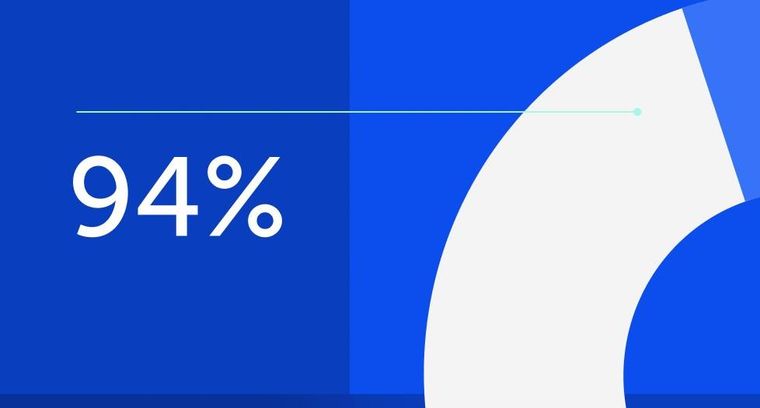
94% of researchers rate our articles as excellent or good
Learn more about the work of our research integrity team to safeguard the quality of each article we publish.
Find out more
ORIGINAL RESEARCH article
Front. Vet. Sci., 06 January 2023
Sec. Veterinary Pharmacology and Toxicology
Volume 9 - 2022 | https://doi.org/10.3389/fvets.2022.1025249
Neutrophils display an array of biological functions including the formation of neutrophil extracellular traps (NETs), web-like structures specialized in trapping, neutralizing, killing and preventing microbial dissemination within the host. However, NETs contribute to a number of inflammatory pathologies, including severe equine asthma. Tamoxifen (TX) is a selective estrogen receptor modulator which belongs to the triphenylethyllenes group of molecules, and which is used as a treatment in all stages of estrogen-positive human breast cancer. Our previous results suggest that tamoxifen can modulate neutrophil functionality and promote resolution of inflammation; this would partly explain the clinical beneficial effect of this drug in horses with airway inflammation. Enhanced NETs production has been reported with tamoxifen use in humans, but minimal data exists regarding the drug's effect on NETs in horses. The aim of this study is to assess the in vitro effect of TX on NETs formation from peripheral blood of healthy horses. Five clinically healthy mixed-breed adult horses were enrolled in the study. For this, cellular free DNA quantification, immunofluorescence for the visualization of NETs, assessment of different types of NETs, and detection of mitochondrial superoxide. TX induced NETs formation at a concentration of 10 uM. Our results show that only two types of NETs were induced by TX: 95% spread NETs (sprNETs) and 5% aggregated NETs (aggNETs). Furthermore, induction of these NETs could be influenced by mitochondrial ROS. Future research should involve an In vivo study of horses with severe asthma and TX treatment, to evaluate BALF neutrophil NET formation. In conclusion, this in vitro study suggests that the resolution of inflammation by TX in horses with airway inflammation is due to inhibition of other neutrophilic functions but not to NET formation.
Neutrophils, the most abundant and important cell component of the innate immune system play a major role in host defense as the first cellular type to be recruited to fight infection and inflammation (1, 2). They are the most abundant leukocytes in circulation and are first recruited to the infected sites. Here, neutrophils destroy pathogens via generation of reactive oxygen species (ROS), phagocytosis, and formation of neutrophil extracellular traps (NETs) (3). NETs are large, web-like structures composed of decondensed chromatin associated with histones and decorated with multiple antimicrobial proteins including elastase (NE) and myeloperoxidase (MPO) (4). These extracellular structures trap, neutralize, kill and prevent microbial dissemination within the host (5). The regulated process of NETs formation (NETosis) depends on the cellular mechanism triggered and activated by a wide range of stimuli (6).
Despite their protective role, dysregulated or excessive NETs formation can be detrimental to surrounding tissues and contribute to many immune-related diseases (5, 6). NETs can play a pathogenic role in many infectious and non-infectious human diseases, including respiratory cases with massive influx of neutrophils into the airways (7, 8). Excessive NETs release is particularly deleterious in lung diseases because NETs can easily expand in the alveolar space, causing lung injury (8), and epithelial and endothelial cell death (9). In horses, inflammatory processes are characterized by a strong neutrophilic response (2), as observed in most asthma-affected horses (10). The presence of NETs in the bronchoalveolar lavage fluid (BALF) from asthma cases but not in the fluid from healthy or remission horses (11), suggests that exacerbated NETosis may play a role as a possible and important promotor of the disease (12).
Tamoxifen (TX), a selective estrogen receptor modulator which belongs to the triphenylethyllenes group of molecules, is used as a treatment in all stages of estrogen-positive human breast cancer. It has potent anti-inflammatory effects: we have described an early pro-apoptotic effect of tamoxifen in granulocytic cells, both in vitro with peripheral blood and BALF (13), and in vivo (14); in addition, we showed that tamoxifen and its metabolites can activate the intrinsic apoptotic pathway in equine neutrophils (15). Our laboratory group has also shown that tamoxifen has an inhibitory action on respiratory burst, chemotaxis and chemokinesis of equine neutrophils in a dose-dependent manner (16, 17), and that tamoxifen induces efferocytosis by macrophages and modulates immune function through an estrogen-independent mechanism (18, 19). In vivo, our group demonstrated that horses with airway inflammation treated with 0.25 mg/kg PO of tamoxifen for five consecutive days display a significant decrease in BALF neutrophils and concomitant improvement in their clinical status in a manner similar to, or even better than that observed in hoses treated with dexamethasone (14). An independent group also found that tamoxifen produces an improvement in airway resistance in horses with exacerbated severe equine asthma, although no significant improvement in BALF neutrophil counts was observed in that study (20). Pharmacokinetic studies conducted by our laboratory showed that it is possible to measure tamoxifen as well as its main metabolites in blood as described in other species (21).
