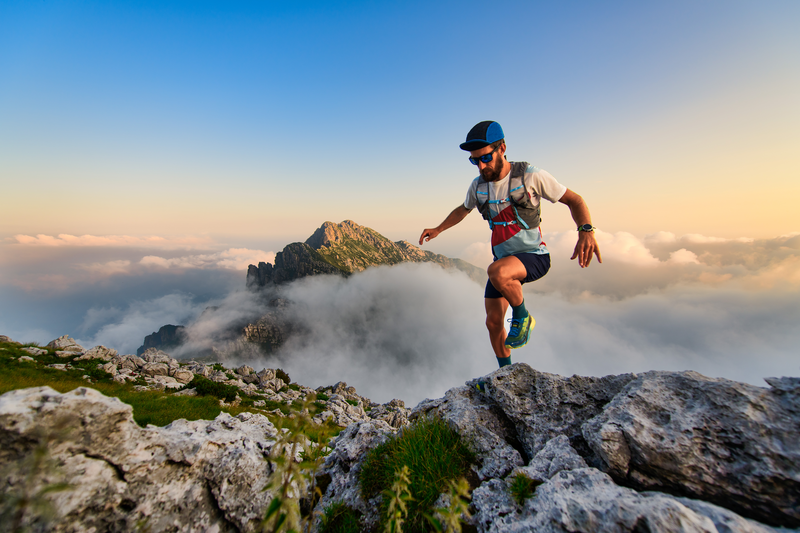
95% of researchers rate our articles as excellent or good
Learn more about the work of our research integrity team to safeguard the quality of each article we publish.
Find out more
ORIGINAL RESEARCH article
Front. Vet. Sci. , 14 October 2022
Sec. Animal Nutrition and Metabolism
Volume 9 - 2022 | https://doi.org/10.3389/fvets.2022.1024430
This experiment was conducted to investigate the effects of Galla Chinensis tannic acid (TA) on growth performance, immune function, and liver health status in broilers. A total of 288 1-day-old Arbor Acres broiler chickens were randomly divided into two groups in a 42-days study. The two groups were a basal diet (CON group) and a basal diet supplemented with 300 mg/kg Galla Chinensis tannic acid (TA group). The results showed that the TA group had significantly decreased feed-to-gain ratio (F/G) throughout the experiment (P < 0.05). The levels of total protein, albumin, low density lipoprotein, high density lipoprotein, urea, total cholesterol, and glucose in the TA group were significantly higher than in the CON group (P < 0.05). In addition, the serum immunoglobulin G, immunoglobulin M, and complements (C3, C4) levels in the TA group were significantly higher than those in the CON group (P < 0.05). Compared with the CON group, the hepatic interleukin-6, interleukin-18, NLRs family pyrin domain containing 3 (NLRP3), caspase-1, and caspase-3 in the TA group were significantly decreased (P < 0.05). Besides, TA group had significantly lower mRNA expression levels of toll-like receptor 4 (TLR4), myeloid differentiation primary response 88 (MyD88), nuclear factor-kappa B (NF-κB), and NLRP3 in liver (P < 0.05). The TA group had significantly higher the mRNA expression levels of Bcl-2 than CON group in liver (P < 0.05). Moreover, TA group tended to decrease Bax/Bcl-2 ratio in liver (P < 0.10). To sum up, dietary supplemented with microencapsulated TA from Galla Chinensis had beneficial effects on growth performance, immune function, and liver health status in broilers. The protective role of TA from Galla Chinensis in liver health of broilers might be related to the inhibition of hepatic apoptosis and pyroptosis via inactivation of TLR4/MyD88/NF-κB signaling pathway.
The liver is not only one of the important metabolic organs, but also performs activities of immune function of the body (1, 2). Liver plays an important role in regulating the production and metabolism of fat, carbohydrate, and protein, and cytokines, complement components, chemokines, and is responsible for the synthesis of cytokines, complement components, chemokines, and acute phase proteins (3, 4). In addition, liver has specific functions in clearing pathogens and exogenous antigens in systemic circulation, detoxify, and phagocytize bacteria (5, 6). Recently, intensive poultry farming significantly boosts livestock productivity and profits, but it also raises the chance of liver damage due to pathogenic bacterium, environmental factors, and poor feed hygiene (7, 8), which adversely affecting the growth performance, liver health, and immunity of broilers. Therefore, liver protection is great significance to ensure the healthy and sustainable development of poultry production.
A huge number of studies illustrated that Chinese herbs extract had anti-inflammatory, antioxidant, and antimicrobial activities (9–11). Supplementation with Chinese herbs extract in broiler diets has been proven to improve growth performance and benefit liver health (11, 12). Galla Chinensis is one of the important Chinese herbs, and its main bioactive component is a hydrolysable tannic acid (TA) called gallotannins that is a glycoside or ester formed by the combination of gallic acid and diacid with glucose (13, 14). It was reported that TA has numerous biological functions such as anti-inflammatory, antioxidant, anti-bacterial, antiviral, and apoptosis regulation (15–18). However, tannins were once thought to be an anti-nutritional feed additive for monogastric animals due to their binding properties with proteins, carbohydrates, minerals, and digestive enzymes, which reduced bioavailability of nutrients in feed (19). Recent studies found that TA supplementation could improve growth performance, promote liver health, and enhance immune function of animals (20, 21), suggesting that TA could be a good feed additive for animal production. However, TA was generally derived from chestnut in majority, and few studies investigated the Galla Chinensis TA as the feed additive in poultry research. Wang, et al. (22) showed that TA from Galla Chinensis addition had beneficial effects on intestinal morphology, intestinal nutrient transporter, and intestinal microbiota in weaning piglets. Besides, our recent study also showed that supplementation of 300 mg/kg TA extracted from Galla Chinensis had beneficial effects on intestinal development and suppressed intestinal mucosal inflammatory responses in broilers (23). When the gut is damaged, it increases the translocation of harmful metabolites to the liver, leading to impaired liver function (24). However, little information is available on the evaluation of the effects of dietary Galla Chinensis TA supplementation on the liver health in broilers.
