- 1Department of Agricultural Sciences, Faculty of Agro-Based Industry, Universiti Malaysia Kelantan, Jeli, Kelantan, Malaysia
- 2Center of Fundamental and Continuing Education, Universiti Malaysia Terengganu, Kuala Nerus, Terengganu, Malaysia
- 3Department of Paraclinical Study, Faculty of Veterinary Medicine, Universiti Malaysia Kelantan, Kota Bharu, Kelantan, Malaysia
- 4Department of Chemical Pathology, School of Medical Sciences, Universiti Sains Malaysia, Kota Bharu, Kelantan, Malaysia
- 5Department of Animal and Aquatic Sciences, Faculty of Agriculture, Chiang Mai University, Chiang Mai, Thailand
- 6Science and Technology Research Institute, Chiang Mai University, Chiang Mai, Thailand
- 7Faculty of Data Science and Information Technology, INTI International University, Nilai, Malaysia
Aeromonas hydrophila is a ubiquitous bacterium with various hosts that causes mass mortality in farm-raised fish species and significant economic losses. The current antibiotic treatment is ineffective in controlling this bacterium infection in aquaculture species. Therefore, an evaluation of potential phytobiotics is needed to find an alternative antimicrobial agent to reduce the over-reliance on antibiotics in aquaculture and safeguard public and environmental health. Furthermore, the rise in antibiotic resistance cases among pathogenic bacteria indicates an urgent need for new fish and shellfish health management solutions. In this context, phytobiotics applications in aquaculture can be defined as any medicinal plant-based antimicrobial agent used in fish and shellfish health management. This review will focus on the impacts of Motile Aeromonas Septicemia (MAS) due to A. hydrophila in aquaculture, the potential of phytobiotics in enhancing the tolerance of aquaculture species against MAS and the combination of phytobiotics with other antimicrobial and therapeutic agents against MAS.
Introduction
The aquaculture industry is essential in sustaining more than 1 billion population worldwide who depends on fish as their primary source of protein (1, 2). This fast-growing industry (3, 4) recorded an all-time high production, with a total live weight of 114.5 million tons (5). As aquaculture intensifies, diseases have become a major constraint for the industry, i.e., Motile Aeromonad Septicemia (MAS) caused by Aeromonas hydrophila. A. hydrophila can be found in various environments such as marine, freshwater, brackish water, water supplies, and incredibly abundant during the warmer seasons (6). The hosts of A. hydrophila are vast, ranging from freshwater to marine aquatic species (7). This bacterium was also reported to be coinfect with epizootic ulcerative syndrome (EUS) (8). Thus, this highly virulent microorganism may cause mass mortality of aquaculture species on farm sites (9).
Aquatic animals infected by MAS exhibit symptoms such as cloacal hemorrhage (10), ascites (11), gastroenteritic hemorrhage (12), and septicemia ulceration on the skin (13). Furthermore, the A. hydrophila strain will determine the appearance of the symptoms in the infected fish (14). Serine protease (ser), cytotoxins and aerolysin (aer) are the common virulence factors that contribute to A. hydrophila pathogenicity (15). In addition, lipase (lip), cytotonic enterotoxins (act, alt, ast), polar flagella (fla), DNases (exu), type III secretion system (ascV), cholesterol acyltransferase (gcaT), and elastase (ahyB) have been associated with A. hydrophila pathogenicity (16–18). These virulence factors, in combination with other factors, will lead to the pathogenicity of A. hydrophila (19).
Traditionally, fish farmers use antibiotics as prevention and treatment for aquaculture species health management. However, the misuse and overexploitation of antibiotics have led to increasing antibiotic resistance cases among pathogenic bacteria from aquaculture sites (20). For example, A. hydrophila, isolated from Nile tilapia, Oreochromis niloticus, was resistant to ampicillin and amoxicillin (21). Therefore, fish farmers have no choice but to increase antibiotic dosages to treat diseases in aquaculture. A study suggests a combination of two different antibiotics, thiamphenicol, and florfenicol, were effective at lower dosages against A. hydrophila infection in Nile tilapia (22). Consequently, studies are ongoing to find alternative treatments such as vaccination programs (23) and phytobiotics applications (24–28). Various studies have revealed the potential of phytobiotics as an alternative to antibiotics, thus, minimizing antibiotics usage in aquaculture. Phytobiotics contain bioactive compounds such as alkaloids, sterol, flavonoids, saponins, and tannins that possess bactericidal properties and stimulatory effects (29). For example, miswak, and Salvadora persica, contain broad spectrum bactericidal compounds such as benzyl isothiocyanate, salvadourea, salvadorine (29), and vitamin C that can promote healing and tissue repair (30).
This review summarized the impacts of MAS caused by A. hydrophila in aquaculture, phytobiotics preparation for aquaculture uses, the antibacterial activity of medicinal herbs in aquaculture, phytobiotics-activated innate immunity in aquaculture species, phytobiotics enhanced tolerance of aquaculture species toward MAS caused by A. hydrophila and phytobiotics in combination with other antimicrobial and therapeutic agents against MAS caused by A. hydrophila.
Impacts of MAS caused by A. hydrophila in aquaculture
Disease outbreak is a major constraint to the growth of the aquaculture industry. One of the sources of diseases is A. hydrophila, an opportunistic pathogen associated with secondary infection (31) and outbreaks (see Table 1). Fish infected with MAS will exhibit symptoms such as lose appetite, skin ulcerations, pale gills, swollen abdomen, and abnormal swimming pattern. Antibiotics such as oxytetracycline and terramycin were commonly used for treatment. A. hydrophila can cause MAS that has detrimental impacts on aquaculture, such as devastating fish farms, causing economic losses, and pose a threat to public health and environmental safety. This disease was first reported by Llobrera and Gacutan (32) in snakehead, catfish, carp and goby in Laguna de Bay, Philippines, where the infected species exhibited lesions and necrotic ulcers. Many studies reported that MAS was responsible for the mass mortality of aquaculture species. For instance, a mass mortality of Channel catfish, Ictalurus punctatus, in commercial fish farms in the Southeastern USA (31). Furthermore, the total loss of this outbreak in commercial fish farms amounted to millions of USD, leading to numerous fish farm closures (33).
The MAS is also reported to alter the appearance of an aquaculture species, contributing to the losses in market value. For example, Dierckens et al. (39) claimed that the Fairy shrimp, Branchinecta gigas (Lynch), infected with MAS appeared black, causing a substantial price drop. Furthermore, the risk of mass mortality among aquaculture species due to this disease forced fish farmers to harvest and sell their products in the market immediately to reduce losses. Moreover, disease control via antibiotics administration without adequately assessing the effectiveness of the treatments contributes to the infiltration of residues in the environment and microflora breakdown in the area (40), besides posing a threat to public health and the environment.
Phytobiotics vs. commercially developed vaccines against MAS
Fish vaccination was developed more than 50 years ago (41) and reported to be effective in disease control. The vaccines in aquaculture can be administrated via immersion or intraperitoneal injection. In addition, live vaccines were reported to be highly effective in stimulating vigorous antibody activity in fish when administered orally or by immersion. An ideal vaccine is safe for the fish, environmentally friendly, cost-effective, user friendly, and highly effective with little or no side effects (41). In a recent study, fish vaccination has been improved by dissolving microneedle patches. Yun et al. (42) developed this novel vaccination administration to prevent A. hydrophila infection in fish. Dissolving microneedle patches were more effective and can be an alternative to injection in fish vaccination. Currently, the commercial vaccine (Alphaject Panga 2) is used against MAS, in stripped catfish farming in Vietnam (41). However, there are several issues with using the vaccine in aquaculture. High cost and labor intensive are two major factors to be considered by the fish farmer where these factors will hinder vaccines applied extensively in aquaculture.