Since their first description in 2004, NETs are now known to play a role in physiology and pathology of different diseases (22), but the detailed mechanisms underlying NETs formation are not fully elucidated in horse neutrophils. Moreover, the effect of TX on NETs production in horses remains unknown. Therefore, the aim of this study is to assess the in vitro effect of tamoxifen on NETs formation from peripheral blood of healthy horses.
Five clinically healthy mixed-breed adult horses (four mares and one gelding, body weight 420–450 kg, age 8–12 years) belonging to and housed at the Universidad Austral de Chile veterinary teaching hospital were enrolled in this study. All animals were housed on pasture and fed grass with free access to water. Physical examinations were performed before sample collection by qualified veterinarians to ensure animals' health. Each horse was sampled once, and each sample was analyzed independently. All procedures were approved by the Universidad Austral de Chile Bioethics Committee for the Use of Animals in Biomedical Research (approval resolution n° 348/2019).
The isolation of blood leukocytes was performed as previously described by our group (14, 16). Briefly, 10 mL of blood obtained by jugular venipuncture was placed in sterile tubes containing 1 mL of 3.8% w/v trisodium citrate. Blood was placed on a discontinuous density gradient (Percoll®, GE Healthcare), with 4 mL of 85% Percoll in the bottom of a 15 mL tube and 4 mL of 70% Percoll above. After centrifugation (45 min, 670 × g), the upper layer containing mononuclear cells, and the lower layer containing granulocytes, were aspirated for further processing. Granulocyte cell purity and viability were assessed by flow cytometry (BD FACS Canto II). Viability was assessed using an Annexin V assay as previously described by our group (14). Cells were subsequently prepared for bioassays.
Quantification of extracellular free DNA was measured by spectrofluorometry. PMNs (1 × 106) resuspended in Hanks' Balanced Salt Solution (HBSS, 136 mM NaCl, 5.3 mM KCl, 5.5 mM glucose, 1 mM MgCl2, 10 mM HEPES, 1.2 mM CaCl2, pH 7.4) were incubated in the presence of tamoxifen (TX, 10 μM, Sigma-Aldrich) or DMSO (0.1%, drug vehicle) for 30 min at 37°C with 5% CO2. Then, to stimulate NETs formation (positive control), cells were incubated with phorbol-12-myristate-13-acetate (PMA; 200 nM, Merck Millipore) for 1 h at 37°C with 5% CO2. Next, micrococcal nuclease (5U/well, New England Biolabs, Ipswich, MA, USA) was added to each sample, and incubated for 15 min at 37°C with 5% CO2. Cells were centrifugated at 300 × g for 5 min, and 100 μL of the supernatants were collected and transferred to a 96-well plate. PicoGreenTM (1:200, Invitrogen, Carlsbad, CA, USA) was added to each well, followed by a 5 min incubation at RT. DNA content and NETs formation was analyzed using an automated Varioskan Flash Reader (Thermo Fisher Scientific, MA, USA) at 485 nm excitation and 530 nm emission.
Fresh isolated blood-derived equine polymorphonuclear cells were resuspended in RPMI medium (Cytiva,HyClone) supplemented with 2% fetal bovine serum. 2.5 × 105 cells/wells (24 wells plate) were then seeded on 12 mm glass coverslips (pre-treated with 0.03% polylysine) and treated with tamoxifen (TX 10 μM; Sigma-Aldrich) or phorbol-12-myristate-13-acetate (PMA 200 nM; Merck Millipore) for 120 min at 37 °C with 5% CO2. Culture slides were fixed in 2% paraformaldehyde and permeabilized with 0.1% Triton X-100, in PBS.
NETs from samples on point 2.4 were evaluated using immunofluorescence. Fixed cells were blocked with 3% bovine serum albumin (#9048-46-8, Sigma Aldrich) for 30 min. After this time, coverslips were incubated overnight at 4°C in the presence of rabbit anti-Histone H4 (Citrulline 3) (07-596, 1:200, Sigma-Aldrich). Next day, coverslips were washed three times with 1X PBS-T (0.05% Triton X-100) and then incubated for 2 hours at room temperature with anti-rabbit IgG (H+L) conjugated to Alexa Fluor 594® (A-21070, 1:1000, Invitrogen). After this time and three washes with 1X PBS-T, nuclei acids were stained with PicoGreen™ (1:1000, Invitrogen) for 30 min at room temperature. Finally, coverslips were washed with 1X PBS-T and mounted using fluorescence mounting medium (Dako North America, Inc). Epifluorescence observations and photo documentation were performed using a Leica DM 2000 led microscope (Leica, Germany) equipped with a digital camera and ocular software (QImagin, Canada). Images were captured with settings kept consistent during collection of all images and processed with ImageJ software. Quantification of NETs was assessed with the DNA area and NETosis analysis (DANA) as described by Rebernick et al. (23). Data is expressed as NETosis percentage (%) and DNA area (μm2).