Therefore, the purpose of this experiment was to investigate the effects of Galla Chinensis TA on growth performance, immune function, and liver health status in chickens, which provided new insights into the use of Galla Chinensis TA as a new feed additive in poultry production.
A total of 288 one-day-old AA broilers with an initial body weight (BW) of 48.05 ± 0.39 g were randomly divided into two treatment groups, each of which included six replicates of 24 broilers. The experiment lasted 42 days. The two experimental groups were as follows: (1) CON group, broilers fed a basal diet; (2) TA group, broilers fed a basal diet supplemented with 300 mg/kg TA (23). The TA production, extracted from Galla Chinensis, was microencapsulated with 40% effective concentrations and provided by the Wufeng Chicheng Biotechnology Co., Ltd (Yichang, China). The basal diets (Table 1) were formulated according to two-phase feeding programs (0–21 d and 21–42 d) recommended by Ministry of Agriculture of China (2004) (25). All broilers had free access to water and feed throughout the trial. Each replicate per group contained 24 broilers and housed in a three-level mental chicken coop placed in an environment-controlled room with continuous light, providing 5 lx of light intensity (26). During the experiment, daily feed intake and BW (on days 21 and 42) per replicate were recorded to calculate average daily feed intake (ADFI), average daily gain (ADG), and feed-to-gain ratio (F/G). The room temperature was maintained at 35°C for the first week and then decreased by 1°C every 2 days until 21°C.
On the morning of day 42, six chickens (one chicken each replicate) were randomly selected from each group. Fasting blood was collected from the wing vein using an evacuated blood collection tube. Then serum was harvested by being centrifuged at 3,500 r/min for 15 min, and stored at −20°C until further analysis. After evisceration, livers were weighed to calculate the ratio of liver weight to BW individually. Besides, 5 g of liver samples were quickly collected from the middle of hepatic lobule. One part of the liver samples was stored at −80°C refrigerator until further analysis after being quick-frozen with liquid nitrogen, and another part was fixed with 4% paraformaldehyde solution for 24 h at room temperature.
After fixation with 4% paraformaldehyde solution for 24 h, liver samples were dehydrated through graded levels of ethyl alcohol (70, 80, 85, 90, 95, 100 I, and 100% II) and embedded in liquid paraffin according to the standard histological procedure (27). Then, paraffin wax embedded liver tissues were cut into 5 μm sections and stained with hematoxylin and eosin (H&E). Finally, liver sections were observed under an Olympus B X 51 microscope (Tokyo, Japan).
After the preserved serum samples were dissolved at 4°C, serum levels of albumin (ALB), total protein (TP), high density lipoprotein (HDL), urea, triglycerides (TG), total cholesterol (TCHO), low density lipoprotein (LDL), glucose (GLU), and alanine aminotransferase (ALT) were determined by using the kits purchased from Nanjing Jiancheng Bioengineering Institute (Nanjing, China) on a COBUS MIRA Plus automatic biochemical analyzer (Roche Diagnostic System Inc, United States).
Serum concentrations of immunoglobulin G (IgG), immunoglobulin A (IgA), immunoglobulin M (IgM), and complements (C3 and C4) were detected with commercial ELISA kits (Jiangsu Meimian Industrial Co., Ltd, Jiangsu, China) on the basis of the procedure previously described (28).
The concentrations of NLRs family pyrin domain containing 3 (NLRP3), tumor necrosis factor-α (TNF-α), interleukin-1β (IL-1β), interleukin-6 (IL-6), interleukin-18 (IL-18), caspase-1, and caspase-3 in liver were measured using a commercial assay kit (R&D Systems Inc., Minneapolis, MN, United States) derived from a previous study (29).
Liver samples were ground in liquid nitrogen and total RNA from the liver samples was extracted using a TRIzon Reagent RNA kit according to the manufacturer's instructions (Accurate Biology, Hunan, China). Equal mass of RNA of each sample was reverse transcribed into cDNA using reverse transcription (RT) kit (Accurate Biology, Hunan, China). The qRT-PCR was performed using SYBR Green Premix Pro Taq HS qPCR Kit (AG11701, Accurate Biology, DaLian, China) and gene specific primers (myeloid differentiation primary response 88, NLRs family pyrin domain containing 3, nuclear factor-kappa B, toll-like Receptor 4, B-cell lymphoma-2, and BCL2-Associated X) according to manufacturer's instruction. Gene expression relative to reference gene β-actin was calculated by 2−ΔΔCT method. Gene specific primers are shown in Table 2.
The replicate was considered the experimental unit for the analysis of growth performance, and individual broiler was considered the experimental unit for all other variables. The t-test procedure of SAS 9.4 software (Institute Inc., Cary, NC, United States) was used to evaluate the significance between the CON group and the TA group after assessing the normality of data. The results were expressed as means ± standard error (SE). Significant differences were indicated by *P < 0.05, **P < 0.01, and ***P < 0.001, while #0.05 ≤ P < 0.1 was indicated as a significant tendency.
As presented in Table 3, from d 21 to 42 and from d 0 to 42, broilers in TA group had significantly lower (P < 0.05) F/G than broilers in CON group, and the ADG in TA group tended to be higher (P < 0.10) than that in CON group. Besides, the BW in TA group tended to be higher (P < 0.10) than that in CON group on d 42, and TA group tended to be higher (P < 0.10) ADFI than CON group from d 0 to 21.
Table 3. Effects of dietary supplemented with microencapsulated TA from Galla Chinensis on growth performance of broiler chickens.
Serum metabolites concentrations of broilers on day 42 were presented in Figure 1. The serum TP, ALB, HDL, UREA, TCHO, LDL, and GLU concentrations in broilers fed TA diet were significantly higher (P < 0.05) than those in broilers fed CON diet. In contrast, the TA group tended to have lower (P < 0.10) ALT activity than the CON group. No significant difference (P > 0.05) was observed in TG concentration between the CON group and the TA group.
