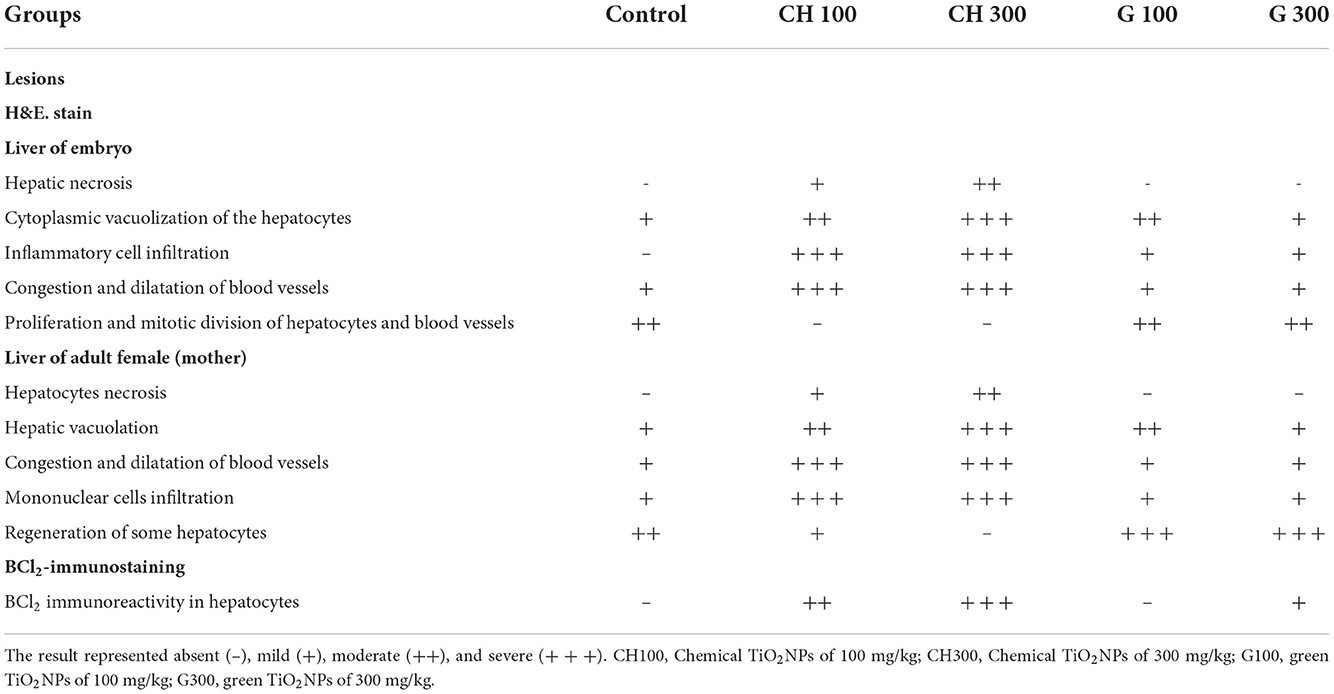
Table 5. Histopathological and immunostaining scores from the liver of the embryo and mother of control and other experimental groups.
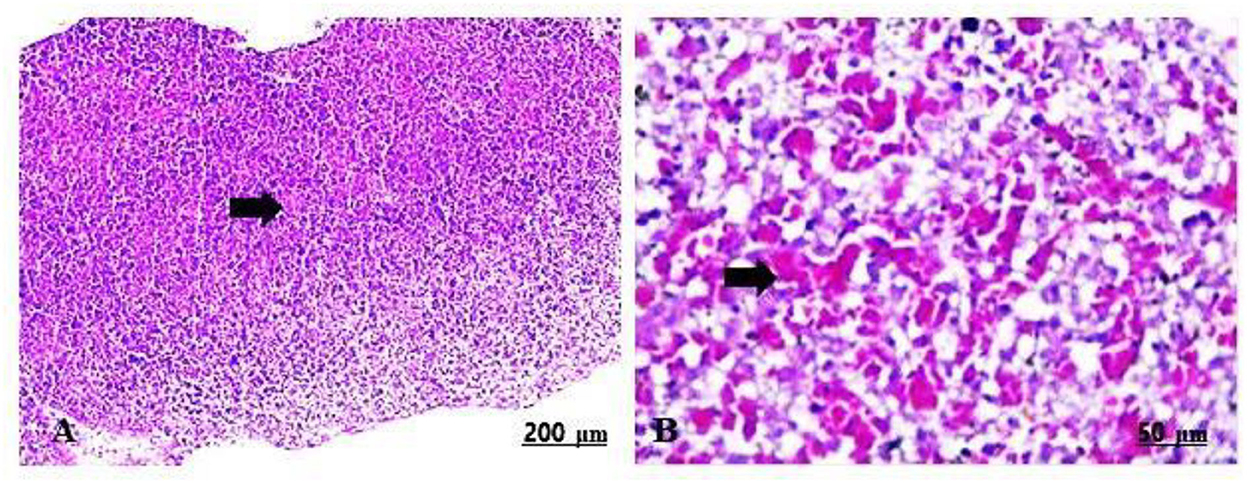
Figure 3. (A,B) Light photomicrograph of sections from the fetal liver of control stained with H&E: control showing intense infiltration of hematopoietic cells, mainly RBCs and lymphocytes in the blood sinusoids (A,B).
The fetal livers treated with 100 mg/kg of CHTiO2NPs showed extensive hepatic vacuolation, thrombotic vasculitis of the central vein, and lymphocyte infiltration (Table 5, Figures 4A,B), while those treated with 300 mg/kg of CHTiO2NPs showed remarkable congestion and dilation of the blood vessels (Table 5, Figure 4C), as well as severe necrosis and cytoplasmic vacuolization of the hepatocytes with inflammatory cell infiltration (Table 5, Figure 4D).