Four randomly selected images from each experimental condition were then microscopically analyzed according to the typical morphological features according to Muñoz-Caro et al. (24) and Lange et al. (25): diffuse NETs (diffNETs), aggregated NETs (aggNETs) and spread NETs (sprNETs). The diffNETs are described as consisting of a globular and compact form with a size of 25–28 nm diameter. Whereas, sprNETs were observed consisting of smooth and elongated web- like structures composed exclusively by thin fibers with a diameter of 15–17 nm. The aggNETs, being defined as clusters of NET-like structures with a “ball of yarn” morphology and a size of more than 20 μm in diameter. Within each sample, structures with the specific features described above were counted.
For measurement of mitochondrial ROS (mtROS) release, 1 × 106 neutrophils were incubated with 10 μM rotenone (Tocris Bioscience, Bristol, UK), 200 μm MitoTEMPO (Cayman Chemical, Ann Arbor, MI, USA), 10 μM TX or TX plus rotenone and loaded with 2.5 μM MitoSOX™ Red Mitochondrial Superoxide Indicator (Invitrogen™, Thermo Fisher Scientific, Waltham, MA, USA) at 37 °C for 30 min. After incubation, MitoSOX™ fluorescence intensity was measured using a BD FACSCanto II flow cytometer (Becton Dickinson, Franklin Lakes, NJ, USA) at wavelengths of 510 nm excitation and 580 nm emission.
Results are presented as mean ± standard deviation (SD). Kolmogorov–Smirnov tests showed that the data were normally distributed. A one-way ANOVA was performed between treatments for cellular free DNA quantification, followed by a Tukey's HSD post hoc when significant differences were found. For DNA area and NETosis analysis (DANA), DNA area (μm2) was analyzed with a one-way ANOVA test followed by a Tukey's HSD post hoc when significant differences were found. Mitochondrial superoxide was analyzed with a one-way ANOVA test followed by a Tukey's test. Statistical analyses were performed with the commercial software GraphPad Prism for windows (GraphPad PRISM software v9.1.0, La Jolla California, USA). Statistical significance was defined at a p-value < 0.05.
Our results shows that tamoxifen (TX) acts as a NET-inducing factor in equine polymorphonuclear cells of healthy adult horses. In vitro production of cf-DNA, quantified in the supernatants of PMN samples by spectrofluorometry, differed significantly between unstimulated cells and those treated with PMA (p < 0.05) or TX (p < 0.05) (Figure 1). The same effect was observed between the drug vehicle treated cells (DMSO) when compared to both, PMA (p < 0.05) and TX (p < 0.05) treated cells (Figure 1). Both stimuli showed a similar and significant increase in extracellular free-DNA concentrations, with no significative differences between PMA and TX treated cells (p > 0.05) (Figure 1). No statistically significant differences were observed between control cells and those incubated with DMSO (p > 0.05) (Figure 1).
Figure 1. Tamoxifen (TX) triggers the release of cellular free-DNA (cf-DNA) from peripheral blood neutrophils of adult healthy horses. NETosis was quantified by cellular free-DNA measured in the supernatants of fresh-blood isolated neutrophils in the presence or absence of stimuli by spectrofluorometry. Unstimulated control cells (HBSS) and cells treated with DMSO (0.1%), PMA (200 nM) and TX (10 μM) were evaluated. Data is presented as means ± standard deviation (SD). RFU, relative fluorescence units;*p < 0.05; n = 5.
Consistent with these findings, immunofluorescence samples marked with the DNA staining PicoGreen™, showed positive DNA extrusion consistent with NETs structures for PMNs samples treated with TX or PMA. Immunofluorescence samples incubated with citrullinated histone H4 antibody showed positive DNA (PicoGreen™) and citrullinated H4 antibody staining for both TX and PMA treated cells. For control cells, minimal DNA strands-like structures and minimal positive marks for citrullinated histone H4 antibody were observed (Figure 2). For the DANA analysis, our results showed a statistically significant increase in the quantification of DNA area (μm2) for treated cells with TX (p < 0.05) and PMA (p < 0.01) when compared to unstimulated control cells (Figure 3). The same tendency was observed for NETosis percentage quantification, where a significant increase was observed for both TX (p < 0.05)and PMA (P < 0.01) treated cells when compared to the control cells (Figure 3).
Figure 2. Tamoxifen (TX) stimulates equine PMNs NETosis. Representative fluorescence of equine PMNs treated with TX (10 μM) or PMA (200 nM) and marked with DNA staining PicoGreenTM. Strand-like NETs structures marked with PicoGreenTM (green) and citrullinated histone H4 antibody (red) for control and treated cells with TX (10 μM) and PMA (200 nM). Scale bars = 30μm.
Figure 3. Tamoxifen (TX) induced the formation and release of NETs quantified by DNA area and NETosis (DANA) analysis. (A) Quantification of DNA area (μm2) and (B) percentage of NETosis for the three conditions studied. PMA (200 nM) was used as a positive control. Data are presented as mean ± standard deviation (SD). *p < 0.05, **p < 0.01; n = 5.