Figure 1. Effects of dietary supplemented with microencapsulated TA from Galla Chinensis on serum biochemical parameters of broiler chickens. (A) Total protein (TP), (B) Albumin (ALB), (C) High density lipoprotein (HDL), (D) Low density lipoprotein (LDL), (E) UREA, (F) Total cholesterol (TCHO), (G) Triglycerides (TG), (H) Glucose (GLU), (I) Alanine transaminase (ALT). CON, broiler chickens fed a basal diet; TA, broiler chickens fed a basal diet supplemented with 300 mg/kg microencapsulated TA from Galla Chinensis. Values are mean ± standard error (n = 6). *P < 0.05, **P < 0.01, #0.05 < P < 0.1.
As shown in Figure 2, the serum concentrations of IgG, IgM, C3, and C4 were significantly increased (P < 0.05) in TA group than the CON group. There was no significant difference (P > 0.05) in serum IgA levels between the CON and TA groups.
Figure 2. Effects of dietary supplemented with microencapsulated TA from Galla Chinensis on serum immunoglobulins and complements concentrations of broiler chickens. (A) Immunoglobulin A (IgA), (B) Immunoglobulin G (IgG), (C) Immunoglobulin G (IgG), (D) Complement C3, (E) Complement C4. CON, broiler chickens fed a basal diet; TA, broiler chickens fed a basal diet supplemented with 300 mg/kg microencapsulated TA from Galla Chinensis. Values are mean ± standard error (n = 6). **P < 0.01, ***P < 0.001.
The liver histolomorph and index are shown in Figure 3. Compared with the CON group, the liver cells were structurally complete and arranged in an orderly manner in TA group. Moreover, TA group showed lower degrees of liver inflammatory cell infiltration than CON group. There was no significant difference (P > 0.05) in liver index between the two groups.
Figure 3. Effects of dietary supplemented with microencapsulated TA from Galla Chinensis on liver morphological sections and liver index of broiler chickens. (A) Liver morphological sections, (B) Liver index (g/kg) = liver weight (g)/body weight (kg). CON, broiler chickens fed a basal diet; TA, broiler chickens fed a basal diet supplemented with 300 mg/kg microencapsulated TA from Galla Chinensis. Values are mean ± standard error (n = 6).
Effects of TA on liver inflammatory factors concentrations and hepatic caspases activities of broilers are displayed in Figure 4. Broilers in TA group had significantly lower (P < 0.05) levels of IL-6, IL-18, NLRP3, caspase-1, and caspase-3 in livers than those in CON group. There were no significant differences (P > 0.05) in liver TNF-α and IL-1β concentrations between the two groups.
Figure 4. Effects of dietary supplemented with microencapsulated TA from Galla Chinensis on liver inflammatory factor and hepatic caspases levels of broiler chickens. (A) Tumor necrosis factor-α (TNF-α), (B) Interleukin-1β (IL-1β), (C) Interleukin-6 (IL-6), (D) Interleukin-18 (IL-18), (E) NLRs family pyrin domain containing 3 (NLRP3), (F) caspase-1, (G) caspase-3. CON, broiler chickens fed a basal diet; TA, broiler chickens fed a basal diet supplemented with 300 mg/kg microencapsulated TA from Galla Chinensis. Values are mean ± standard error (n = 6). *P < 0.05.
The mRNA expression levels of inflammatory genes in liver are presented in Figure 5. Compared with the CON group, the mRNA expressions levels of TLR4, MyD88, and NLRP3 were significantly lower (P < 0.05) in the TA group, and the mRNA expression levels of NF-κB tended to decease (P < 0.10) in the TA group.
Figure 5. Effects of dietary supplemented with microencapsulated TA from Galla Chinensis on inflammatory genes in live of broiler chickens. (A) Toll-like Receptor 4 (TLR4), (B) Myeloid differentiation primary response 88 (MyD88), (C) Nuclear factor-kappa B (NF-κB), (D) NLRs family pyrin domain containing 3 (NLRP3). CON, broiler chickens fed a basal diet; TA, broiler chickens fed a basal diet supplemented with 300 mg/kg microencapsulated TA from Galla Chinensis. Values are mean ± standard error (n = 6). *P < 0.05, #0.05 < P < 0.1.
The mRNA expression levels of apoptosis regulators are shown in Figure 6. The mRNA expression level of Bcl-2 in liver was significantly lower (P < 0.05) in the TA group than in the CON group, and the Bax/Bcl-2 ratio in the TA group tended to be decreased (P < 0.10) than that in the CON group. No significant difference was observed (P > 0.05) in the mRNA expression levels of Bax in liver between the CON group and the TA group.
Figure 6. Effects of dietary supplemented with microencapsulated TA from Galla Chinensis on apoptosis-related genes in live of broiler chickens. (A) BCL2-Associated X (Bax), (B) B-cell lymphoma-2 (Bcl-2), (C) Bax/Bcl-2 ratio. CON, broiler chickens fed a basal diet; TA, broiler chickens fed a basal diet supplemented with 300 mg/kg microencapsulated TA from Galla Chinensis. Values are mean ± standard error (n = 6). *P < 0.05, #0.05 < P < 0.1.
In the present study, we found that dietary supplemented with 300 mg/kg microencapsulated TA from Galla Chinensis increased final BW and decreased the F/G in broilers throughout the trial. Similarly, Masek, et al. (30) showed that dietary supplemented with 5 g/kg TA increased final BW and ADG, and decreased the F/G of broilers. It was also reported that supplementing 500 mg/kg TA alone increased F/G in coccidiosis-vaccinated broilers (31). Another study in piglets showed that dietary Galla Chinensis TA supplementation benefited to the growth performance (32). However, Ebrahim, et al. (33) showed that 1% TA in the diet reduced feed intake of broiler chicken, which might be related to the poor palatability of TA. Previous study indicated that microencapsulation was a potential scheme to improve the palatability in animal feed, and could avoid the undesirable irritation of TA (22). Above all, the results suggested that dietary supplemented with 300 mg/kg microencapsulated TA from Galla Chinensis had the potential to improve the growth performance of broilers.