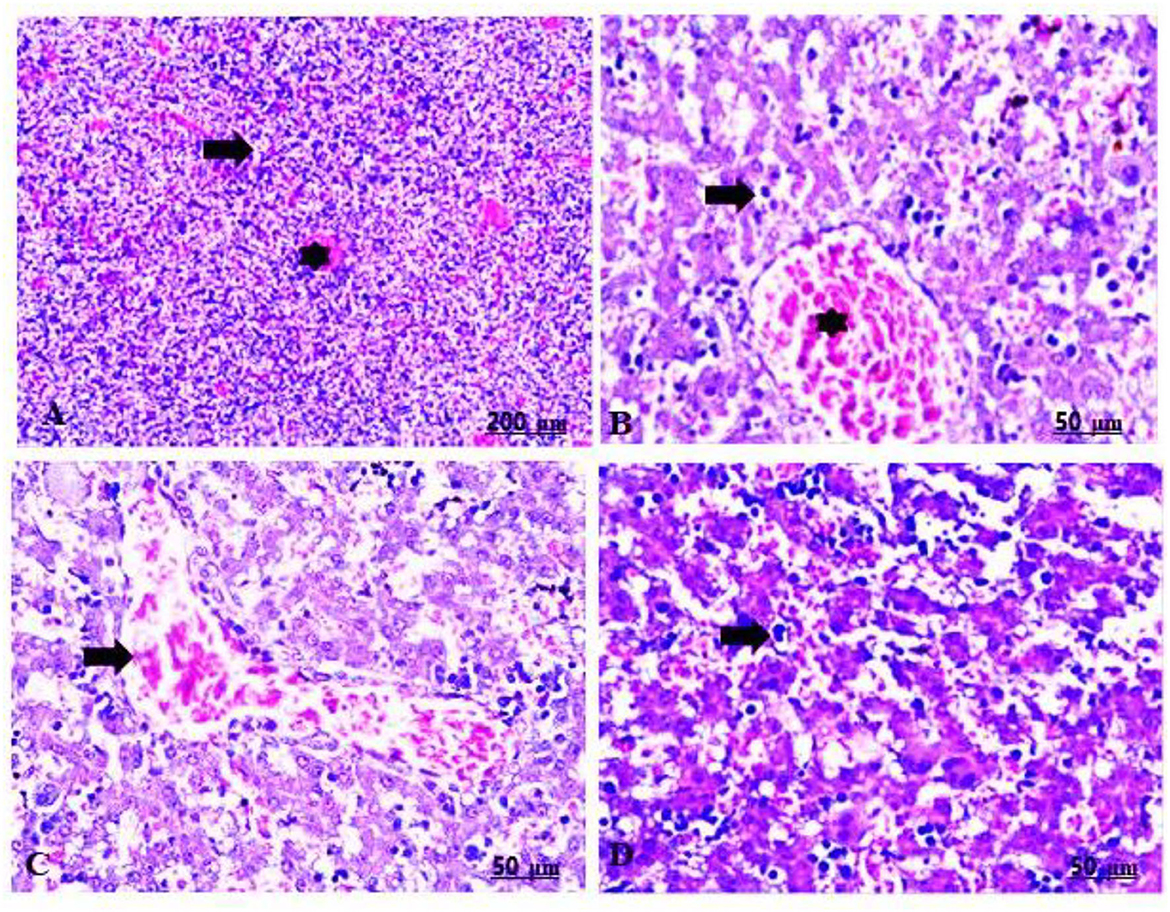
Figure 4. (A–D) Light photomicrograph of sections from the fetal liver of CHTiO2NPs exposed groups. CHTiO2NPs of 100 mg/kg bwt showing extensive hepatic vacuolation (arrow), thrombotic vasculitis of the central vein, and lymphocyte infiltration (star) (A,B). CHTiO2NPs of 300 mg/kg bwt show remarkable congestion and dilatation of the blood vessels (C), besides severe necrosis and cytoplasmic vacuolization of the hepatocytes with inflammatory cell infiltration (D).
The fetal livers of the group treated with 100 mg/kg of GTiO2NPs exhibited slight congestion and dilatation of the central vein (Table 5, Figure 5A), in addition to cytoplasmic vacuolation and inflammatory cell infiltration (Table 5, Figure 5B). However, the fetal livers that were administered 300 mg/kg of GTiO2NPs induced mitotic division of the hepatocytes in addition to congestion and dilatation of the blood vessels (Table 5, Figures 5C,D).
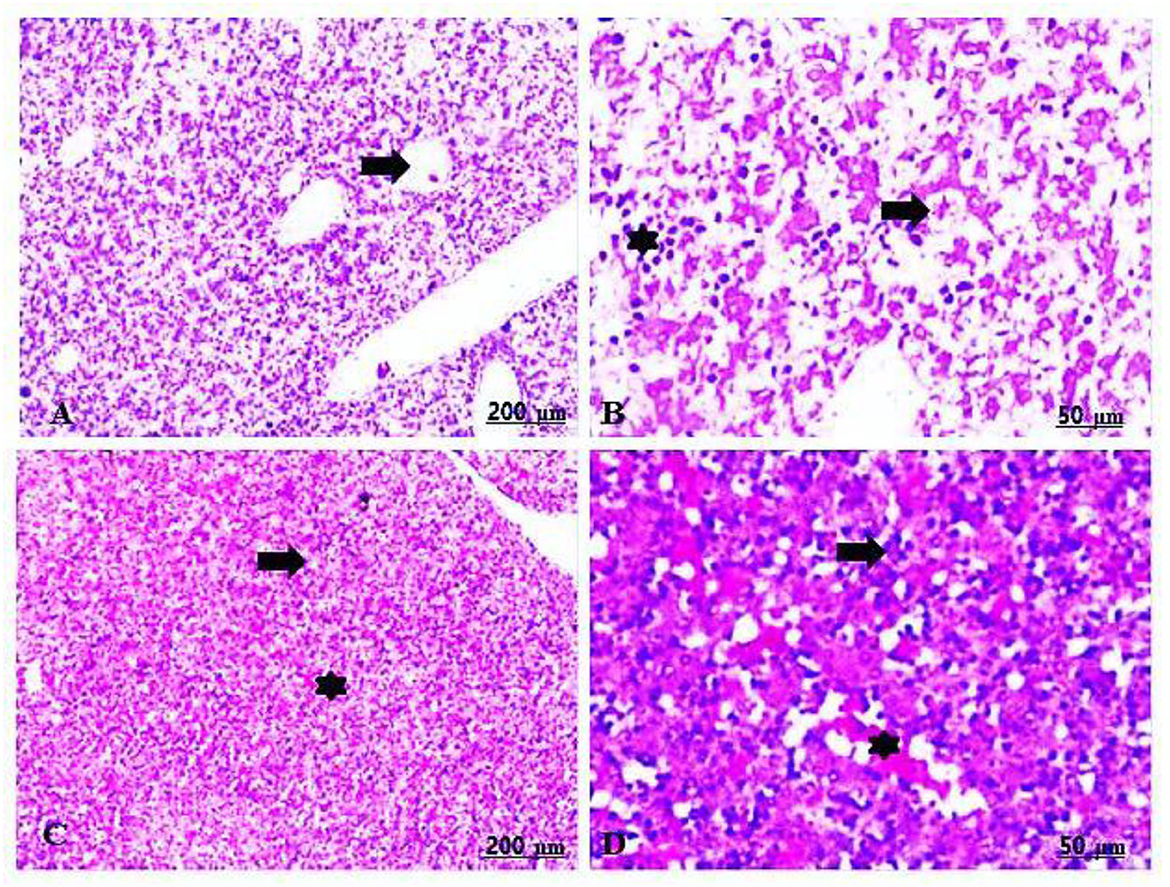
Figure 5. (A–D) Light photomicrograph of sections from the fetal liver of GTiO2NPs exposed groups. GTiO2NPs of 100 mg/kg bwt show slight congestion and dilatation of the central vein (A), in addition to cytoplasmic vacuolation (arrow) and inflammatory cell infiltration (star) (B). GTiO2NPs of 300 mg/kg bwt show proliferation of the hepatocytes with mitotic division (arrow) in addition to congestion and dilatation of the blood vessels (star) (C,D).
Liver of maternal rats
The maternal livers of the control group implied a normal arrangement of the hepatocytes and intact vasculature (Table 5, Figures 6A,B).