In general, NETs can manifest in different morphological forms, i.e., as diffNETs, aggNETs and sprNETs. Our results show that only two types of NETs were induced by TX. Morphological analysis showed that TX induced mainly sprNETs and to a lesser extent aggNETs. The sprNETs consist of smooth, elongated, cobweb-like structures of decondensed chromatin and antimicrobial proteins and are composed exclusively of fine fibers (Figure 4). The aggNETs, which are defined as clusters of NET-like structures with a “ball of yarn” morphology (Figure 5) (26). Quantification of the total samples showed that TX-induced sprNETs were 95%, while TX-induced aggNETs were only 5% of the total. No other NETs were found in the samples.
Figure 4. Tamoxifen triggers netosis mainly of the sprNETS type. Representative fluorescence of equine PMNs treated with TX (10 μM) and marked with DNA staining PicoGreenTM. Strand-like NETs structures marked with PicoGreenTM (green) and citrullinated histone H4 antibody (red). Note the formation of sprNETs (consist of smooth, elongated, cobweb-like structures of decondensed chromatin, composed exclusively of fine fibers). Scale bars = 30μm.
Figure 5. Tamoxifen triggers aggNETs type netosis in a very low percentage. Representative fluorescence of equine PMNs treated with TX (10 μM) and marked with DNA staining PicoGreenTM. Strand-like NETs structures marked with PicoGreenTM (green) and citrullinated histone H4 antibody (red). Note the formation of aggNETs defined as clusters of NET-like structures with a “ball of yarn” morphology.
The effect of TX on mitochondrial ROS generation was assessed with the mitochondrial superoxide indicator mitosox. Cells treated with 10 uM TX showed a clear increase in mtROS levels, which was significantly enhanced when neutrophils were also pre-incubated with 10 uM rotenone (inhibitor of complex I of the electron chain). On the other hand, the effect of TX on mtROS was significantly reduced when cells were pre-incubated with the antioxidant mitoTEMPO (Figure 6).
Figure 6. Tamoxifen induces mitochondrial ROS production. Equine neutrophils were stained with Mitosox and incubated with tamoxifen (10 uM), rotenone (10 uM), mitotempo(200 uM) or vehicle (DMSO 0.1%) for 30 min at 37°C. Where indicated, prior to the addition of tamoxifen (10 uM) the neutrophils were preincubated with rotenone (10 uM) or mitotempo (200 uM) for 30 min at 37°C. The mean fluorescence intensity (MIF) was analyzed by flow cytometry. The result corresponds to the average +/- S.E.M of 5 independent experiments.*p < 0.05; **p < 0.01.
Our study results show that the stimulation of equine polymorphonuclear cells with TX triggers the production of NETs in healthy adult horses. In humans, TX has been shown to strongly promote NETs production in neutrophils by an estrogen receptor-independent pathway, which seems to be modulated by sphingolipid biosynthesis, which plays an important role in neutrophil activity, and is considered a ROS-independent Ceramide/PKCζ signaling pathway (27). Furthermore, the TX concentration of 10 μm was used in this study, based on the findings of Corriden et al. (27) in which he observed NETs in human neutrophils using this TX concentration.
During NET formation, the characteristic nuclear lobules of neutrophils disappear, and the chromatin expands while the cytoplasmic membrane remains intact. Two hours after stimulation, in this case with TX, this membrane ruptures, releasing chromatin decorated with antimicrobial proteins. The most marked morphological changes during netosis occur in the area, perimeter and shape of the nucleus. We observed that treatment with 10 μM TX induces spread-forming NETs (Figure 3). These sprNETs occupy three to five times the volume of condensed chromatin. Several proteins, as mentioned above, are attached to NETs, including histones and more than 30 components of primary and secondary granules, including components with bactericidal activity such as elastase, myeloperoxidase, cathepsin G, lactoferrin pentraxin 3, gelatinase, proteinase 3, LL37, peptidoglycan-binding proteins, and others with bactericidal activity capable of destroying virulence factors (4).
The importance of NETs in the progression of human asthma is controversial. Neutrophils do increase in sputum or BALF samples from severely asthmatic patients, either with acute exacerbation episodes or with persistent asthma (28). NETs can be deleterious to epithelial barrier function in the lung (29) and they can also promote proinflammatory mediator secretion from dendritic cells and protease release from neutrophils (30). Some authors suggest that NETs may act directly on airway epithelial cells, aggravating airway inflammation and respiratory clinical signs (31, 32). Proteases contained in NETs also activate proinflammatory cytokines, aggravating the inflammatory response even more (33). Together, all these studies suggest that NETs damage the airway epithelium, increase cytokine secretion, and worsen asthma. In asthmatic horses, NETs are found in BALF and correlate with clinical severity (34). Our previous studies mentioned above suggest that TX can modulate neutrophil functionality in equines; this would partly explain the beneficial effect of this drug in horses with airway inflammation. However, it was a surprise to observe that TX could trigger NETs formation. It seems that the inflammation-resolving effect of TX in horses with neutrophilic airway inflammation could be due to the other neutrophilic functional inhibitions described above, rather than through the inhibition of NETs triggering. It would certainly be necessary to perform an in vivo study of horses with severe asthma, treating them with TX and observing the behavior of neutrophils in BALF with respect to NETs formation.