Immune function is an important factor in improving growth performance of broilers (11). The results of this study showed that TA increased serum concentrations of IgG, IgM, C3, and C4. Immunoglobulin is the one of the main anti-infective components of blood (34). As one of the hallmarks of adaptive immunity, immunoglobulins serve as the first line of host defense against infections, and play a vital role in immune regulation and immunological tolerance (35). However, Marzo, et al. (36) demonstrated that adding 25 or 30 g/kg TA to the diet reduced total IgM and IgA levels in blood, which might be due to the high tannin contentions in the diet. Besides, the complement system is an ancient and evolutionary conserved element of the innate immune mechanism, and plays a crucial role in tissue homeostasis and pathogen immunosurveillance (37). The increased immunoglobulin concentrations could also stimulate the increase of complement concentrations and produce a specific immune mechanism (38). In addition, liver is one of the main sites of complement synthesis, serum complement C3 will decrease correspondingly when the liver lesions occur (39). The increased complement C3 and C4 in TA group also suggested that TA benefited to liver protection. To sum up, our study indicated that 300 mg/kg microencapsulated Galla Chinensis TA supplementation enhanced the immune function in liver of broilers.
Serum metabolites could indirectly reflect the physiological, health status, and material metabolism status of animals (40). Our current study showed that Galla Chinensis TA supplementation increased serum TP, ALB, HDL, LDL, UREA, TCHO, and GLU, and decreased serum ALT activity. The concentrations of TP and ALB in serum reflect the metabolism and nutritional status of protein, and serum TP and ALB are the raw materials of immunoglobulin synthesis (41). Previous study in buffalo showed that dietary TA increased the serum levels of TP and ALB, but did not impact serum concentrations of TCHO and UREA (42). These results indicated that TA could promote protein synthesis, which was beneficial to the growth and immunity of broilers. Previous studies showed that LDL promotes fat deposition, while HDL reduced fat deposition and transfers fat around the liver (43). Yugaran, et al. (44) found that feeding TA increased HDL in serum of rats. The levels of UREA and GLU reflect the metabolic ability of the body (45). Liu, et al. (11) found that the vigorous metabolism of broilers may lead to the increase of serum metabolites of broilers. Therefore, in our study, the increase in serum metabolite concentrations of broilers in TA group may be due to the improvement of growth performance and metabolism of broilers by Galla Chinensis TA. Alanine transaminase is a major hepatic transaminase, and ALT will be released into the bloodstream when the liver is damaged (46). Chu, et al. (47) found that TA could reduce the concentrations of ALT in serum of rat carbon tetrachloride-induced liver injury. Based on this, dietary 300 mg/kg microencapsulated TA from Galla Chinensis supplementation benefited liver health of broilers.
In order to clarify the underlying mechanism of TA protecting liver, inflammatory factors levels and TLR4/MyD88/NF-κB signaling pathway were assessed in this study. We found that dietary Galla Chinensis TA supplementation reduced the levels of IL-6, IL-18, and NLRP3 in liver of broilers. The proinflammatory cytokines IL-6 and IL-18 have critical roles in establishment of inflammation, and involved in the induction of liver diseases (48, 49). Yin, et al. (50) also found that diets supplemented with 500 mg/kg TA decreased serum IL-6 contention in broilers. The inflammasome NLRP3 plays a key role in a variety of disease processes (51). Studies had shown that NLRP3 could activate caspase-1, leading to the maturation of IL-1β and IL-18 and cell necrosis (52, 53). Sun, et al. (54) found that Galla Chinensis could significantly reduce the levels of proinflammatory cytokines. These results showed that supplemented with Galla Chinensis TA reduced the secretions of inflammatory cytokines in liver of broilers. Besides, the TLR4/MyD88/NF-κB signal mainly mediates the inflammatory response, and is a signal transduction pathway regulating liver diseases (55). In this study, we found that the mRNA expression levels of TLR4, MyD88, NF-κB, and NLRP3 were lower in the TA group than in the CON group. The NF-κB is a key transcription factor mediating inflammatory response and is a prerequisite for the activation of NLRP3 inflammasome (56–58). Studies have shown that TLR4 is a transmembrane protein and belongs to the pattern recognition receptor family, and can also participate in the conduction of inflammatory signals, leading to the release of inflammatory factors such as IL-6, IL-1β, and TNF-α (59, 60). Besides, MyD88, a key adaptor protein of TLR4, and can activate NF-κB and stimulate the secretion of proinflammatory cytokines (61). Previous studies indicated that inhibiting the TLR4/MyD88/NF-κB signaling pathway benefited to attenuate hepatic inflammatory injury (62). Many researches also revealed that TA could inhibit the expression of TLR4 and NF-κB to suppress the inflammatory response in inflammatory cells (63, 64). Therefore, our results suggested that the addition of 300 mg/kg Galla Chinensis TA could inhibit hepatic inflammatory response through TLR4/MyD88/NF-κB signaling pathway.
Hepatocyte cell death, as well as liver inflammation, had been well–recognized as central characteristics of liver disease (65). In present study, dietary 300 mg/kg TA supplementation was found to decrease the activities of caspase-1 and caspase-3 and Bax/Bcl-2 ratio, and increase the mRNA expression levels of Bcl-2 in liver of broiler. Caspases are a family of endoproteases, and activation of caspases ultimately results in programmed execution of cell death including cell apoptosis and pyroptosis (66). Caspase 1 and caspase 3 have been shown to be involved extensively in the apoptotic process, caspase-1 known as IL-1β-converting enzyme can be activated by various inflammasomes such as NLRP3, resulting in pyroptosis of damaged cells (67). Besides, mature caspase-1 effector molecules precaspase-1 can induce IL-1β and IL-18 maturation, leading to cell necrosis (68). Caspase-3, onto which there is a convergence of the extrinsic and intrinsic apoptotic pathways, is the main executioner of apoptosis (69). Activation of caspase-3 was reported to mediate proteasome inhibitor-induced apoptosis (70). Anti-apoptotic Bcl-2 could inhibit apoptosis induced by a variety of factors and play an important role in regulating the apoptotic response (71). The Bax/Bcl-2 ratio has been shown to serve as a check point for the death, and a high Bax/Bcl-2 ratio was confirmed to increase activation of caspases including caspase-1 and caspase-3 (24, 72). Ji, et al. (73) found that Galla Chinensis increased Bcl-2 protein expression and decreased epithelial cell damage in patients with pneumonia. Previous studies had shown that TA reduced mitosis and apoptosis in the caecum and descending colon of piglets (74). Inflammation is a potent inducer of cell death through increasing the Bax/Bcl-2 ratio and activating caspases (75). Above all, it demonstrated that the addition of 300 mg/kg microencapsulated Galla Chinensis TA could decrease hepatic apoptosis and pyroptosis partially via inhibiting inflammation.