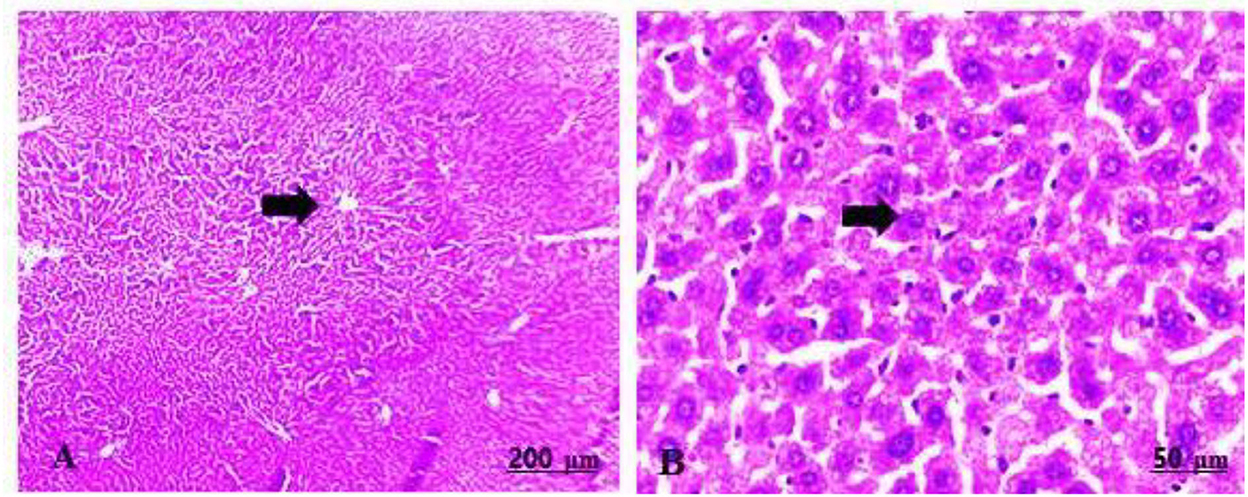
Figure 6. (A,B) Light photomicrograph sections from maternal liver of control group showing normally arranged hepatic cords and blood vessels.
The maternal livers of the group treated with 100 mg/kg of CHTiO2NPs showed sharp dilatation of the central vein with perivascular cell infiltration, mainly lymphocytes, as well as prominent vacuolation and necrosis of the hepatocytes (Table 5, Figures 7A,B), while the group treated with 300 mg/kg of CHTiO2NPs showed congestion of the blood vessels, fundamentally the central vein and blood sinusoids (Table 5, Figure 7C), besides portal fibrosis and inflammation (Table 5, Figure 7D).
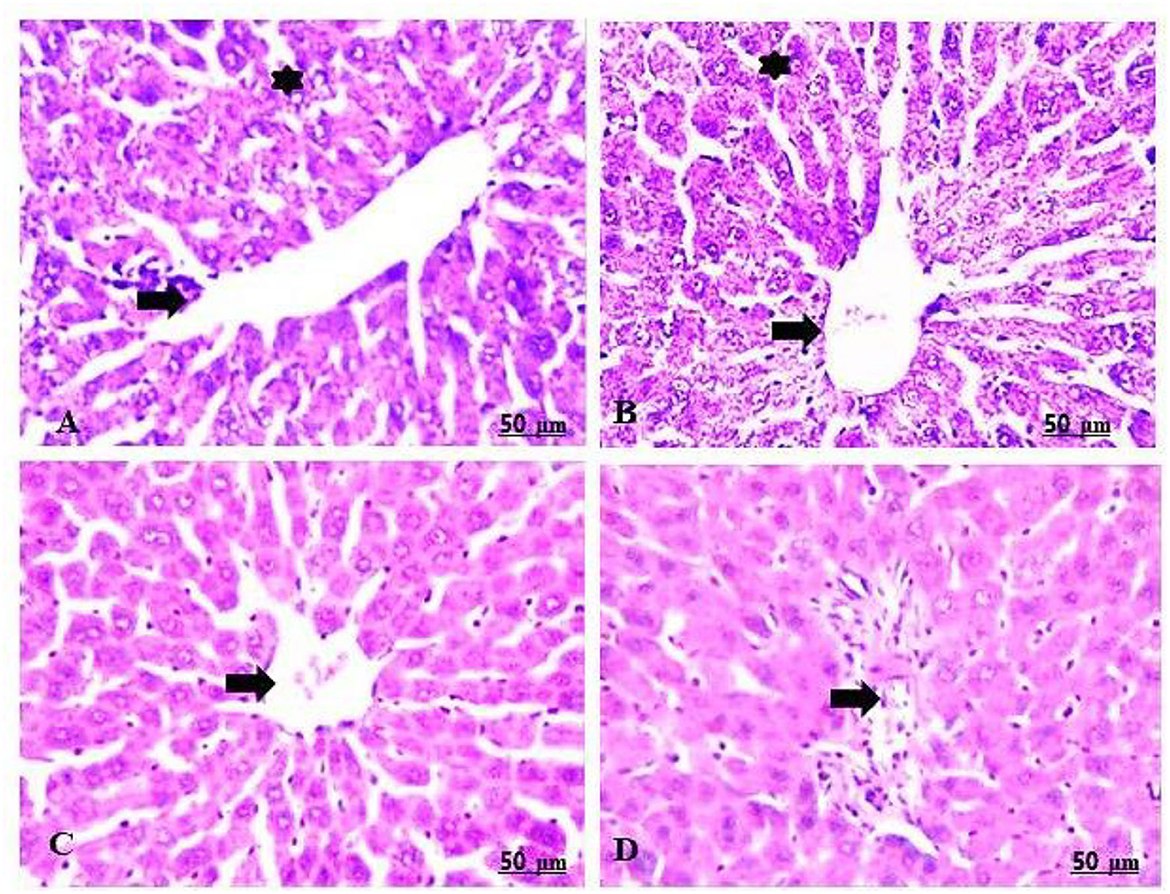
Figure 7. (A–D) Light photomicrograph of sections from the liver of CHTiO2NPs exposed groups. CHTiO2NPs of 100 mg/kg bwt show noticeable dilatation of the central vein with perivascular mononuclear infiltration, mostly lymphocytes (arrow), also prominent vacuolation and necrosis of the hepatocytes (star) (A,B). CHTiO2NPs of 300 mg/kg bwt showed congestion of the central vein and blood sinusoids (C), and portal inflammation and fibrosis were seen (D).
The maternal livers in the treated group with 100 mg/kg of GTiO2NPs manifested a mild degree of congestion and a dilated central vein, in addition to the regeneration of some hepatocytes (Table 5, Figures 8A,B). Similarly, the maternal livers in the group treated with 300 mg/kg of GTiO2NPs elucidated regeneration of the hepatocytes in trials to restore normal histological structures (Table 5, Figures 8C,D).