On the other hand, another interesting finding in this study was that 5% of all NETs were aggNETs. In human gout, aggNETs produced by neutrophils induced by monosodium urate crystals (MSU) apparently orchestrate the resolution of inflammation through the degradation of inflammatory cytokines (35). The anti-inflammatory effect of aggNETs is produced by serine proteases which proteolytically degrade inflammatory cytokines and chemokines; this could have a potential impact on different inflammatory diseases associated with neutrophil accumulation (36). Moreover, Mahajan et al. (37) note that ocular rheuma, which forms after night-time sleep or after prolonged eye closure, favors a continuous infiltration of neutrophils on ocular surfaces. These authors demonstrated the coordinated sequence of events of neutrophil activity during eye closure, including infiltration, degranulation and NET formation, and found that aggNETs resolved neutrophilic inflammation by degrading cytokines and chemokines and thereby preventing overload, uncontrolled neutrophil recruitment and activation. As stated above, proteolytic degradation of inflammatory mediators by aggNETs results in the resolution of gouty arthritis in humans and mice (10). Therefore, the formation of aggNETs could be considered a type of negative feedback to promote the resolution of inflammation and as protection to contain neutrophilic inflammation, since independently of the stimulus that triggers aggNETs formation, at high enough cell density, proteolytic degradation of inflammatory mediators outpaces the release of inflammatory mediators, leading to resolution of inflammation as degradation is faster than release (36). However, some authors conclude that this mechanism (aggNETs) probably does not have a major role in vivo in the context of MSU-crystal-induced footpad or joint inflammation in mice (38).
Equine neutrophils reactive oxygen species (ROS) production was dampened after treatment with TX (16). Lim et al. (39) had previously reported an inhibitory effect of TX 5 μM on H2O2 production in TPA-stimulated neutrophils from healthy humans. Other authors also observed a profound inhibition of ROS production in stimulated human neutrophils after treatment with TX (27, 40). Thus, the source of ROS that triggers NETs by TX should come from the mitochondria. Likewise, Albornoz et al. (15) showed that tamoxifen, N-desmethyltamoxifen and endoxifen depolarize the mitochondrial membrane and activate caspase-3 in healthy equine neutrophils in vitro. These results suggest that TX has the capacity to produce alterations in mitochondria, probably by stimulating the release of mtROS. Mitochondria have important roles in cellular function, including participation in energy generation and modulation of apoptosis (41). In addition, mitochondria produce large amounts of ROS and are important participants in redox-dependent intracellular signaling (42). For a long time, it was believed that mitochondria do not play a significant role in the functioning of neutrophils, since their content in these cells is low, energy supply is supported by glycolysis, and NADPH oxidase is the main source of ROS. However, mitochondrial dysfunction and oxidative stress in and around mitochondria have been implicated in pathogenic mechanisms, including inflammation and autoimmune reactions (43). Mitochondria are involved in the transmission of signals that determine main neutrophil responses to pathogens (44). It was found that mitochondrial ROS are involved in the activation of NADPH oxidase and in the induction of NETosis caused by various stimuli (45, 46). Therefore, the present study wanted to test whether TX can trigger mtROS, which would partly explain the formation of NETs. For this, rotenone was used as a strong inhibitor of complex I of the mitochondrial respiratory chain. The mechanism of action comprises inhibition of electron transfer from the iron-sulfur centers in complex I to ubiquinone, leading to a blockade of oxidative phosphorylation with limited synthesis of ATP (47). Furthermore, incomplete electron transfer to oxygen could lead to ROS formation. This rotenone-induced ROS production, with an assumed damage of mitochondria components, including mitochondrial DNA, can eventually lead to apoptosis (48). However, at our incubation times rotenone did not influence significative mtROS release; but in the presence of tamoxifen there was a synergistic effect between the two drugs. Activation of NADPH oxidase by mtROS was first demonstrated by Dikalov and coworkers (28) in endothelial cells by mitoTEMPO. This drug is a mitochondria-targeted antioxidant that prevents mitochondrial oxidative damage. Our results indicate that the use of mitoTEMPO reduces the levels of mtROS produced by TX in equine neutrophils (Figure 5). This latter result may suggest that mitochondria play an important role in the formation of NETs in the presence of TX. However, a weakness of this study was the non-performance of immunofluorescence to observe the effect of TX on NETs production in the presence of rotenone and mitoTEMPO.
In conclusion, neutrophils and NETs play an important role in the defense against invading pathogens, possibly in the resolution of inflammation. NETs and NET-associated proteases trap and clear pathogens; aggNETs could have anti-inflammatory effects by clearing and degrading inflammatory cytokines. TX triggered NETs formation (mainly sprNEts and to a very low percentage aggNETs). Furthermore, this study suggests that TX- induced NETs in equine neutrophils may be triggered by mtROS. However, disruption of the delicate balance between the formation, degradation and elimination of NETs may contribute to the development and pathogenesis of various inflammatory diseases such as equine asthma. NETs and their components interact with other cells of the innate and adaptive immune system and thus modulate immune responses. Therefore, it is imperative to perform in vivo studies to see the real effect of TX on NET production.