In conclusion, dietary supplemented with 300 mg/kg microencapsulated Galla Chinensis TA had beneficial effects on growth performance, immune function, and liver health status in broilers. The protective role of TA in liver health of broilers might be related to the inhibition of hepatic apoptosis and pyroptosis via inactivation of TLR4/MyD88/NF-κB signaling pathway. These findings provided new insights into the use of microencapsulated TA from Galla Chinensis as a new feed additive in poultry production.
The original contributions presented in the study are included in the article/supplementary material, further inquiries can be directed to the corresponding authors.
The animal study was reviewed and approved by the Protocol for this Experiment Involving Animals was in Accordance with the Guidelines of the Care and Use Committee of Shandong Agricultural University (Ethics Approval code: SDAUA-2021-019).
HL and YLi: conceptualization. JN and YLiu: data curation. JN, QW, CJ, and WY: formal analysis. NJ and LH: funding acquisition. CJ and QG: investigation. CJ, HL, and YLi: methodology. JN, QW, YLiu, and YLi: project administration. JN, QW, and HL: software. YLi and WY: supervision. SJ and YLi: validation. JN: writing—original draft. QW, YLi, and WY: writing—review and editing. All authors contributed to the article and approved the submitted version.
This research was funded by the Shandong Province Pig Industry Technology System (SDAIT-08-04 and SDAIT-08-05) and Postdoctoral Science Foundation of Shandong Agricultural University, grant number 040/76598.
Author QW is employed by Shandong Wonong Agro-tech Group Co., Ltd. Author QG is employed by Shandong Landoff Biotechnology Co., Ltd.
The remaining authors declare that the research was conducted in the absence of any commercial or financial relationships that could be construed as a potential conflict of interest.
All claims expressed in this article are solely those of the authors and do not necessarily represent those of their affiliated organizations, or those of the publisher, the editors and the reviewers. Any product that may be evaluated in this article, or claim that may be made by its manufacturer, is not guaranteed or endorsed by the publisher.
1. Zaefarian F, Abdollahi MR, Cowieson A, Ravindran V. Avian liver: the forgotten organ. Animals. (2019) 9:63. doi: 10.3390/ani9020063
2. Nemeth E, Baird AW, O'Farrelly C. Microanatomy of the liver immune system. Semin Immunopathol. (2009) 31:333–43. doi: 10.1007/s00281-009-0173-4
3. Hijmans BS, Grefhorst A, Oosterveer MH, Groen AK. Zonation of glucose and fatty acid metabolism in the liver: mechanism and metabolic consequences. Biochimie. (2014) 96:121–9. doi: 10.1016/j.biochi.2013.06.007
4. Huang XY, Ansari AR, Huang HB, Zhao X, Li NY, Sun ZJ, et al. Lipopolysaccharide mediates immuno-pathological alterations in young chicken liver through TLR4 signaling. BMC Immunol. (2017) 18:1–9. doi: 10.1186/s12865-017-0199-7
5. Robinson MW, Harmon C, O'Farrelly C. Liver immunology and its role in inflammation and homeostasis. Cell Mol Immunol. (2016) 13:267–76. doi: 10.1038/cmi.2016.3
6. Bogdanos DP, Gao B, Gershwin ME. Liver immunology. Compr Physiol. (2013) 3:567. doi: 10.1002/cphy.c120011
7. Han C, Wei Y, Wang X, Ba C, Shi W. Protective effect of salvia miltiorrhiza polysaccharides on liver injury in chickens. Poultry Sci. (2019) 98:3496–503. doi: 10.3382/ps/pez153
8. Porowski D, Wirkowska A, Hryniewiecka E, Wyzgał J, Pacholczyk M, Paczek L. Liver failure impairs the intrahepatic elimination of interleukin-6, tumor necrosis factor-alpha, hepatocyte growth factor, and transforming growth factor-beta. BioMed Res Int. (2015) 2015:934065. doi: 10.1155/2015/934065
9. Chen J, Luo Y, Li Y, Chen D, Yu B, He J. Chlorogenic acid attenuates oxidative stress-induced intestinal epithelium injury by co-regulating the PI3K/Akt and IκBα/NF-κB signaling. Antioxidants. (2021) 10:1915. doi: 10.3390/antiox10121915
10. Chen J, Yu B, Chen D, Zheng P, Luo Y, Huang Z, et al. Changes of porcine gut microbiota in response to dietary chlorogenic acid supplementation. Appl Microbiol Biot. (2019) 103:8157–68. doi: 10.1007/s00253-019-10025-8
11. Liu Y, Li Y, Niu J, Hua L, Jiao N, Huang L, et al. Effects of dietary Macleaya Cordata extract containing isoquinoline alkaloids supplementation as an alternative to antibiotics in the diets on growth performance and liver health of broiler chickens. Front Veteri Sci. (2022) 1024. doi: 10.3389/fvets.2022.950174
12. Chen Y, Chen Y, Zhang H, Wang T. Pterostilbene as a protective antioxidant attenuates diquat-induced liver injury and oxidative stress in 21-day-old broiler chickens. Poultry Sci. (2020) 99:3158–67. doi: 10.1016/j.psj.2020.01.021
13. Lattanzio V, Cardinali A, Linsalata V. Plant phenolics: a biochemical and physiological perspective. Recent Adv Polyphenol Res. (2012) 3:1–393. doi: 10.1002/9781118299753.ch1
14. Mueller-Harvey I. Analysis of hydrolysable tannins. Anim Feed Sci Tech. (2001) 91:3–20. doi: 10.1016/S0377-8401(01)00227-9
15. Montanari E, Gennari A, Pelliccia M, Gourmel C, Lallana E, Matricardi P, et al. Hyaluronan/tannic acid nanoparticles via catechol/boronate complexation as a smart antibacterial system. Macromol Biosci. (2016) 16:1815–23. doi: 10.1002/mabi.201600311
16. Gulçin I, Huyut Z, Elmastaş M, Aboul-Enein HY. Radical scavenging and antioxidant activity of tannic acid. Arab J Chem. (2010) 3:43–53. doi: 10.1016/j.arabjc.2009.12.008
17. Xie Q, Li JY, Zuo YL, Zhou XD. The effect of galla chinensis on the growth of cariogenic bacteria in vitro. West China J Stomatol. (2005) 23:82–4.