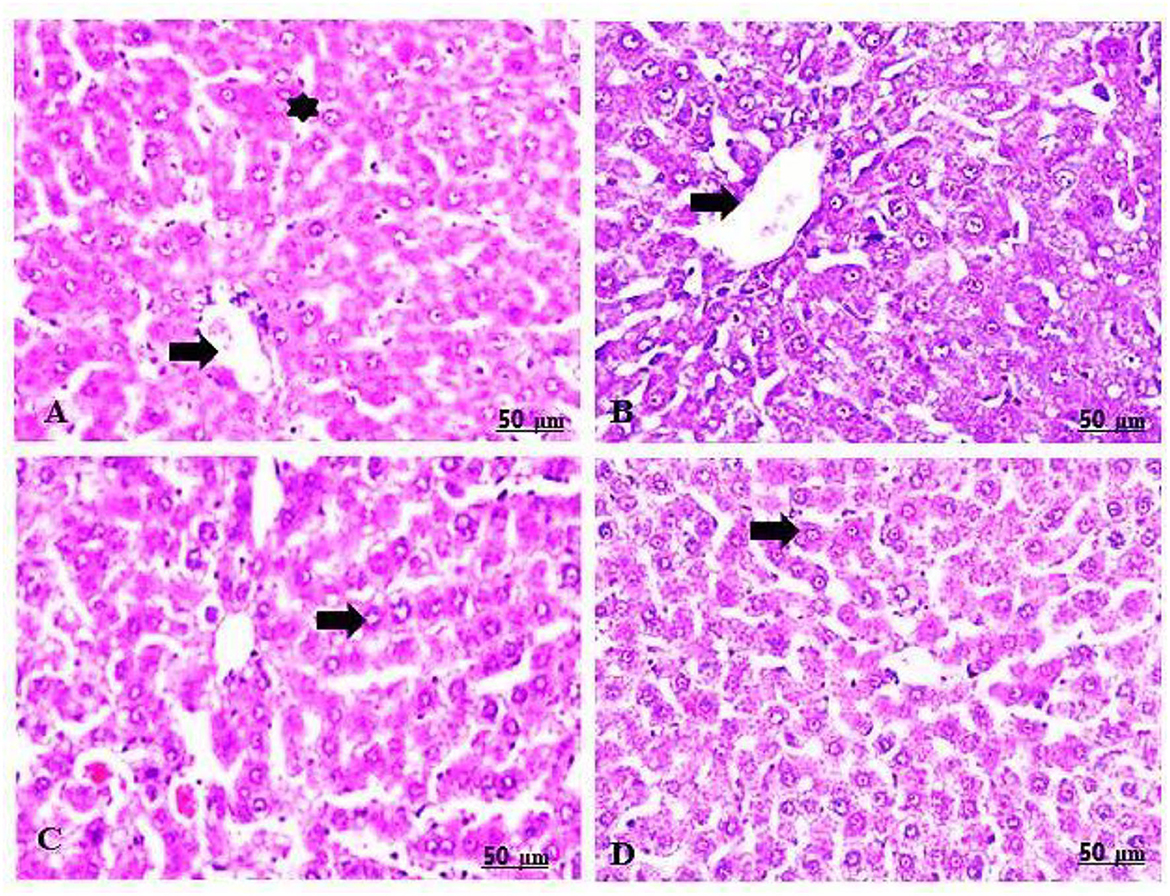
Figure 8. (A–D) Light photomicrograph of sections from the maternal liver of GTiO2NPs exposed groups. GTiO2NPs of 100 mg/kg bwt show slight congestion and dilatation of the central vein (arrow), in addition to the regeneration of some hepatocytes (star) (A,B). GTiO2NPs of 300 mg/kg bwt showing regeneration of the hepatocytes in trial to restore normal histological structures (C,D).
Immunohistochemical findings (Bax-IHC stain)
Liver of rat fetuses
The livers of the rats in the control group showed negative Bax immunoreactivity toward the hepatocytes (Table 5, Figure 9A). In contrast, livers of rats in the 100 and 300 mg/kg CHTiO2NPs treated groups expressed a sharp positive Bax immunostaining in the hepatic parenchyma (Table 5, Figures 9B,C). Meanwhile, rats that were administered 100 mg/kg of GTiO2NPs by Bax immunostaining showed an apparent negative reaction to Bax in the liver tissues (Table 5, Figure 9D). Moreover, the rats treated with 300 mg/kg of GTiO2NPs showed weak Bax immunoassays in the hepatocytes (Table 5, Figure 9E).
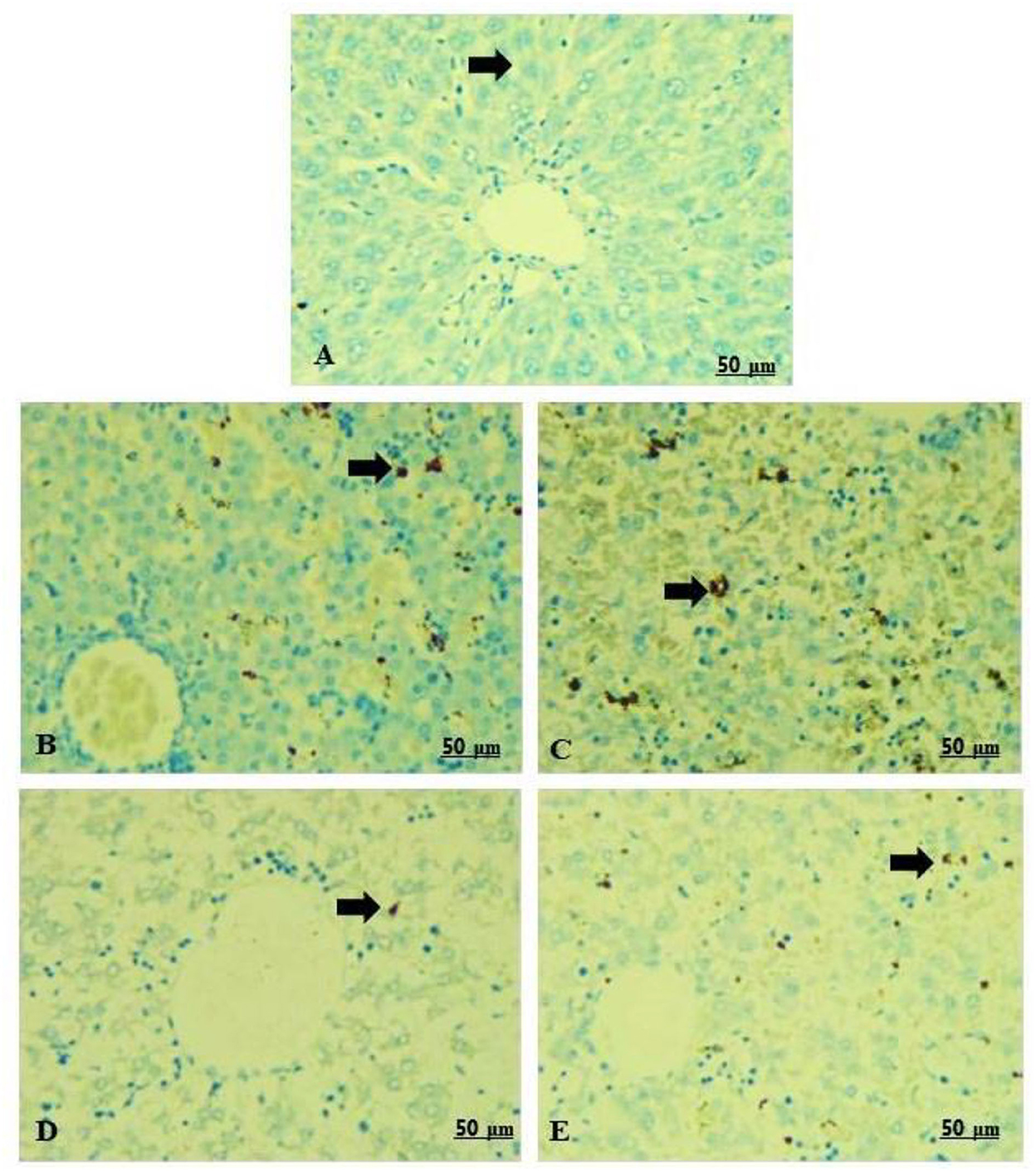
Figure 9. (A–E) Light photomicrograph of sections from the fetal liver of control and other exposed groups by Bax-IHC: control showing negative Bax-immunostaining in the hepatocytes (A). Groups 2 and 3 show intense positive staining reactions of Bax in the hepatocytes (B,C). Group 4 shows negative Bax-immunoreactivity in the hepatocytes (D). Group 5 shows a weak positive Bax-immunoreactivity in the hepatocytes (E).