On the other hand, phytobiotics are plants or plant-based bioactive compounds beneficial for farmers, animals and humans. Examples of phytobiotics are essential oil, legumes, herbs, fruits and vegetables, alkaloids, carotenoids, and phenolic compounds (43, 44). These phytobiotics are widely used in aquaculture feed additives to enhance the immune system and protect aquatic animals against MAS. Since phytobiotics are abundant and inexpensive, fish farmers utilized this treatment as a vaccine replacement in aquaculture health management.
Phytobiotics preparation for aquaculture uses
There are several methods to prepare phytobiotics for aquaculture uses, such as aqueous extracts, methanol extract, and powder form. Methanol is a universal solvent used to extract polar compounds, especially in plants (45), whereas aqueous extracts derive non-polar compounds in phytobiotics. Several studies utilize methanol as a polar aqueous solvent for non-polar extraction in phytobiotics preparation. For example, Wei et al. (46) and Zhou et al. (36) compared the efficacy of bacterial inhibition between methanolic and aqueous extracts. Most studies used methanol in phytobiotics preparation since this solvent was found effectively derives all the properties in the sample (45), Sheikhlar et al. (47), Lee et al. (20), Sheikhlar et al. (48), Bao et al. (49), and Rashmeei et al. (50). The extracts were then used as feed additives in the feeding trials.
Phytobiotics can also be prepared in powder form. For example, Thanikachalam et al. (51) prepared dried garlic peel powder before being incorporated into fish feed for a feeding trial. Similarly, phytobiotics are prepared as feed additives using Rosemary leaf (52), Psidium guajava L. leaf (53, 54), and Spirulina (Arthrospira platensis) (55). Furthermore, some phytobiotics can be commercially available such as tapioca (56), curcumin (57), origanum essential oil (38), and thyme, red thyme and pepper rosemary essential oil (58). Recently, studies have utilized the polysaccharide derived from Pistacia vera hulls (59) or fermentation in preparing phytobiotics (60).
Antibacterial activity of medicinal herbs in aquaculture
Despite the rapid action of antibiotics in controlling a disease outbreak, the residues can disrupt the natural microflora by seeping into the surrounding sediment and water bodies (61). Furthermore, antibiotics such as oxolinic acid, flumequine, oxytetracycline, and sulfadiazine are stable for up to 3 months in soil (62). Thus, there is a dire need for an alternative antimicrobial agent for aquaculture uses.
Medicinal herbs are extensively used as a treatment for human diseases. The whole plant or part of the herbaceous plant, such as twigs, roots, stem, flower, and fruit, are subjected to the extraction process for bioactive compound derivation (63). Bioactive compounds include terpenoids, tannins, alkaloids, and flavonoids, which possess antibacterial properties (64). Furthermore, numerous studies have revealed the potential of medicinal herbs for aquaculture uses; thus, researchers have developed new and improved approaches for antibacterial discoveries from plants. For instance, antibacterial properties were characterized in polysaccharides derived from macroalgae, Chaetomorpha aerea (65) and nanoscale silver particles of butter fruit, Persea americana (66). These studies highlighted future research prospects for plant-based polysaccharide-derived compounds and nano-synthetic substances.
Based on the literature, many medicinal herbs were reported to demonstrate antibacterial properties against A. hydrophila. For instance, Murraya koenigii, Pandanus odoratissimus (46), Colocasia esculenta (67), and Euphorbia hirta (48) inhibited the growth of A. hydrophila (Table 2). These medicinal herbs contain bioactive compounds, such as carbazole alkaloids (68), phenolic compounds (69), polypeptides (70), and alkaloids (71), that was responsible for the antibacterial activities. Moreover, recent studies showed that pomegranate, Punica granatum (Peel), Prunus mume (fruit), Fructus toosendan (fruit), Artemisia argyi (leaves), Polygonum aviculare (leaves), Cephalanoplos segetum (leaves), and Artemisia capillaries (leaves) demonstrated the ability to inhibit A. hydrophila activities (36). Similarly, Piper betle, Piper sarmentosum, and Piper nigrum demonstrated inhibitory activities, as reported by Anjur et al. (72).
Phytobiotics activated the innate immunity of aquaculture species
Antibiotics are essential for aquaculture species health management (40, 75, 76). Nevertheless, antibiotic action is not limited to the target bacteria but also other microorganisms in aquatic animals and the environment (77). Moreover, antibiotic residues in the environment contribute to the development of resistance genes in the microorganisms (78). Vivekanandhan et al. (79) reported that almost 99% of A. hydrophila isolated from seafood (fish and prawn) in the wet market of South India were resistant to novobiocin, bacitracin, rifampicin, and methicillin. Meanwhile, De Silva et al. (80) have revealed that all A. hydrophila isolated from Yesso scallop, Patinopecten yessoensis were resistant to ampicillin, colistin, vancomycin, and cephalothin. Therefore, phytobiotics offer an alternative solution in aquaculture species health management to overcome antibiotic resistance.
Phytobiotics can also activate the innate immunity of aquaculture species to stimulate disease resistance. Many studies have proven the potential of phytobiotics as an alternative antimicrobial agent for aquaculture uses. For instance, Boswellia serrata resin extract was reported can be phytobiotic to enhance the immune system of Nile tilapia and Oreochromis niloticus against Staphylococcus aureus infection (81). In the study, B. serrata resin extract can activate the innate immune system of O. niloticus via the immune response assay, disease resistance assay and growth performance experiment. Similar findings were reported on Spophora flavescens (82), peppermint (Mentha piperita) (83), Aloe vera powder (84), Spirulina platensis (85), citrus lemon peel essential oil (86). The mentioned phytobiotics can improve aquaculture species' growth performance and activate their innate immune system against diseases.
Phytobiotics also stimulate mucus production on fish skin, which acts as a non-specific immunity. Fish skin mucus serves as a primary physical defense against various pathogens. A recent study showed that feeding Siberian sturgeon (Acipenser baerii) with barbery fruit extract for 56 days caused a remarkable improvement in the skin mucus bactericidal activity against A. hydrophila, where the bacterial inhibition zone diameter is higher compared to the fish fed with unsupplemented diet (control group) (87). Another phytobiotics mechanism is through immune cells improvement and macrophages activation, as reported by Chen et al. (88), where Salvia miltiorrhiza polysaccharide exhibited the ability to modulate the disease resistance of sturgeon against A. hydrophila infection. In addition, phytobiotics from plant secondary metabolites such as saponin, essential oils, phenolic compounds, polysaccharides, and polypeptides demonstrated the potential to treat the bacterial infection with low toxicity (89). The phytobiotics can also act as active site modulators, enzymes as catalytic sites, receptors and proteins for disease treatments (90).