18. Biagi G, Cipollini I, Paulicks BR, Roth FX. Effect of tannins on growth performance and intestinal ecosystem in weaned piglets. Arch Anim Nutr. (2010) 64:121–35. doi: 10.1080/17450390903461584
19. Chung KT, Wong TY, Wei CI, Huang YW, Lin Y. Tannins and human health: a review. Crit Rev Food Sci. (1998) 38:421–64. doi: 10.1080/10408699891274273
20. Brus M, Dolinsek J, Cencic A, Skorjanc D. Effect of chestnut (Castanea sativa Mill) wood tannins and organic acids on growth performance and faecal microbiota of pigs from 23 to 127 days of age. Bul J Agricult Sci. (2013) 19:841–7.
21. Go J, Kim JE, Koh EK, Song SH, Sung JE, Lee HA, et al. Protective effect of gallotannin-enriched extract isolated from Galla Rhois against CCl4-induced hepatotoxicity in ICR mice. Nutrients. (2016) 8:107. doi: 10.3390/nu8030107
22. Wang M, Huang H, Hu Y, Huang J, Yang H, Wang L, et al. Effects of dietary microencapsulated tannic acid supplementation on the growth performance, intestinal morphology, and intestinal microbiota in weaning piglets. J Anim Sci. (2020) 98:skaa112. doi: 10.1093/jas/skaa112
23. Jing C, Niu J, Liu Y, Jiao N, Huang L, Jiang S, et al. Tannic acid extracted from Galla Chinensis supplementation in the diet improves intestinal development through suppressing inflammatory responses via blockage of NF-κB in broiler chickens. Animals. (2022) 12:2397. doi: 10.3390/ani12182397
24. Li Y, Zhao X, Jiang X, Chen L, Hong L, Zhuo Y, et al. Effects of dietary supplementation with exogenous catalase on growth performance, oxidative stress, and hepatic apoptosis in weaned piglets challenged with lipopolysaccharide. J Anim Sci. (2020) 98:skaa067. doi: 10.1093/jas/skaa067
25. Chinese Ministry of Agriculture. Feeding Standard of Chicken. China (NY/T 33–2004). Hunan Feed. (2004) 4:19–27.
26. Blatchford RA, Klasing KC, Shivaprasad HL, Wakenell PS, Archer GS, Mench JA, et al. The effect of light intensity on the behavior, eye and leg health, and immune function of broiler chickens. Poultry Sci. (2009) 88:20–8. doi: 10.3382/ps.2008-00177
27. Zhang P, Jing C, Liang M, Jiang S, Huang L, Jiao N, et al. Zearalenone exposure triggered cecal physical barrier injury through the TGF-β1/smads signaling pathway in weaned piglets. Toxins. (2021) 13:902. doi: 10.3390/toxins13120902
28. Chen J, Li F, Yang W, Jiang S, Li Y. Comparison of gut microbiota and metabolic status of sows with different litter sizes during pregnancy. Front Veteri Sci. (2021) 8. doi: 10.3389/fvets.2021.793174
29. Chen J, Li F, Yang W, Jiang S, Li Y. Supplementation with exogenous catalase from penicillium notatum in the diet ameliorates lipopolysaccharide-induced intestinal oxidative damage through affecting intestinal antioxidant capacity and microbiota in weaned pigs. Microbiol Spect. (2021) 9:e00654–21. doi: 10.1128/Spectrum.00654-21
30. Masek T, Starcevic K, Mikulec Z. The influence of the addition of thymol, tannic acid or gallic acid to broiler diet on growth performance, serum malondialdehyde value and cecal fermentation. Yeast. (2014) 5. doi: 10.1399/eps.2014.64
31. Tonda RM, Rubach JK, Lumpkins BS, Mathis GF, Poss MJ. Effects of tannic acid extract on performance and intestinal health of broiler chickens following coccidiosis vaccination and/or a mixed-species Eimeria challenge. Poultry Sci. (2018) 97:3031–42. doi: 10.3382/ps/pey158
32. Cha CN Yu EA, Park EK, Kim S, Lee HJ. Effects of dietary supplementation with Galla Rhois on growth performance and diarrhea incidence in postweaning piglets. J Veteri Clin. (2013) 30:353–8.