The raw data supporting the conclusions of this article will be made available by the authors, without undue reservation.
All procedures were approved by the Universidad Austral de Chile Bioethics Committee for the Use of Animals in Biomedical Research (approval resolution n° 348/2019).
CS, KB, JS, and AA performed the experiments. JQ, PA, and RB performed the data analysis. RB, CH, PE, BU, and GM conceived and designed the experiments. GM and BU wrote the manuscript. All authors contributed to the article and approved the submitted version.
This work was supported by grants N° 1190369, 1210839, and 1201635 from Fondo Nacional de desarrollo Científico y Tecnológico (FONDECYT) from National Agency for Research and Development (ANID, Chile).
The authors declare that the research was conducted in the absence of any commercial or financial relationships that could be construed as a potential conflict of interest.
All claims expressed in this article are solely those of the authors and do not necessarily represent those of their affiliated organizations, or those of the publisher, the editors and the reviewers. Any product that may be evaluated in this article, or claim that may be made by its manufacturer, is not guaranteed or endorsed by the publisher.
1. Teng TS, Ji AL, Ji XY, Li YZ. Neutrophils and immunity: from bactericidal action to being conquered. J Immunol Res. (2017) 2017:9671604. doi: 10.1155/2017/9671604
2. Anderson SL, Singh B. Equine neutrophils and their role in ischemia reperfusion injury and lung inflammation. Cell Tissue Res. (2018) 371:639–48. doi: 10.1007/s00441-017-2770-1
3. Rosales C. Neutrophil: A cell with many roles in inflammation or several cell types? Front Physiol. (2018) 9:113. doi: 10.3389/fphys.2018.00113
4. Delgado-Rizo V, Martínez-Guzmán MA, Iñiguez-Gutierrez L, García-Orozco A, Alvarado-Navarro A, Fafutis-Morris M. Neutrophil extracellular traps and its implications in inflammation: an overview. Front Immunol. (2017) 8:1–20. doi: 10.3389/fimmu.2017.00081
5. Papayannopoulos V. Neutrophil extracellular traps in immunity and disease. Nat Rev Immunol. (2018) 18:134–47. doi: 10.1038/nri.2017.105
6. Yang H, Biermann MH, Brauner JM, Liu Y, Zhao Y, Herrmann M. New insights into neutrophil extracellular traps: mechanisms of formation and role in inflammation. Front Immunol. (2016) 7:302. doi: 10.3389/fimmu.2016.00302
7. Trivedi A, Khan MA, Bade G, Talwar A. Orchestration of neutrophil extracellular traps (nets), a unique innate immune function during chronic obstructive pulmonary disease (COPD) development. Biomedicines. (2021) 9:1–25. doi: 10.3390/biomedicines9010053
8. Porto BN, Stein RT. Neutrophil extracellular traps in pulmonary diseases: too much of a good thing? Front Immunol. (2016) 7:311. doi: 10.3389/fimmu.2016.00311
9. Saffarzadeh M, Juenemann C, Queisser MA, Lochnit G, Barreto G, Galuska SP, et al. Neutrophil extracellular traps directly induce epithelial and endothelial cell death: a predominant role of histones. PLoS ONE. (2012) 7:e32336. doi: 10.1371/journal.pone.0032366
10. Uberti B, Morán G. Role of neutrophils in equine asthma. Anim Heal Res Rev. (2018) 19:65–73. doi: 10.1017/S146625231800004X
11. Côté O, Clark ME, Viel L, Labbé G, Seah SYK, Khan MA, et al. Secretoglobin 1A1 and 1A1A differentially regulate neutrophil reactive oxygen species production, phagocytosis and extracellular trap formation. PLoS One. (2014) 9:e96217. doi: 10.1371/journal.pone.0096217
12. Vargas A, Boivin R, Cano P, Murcia Y, Bazin I, Lavoie JP. Neutrophil extracellular traps are downregulated by glucocorticosteroids in lungs in an equine model of asthma. Respir Res. (2017) 18:1–11. doi: 10.1186/s12931-017-0689-4
13. Sarmiento J, Perez B, Morales N, Henriquez C, Vidal L, Folch H, et al. Apoptotic effects of tamoxifen on leukocytes from horse peripheral blood and bronchoalveolar lavage fluid. Vet Res Commun. (2013) 37:333–8. doi: 10.1007/s11259-013-9571-0
14. Perez B, Henriquez C, Sarmiento J, Morales N, Folch H, Galesio JS, et al. Tamoxifen as a new therapeutic tool for neutrophilic lung inflammation. Respirology. (2016) 21:112–8. doi: 10.1111/resp.12664
15. Albornoz A, Morales N, Uberti B, Henriquez C, Burgos RA, Alarcon P, et al. Tamoxifen and its metabolites induce mitochondrial membrane depolarization and caspase-3 activation in equine neutrophils. Vet Med Sci. (2020) 6:673–8. doi: 10.1002/vms3.316
16. Borlone C, Morales N, Henriquez C, Folch H, Olave C, Sarmiento J, et al. In vitro effects of tamoxifen on equine neutrophils. Res Vet Sci. (2017) 110:60–4. doi: 10.1016/j.rvsc.2016.11.003
17. Morales N, Henriquez C, Sarmiento J, Uberti B, Moran G. Tamoxifen inhibits chemokinesis in equine neutrophils. Ir Vet J. (2018) 71:22. doi: 10.1186/s13620-018-0133-1
18. Olave C, Morales N, Uberti B, Henriquez C, Sarmiento J, Ortloff A, et al. Tamoxifen induces apoptotic neutrophil efferocytosis in horses. Vet Res Commun. (2018) 42:57–63. doi: 10.1007/s11259-017-9709-6