Phytobiotics enhanced aquaculture species tolerance against MAS
Existing studies indicated that fish farmers utilize antibiotics, chemicals, phytobiotics, probiotics, prebiotics, yeast extract, vaccine, and disinfectants to control MAS caused by Aeromonas hydrophila in commercial farms (91). Nonetheless, these treatments have been proven unsustainable in preventing MAS caused by A. hydrophila. This catastrophic bacterial disease is responsible for the mass mortality of aquaculture species and significant economic losses. Nevertheless, excessive antibiotic usage will increase antibiotic resistance among pathogenic bacteria, leading to its inefficacy in aquaculture disease control. Therefore, fish farmers must be given more options to use other antimicrobial agents instead of over-relying on antibiotics. Recent studies showed promising findings on phytobiotics in controlling A. hydrophila infection in aquaculture species. The phytobiotics were used as a feed additive given together with feed to aquaculture species for some time can activate and enhance the immune system of aquaculture species against A. hydrophila. For example, Zhang et al. (92) revealed that 1–2% of Flos populi extract incorporated in feed and given to Goldfish (Carassius auratus) for 45 consecutive days could enhance medicated fish growth, antioxidative status, non-specific immunity, and disease resistance to A. hydrophila infection (Table 3). Many other studies have shown a similar trend of findings in the literature. Therefore, there is no doubt phytobiotics can enhance the tolerance of aquaculture species against Motile Aeromonas Septicemia.
Phytobiotics enhance the fish's immune system by increasing the presence of immune markers such as immunoglobulin M (IgM), nitric oxide and lysozyme. For example, Abdellatief et al. (108) revealed that a combination of sage (Salvia officinalis) and Spirulina platensis (Arthrospira platensis) can boost the immune system of Nile tilapia by increasing their immune response against Pseudomonas aeruginosa. Furthermore, phytobiotics, in the form of antioxidant compounds such as phenols and polyphenols, are beneficial for fish health by improving the immune system of aquatic animals against A. hydrophila (109). For instance, Naiel et al. (52) revealed that 10 g/kg rosemary (Salvia rosmarinus) leaves enhanced Nile tilapia's immune system and increased their disease resistance against A. hydrophila. Moreover, the phytobiotic promoted lysozyme and serum catalase activity in fish against MAS.
Overall, phytobiotics with low dose and shorter administration duration are the best candidate as antimicrobial aganet to against MAS. Based on literature survey, phytobiotics such as polysaccharide of Ficus carica (106), essential oil of thyme, red thyme and pepper rosemary (58) and methanolic extract of Pepperomia pellucida (leaves) (20) were effective in controlling MAS infection as the duration of administration is shorter compared to other phytobiotics. Furthermore, low dose is needed for the mentioned phytobiotics. Further studies need to be carried out in the near future to reveal potential bioactive compounds that present in Gracilaria persica (107), Salvadora persica (29), Andrographis paniculata (105), Curcumin (57), Moringa oleifera (60), and many more. The future study findings are important to understand the mechanisms of the bioactive compounds in mitigating A. hydrophila impacts on aquatic animals.
Phytobiotics combined with other antimicrobial agents/therapeutic agents against MAS
The emergence of antibiotic resistance among pathogenic bacteria from aquaculture sites revealed that antibiotics alone are not a sustainable antimicrobial agent (110). Therefore, researchers have proposed alternative solutions, such as phytobiotics. Several studies have also reported on the synergistic effects between phytobiotics and other supplements such as boron (94), probiotics (111, 112), Spirulina (113), and antibiotic (36) to relieve the impacts of A. hydrophila in aquaculture species (Table 4). Thus, these combinations are promising antimicrobial agents for cost-effective treatments in aquaculture disease management.
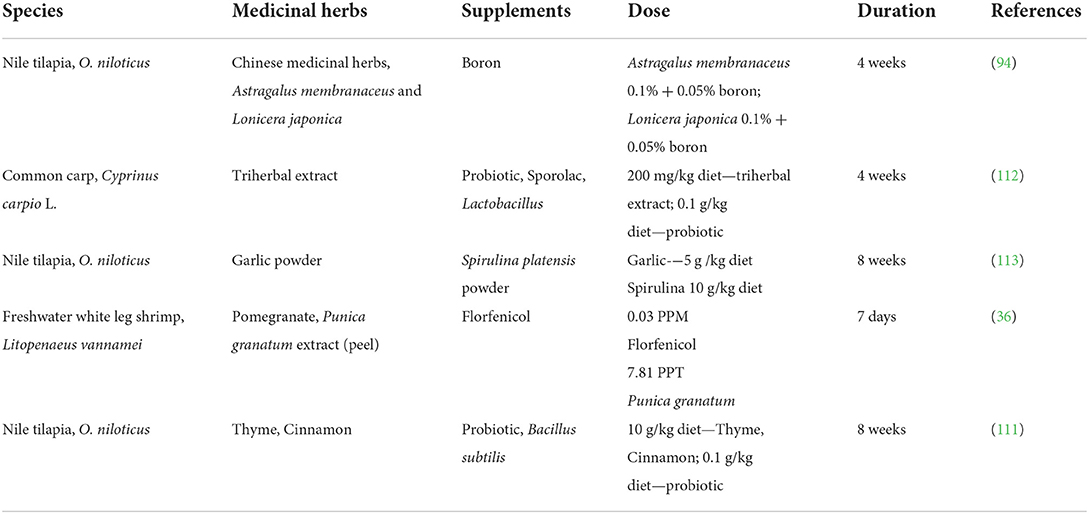
Table 4. Medicinal herbs and antimicrobial/therapeutic agents reduce the impacts of A. hydrophila on aquatic animals.
Conclusion and recommendation
In summary, MAS is a catastrophic disease in the aquaculture industry that can lead to significant economic losses and environmental and public health hazards. Antibiotics have been proven a short-term solution in managing bacterial disease in the aquaculture industry. Therefore, phytobiotics are a viable alternative for aquaculture species health management and for maintaining public health and environmental safety. Despite that, researchers must monitor phytobiotics toxicity as a prerequisite for aquaculture application. Numerous studies have demonstrated the potential of phytobiotics in activating the innate immune system and stimulating disease resistance of aquaculture species against MAS such as essential oil of thyme, red thyme and pepper rosemary whereas methanolic extraction was the best way in preparing phytobiotics. Besides, bioactive compound such as polysaccharide of Ficus carica can be effective phytobiotics. Furthermore, applying less ecological footprint antimicrobial agents like phytobiotics can gain consumer confidence in aquaculture products. Nevertheless, there is a gap between the scientific approach and practical use of phytobiotics in aquaculture that can be addressed through knowledge and technology transfer among aquaculturists. Moreover, phytobiotics application in aquaculture remains inconsistent in terms of dosage and duration. These factors are crucial in phytobiotics against MAS because different dosages and duration will lead to variable outcomes. Additionally, phytobiotics efficacy in controlling MAS depends on the source of phytobiotics and environmental conditions. Also, phytobiotics can be administrated orally via incorporation in aquaculture feed, thus, a practical, non-stressful, and convenient administration of feed additives for various aquaculture species. Overall, phytobiotics are promising antimicrobial agents in controlling MAS. Most importantly, phytobiotics derived from agricultural wastes have been proven sustainable in aquaculture practices. Further studies need to be carried out in the near future to characterize bioactive compounds that present in phytobiotics and responsible to inhibitory activity against MAS. This is important to understand the mechanisms of the bioactive compounds. In addtion, new potential phytobiotics can be explored from plant families such as Rutaceae, Fabaceae, and Moringaceae.