Discussion
Due to its safety, environmental friendliness, simplicity of manufacturing, and low production costs, the green synthesis of nanoparticles has recently attracted much interest (13). The use of antioxidant agents such as plant extracts, natural chemicals, vitamins, and minerals in synthesizing NPs has produced positive results (16). Garlic is well known for its prevalent anti-inflammatory, antioxidant, antibacterial, antiviral, antifungal, and antimutagenic properties (30). Garlic can also offer medicinal and favorable effects such as reducing risk factors for cardiovascular diseases, enhancing the detoxificationprocess, and providing hepatoprotection (31, 32). Its properties have a significant impact on the prevention of many tumors and oral disorders. Garlic pretreatment reduces oxidation, blood glucose, and lipid levels in the liver and the kidney (33). It can actively lower free radical (ROS) levels, enhancing the antioxidant system and defending the body against DNA damage (34).
TiO2NPs are one of the most widely used and highly manufactured nanoparticles (35). They are commonly used in our daily lives, for example, in feed additives, the diagnosis of diseases, water treatment, implants, and clothing (36). Unfortunately, most chemically synthesized nanoparticles can cause eco-toxicological problems. As a result, there has been a rise in scientists' interest in the potential effects of nanoparticles on human health and the environment (37). The greenly synthesized nanoparticles have recently been enriched to synthesize several plant extracts (38). The reason for this was to intensify the light on the potential of greenly synthesized TiO2NPs using garlic extract to ameliorate chemical TiO2NPs induced genotoxicity and hepatotoxicity in female Albino rats.
In this study, garlic extract was successfully used to synthesize TiO2NPs. The synthesis of TiO2NPs was verified by XRD and HRTEM techniques. The two samples' XRD patterns showed all of the distinctive peaks of the TiO2NPs. Furthermore, the lack of diffraction peaks for impurities such as NaCl and Na2TiO3, which represent the purity and polycrystalline nature of the synthesized samples, was further evidence of the absence of impurities in the two samples' XRD patterns. The reported card supports all recorded peaks from the anatase phase of TiO2NPs (JCPDS No. 21-1272), supported by the reported card (39). Garlic extract was used to completely reduce titanium isopropoxide, as evidenced by the production of the anatase phase (40). The higher crystallinity of GTiO2 NPs than the CHTiO2NPs sample is indicated by the increase in 101-plan intensity. Garlic did not alter the structural characteristics of TiO2 because there were no structural variations between the two samples. These results align well with those of sustainable TiO2 nanoparticles (17). The crystal size of the produced samples was established by Scherer using the Debye Equation (41):
where d is the average NPs crystal size, λ is the x-ray source wavelength, a constant crystal shape factor of 0.89, θ is Bragg's diffraction angle, and full width at half maximum (FWHM) of angular XRD peaks was recorded at diffraction angle 2θ. The difference between the estimated crystal sizes of CHTiO2NPs and GTiO2NPs, which was increased to 53.31 nm from 48.11 nm, may be due to the electrostatic attraction between the biomolecules on the NP's surface (42). Due to the biomolecules on its surface, there are no harmful effects from exposure to GTiO2NPs. According to reports, the bioactivity of NPs produced through green syntheses, such as their ability to combat cancer and germs, is greatly enhanced by the capping agents provided by biomolecules (36, 43, 44).
Nanoparticles can be toxic to cells and tissues both in vivo and in vitro, depending on their dose and size (45). TiO2NPs are easily ingested by humans through a variety of methods and can disrupt the metabolism. The hepatotoxicity of TiO2NPs was deeply investigated by many researchers (11, 46–48). The main mechanism is the production of cellular oxidative stress, which in turn oxidizes the unsaturated lipids in the cell membrane, causing cellular leakage and liver's functional cell integrity loss (49). Compared to male rats, female rats were more susceptible to the toxicity of TiO2NPs. Recent research indicated that oxidative stress may be intimately related to this damage. The different antioxidant capacities of male and female rats may contribute to gender disparities.
Additionally, TiO2NPs taken orally could have several harmful health effects, such as liver damage, without appearing in the blood or other internal organs (50). This can be observed by the significant increase in the liver enzyme levels of ALT, AST, ALP, total bilirubin, and protein in groups 2 and 3. On the contrary, groups 4 and 5, treated with green TiO2NPs, showed a significant decrease in the AST level, which can be attributed to the antioxidant activity of garlic extract (34).
Garlic includes water-soluble organ sulfur compounds (OSCs) such as cycloalliin and S-allyl cysteine and oil-soluble OSCs like diallyl sulfide. According to a recent report, garlic-derived OSCs are responsible for the hepatoprotective effects of garlic (51). These OSCs have been recorded to enhance antioxidants such as glutathione, catalase, and GSH peroxidase (52). According to one study, pretreatment with aqueous garlic extract helped to mitigate the liver damage and oxidative stress caused by galactosamine/lipoploysaccharide (53).
The garlic extract has a sufficient proportion of polyphenols, sterols, and tocopherols. These active ingredients were employed in the hepatoprotective inquiry study and have been shown to have antioxidant, hypoglycemic, and cholesterol-lowering properties (54).
The histological studies confirmed the biochemical results. Hepatic abnormalities were noticed in the livers of maternal and embryo-fetal rats exposed to CHTiO2NPs in a dose-dependent manner. The observed hepatic abnormalities were congestion, dilatation of the blood vessels, necrosis, vacuolization, and inflammatory cell infiltration. The discovery of inflammatory cells in the liver suggests that TiO2NPs interact with enzymes and other proteins in the interstitium, disrupt the antioxidant defense system, and produce excessive amounts of ROS, leading to inflammatory conditions and blood vessel dilation because TiO2NPs disturb the permeability of cell membranes in hepatocytes and the endothelial lining of blood vessels (55). However, these abnormalities were reduced in the groups treated with GTiO2NPs linked with hepatocyte generation enhancement in a dose-dependent manner. These ameliorative effects could be attributed to garlic's bioactive compounds, such as diallyl sulfide, allicin, ajoene, and allium. These compounds are directly related to oxidative stress induction, lipid peroxidation, inflammatory responses, and apoptotic effects (31, 32).