33. Ebrahimi R, Liang JB, Jahromi MF, Shokryazdan P, Ebrahimi M. Li Chen, et al. Effects of tannic acid on performance and fatty acid composition of breast muscle in broiler chickens under heat stress. Italian J Anim Sci. (2015) 14:3956. doi: 10.4081/ijas.2015.3956
34. Balan P, Sik-Han K, Moughan PJ. Impact of oral immunoglobulins on animal health—A review. Anim Sci J. (2019) 90:1099–110. doi: 10.1111/asj.13258
35. Sun Y, Huang T. Hammarstrom, L, Zhao Y. The immunoglobulins: new insights, implications, and applications. Annu Rev Animal Biosci. (2020) 8:145–69. doi: 10.1146/annurev-animal-021419-083720
36. Marzo F, Tosar A, Santidrian S. Effect of tannic acid on the immune response of growing chickens. J Anim Sci. (1990) 68:3306–12. doi: 10.2527/1990.68103306x
37. Vignesh P, Rawat A, Sharma M, Singh S. Complement in autoimmune diseases. Clin Chim Acta. (2017) 465:123–30. doi: 10.1016/j.cca.2016.12.017
38. Sakai DK. Repertoire of complement in immunological defense mechanisms of fish. Annu Rev Fish Dis. (1992) 2:223–47. doi: 10.1016/0959-8030(92)90065-6
39. Long LN, Kang BJ. JiangQ, Chen JS. Effects of dietary Lycium barbarum polysaccharides on growth performance, digestive enzyme activities, antioxidant status, and immunity of broiler chickens. Poultry Sci. (2020) 99:744–51. doi: 10.1016/j.psj.2019.10.043
40. Geng AL, Zhang Y, Zhang J, Zeng LC, Chang C, Wang HH, et al. Effects of light regime on the hatching performance, body development and serum biochemical indexes in Beijing You Chicken. Poultry Sci. (2021) 100:101270. doi: 10.1016/j.psj.2021.101270
41. Banales JM, Inarrairaegui M, Arbelaiz A, Milkiewicz P, Muntane J, Munoz-Bellvis L, et al. Serum metabolites as diagnostic biomarkers for cholangiocarcinoma, hepatocellular carcinoma, and primary sclerosing cholangitis. Hepatology. (2019) 70:547–62. doi: 10.1002/hep.30319
42. Lakhani N, Kamra DN, Lakhani P, Alhussien MN. Immune status and haemato-biochemical profile of buffalo calves supplemented with phytogenic feed additives rich in tannins, saponins and essential oils. Trop Anim Health Pro. (2019) 51:565–73. doi: 10.1007/s11250-018-1727-z
43. Kwiterovich PO. The metabolic pathways of high-density lipoprotein, low-density lipoprotein, and triglycerides: a current review. Am J Cardiol. (2000) 86:5–10. doi: 10.1016/S0002-9149(00)01461-2
44. Yugarani T, Tan BKH, Das NP. The effects of tannic acid on serum lipid parameters and tissue lipid peroxides in the spontaneously hypertensive and Wistar Kyoto rats. Planta Med. (1993) 59:28–31. doi: 10.1055/s-2006-959598
45. Almdal TP, Vilstrup H. Strict insulin therapy normalises organ nitrogen contents and the capacity of urea nitrogen synthesis in experimental diabetes in rats. Diabetologia. (1988) 31:114–8. doi: 10.1007/BF00395558
46. Sohail MU, Rahman ZU, Ijaz A, Yousaf MS, Ashraf K, Yaqub T, et al. Single or combined effects of mannan-oligosaccharides and probiotic supplements on the total oxidants, total antioxidants, enzymatic antioxidants, liver enzymes, and serum trace minerals in cyclic heat-stressed broilers. Poultry Sci. (2011) 90:2573–7. doi: 10.3382/ps.2011-01502
47. Chu X, Wang H, Jiang YM, Zhang YY, Bao YF, Zhang X, et al. Ameliorative effects of tannic acid on carbon tetrachloride-induced liver fibrosis in vivo and in vitro. J Pharmacol Sci. (2016) 130:15–23. doi: 10.1016/j.jphs.2015.12.002
48. Wallenius V, Wallenius K, Hisaoka M, Sandstedt J, Ohlsson C, Kopf M, et al. Retarded liver growth in interleukin-6-deficient and tumor necrosis factor receptor-1-deficient mice. Endocrinology. (2001) 142:2953–60. doi: 10.1210/endo.142.7.8270
49. Liu X, Pan Z, Su D, Yang Z, Zheng B, Wang X, et al. Remifentanil ameliorates liver ischemia-reperfusion injury through inhibition of interleukin-18 signaling. Transplantation. (2015) 99:2109–17. doi: 10.1097/TP.0000000000000737
50. Yin X, Ding P, Xiao J, Yang Y, Song Z, He X, et al. Effects of Galla Chinensis extract on growth performance, carcass traits, serum antioxidation, immune function, and gut microbiota of broilers. Front Anim Sci. (2022) 38. doi: 10.3389/fanim.2022.880237
51. Swanson KV, Deng M, Ting JPY. The NLRP3 inflammasome: molecular activation and regulation to therapeutics. Nat Rev Immunol. (2019) 19:477–89. doi: 10.1038/s41577-019-0165-0
52. He Y, Hara H, Nunez G. Mechanism and regulation of NLRP3 inflammasome activation. Trends Biochem Sci. (2016) 41:1012–21. doi: 10.1016/j.tibs.2016.09.002
53. Lamkanfi M. Emerging inflammasome effector mechanisms. Nat Rev Immunol. (2011) 11:213–20. doi: 10.1038/nri2936
54. Sun K, Song X, Jia R, Yin Z, Zou Y, Li L, et al. Evaluation of analgesic and anti-inflammatory activities of water extract of Galla chinensis in vivo models. Evid-Based Compl Alt. (2018). doi: 10.1155/2018/6784032
55. Qu J, Wang W, Zhang Q, Li S. Inhibition of lipopolysaccharide-induced inflammation of chicken liver tissue by selenomethionine via TLR4-NF-κB-NLRP3 signaling pathway. Biol Trace Elem Res. (2020) 195:205–14. doi: 10.1007/s12011-019-01841-0
56. Singh A, Koduru B, Carlisle C, Akhter H, Liu RM, Schroder K, et al. NADPH oxidase 4 modulates hepatic responses to lipopolysaccharide mediated by Toll-like receptor-4. Sci Rep-UK. (2017) 7:1–12. doi: 10.1038/s41598-017-14574-8
57. Park JE, Lee H, Kim SY, Lim Y. Lespedeza bicolor extract ameliorated renal inflammation by regulation of NLRP3 inflammasome-associated hyperinflammation in type 2 diabetic mice. Antioxidants. (2020) 9:148. doi: 10.3390/antiox9020148
58. Jawien J, Gajda M, Mateuszuk L, Olszanecki R, Jakubowski A, Szlachcic A, et al. Inhibition of nuclear factor-kappaB attenuates artherosclerosis in apoE/LDLR-double knockout mice. J Physiol Pharmacol. (2005) 56:483–9.