Author contributions
ZA: conceptualization, writing—review and editing, and writing—original draft. WW: writing—original draft and writing—review and editing. SM, HC, MH, MI and HV: writing—review and editing. KW: supervision and conceptualization. LS: project administration, writing—original draft, and writing—review and editing. All authors contributed to the article and approved the submitted version.
Funding
This project was funded by the Ministry of Education, Malaysia, under the Fundamental Research Grant Scheme (FRGS/1/2022/STG03/UMK/03/1), Niche Research Grant Scheme (NRGS) (R/NRGS/A0.700/00387A/006/2014/00152), and Universiti Malaysia Kelantan Matching Grant (Grant no: R/UMK MATCH/A0.700/00387A/008/2022). This research work was partially supported by Chiang Mai University.
Conflict of interest
The authors declare that the research was conducted in the absence of any commercial or financial relationships that could be construed as a potential conflict of interest.
Publisher's note
All claims expressed in this article are solely those of the authors and do not necessarily represent those of their affiliated organizations, or those of the publisher, the editors and the reviewers. Any product that may be evaluated in this article, or claim that may be made by its manufacturer, is not guaranteed or endorsed by the publisher.
References
1. Kari ZA, Kabir MA, Dawood MA, Razab MKAA, Ariff NSNA, Sarkar T, et al. Effect of fish meal substitution with fermented soy pulp on growth performance, digestive enzyme, amino acid profile, and immune-related gene expression of African catfish (Clarias gariepinus). Aquaculture. (2022) 546:737418. doi: 10.1016/j.aquaculture.2021.737418
2. Obi F, Ugwuishiwu B, Nwakaire J. Agricultural waste concept, generation, utilization and management. Nigerian J Technol. (2016) 35:957–64. doi: 10.4314/njt.v35i4.34
3. Akmal M, Rahimi-Midani A, Hafeez-ur-Rehman M, Hussain A, Choi T-J. Isolation, characterization, and application of a bacteriophage infecting the fish pathogen Aeromonas hydrophila. Pathogens. (2020) 9:215. doi: 10.3390/pathogens9030215
4. Kari ZA, Kabir MA, Mat K, Rusli ND, Razab MKAA, Ariff NSNA, et al. The possibility of replacing fish meal with fermented soy pulp on the growth performance, blood biochemistry, liver, and intestinal morphology of African catfish (Clarias gariepinus). Aquacult Rep. (2021) 21:100815. doi: 10.1016/j.aqrep.2021.100815
5. FAO F. The State of World Fisheries and Aquaculture. Opportunities and Challenges. Rome, Italy: Food and Agriculture Organization of the United Nations (2020).
6. Jiravanichpaisal P, Roos S, Edsman L, Liu H, Söderhäll K. (2009). A highly virulent pathogen, Aeromonas hydrophila, from the freshwater crayfish Pacifastacus leniusculus. J. Invertebr. Pathol. 101:56–66. doi: 10.1016/j.jip.2009.02.002
7. Larsen J, Jensen N. An Aeromonas species implicated in ulcer-disease of the cod (Gadus morhua). Nord Vet Med. (1977) 29:199–211.
9. Jun JW, Kim JH, Shin SP, Han JE, Chai JY, Park SC. (2013). Protective effects of the Aeromonas phages pAh1-C and pAh6-C against mass mortality of the cyprinid loach (Misgurnus anguillicaudatus) caused by Aeromonas hydrophila. Aquac. 416:289–95. doi: 10.1016/j.aquaculture.2013.09.045
10. Zhang X, Wu W, Li L, Ma X, Chen J. Genetic variation and relationships of seven sturgeon species and ten interspecific hybrids. Genet Sel Evol. (2013) 45:21. doi: 10.1186/1297-9686-45-21
11. Pridgeon J, Klesius P. Molecular identification and virulence of three Aeromonas hydrophila isolates cultured from infected channel catfish during a disease outbreak in west Alabama (USA). Dis Aqua Org. (2011) 94:249–53. doi: 10.3354/dao02332
12. Elsheshtawy A, Yehia N, Elkemary M, Soliman H. Investigation of nile tilapia summer mortality in Kafr El-Sheikh Governate, Egypt. Genet Aqua Org. (2019) 3:17–25. doi: 10.4194/2459-1831-v3_1_03
13. El-Son MA, Abdelkhalek NK, El-Ashram AM, Zaki VH. Phenotypic and biochemical detection of Aeromonas hydrophila isolated from cultured Oreochromis niloticus during disease outbreaks. Int J Fish Aqua Stud. (2019) 7:197–202.
14. Bakiyev S, Izat S, Irina Z, Saidina K, Nurlan S, Gaisa A, et al. Isolation, identification, and characterization of pathogenic Aeromonas hydrophila from critically endangered Acipenser baerii. Aquacult Rep. (2022) 26:101293. doi: 10.1016/j.aqrep.2022.101293
15. Li J, Ni XD, Liu YJ, Lu CP. Detection of three virulence genes alt, ahp and aerA in Aeromonas hydrophila and their relationship with actual virulence to zebrafish. J Appl Microbiol. (2011) 110:823–30. doi: 10.1111/j.1365-2672.2011.04944.x
16. Pattanayak S, Priyadarsini S, Paul A, Kumar PR, Sahoo PK. Diversity of virulence-associated genes in pathogenic Aeromonas hydrophila isolates and their in vivo modulation at varied water temperatures. Micro Patho. (2020) 147:104424. doi: 10.1016/j.micpath.2020.104424
17. Rasmussen CRI, Figueras MJ, McGarey D, Liles MR. Virulence factors of Aeromonas hydrophila: in the wake of reclassification. Front Microbiol. (2016) 7:1337. doi: 10.3389/fmicb.2016.01337
18. Allan BJ, Stevenson RM. Extracellular virulence factors of Aeromonas hydrophila in fish infections. Can J Microbiol. (1981) 27:1114–22. doi: 10.1139/m81-174
19. Zhao XL, Wu G, Chen H, Li L, Kong XH. Analysis of virulence and immunogenic factors in Aeromonas hydrophila: towards the development of live vaccines. J Fish Dis. (2020) 43:747–55. doi: 10.1111/jfd.13174
20. Lee S, Sim K, Wendy W, Zulhisyam A. Peperomia pellucida leaf extract as immunostimulator in controlling motile aeromonad septicemia due to Aeromonas hydrophila in red hybrid tilapia, Oreochromis spp. farming. Vet World. (2016) 9:231. doi: 10.14202/vetworld.2016.231-234