19. Olave C, Alvarez P, Uberti B, Morales N, Henriquez C, Folch H, et al. Tamoxifen induces apoptosis and inhibits respiratory burst in equine neutrophils independently of estrogen receptors. J Vet Pharmacol Ther. (2019) 42:248–54. doi: 10.1111/jvp.12728
20. Mainguy-Seers S, Picotte K, Lavoie JP. Efficacy of tamoxifen for the treatment of severe equine asthma. J Vet Intern Med. (2018) 32:1748–53. doi: 10.1111/jvim.15289
21. Gajardo G, López-Muñoz R, Plaza A, Uberti B, Sarmiento J, Morán G, et al. Tamoxifen in horses: pharmacokinetics and safety study. Ir Vet J. (2019) 72:5. doi: 10.1186/s13620-019-0143-7
22. Manda A, Pruchniak MP, Arazna M, Demkow UA. Neutrophil extracellular traps in physiology and pathology. Cent Eur J of Immunology. (2014) 39:116–21. doi: 10.5114/ceji.2014.42136
23. Rebernick R, Fahmy L, Glover C, Bawadekar M, Shim D, Holmes CL, et al. Area and NETosis analysis (DANA): a high-throughput method to quantify neutrophil extracellular traps in fluorescent microscope images. Biol Proced Online. (2018) 20:1–9. doi: 10.1186/s12575-018-0072-y
24. Muñoz-Caro T, Rubio R MC, Silva LMR, Magdowski G, Gärtner U, McNeilly TN, et al. Leucocyte-derived extracellular trap formation significantly contributes to Haemonchus contortus larval entrapment. Parasites and Vectors. (2015) 8:607. doi: 10.1186/s13071-015-1219-1
25. Lange MK, Penagos-Tabares F, Muñoz-Caro T, Gärtner U, Mejer H, Schaper R, et al. Gastropod-derived haemocyte extracellular traps entrap metastrongyloid larval stages of Angiostrongylus vasorum, Aelurostrongylus abstrusus and Troglostrongylus brevior. Parasites and Vectors. (2017) 10:1–12. doi: 10.1186/s13071-016-1961-z
26. Muñoz-Caro T, Conejeros I, Zhou E, Pikhovych A, Gärtner U, Hermosilla C, et al. Dirofilaria immitis microfilariae and third-stage larvae induce canine NETosis resulting in different types of neutrophil extracellular traps. Front Immunol. (2018) 9:968. doi: 10.3389/fimmu.2018.00968
27. Corriden R, Hollands A, Olson J, Derieux J, Lopez J, Chang JT, et al. Tamoxifen augments the innate immune function of neutrophils through modulation of intracellular ceramide. Nat Commun. (2015) 6:8369. doi: 10.1038/ncomms9369
28. Chen F, Yu M, Zhong Y, Wang L, Huang H. Characteristics and role of neutrophil extracellular traps in asthma. Inflamm. (2021) 45:6–13. doi: 10.1007/s10753-021-01526-8
29. Narasaraju T, Yang E, Samy RP, Ng HH, Poh WP, Liew AA, et al. Excessive neutrophils and neutrophil extracellular traps contribute to acute lung injury of influenza pneumonitis. Am J Pathol. (2011) 179:199. doi: 10.1016/j.ajpath.2011.03.013
30. Twaddell SH, Baines KJ, Grainge C, Gibson PG. The emerging role of neutrophil extracellular traps in respiratory disease. Chest. (2019) 156:774–82. doi: 10.1016/j.chest.2019.06.012
31. Lachowicz-Scroggins ME, Dunican EM, Charbit AR, Raymond W, Looney MR, Peters MC, et al. Extracellular DNA, neutrophil extracellular traps, and inflammasome activation in severe asthma. Am J Respir Crit Care Med. (2019) 199:1076–85. doi: 10.1164/rccm.201810-1869OC
32. Pham DL, Ban GY, Kim SH, Shin YS, Ye YM, Chwae YJ, et al. Neutrophil autophagy and extracellular DNA traps contribute to airway inflammation in severe asthma. Clin Exp Allergy. (2017) 47:57–70. doi: 10.1111/cea.12859
33. Fu Z, Akula S, Thorpe M, Hellman L. Potent and broad but not unselective cleavage of cytokines and chemokines by human neutrophil elastase and proteinase 3. Int J Mol Sci. (2020) 21:651. doi: 10.3390/ijms21020651
34. Janssen P, Tosi I, Hego A, Maréchal P, Marichal T, Radermecker C. Neutrophil extracellular traps are found in bronchoalveolar lavage fluids of horses with severe asthma and correlate with asthma severity. Front Immunol. (2022) 13:3629. doi: 10.3389/fimmu.2022.921077
35. Schauer C, Janko C, Munoz LE, Zhao Y, Kienhöfer D, Frey B, et al. Aggregated neutrophil extracellular traps limit inflammation by degrading cytokines and chemokines. Nat Med. (2014) 20:511–7. doi: 10.1038/nm.3547
36. Hahn J, Schauer C, Czegley C, Kling L, Petru L, Schmid B, et al. Aggregated neutrophil extracellular traps resolve inflammation by proteolysis of cytokines and chemokines and protection from antiproteases. FASEB J. (2019) 33:1401–14. doi: 10.1096/fj.201800752R
37. Mahajan A, Grüneboom A, Petru L, Podolska MJ, Kling L, Maueröder C, et al. Frontline science: aggregated neutrophil extracellular traps prevent inflammation on the neutrophil-rich ocular surface. J Leukoc Biol. (2019) 105:1087–98. doi: 10.1002/JLB.HI0718-249RR
38. Reber LL, Gaudenzio N, Starkl P, Galli SJ. Neutrophils are not required for resolution of acute gouty arthritis in mice. Nat Med. (2016) 22:1382–4. doi: 10.1038/nm.4216
39. Lim JS, Frenkel K, Troll W. Tamoxifen suppresses tumor promoter-induced hydrogen peroxide formation by human neutrophils. Cancer Res. (1992) 52:4969–72.