59. Su Q, Li L, Sun Y, Yang H, Ye Z, Zhao J. Effects of the TLR4/Myd88/NF-κB signaling pathway on NLRP3 inflammasome in coronary microembolization-induced myocardial injury. Cell Physiol Biochem. (2018) 47:1497–508. doi: 10.1159/000490866
60. Subramani T, Rathnavelu V, Alitheen N B, Padmanabhan P. Cellular crosstalk mechanism of Toll-like receptors in gingival overgrowth. In J Mol Med. (2015) 35:1151–8. doi: 10.3892/ijmm.2015.2144
61. Saikh KU. MyD88 and beyond: a perspective on MyD88-targeted therapeutic approach for modulation of host immunity. Immunol Res. (2021) 69:117–28. doi: 10.1007/s12026-021-09188-2
62. Chen F, Chen ZQ, Zhong GL, Zhu JJ. Nicorandil inhibits TLR4/MyD88/NF-κB/NLRP3 signaling pathway to reduce pyroptosis in rats with myocardial infarction. Exp Biol Med. (2021) 246:1938–47. doi: 10.1177/15353702211013444
63. Sivanantham A, Pattarayan D, Bethunaickan R, Kar A, Mahapatra SK, Thimmulappa RK, et al. Tannic acid protects against experimental acute lung injury through downregulation of TLR4 and MAPK. J Cell Physiol. (2019) 234:6463–76. doi: 10.1002/jcp.27383
64. Wu Y, Zhong L, Yu Z, Qi J. Anti-neuroinflammatory effects of tannic acid against lipopolysaccharide-induced BV2 microglial cells via inhibition of NF-κB activation. Drug Develop Res. (2019) 80:262–8. doi: 10.1002/ddr.21490
65. Shojaie L, Iorga A, Dara L. Cell death in liver diseases: a review. Int J Mol Sci. (2020) 21:9682. doi: 10.3390/ijms21249682
66. Kesavardhana S, Malireddi RS, Kanneganti TD. Caspases in cell death, inflammation, and gasdermin-induced pyroptosis. Annu Rev Immunol. (2020) 38:567. doi: 10.1146/annurev-immunol-073119-095439
67. Miao EA, Rajan JV, Aderem A. Caspase-1-induced pyroptotic cell death. Immunol Rev. (2011) 243:206–14. doi: 10.1111/j.1600-065X.2011.01044.x
68. Kelley N, Jeltema D, Duan Y, He Y. The NLRP3 inflammasome: an overview of mechanisms of activation and regulation. Int J Mol Sci. (2019) 20:3328. doi: 10.3390/ijms20133328
69. Lossi L, Castagna C, Merighi A. Caspase-3 mediated cell death in the normal development of the mammalian cerebellum. Int J Mol Sci. (2018) 19:3999. doi: 10.3390/ijms19123999
70. Wagenknecht B, Hermisson M, Groscurth P, Liston P, Krammer PH, Weller M. Proteasome inhibitor-induced apoptosis of glioma cells involves the processing of multiple caspases and cytochrome c release. J Neurochem. (2000) 75:2288–97. doi: 10.1046/j.1471-4159.2000.0752288.x
71. Youle RJ, Strasser A. The BCL-2 protein family: opposing activities that mediate cell death. Nat Rev Mol Cell Bio. (2008) 9:47–59. doi: 10.1038/nrm2308
72. Cao XY, Liu D, Bi RC, He YL, He Y, Liu JL. The protective effects of a novel polysaccharide from Lentinus edodes mycelia on islet β (INS-1) cells damaged by glucose and its transportation mechanism with human serum albumin. Int J Biol Macromol. (2019) 134:344–53. doi: 10.1016/j.ijbiomac.2019.05.033
73. Ji C, Wang M, Fan X. Water extract of gallnut reduces the injury of alveolar epithelial cells induced by streptococcus pneumoniae by up-regulating miRNA-338-3p. J Biomater Tiss Eng. (2021) 11:1969–76. doi: 10.1166/jbt.2021.2775
74. Bilic-Sobot D, Kubale V, Skrlep M, Candek-Potokar M. Prevolnik PovseM, Fazarinc G, et al. Effect of hydrolysable tannins on intestinal morphology, proliferation and apoptosis in entire male pigs. Arch Anim Nutr. (2016) 70:378–88. doi: 10.1080/1745039X.2016.1206735
Keywords: broiler chicken, Galla Chinensis, immune function, liver health, tannic acid, TLR4/MyD88/NF-κB
Citation: Niu J, Wang Q, Jing C, Liu Y, Liu H, Jiao N, Huang L, Jiang S, Guan Q, Li Y and Yang W (2022) Dietary Galla Chinensis tannic acid supplementation in the diets improves growth performance, immune function and liver health status of broiler chicken. Front. Vet. Sci. 9:1024430. doi: 10.3389/fvets.2022.1024430
Received: 21 August 2022; Accepted: 26 September 2022;
Published: 14 October 2022.
Edited by:
Fazul Nabi, Lasbela University of Agriculture, PakistanReviewed by:
Hongbin Si, Guangxi University, ChinaCopyright © 2022 Niu, Wang, Jing, Liu, Liu, Jiao, Huang, Jiang, Guan, Li and Yang. This is an open-access article distributed under the terms of the Creative Commons Attribution License (CC BY). The use, distribution or reproduction in other forums is permitted, provided the original author(s) and the copyright owner(s) are credited and that the original publication in this journal is cited, in accordance with accepted academic practice. No use, distribution or reproduction is permitted which does not comply with these terms.
*Correspondence: Yang Li, bGlfeWFuZ0BzZGF1LmVkdS5jbg==; Weiren Yang, d3J5YW5nQHNkYXUuZWR1LmNu
Disclaimer: All claims expressed in this article are solely those of the authors and do not necessarily represent those of their affiliated organizations, or those of the publisher, the editors and the reviewers. Any product that may be evaluated in this article or claim that may be made by its manufacturer is not guaranteed or endorsed by the publisher.
Research integrity at Frontiers
Learn more about the work of our research integrity team to safeguard the quality of each article we publish.