21. Tartor YH, EL-Naenaeey E-SY, Abdallah HM, Samir M, Yassen MM, Abdelwahab AM. Virulotyping and genetic diversity of Aeromonas hydrophila isolated from Nile tilapia (Oreochromis niloticus) in aquaculture farms in Egypt. Aquaculture. (2021) 541:736781. doi: 10.1016/j.aquaculture.2021.736781
22. Assane IM, Gozi KS, Valladao GMR, Pilarski F. Combination of antimicrobials as an approach to reduce their application in aquaculture: emphasis on the use of thiamphenicol/florfenicol against Aeromonas hydrophila. Aquaculture. (2019) 507:238–45. doi: 10.1016/j.aquaculture.2019.04.021
23. Kayansamruaj P, Areechon N, Unajak S. Development of fish vaccine in Southeast Asia: a challenge for the sustainability of SE Asia aquaculture. Fish Shellfish Immunol. (2020) 103:73–87. doi: 10.1016/j.fsi.2020.04.031
24. Maulu S, Langi S, Hasimuna OJ, Missinhoun D, Munganga BP, Hampuwo BM, et al. Recent advances in the utilization of insects as an ingredient in aquafeeds: a review. Animal Nutr. (2022). doi: 10.1016/j.aninu.2022.07.013
25. Kari ZA, Goh KW, Edinur HA, Mat K, Khalid H-NM, Rusli ND, et al. Palm date meal as a non-traditional ingredient for feeding aquatic animals: a review. Aquacult Rep. (2022) 25:101233. doi: 10.1016/j.aqrep.2022.101233
26. Kari ZA, Wee W, Hamid NKA, Mat K, Rusli ND, Khalid HNM, et al. Recent advances of phytobiotic utilization in carp farming: a review aquaculture. Nutrition. (2022). doi: 10.1155/2022/7626675
27. Dawood MA, Habotta OA, Elsabagh M, Azra MN, Van Doan H, Kari ZA, et al. Fruit processing by-products in the aquafeed industry: a feasible strategy for aquaculture sustainability. Rev Aquacult. (2022). doi: 10.1111/raq.12680
28. Dawood MA, Basuini MFE, Yilmaz S, Abdel-Latif HM, Kari ZA, Abdul Razab MKA, et al. Selenium nanoparticles as a natural antioxidant and metabolic regulator in aquaculture: a review. Antioxidants. (2021) 10:1364. doi: 10.3390/antiox10091364
29. Abd El-latif AM, Abd El-Gawad EA, Soror EI, Shourbela RM, Zahran E. Dietary supplementation with miswak (Salvadora persica) improves the health status of Nile tilapia and protects against Aeromonas hydrophila infection. Aquacult Rep. (2021) 19:100594. doi: 10.1016/j.aqrep.2021.100594
30. Haque MM, Alsareii SA. A review of the therapeutic effects of using miswak (Salvadora persica) on oral health. J Saudi Med. (2015) 36:530. doi: 10.15537/smj.2015.5.10785
31. Zhang D, Xu D-H, Shoemaker C. Experimental induction of motile Aeromonas septicemia in channel catfish (Ictalurus punctatus) by waterborne challenge with virulent Aeromonas hydrophila. Aquacult Rep. (2016) 3:18–23. doi: 10.1016/j.aqrep.2015.11.003
32. Llobrera AT, Gacutan RQ. Aeromonas hydrophila associated with ulcerative disease epizootic in Laguna de Bay, Philippines. Aquaculture. (1987) 67:273–8. doi: 10.1016/0044-8486(87)90211-0
33. Peterman MA, Posadas BC. Direct economic impact of fish diseases on the East Mississippi catfish industry. N Am J Aquac. (2019) 81:222–9. doi: 10.1002/naaq.10090
34. Gai C, Ye W, Lu L, Li Y, Yang X, Cao H. Aeromonas hydrophila: a causative agent for tail rot disease in freshwater cultured Murray cod Maccullochella peelii. Israeli J Aquacult Bamidgeh. (2016) 8.
35. Kusdarwati R, Kurniawan H, Prayogi YT. Isolation and identification of Aeromonas hydrophila and Saprolegnia sp. on catfish (Clarias gariepinus) in floating cages in Bozem Moro Krembangan Surabaya. IOP Confer Ser Earth Environ. Sci. (2017). doi: 10.1088/1755-1315/55/1/012038
36. Zhou H, Gai C, Ye G, An J, Liu K, Xu L, et al. Aeromonas hydrophila, an emerging causative agent of freshwater-farmed whiteleg shrimp Litopenaeus vannamei. Microorganisms. (2019) 7:450. doi: 10.3390/microorganisms7100450
37. Rodrigues MV, Dias MFF, Francisco CJ, David GS, da Silva RJ, Junior JPA. Aeromonas hydrophila in Nile tilapia (Oreochromis niloticus) from Brazilian aquaculture: a public health problem. Emerg Life Sci. Res. (2019) 5:48–55. doi: 10.31783/elsr.2019.514855
38. Abdel-Latif HM, Abdel-Tawwab M, Khafaga AF, Dawood MA. Dietary oregano essential oil improved the growth performance via enhancing the intestinal morphometry and hepato-renal functions of common carp (Cyprinus carpio L.) fingerlings. Aquaculture. (2020) 526:735432. doi: 10.1016/j.aquaculture.2020.735432
39. Dierckens K, Vandenberghe J, Beladjal L, Huys G, Mertens J, Swings J. (1998). Aeromonas hydrophila causes “black disease” in fairy shrimps (Anostraca; Crustacea). J. Fish Dis. 21:113–9. doi: 10.1046/j.1365-2761.1998.00085.x
40. Liu J, Gao S, Dong Y, Lu C, Liu Y. Isolation and characterization of bacteriophages against virulent Aeromonas hydrophila. BMC Microbiol. (2020) 20:1–13. doi: 10.1186/s12866-020-01811-w
41. Ma J, Bruce TJ, Jones EM, Cain KD. A review of fish vaccine development strategies: conventional methods and modern biotechnological approaches. Microorganisms. (2019) 7:568. doi: 10.3390/microorganisms7110569
42. Yun S, Lee SJ, Giri SS, Kim HJ, Kim SG, Kim SW, et al. Vaccination of fish against Aeromonas hydrophila infections using the novel approach of transcutaneous immunization with disolving microneedle patches in aquaculture. Fish Shellfish Immunol. (2020) 97:34–40. doi: 10.1016/j.fsi.2019.12.026
43. Liu RH. Potential synergy of phytochemicals in cancer prevention: mechanism of action. J Nutr. (2004) 134:3479–85. doi: 10.1093/jn/134.12.3479S
44. Kikusato M. Phytobiotics to improve health and production of broiler chickens: functions beyond the antioxidant activity. Anim Biosci. (2021) 34:345–53. doi: 10.5713/ab.20.0842
45. Zhang Q-W, Lin L-G, Ye W-C. Techniques for extraction and isolation of natural products: a comprehensive review. Chin Med. (2018) 13:1–26. doi: 10.1186/s13020-018-0177-x
46. Wei LS, Musa N, Sengm CT, Wee W, Shazili NAM. Antimicrobial properties of tropical plants against 12 pathogenic bacteria isolated from aquatic organisms. Afr J Biotechnol. (2008) 7:2275–78.