40. Ahluwalia J. Tamoxifen does not inhibit the swell activated chloride channel in human neutrophils during the respiratory burst. Biochem Biophys Res Commun. (2008) 375:596–601. doi: 10.1016/j.bbrc.2008.08.067
41. Zmijewski JW, Lorne E, Banerjee S, Abraham E. Participation of mitochondrial respiratory complex III in neutrophil activation and lung injury. Am J Physiol. (2009) 296:L624–34. doi: 10.1152/ajplung.90522.2008
42. Dröge W. Free radicals in the physiological control of cell function. Physiol Rev. (2002) 82:47–95. doi: 10.1152/physrev.00018.2001
43. Vorobjeva N V, Chernyak B V. NETosis: molecular mechanisms, role in physiology and pathology. Biochemistry. (2020) 85:1178–90. doi: 10.1134/S0006297920100065
44. Pinegin B, Vorobjeva N, Pashenkov M, Chernyak B. The role of mitochondrial ROS in antibacterial immunity. J Cell Physiol. (2018) 233:3745–54. doi: 10.1002/jcp.26117
45. Vorobjeva N, Prikhodko A, Galkin I, Pletjushkina O, Zinovkin R. Sud'ina G, Chernyak B, Pinegin B. Mitochondrial reactive oxygen species are involved in chemoattractant-induced oxidative burst and degranulation of human neutrophils in vitro. Eur J Cell Biol. (2017) 96:254–65. doi: 10.1016/j.ejcb.2017.03.003
46. Vorobjeva N, Galkin I, Pletjushkina O, Golyshev S, Zinovkin R, Prikhodko A, Pinegin V, Kondratenko I, Pinegin B, Chernyak B. Mitochondrial permeability transition pore is involved in oxidative burst and NETosis of human neutrophils. Biochim Biophys Acta Mol basis Dis. (2020) 1866:165664. doi: 10.1016/j.bbadis.2020.165664
47. Heinz S, Freyberger A, Lawrenz B, Schladt L, Schmuck G, Ellinger-Ziegelbauer H. Mechanistic investigations of the mitochondrial complex i inhibitor rotenone in the context of pharmacological and safety evaluation. Sci Reports. (2017) 7:1–13. doi: 10.1038/srep45465
Keywords: Neutrophil extracellular traps, tamoxifen, horses, sprNETs, aggNETs
Citation: Salinas C, Barriga K, Albornoz A, Alarcon P, Quiroga J, Uberti B, Sarmiento J, Henriquez C, Ehrenfeld P, Burgos RA and Moran G (2023) Tamoxifen triggers the in vitro release of neutrophil extracellular traps in healthy horses. Front. Vet. Sci. 9:1025249. doi: 10.3389/fvets.2022.1025249
Received: 22 August 2022; Accepted: 08 December 2022;
Published: 06 January 2023.
Edited by:
Benito Soto-Blanco, Federal University of Minas Gerais, BrazilReviewed by:
M. Katie Sheats, North Carolina State University, United StatesCopyright © 2023 Salinas, Barriga, Albornoz, Alarcon, Quiroga, Uberti, Sarmiento, Henriquez, Ehrenfeld, Burgos and Moran. This is an open-access article distributed under the terms of the Creative Commons Attribution License (CC BY). The use, distribution or reproduction in other forums is permitted, provided the original author(s) and the copyright owner(s) are credited and that the original publication in this journal is cited, in accordance with accepted academic practice. No use, distribution or reproduction is permitted which does not comply with these terms.
*Correspondence: Gabriel Moran, Z21vcmFuQHVhY2guY2w=
Disclaimer: All claims expressed in this article are solely those of the authors and do not necessarily represent those of their affiliated organizations, or those of the publisher, the editors and the reviewers. Any product that may be evaluated in this article or claim that may be made by its manufacturer is not guaranteed or endorsed by the publisher.
Research integrity at Frontiers
Learn more about the work of our research integrity team to safeguard the quality of each article we publish.