Several conserved domains known as Bcl-2 homology (BH) are found in the Bcl-2 family of proteins. High homology is found in the BHs domain of the pro-apoptotic Bcl-2 family members Bax and Bak (56). The overexpression of the Bax protein causes apoptotic cell death (57). The immunohistochemical results reflect a positive detection of Bax in groups treated with both 100 mg/kg of CHTiO2NPs and 300 mg/kg of CHTiO2NPs, compared to a negative detection in the 100 mg/kg of GTiO2NPs, 300 mg/kg of GTiO2NPs, and the control group. These results suggested that the internalization of CHTiO2NPs produces ROS that disrupts the cellular redox balance, speeds up cell death and lipid peroxidation, changes gene expression, attaches to the mitochondrial membrane, and causes DNA damage and apoptosis in cells (58–61).
Conclusion
Garlic extract was successfully used to synthesize TiO2NPs. The produced TiO2NPs showed a sort of agglomeration with a semispherical shape and no toxic effect on HepG2 under our experimental conditions. Maternal exposure to chemically synthesized TiO2 NPs at higher doses induced a significant decrease in the maternal and fetal body weights, contrary with a significant increase in the mean level of serum AST and ALT activity and total protein level, with a remarkable histological alteration in the fetal and maternal livers. In addition, distinct positive staining of Bax is expressed in the hepatocytes, which directly indicates liver damage in both maternal and embryonic rats. Nevertheless, biosynthesis of TiO2NPs using garlic extract could mitigate the altered parameters and minimally affect the liver's normal architecture. Depending on the discussed findings, it could be concluded that the bioactivity of TiO2NPs can be modified with green synthesis using garlic extract. Compared to CHTiO2NPs, the exposure to GTiO2NPs showed reduced damage in the liver tissues of maternal and embryo-fetal rats.
Data availability statement
The original contributions presented in the study are included in the article/supplementary material, further inquiries can be directed to the corresponding authors.
Ethics statement
Animal Experimental Guidelines were followed, and the Animal Care and Use Committee of the Animal Health Research Institute, Faculty of Science, South Valley University, Qena, Egypt, approved the experimental procedures (approval no. 002/9/22).
Author contributions
ZK and AAE jointly developed the hypothesis and concept of the study, contributed to the chemical and material preparation, and the techniques performed. For this research and scientific paper, ZA-A, AHS, FZ, SO, AAM, and IFR were involved in the experimental procedures and analyses. All authors revised, edited, read, and approved the final manuscript.
Funding
This work was supported by Slovak Grant KEGA No. 012UVLF-4/2020: Implementation of modern and innovative technologies into the teaching process of physiology and the Academy of Scientific Research and Technology (ASRT), Egypt, Grant No. 6658.
Conflict of interest
The authors declare that the research was conducted in the absence of any commercial or financial relationships that could be construed as a potential conflict of interest.
Publisher's note
All claims expressed in this article are solely those of the authors and do not necessarily represent those of their affiliated organizations, or those of the publisher, the editors and the reviewers. Any product that may be evaluated in this article, or claim that may be made by its manufacturer, is not guaranteed or endorsed by the publisher.
References
1. Botelho MC, Costa C, Silva S, Costa S, Dhawan A, Oliveira PA, et al. Effects of titanium dioxide nanoparticles in human gastric epithelial cells in vitro. Biomed Pharmacother. (2014) 68:59–64. doi: 10.1016/j.biopha.2013.08.006
2. Wang Y, Cai R, Chen C. The nano–bio interactions of nanomedicines: understanding the biochemical driving forces and redox reactions. Acc Chem Res. (2019) 52:1507–18. doi: 10.1021/acs.accounts.9b00126
3. Vance ME, Kuiken T, Vejerano EP, McGinnis SP, Hochella MF, Hull DR. Nanotechnology in the real world: redeveloping the nanomaterial consumer products inventory. Beilstein J Nanotechnol. (2015) 6:1769–80. doi: 10.3762/bjnano.6.181
4. Weir A, Westerhoff P, Fabricius L, Hristovski K, Von Goetz N. Titanium dioxide nanoparticles in food and personal care products. Environ Sci Technol. (2012) 46:2242–50. doi: 10.1021/es204168d
5. Rashidi L, Khosravi-Darani K. The applications of nanotechnology in food industry. Crit Rev Food Sci Nutr. (2011) 51:723–30. doi: 10.1080/10408391003785417
6. Shi H, Magaye R, Castranova V, Zhao J. Titanium dioxide nanoparticles: a review of current toxicological data. Part Fibre Toxicol. (2013) 10:1–33. doi: 10.1186/1743-8977-10-15
7. Shinohara N, Danno N, Ichinose T, Sasaki T, Fukui H, Honda K, et al. Tissue distribution and clearance of intravenously administered titanium dioxide (TiO2) nanoparticles. Nanotoxicology. (2014) 8:132–41. doi: 10.3109/17435390.2012.763001
8. Ferin J, Oberdörster G, Penney DP. Pulmonary retention of ultrafine and fine particles in rats. Am J Respir Cell Mol Biol. (1992) 6:535–42. doi: 10.1165/ajrcmb/6.5.535
9. Thomas S, Harshita BSP, Mishra P, Talegaonkar S. Ceramic nanoparticles: fabrication methods and applications in drug delivery. Curr Pharm Des. (2015) 21:6165–88. doi: 10.2174/1381612821666151027153246