47. Sheikhlar A, Alimon AR, Daud H, Saad CR, Webster CD, Meng GY, et al. White mulberry (Morus alba) foliage methanolic extract can alleviate Aeromonas hydrophila infection in African Catfish (Clarias gariepinus). Sci World J. (2014). doi: 10.1155/2014/592709
48. Sheikhlar A, Meng GY, Alimon R, Romano N, Ebrahimi M. Dietary Euphorbia hirta extract improved the resistance of sharptooth catfish Clarias gariepinus to Aeromonas hydrophila. J Aquat Anim Health. (2017) 29:225–35. doi: 10.1080/08997659.2017.1374310
49. Bao L, Chen Y, Li H, Zhang J, Wu P, Ye K, et al. Dietary Ginkgo biloba leaf extract alters immune-related gene expression and disease resistance to Aeromonas hydrophila in common carp Cyprinus carpio. Fish Shellfish Immunol. (2019) 94:810–8. doi: 10.1016/j.fsi.2019.09.056
50. Rashmeei M, Shekarabi SPH, Mehrgan MS, Paknejad H. Stimulatory effect of dietary chasteberry (Vitex agnus-castus) extract on immunity, some immune-related gene expression, and resistance against Aeromonas hydrophila infection in goldfish (Carassius auratus). Fish Shellfish Immunol. (2020) 107:129–36. doi: 10.1016/j.fsi.2020.09.037
51. Thanikachalam K, Kasi M, Rathinam X. Effect of garlic peel on growth, hematological parameters and disease resistance against Aeromonas hydrophila in African catfish Clarias gariepinus (Bloch) fingerlings. Asian Pac J Trop Med. (2010) 3:614–8. doi: 10.1016/S1995-7645(10)60149-6
52. Naiel MA, Ismael NE, Negm SS, Ayyat MS, Al-Sagheer AA. Rosemary leaf powder-supplemented diet enhances performance, antioxidant properties, immune status, and resistance against bacterial diseases in Nile Tilapia (Oreochromis niloticus). Aquaculture. (2020) 526:735370. doi: 10.1016/j.aquaculture.2020.735370
53. Giri SS, Sen SS, Chi C, Kim HJ, Yun S, Park SC, et al. Effect of guava leaves on the growth performance and cytokine gene expression of Labeo rohita and its susceptibility to Aeromonas hydrophila infection. Fish Shellfish Immunol. (2015) 46:217–24. doi: 10.1016/j.fsi.2015.05.051
54. Pachanawan A, Phumkhachorn P, Rattanachaikunsopon P. Potential of Psidium guajava supplemented fish diets in controlling Aeromonas hydrophila infection in tilapia (Oreochromis niloticus). J Biosci Bioeng. (2008) 106:419–24. doi: 10.1263/jbb.106.419
55. Cao S, Zhang P, Zou T, Fei S, Han D, Jin J, et al. Replacement of fishmeal by spirulina Arthrospira platensis affects growth, immune related-gene expression in gibel carp (Carassius auratus gibelio var. CAS III), and its challenge against Aeromonas hydrophila infection. Fish Shellfish Immunol. (2018) 79:265–73. doi: 10.1016/j.fsi.2018.05.022
56. Verma A, Rani AB, Rathore G, Saharan N, Gora AH. Growth, non-specific immunity and disease resistance of Labeo rohita against Aeromonas hydrophila in biofloc systems using different carbon sources. Aquaculture. (2016) 457:61–7. doi: 10.1016/j.aquaculture.2016.02.011
57. Ming J, Ye J, Zhang Y, Xu Q, Yang X, Shao X, et al. Optimal dietary curcumin improved growth performance, and modulated innate immunity, antioxidant capacity and related genes expression of NF-κB and Nrf2 signaling pathways in grass carp (Ctenopharyngodon idella) after infection with Aeromonas hydrophila. Fish Shellfish Immunol. (2020) 97:540–53. doi: 10.1016/j.fsi.2019.12.074
58. De Rezende RAE, Soares MP, Sampaio FG, Cardoso IL, Ishikawa MM, Dallago BSL, et al. Phytobiotics blend as a dietary supplement for Nile tilapia health improvement. Fish Shellfish Immunol. (2021) 114:293–300. doi: 10.1016/j.fsi.2021.05.010
59. Mohammadi G, Rafiee G, El Basuini MF, Abdel-Latif HM, Dawood MA. The growth performance, antioxidant capacity, immunological responses, and the resistance against Aeromonas hydrophila in Nile tilapia (Oreochromis niloticus) fed Pistacia vera hulls derived polysaccharide. Fish Shellfish Immunol. (2020) 106:36–43. doi: 10.1016/j.fsi.2020.07.064
60. Zhang X, Sun Z, Cai J, Wang J, Wang G, Zhu Z, et al. Effects of dietary fish meal replacement by fermented moringa (Moringa oleifera Lam.) leaves on growth performance non-specific immunity and disease resistance against Aeromonas hydrophila in juvenile gibel carp (Carassius auratus gibelio var CAS III). Fish Shellfish Immunol. (2020) 102:430–9. doi: 10.1016/j.fsi.2020.04.051
61. Ma D, Hu Y, Wang J, Ye S, Li A. Effects of antibacterials use in aquaculture on biogeochemical processes in marine sediment. Sci Total Environ. (2006) 367:273–7. doi: 10.1016/j.scitotenv.2005.10.014
62. Samuelsen O, Lunestad B, Ervik A, Fjelde S. Stability of antibacterial agents in an artificial marine aquaculture sediment studied under laboratory conditions. Aquaculture. (1994) 126:283–90. doi: 10.1016/0044-8486(94)90044-2
63. Khan UA, Rahman H, Niaz Z, Qasim M, Khan J. Antibacterial activity of some medicinal plants against selected human pathogenic bacteria. Eur J Microbiol Immunol. (2013) 3:272–4. doi: 10.1556/EuJMI.3.2013.4.6
64. Talib WH, Mahasneh AM. Antimicrobial, cytotoxicity and phytochemical screening of Jordanian plants used in traditional medicine. Molecules. (2010) 15:1811–24. doi: 10.3390/molecules15031811
65. Zammuto V, Rizzo MG, Spanò A, Genovese G, Morabito M, Spagnuolo D, et al. In vitro evaluation of antibiofilm activity of crude extracts from macroalgae against pathogens relevant in aquaculture. Aquaculture. (2022) 549:737729. doi: 10.1016/j.aquaculture.2021.737729
66. Mani R, Vijayakumar P, Dhas S, Velu K, Inbakandan D, Thamaraiselvi C, et al. Biogenic nanoscale silver particles synthesis using butter fruit pulp extract and study of antibacterial efficacy against Providencia vermicola in rohu fish. J King Saud Univ Sci. (2022) 101814. doi: 10.1016/j.jksus.2021.101814
67. Wei L, Musa N, Wee W. In vitro antimicrobial activities of Colocasia esculenta extract against Vibrio spp.-short communication. Agricultura. (2010) 7:5–7.
68. Balakrishnan R, Vijayraja D, Jo SH, Ganesan P, Kim IS, Choi DK. Medicinal profile, phytochemistry, and pharmacological activities of Murraya koenigii and its primary bioactive compound. Antioxidants. (2020) 9:101. doi: 10.3390/antiox9020101
69. Adkar P, Bhaskar VH. Pandanus odoratissimus (Kewda): a review on ethnopharmacology, phytochemistry, and nutritional aspects. Adv Pharmacol Sci. (2014) 2014:120895. doi: 10.1155/2014/120895
70. Pereira PR, Silva JT, Vericimo MA, Paschoalin VMF, Teixeira, GAPB. Crude extracts from taro (Colocasia esculenta) as a natural source of bioactive proteins able to stimulate haematopoietic cells in two murine models. J Func Foods. (2015) 18:333–43. doi: 10.1016/j.jff.2015.07.014
71. Kumar S, Malhotra R, Kumar D. Euphorbia hirta: its chemistry, traditional and medicinal uses, and pharmacological activities. Pharmacogn Rev. (2010) 4:58–61. doi: 10.4103/0973-7847.65327
72. Anjur N, Sabran SF, Daud HM, Othman NZ (2022). Antibacterial Activity and Toxicity Study of Selected Piper Leave Extracts Against the Fish Pathogen (Aeromonas hydrophila). 7th International Conference on Biological Science (ICBS 2021), Vol. 22, 118–123. doi: 10.2991/absr.k.220406.018
73. Genovese G, Faggio C, Gugliandolo C, Torre A, Spanò A, Morabito M, et al. In vitro evaluation of antibacterial activity of Asparagopsis taxiformis from the straits of Messina against pathogens relevant in aquaculture. Mar Environ Res. (2012) 73:1–6. doi: 10.1016/j.marenvres.2011.10.002
74. Xia Y-T, Chan GK-L, Wang H-Y, Dong TT-X, Duan R, Hu W-H, et al. The antibacterial effects of aerial parts of Scutellaria baicalensis: potential application as an additive in aquaculture feedings. Aquaculture. (2020) 526:735418. doi: 10.1016/j.aquaculture.2020.735418
75. Van Boeckel TP, Brower C, Gilbert M, Grenfell BT, Levin SA, Robinson TP, et al. Global trends in antimicrobial use in food animals. Proc Nat Acad Sci USA. (2015) 112:5649–54. doi: 10.1073/pnas.1503141112
76. Van Boeckel TP, Pires J, Silvester R, Zhao C, Song J, Criscuolo NG, et al. Global trends in antimicrobial resistance in animals in low-and middle-income countries. Science. (2019) 365:6459. doi: 10.1126/science.aaw1944
77. Martinez JL. Environmental pollution by antibiotics and by antibiotic resistance determinants. Environ Pollut. (2009) 157:2893–902. doi: 10.1016/j.envpol.2009.05.051
78. Stratev D, Odeyemi OA. Antimicrobial resistance of Aeromonas hydrophila isolated from different food sources: a mini-review. J Infect Public Health. (2016) 9:535–44. doi: 10.1016/j.jiph.2015.10.006
79. Vivekanandhan G, Savithamani K, Hatha A, Lakshmanaperumalsamy P. Antibiotic resistance of Aeromonas hydrophila isolated from marketed fish and prawn of South India. Int J Food Microbiol. (2002) 76:165–8. doi: 10.1016/S0168-1605(02)00009-0
80. De Silva B, Hossain S, Dahanayake P, Heo GJ. Aeromonas spp. from marketed Yesso scallop (Patinopecten yessoensis): molecular characterization, phylogenetic analysis, virulence properties and antimicrobial susceptibility. J Appl Microbiol. (2019) 126:288–99. doi: 10.1111/jam.14106
81. Montaser MM, El-Sharnouby ME, El-Noubi G, El-Shaer HM, Khalil AA, Hassanin M, et al. Boswellia serrata resin extract in diets of Nile Tilapia, Oreochromis niloticus: effects on the growth, health, immune response, and disease resistance to Staphylococcus aureus. Animals. (2021) 11:446. doi: 10.3390/ani11020446
82. Wu Y-r, Gong Q-f, Fang H, Liang W-w, Chen M, He R.-j. Effect of Sophora flavescens on non-specific immune response of tilapia (GIFT Oreochromis niloticus) and disease resistance against Streptococcus agalactiae. Fish Shellfish Immunol. (2013) 34:220–7. doi: 10.1016/j.fsi.2012.10.020
83. Talpur AD. Mentha piperita (Peppermint) as feed additive enhanced growth performance, survival, immune response and disease resistance of Asian seabass, Lates calcarifer (Bloch) against Vibrio harveyi infection. Aquaculture. (2014) 420:71–8. doi: 10.1016/j.aquaculture.2013.10.039
84. Gabriel NN, Qiang J, He J, Ma XY, Kpundeh MD, Xu P. Dietary Aloe vera supplementation on growth performance, some haemato-biochemical parameters and disease resistance against Streptococcus iniae in tilapia (GIFT). Fish Shellfish Immunol. (2015) 44:504–14. doi: 10.1016/j.fsi.2015.03.002
85. Adel M, Yeganeh S, Dadar M, Sakai M, Dawood MA. Effects of dietary Spirulina platensis on growth performance, humoral and mucosal immune responses and disease resistance in juvenile great sturgeon (Huso huso Linnaeus, 1754). Fish Shellfish Immunol. (2016) 56:436–44. doi: 10.1016/j.fsi.2016.08.003
86. Baba E, Acar Ü, Öntaş C, Kesbiç OS, Yilmaz S. Evaluation of Citrus limon peels essential oil on growth performance, immune response of Mozambique tilapia Oreochromis mossambicus challenged with Edwardsiella tarda. Aquaculture. (2016) 465:13–8. doi: 10.1016/j.aquaculture.2016.08.023
87. Shekarabi SPH, Mehrgan MS, Ramezani F, Dawood MAO, Doan HV, Moonmanee T, et al. Effect of dietary barberry fruit (Berberis vulgaris) extract on immune function, antioxidant capacity, antibacterial activity, and stress-related gene expression of Siberian sturgeon (Acipenser baerii). Aquacult Rep. (2022) 23:101041. doi: 10.1016/j.aqrep.2022.101041
88. Chen D, Yang L, Yang F, Pei Q, Lu L, Huang X, et al. Salvia miltiorrhiza polysaccharide activated macrophages and improved the disease resistance of sturgeon against Aeromonas hydrophila. Fish Shellfish Immunol. (2022) 127:594–603. doi: 10.1016/j.fsi.2022.06.062
89. Paray BA, Hoseini SM, Hoseinifar SH, Van Doan H. Effects of dietary oak (Quercus castaneifolia) leaf extract on growth, antioxidant, and immune characteristics and responses to crowding stress in common carp (Cyprinus carpio). Aquaculture. (2020) 524:735276. doi: 10.1016/j.aquaculture.2020.735276
90. Wink M. Evolution of toxins and antinutritional factors in plants with special emphasis on Leguminosae. In:Acamovic T, Stewart CS, Pennycott TW, editors. Poisonous Plants and Related Toxins. Oxford: CABI Publishing (2004). p. 1–25.