10. Moss O, Wong V. When nanoparticles get in the way: Impact of projected area on in vivo and in vitro macrophage function. Inhal Toxicol. (2006) 18:711–6. doi: 10.1080/08958370600747770
11. Wang J, Zhou G, Chen C, Yu H, Wang T, Ma Y, et al. Acute toxicity and biodistribution of different sized titanium dioxide particles in mice after oral administration. Toxicol Lett. (2007) 168:176–85. doi: 10.1016/j.toxlet.2006.12.001
12. Li JJ. en, Muralikrishnan S, Ng CT, Yung LYL, Bay BH. Nanoparticle-induced pulmonary toxicity. Exp Biol Med. (2010) 235:1025–33. doi: 10.1258/ebm.2010.010021
13. Ahmed S, Ahmad M, Swami BL, Ikram S, A. review on plants extract mediated synthesis of silver nanoparticles for antimicrobial applications: a green expertise. J Adv Res. (2016) 7:17–28. doi: 10.1016/j.jare.2015.02.007
14. Metwally AA, Abdel-Hady A-NAA, Haridy MAM, Ebnalwaled K, Saied AA, Soliman AS. Wound healing properties of green (using Lawsonia inermis leaf extract) and chemically synthesized ZnO nanoparticles in albino rats. Environ Sci Pollut Res. (2022) 29:23975–87. doi: 10.1007/s11356-021-17670-5
15. de Almeida CLF, Falcão H de S, Lima GR, Montenegro C de A, Lira NS, de Athayde-Filho PF, et al. Bioactivities from marine algae of the genus Gracilaria. Int J Mol Sci. (2011) 12:4550–73. doi: 10.3390/ijms12074550
16. Vanlalveni C, Lallianrawna S, Biswas A, Selvaraj M, Changmai B, Rokhum SL. Green synthesis of silver nanoparticles using plant extracts and their antimicrobial activities: a review of recent literature. RSC Adv. (2021) 11:2804–37. doi: 10.1039/D0RA09941D
17. Jadoun S, Arif R, Jangid NK, Meena RK. Green synthesis of nanoparticles using plant extracts: a review. Environ Chem Lett. (2021) 19:355–74. doi: 10.1007/s10311-020-01074-x
18. Tasduq SA, Singh K, Satti NK, Gupta DK, Suri KA, Johri RK. Terminalia chebula (fruit) prevents liver toxicity caused by sub-chronic administration of rifampicin, isoniazid and pyrazinamide in combination. Hum Exp Toxicol. (2006) 25:111–8. doi: 10.1191/0960327106ht601oa
19. Agarwal KC. Therapeutic actions of garlic constituents. Med Res Rev. (1996) 16:111–24. doi: 10.1002/(SICI)1098-1128(199601)16:1<111::AID-MED4=3.0.CO;2-5
20. Nemeth K, Piskula MK. Food content, processing, absorption and metabolism of onion flavonoids. Crit Rev Food Sci Nutr. (2007) 47:397–409. doi: 10.1080/10408390600846291
21. Zhang L, Xie X, Zhou Y, Yu D, Deng Y, Ouyang J, et al. Gestational exposure to titanium dioxide nanoparticles impairs the placentation through dysregulation of vascularization, proliferation and apoptosis in mice. Int J Nanomedicine. (2018) 13:777. doi: 10.2147/IJN.S152400
22. Sorbiun M, Shayegan Mehr E, Ramazani A, Mashhadi Malekzadeh A. Biosynthesis of metallic nanoparticles using plant extracts and evaluation of their antibacterial properties. Nanochemistry Res. (2018) 3:1–16. doi: 10.22036/NCR.2018.01.001
23. Meléndez-Villanueva MA, Morán-Santibañez K, Martínez-Sanmiguel JJ, Rangel-López R, Garza-Navarro MA, Rodríguez-Padilla C, et al. Virucidal activity of gold nanoparticles synthesized by green chemistry using garlic extract. Viruses. (2019) 11:1111. doi: 10.3390/v11121111
24. Sanghavin NM, Jivani NG, A. colorimetric method for the determination of nitrazepam. Talanta. (1979) 26:63–4. doi: 10.1016/0039-9140(79)80158-7
25. Gornall AG, Bardawill CJ, David MM. Determination of serum proteins by means of the biuret reaction. J Biol Chem. (1949) 177:751–66. doi: 10.1016/S0021-9258(18)57021-6
26. Doumas BT, Ard Watson W, Biggs HG. Albumin standards and the measurement of serum albumin with bromcresol green. Clin Chim Acta. (1971) 31:87–96. doi: 10.1016/0009-8981(71)90365-2
27. Larson K, Ho HH, Anumolu PL, Chen TM. Hematoxylin and eosin tissue stain in Mohs micrographic surgery: a review. Dermatologic Surg. (2011) 37:1089–99. doi: 10.1111/j.1524-4725.2011.02051.x
28. Orazizadeh M, Khorsandi L, Mansouri E, Fakhredini F. The effect of glycyrrhizin acid on Bax and Bcl2 expression in hepatotoxicity induced by Titanium dioxide nanoparticles in rats. Gastroenterol Hapatol Bed Bench. (2020) 13:168. doi: 10.22037/ghfbb.v13i2.1882
30. Ahmad TA, El-Sayed BA, El-Sayed LH. Development of immunization trials against Eimeria spp. Trials Vaccinol. (2016) 5:38–47. doi: 10.1016/j.trivac.2016.02.001
31. Aviello G, Abenavoli L, Borrelli F, Capasso R, Izzo AA, Lembo F, et al. Garlic: empiricism or science? PubMed. Nat Prod Commun. (2009) 4:1934578X0900401231. doi: 10.1177/1934578X0900401231
32. Colín-González AL, Santana RA, Silva-Islas CA, Chánez-Cárdenas ME, Santamaría A, Maldonado PD. The antioxidant mechanisms underlying the aged garlic extract- and S-allylcysteine-induced protection. Oxid Med Cell Longev. (2012) 2012:1–14. doi: 10.1155/2012/907162
33. Rahalathan P, Kumar S, Raja B. Reno-protective effect of garlic extract against immobilization stress induced changes in rats. Asian Pac J Trop Biomed. (2012) 2:443–8. doi: 10.1016/S2221-1691(15)30370-1
34. Pereira-Wilso C. DNA damage protection and induction of repair by dietary phytochemicals and cancer prevention: What do we know? Sel Top DNA Repair. (2011) 26:237–70. doi: 10.5772/22125
35. Dudefoi W, Moniz K, Allen-Vercoe E, Ropers MH, Walker VK. Impact of food grade and nano-TiO2 particles on a human intestinal community. Food Chem Toxicol. (2017) 106:242–9. doi: 10.1016/j.fct.2017.05.050
36. Hashem M, Al-Karagoly H. Synthesis, characterization, and cytotoxicity of titanium dioxide nanoparticles and in vitro study of its impact on lead concentrations in bovine blood and milk. J Biotech Res. (2021) 12:93–105.