91. Stratev D, Odeyemi OA. An overview of motile Aeromonas septicaemia management. Aquacult Int. (2017) 25:1095–105. doi: 10.1007/s10499-016-0100-3
92. Zhang X, Sun Z, Wang Y, Cao Y, Wang G, Cao F. Enhancement of growth, antioxidative status, non-specific immunity, and disease resistance in gibel carp (Carassius auratus) in response to dietary Flos populi extract. Fish Physiol Biochem. (2022) 48:67–83. doi: 10.1007/s10695-021-00992-z
93. Sahu S, Das B, Mishra B, Pradhan J, Sarangi N. Effect of Allium sativum on the immunity and survival of Labeo rohita infected with Aeromonas hydrophila. J Appl Ichthyol. (2007) 23:80–6. doi: 10.1111/j.1439-0426.2006.00785.x
94. Ardó L, Yin G, Xu P, Váradi L, Szigeti G, Jeney Z, et al. Chinese herbs (Astragalus membranaceus and Lonicera japonica) and boron enhance the non-specific immune response of Nile tilapia (Oreochromis niloticus) and resistance against Aeromonas hydrophila. Aquaculture. (2008) 275:26–33. doi: 10.1016/j.aquaculture.2007.12.022
95. Harikrishnan R, Balasundaram C, Heo M-S. Herbal supplementation diets on hematology and innate immunity in goldfish against Aeromonas hydrophila. Fish Shellfish Immunol. (2010) 28:354–61. doi: 10.1016/j.fsi.2009.11.013
96. Abdel-Tawwab M, Ahmad MH, Seden ME, Sakr SF. Use of green tea. Camellia sinensis L, in practical diet for growth and protection of Nile tilapia, Oreochromis niloticus (L), against Aeromonas hydrophila infection. J World Aquacult Soc. (2010) 41:203–13. doi: 10.1111/j.1749-7345.2010.00360.x
97. Ahmad MH, El Mesallamy AM, Samir F, Zahran F. Effect of cinnamon (Cinnamomum zeylanicum) on growth performance, feed utilization, whole-body composition, and resistance to Aeromonas hydrophila in Nile tilapia. J Appl Aquacult. (2011) 23:289–98. doi: 10.1080/10454438.2011.626350
98. Abdel-Tawwab M. The use of American ginseng (Panax quinquefolium) in practical diets for Nile tilapia (Oreochromis niloticus): growth performance and challenge with Aeromonas hydrophila. J Appl Aquacult. (2012) 24:366–76. doi: 10.1080/10454438.2012.733593
99. Park K-H, Choi S-H. The effect of mistletoe, Viscum album coloratum, extract on innate immune response of Nile tilapia (Oreochromis niloticus). Fish Shellfish Immunol. (2012) 32:1016–21. doi: 10.1016/j.fsi.2012.02.023
100. Tang J, Cai J, Liu R, Wang J, Lu Y, Wu Z, et al. Immunostimulatory effects of artificial feed supplemented with a Chinese herbal mixture on Oreochromis niloticus against Aeromonas hydrophila. Fish Shellfish Immunol. (2014) 39:401–6. doi: 10.1016/j.fsi.2014.05.028
101. Wang E, Chen X, Wang K, Wang J, Chen D, Geng Y, et al. Plant polysaccharides used as immunostimulants enhance innate immune response and disease resistance against Aeromonas hydrophila infection in fish. Fish Shellfish Immunol. (2016) 59:196–202. doi: 10.1016/j.fsi.2016.10.039
102. Dos Santos AC, Sutili FJ, Heinzmann BM, Cunha MA, Brusque IC, Baldisserotto B, et al. Aloysia triphylla essential oil as additive in silver catfish diet: blood response and resistance against Aeromonas hydrophila infection. Fish Shellfish Immunol. (2017) 62:213–6. doi: 10.1016/j.fsi.2017.01.032
103. Bilen S, Kenanoglu ON, Terzi E, Ozdemir RC, Sonmez AY. Effects of tetra (Cotinus coggygria) and common mallow (Malva sylvestris) medicinal plants on growth performance and immune response in Gilthead Sea bream (Sparus aurata) and European Sea bass (Dicentrarchus labrax). Aquaculture. (2019) 512:734251. doi: 10.1016/j.aquaculture.2019.734251
104. Nafiqoh N, Zairin M, Lusiastuti A, Sarter S, Caruso D, Avarre J-C. Antimicrobial properties against Aeromonas hydrophila and immunostimulant effect on Clarias gariepinus of Piper betle, Psidium guajava, and Tithonia diversifolia plants. Aquacult Int. (2020) 28:1–13. doi: 10.1007/s10499-019-00439-6
105. Maiti S, Saha S, Jana P, Chowdhury A, Khatua S, Ghosh TK. Effect of dietary Andrographis paniculata leaf extract on growth, immunity, and disease resistance against Aeromonas hydrophila in Pangasianodon hypopthalmus. J Appl Aquacult. (2021) 1–25. doi: 10.1080/10454438.2021.1959861
106. Wang E, Chen X, Liu T, Wang K. Effect of dietary Ficus carica polysaccharides on the growth performance, innate immune response and survival of crucian carp against Aeromonas hydrophila infection. Fish Shellfish Immunol. (2021) 120:434–40. doi: 10.1016/j.fsi.2021.12.018
107. Fahimeh S, Milad A, Shekarabi SPH, Dawood MAO, Amin G. Effects of dietary Gracilaria persica on the intestinal microflora, thyroid hormones, and resistance against Aeromonas hydrophila in Persian sturgeon (Acipenser persicus). Ann Animal Sci. (2022) 22:1057–62. doi: 10.2478/aoas-2022-0003
108. Abdellatief SA, Abdel Rahman AN, Abdallah FD. Evaluation of immunostimulant activity of Spirulina platensis (Arthrospira platensis) Sage (Salvia officinalis) in Nile tilapia (Oreochromis niloticus). Zagazig Vet J. (2018) 46:25–36. doi: 10.21608/zvjz.2018.7621
109. Sultana B, Anwar F, Ashraf M. Effect of extraction solvent/technique on the antioxidant activity of selected medicinal plant extracts. Molecules. (2009) 14:2167–80. doi: 10.3390/molecules14062167
110. Cheesman M, Ilanko A, Blonk B, Cock I. Pharmacognosy reviews developing new antimicrobial therapies: are synergistic combinations of plant extracts/compounds with conventional antibiotics the solution. Pharmacogn Rev. (2017) 11:57–72. doi: 10.4103/phrev.phrev_21_17
111. Ali GI, Abeer E-KM, El-Shenway AM, Naena NA. Using of some phytobiotics and probiotics as promotors to cultured Nile Tilapia. Int J Fisheries Aquatic Stud. (2020) 8:148–59.
112. Harikrishnan R, Balasundaram C, Heo M-S. Potential use of probiotic-and triherbal extract-enriched diets to control Aeromonas hydrophila infection in carp. Dis Aquat Organ. (2010) 92:41–9. doi: 10.3354/dao02240
113. Abu-Elala N, Galal M, Abd-Elsalam R, Mohey-Elsaeed O, Ragaa N. Effects of dietary supplementation of Spirulina platensis and garlic on the growth performance and expression levels of immune-related genes in Nile tilapia (Oreochromis niloticus). J Aquacult Res Dev. (2016) 7:433–42. doi: 10.4172/2155-9546.1000433
Keywords: motile aeromonad septicemia (MAS), plant extract, anti-bacterial activity, innate immunity, disease resistance, synergistic
Citation: Abdul Kari Z, Wee W, Mohamad Sukri SA, Che Harun H, Hanif Reduan MF, Irwan Khoo M, Van Doan H, Wen Goh K and Seong Wei L (2022) Role of phytobiotics in relieving the impacts of Aeromonas hydrophila infection on aquatic animals: A mini-review. Front. Vet. Sci. 9:1023784. doi: 10.3389/fvets.2022.1023784
Received: 20 August 2022; Accepted: 15 September 2022;
Published: 06 October 2022.
Edited by:
Ghasem Rashidian, Tarbiat Modares University, IranReviewed by:
Seyed Pezhman Hosseini Shekarabi, Islamic Azad University, IranCopyright © 2022 Abdul Kari, Wee, Mohamad Sukri, Che Harun, Hanif Reduan, Irwan Khoo, Van Doan, Wen Goh and Seong Wei. This is an open-access article distributed under the terms of the Creative Commons Attribution License (CC BY). The use, distribution or reproduction in other forums is permitted, provided the original author(s) and the copyright owner(s) are credited and that the original publication in this journal is cited, in accordance with accepted academic practice. No use, distribution or reproduction is permitted which does not comply with these terms.
*Correspondence: Lee Seong Wei, leeseong@umk.edu.my; Khang Wen Goh, khangwen.goh@newinti.edu.my; Hien Van Doan, hien.d@cmu.ac.th