37. Nel A, Xia T, Mädler L, Li N. Toxic potential of materials at the nanolevel. Science. (2006) 311:622–7. doi: 10.1126/science.1114397
38. Singh P, Kim YJ, Zhang D, Yang DC. Biological synthesis of nanoparticles from plants and microorganisms. Trends Biotechnol. (2016) 34:588–99. doi: 10.1016/j.tibtech.2016.02.006
39. Al-Shabib NA, Husain FM, Qais FA, Ahmad N, Khan A, Alyousef AA, et al. Phyto-mediated synthesis of porous titanium dioxide nanoparticles from withania somnifera root extract: broad-spectrum attenuation of biofilm and cytotoxic properties against hepg2 cell lines. Front Microbiol. (2020) 11:1680. doi: 10.3389/fmicb.2020.01680
40. Arya S, Sonawane H, Math S, Tambade P, Chaskar M, Shinde D. Biogenic titanium nanoparticles (TiO2NPs) from Tricoderma citrinoviride extract: synthesis, characterization and antibacterial activity against extremely drug-resistant Pseudomonas aeruginosa. Int Nano Lett. (2021) 11:35–42. doi: 10.1007/s40089-020-00320-y
41. Dunlap RA. X-ray diffraction techniques. Nov Microstruct Solids. (2018) 2–12. doi: 10.1088/2053-2571/aae653ch2
42. Arabi N, Kianvash A, Hajalilou A, Abouzari-Lotf E, Abbasi-Chianeh V, A. facile and green synthetic approach toward fabrication of Alcea- and Thyme-stabilized TiO2 nanoparticles for photocatalytic applications. Arab J Chem. (2020) 13:2132–41. doi: 10.1016/j.arabjc.2018.03.014
43. Nadeem M, Tungmunnithum D, Hano C, Abbasi BH, Hashmi SS, Ahmad W, et al. The current trends in the green syntheses of titanium oxide nanoparticles and their applications. Green Chem Lett Rev. (2018) 11:492–502. doi: 10.1080/17518253.2018.1538430
44. Długosz O, Szostak K, Staroń A, Pulit-Prociak J, Banach M. Methods for reducing the toxicity of metal and metal oxide NPs as biomedicine. Materials. (2020) 13:279. doi: 10.3390/ma13020279
45. Ashraf S, Said AH, Hartmann R, Assmann A, Feliu N, Lenz P, Parak WJ. Quantitative particle uptake by cells as analyzed by different methods. Angew Chemie Int Ed. (2020) 2–18. doi: 10.1002/anie.201906303
46. El-behairy DSA, Diaa Eldin NH, Elbadry EE, Mohamed AA, Farrag IM. Hepato-renal toxicity of titanium dioxide nanoparticles and the protective effects of moringa oleifera leaves extract and vitamin e in male albino rats Egypt. J Hosp Med. (2019) 75:2261–71. doi: 10.21608/ejhm.2019.30526
47. Alarifi S, Ali D, Al-Doaiss AA, Ali BA, Ahmed M, Al-Khedhairy AA. Histologic and apoptotic changes induced by titanium dioxide nanoparticles in the livers of rats. Int J Nanomedicine. (2013) 8:3937–43. doi: 10.2147/IJN.S47174
48. Rizk MZ, Ali SA, Hamed MA, El-Rigal NS, Aly HF, Salah HH. Toxicity of titanium dioxide nanoparticles: Effect of dose and time on biochemical disturbance, oxidative stress and gentoxicity in mice. Biomed Pharmacother. (2017) 90:466–72. doi: 10.1016/j.biopha.2017.03.089
49. Liu H, Ma L, Zhao J, Liu J, Yan J, Ruan J, et al. Biochemical toxicity of nano-anatase TiO2 particles in mice. Biol Trace Elem Res. (2009) 129:170–80. doi: 10.1007/s12011-008-8285-6
50. Chen Z, Zhou D, Zhou S, Jia G. Gender difference in hepatic toxicity of titanium dioxide nanoparticles after subchronic oral exposure in Sprague-Dawley rats. J Appl Toxicol. (2019) 39:807–19. doi: 10.1002/jat.3769
51. Guan M-J, Zhao N, Xie K-Q, Zeng T. Hepatoprotective effects of garlic against ethanol-induced liver injury: a mini-review. Food Chem Toxicol. (2018) 111:467–73. doi: 10.1016/j.fct.2017.11.059
52. Lin C, Yin M. Effects of cysteine-containing compounds on biosynthesis of triacylglycerol and cholesterol and anti-oxidative protection in liver from mice consuming a high-fat diet. Br J Nutr. (2008) 99:37–43. doi: 10.1017/S0007114507793881
53. El-Beshbishy HA. Aqueous garlic extract attenuates hepatitis and oxidative stress induced by galactosamine/lipoploysaccharide in rats. Phyther Res An Int J Devoted to Pharmacol Toxicol Eval Nat Prod Deriv. (2008) 22:1372–9. doi: 10.1002/ptr.2505
54. Pathak S, Catanzaro R, Vasan D, Marotta F, Chabria Y, Jothimani G, et al. Benefits of aged garlic extract in modulating toxicity biomarkers against p-dimethylaminoazobenzene and phenobarbital induced liver damage in Rattus norvegicus. Drug Chem Toxicol. (2020) 43:454–67. doi: 10.1080/01480545.2018.1499773
55. Shakeel M, Jabeen F, Qureshi NA, Fakhr-e-Alam M. toxic effects of titanium dioxide nanoparticles and titanium dioxide bulk salt in the liver and blood of male sprague-dawley rats assessed by different assays. Biol Trace Elem Res. (2016) 173:405–26. doi: 10.1007/s12011-016-0677-4
56. Zhang HM, Cheung P, Yanagawa B, McManus BM, Yang DC. BNips: A group of Pro-apoptotic Proteins in The Bcl-2 Family. Apoptosis. (2003) 8:229–36. doi: 10.1023/a:1023616620970
57. Hara A, Iwai T, niwa M, Uematsu T, Yoshimi N, Tanaka T, Mori H. Immunohistochemical detection of bax and Bcl2 proteins in gerbil hippocampus following transient forebrain ischemia. Brain Res. (1996) 711:249–53. doi: 10.1016/0006-8993(95)01436-5
58. Hong J, Zhang YQ. Murine liver damage caused by exposure to nano-titanium dioxide. Nanotechnology. (2016) 27:1122001. doi: 10.1088/0957-4484/27/11/112001
59. Cui Y, Gong X, Duan Y, Li N, Hu R, Liu H, et al. Hepatocyte apoptosis and its molecular mechanisms in mice caused by titanium dioxide nanoparticles. J Hazard Mater. (2010) 183:874–80. doi: 10.1016/j.jhazmat.2010.07.109
60. Essers J, Theil AF, Baldeyron C, van Cappellen WA, Houtsmuller AB, Kanaar R, et al. Nuclear dynamics of PCNA in DNA replication and repair. Mol Cell Biol. (2005) 25:9350–9. doi: 10.1128/MCB.25.21.9350-9359.2005
Keywords: Bax-immunohistochemically, garlic, bodyweight, fetuses, histology, liver, TiO2NPs
Citation: Kamal Z, Ebnalwaled AA, Al-Amgad Z, Said AH, Metwally AA, Zigo F, Ondrašovičová S and Rehan IF (2022) Ameliorative effect of biosynthesized titanium dioxide nanoparticles using garlic extract on the body weight and developmental toxicity of liver in albino rats compared with chemically synthesized nanoparticles. Front. Vet. Sci. 9:1049817. doi: 10.3389/fvets.2022.1049817
Received: 21 September 2022; Accepted: 11 November 2022;
Published: 16 December 2022.
Edited by:
Xun Wang, Sichuan Agricultural University, ChinaReviewed by:
Leyla Rios, Mississippi State University, United StatesSabreen Ezzat Fadl, Matrouh University, Egypt
Copyright © 2022 Kamal, Ebnalwaled, Al-Amgad, Said, Metwally, Zigo, Ondrašovičová and Rehan. This is an open-access article distributed under the terms of the Creative Commons Attribution License (CC BY). The use, distribution or reproduction in other forums is permitted, provided the original author(s) and the copyright owner(s) are credited and that the original publication in this journal is cited, in accordance with accepted academic practice. No use, distribution or reproduction is permitted which does not comply with these terms.
*Correspondence: Ibrahim F. Rehan, ibrahim.rehan@vet.menofia.edu.eg; František Zigo, frantisek.zigo@uvlf.sk