- 1Wise Owl Animal Hospital, Micronesian Exotic Specialty Services, Tamuning, GU, United States
- 2The Royal (Dick) School of Veterinary Studies and the Roslin Institute, University of Edinburgh, Edinburgh, United Kingdom
- 3Southeastern Cooperative Wildlife Disease Study, College of Veterinary Medicine, University of Georgia, Athens, GA, United States
- 4Warnell School of Forestry and Natural Resources, University of Georgia, Athens, GA, United States
Background: Guam, a United States of America (USA) island territory in the Pacific Ocean, is known to have large populations of ticks; however, it is unclear what the risk is to wildlife and humans living on the island. Dog (Canis familiaris), cat (Felis catus), and wild pig (Sus scrofa) sentinels were examined for ticks, and environmental sampling was conducted to determine the ticks present in Guam and the prevalence of tick-borne pathogens in hosts.
Methods and Results: From March 2019-November 2020, ticks were collected from environmental sampling, dogs, cats, and wild pigs. Blood samples were also taken from a subset of animals. A total of 99 ticks were collected from 27 environmental samples and all were Rhipicephalus sanguineus, the brown dog tick. Most ticks were collected during the dry season with an overall sampling success rate of 63% (95% CI: 42.4–80.6). 6,614 dogs were examined, and 12.6% (95% CI: 11.8–13.4) were infested with at least one tick. One thousand one hundred twelve cats were examined, and six (0.54%; 95% CI: 0.20–1.1) were found with ticks. Sixty-four wild pigs were examined and 17.2% (95% CI: 9.5–27.8) had ticks. In total, 1,956 ticks were collected and 97.4% of ticks were R. sanguineus. A subset of R. sanguineus were determined to be the tropical lineage. The other tick species found were Rhipicephalus microplus (0.77%), Amblyomma breviscutatum (0.77 %), and a Haemaphysalis sp. (0.51%). Blood samples from 136 dogs, four cats, and 64 wild pigs were tested using polymerase chain reaction (PCR) and DNA sequencing methods. Five different tick-borne pathogens with the following prevalences were found in dogs: Anaplasma phagocytophilum 5.9% (95% CI: 2.6–11.3); Anaplasma platys 19.1% (95% CI: 12.9–26.7); Babesia canis vogeli 8.8% (95% CI: 4.6–14.9); Ehrlichia canis 12.5% (95% CI: 7.5–19.3); Hepatozoon canis 14.7% (95% CI: 9.2–28.8). E. canis was detected in one cat, and no tick-borne pathogens were detected in wild pigs. Overall, 43.4% (95% CI: 34.9–52.1) of dogs had at least one tick-borne pathogen. Serological testing for antibodies against Ehrlichia spp. and Anaplasma spp. showed prevalences of 14.7% (95% CI: 9.2–28.8) and 31.6% (95% CI: 23.9–40), respectively.
Conclusion: Four different tick species were found in Guam to include a Haemaphysalis sp., which is a previously unreported genus for Guam. Dogs with ticks have a high prevalence of tick-borne pathogens which makes them useful sentinels.
Introduction
Vector-borne diseases are one of the greatest threats to human and animal health. After mosquitoes, ticks are the second most significant vector (1). Ticks are critical vectors of deadly pathogens worldwide and a variety of bacterial, protozoal, filarial, and viral pathogens have been identified in the microbial flora of ticks. Some examples of major tick-borne diseases (TBD) include babesiosis, theileriosis, cytauxzoonosis, hepatozoonosis, acanthocheilonemiasis, anaplasmosis, ehrlichiosis, typhus, spotted fevers, Lyme borreliosis, tularemia, bartonellosis, and viral encephalitis (2–4). Tick-borne disease provides good examples of the intersection between human, domestic animal, and wildlife health. Many of the estimated 900 different species of ticks are generalists and can easily attach to other hosts when their preferred host is unavailable (5). Similarly, tick-borne pathogens (TBP) may have their reservoir in one vertebrate species but incidentally infect another species (3). Tick-borne pathogens can cause morbidity in domestic animals and humans. Each year in the US alone, there are estimated to be about 300,000 cases of Lyme disease in humans (6) as well as increasing cases of other TBD such as ehrlichiosis and anaplasmosis (7). Tick-borne disease in cattle such as anaplasmosis can result in an 80% economic loss in some parts of the world (8). Ticks can transmit disease to wildlife (9). For example, in one study in southern Africa, domestic animals were found to be essential in the transmission of ticks and TBP to wildlife (10).
Infectious disease in general can influence the conservation of vulnerable species. The impact of TBD specifically in wildlife is not completely understood; however, many studies over the last decade have found evidence of TBP in wildlife populations worldwide to include: Rickettsia spp. and Anaplasma ovis in ticks from Cypriot mouflon (Ovis orientalis ophion) (11); Babesia spp. in gray kangaroos (Macropus giganteus) (12); Theileria, Anaplasma, Ehrlichia, and Babesia genera in wildlife in South Africa (13) and in Tanzania (14); Borrelia burgdorferi in brown bears (Ursus arctos) in Sweden (15); Borrelia spp. and Rickettsia spp. in Mongolian small mammals (16); novel species of Anaplasma and Ehrlichia organisms in Amazonian wildlife (17); and, a variety of TBP in raccoons (Procyon lotor), opossums (Didelphis virginiana), feral swine (Sus scrofa), and white-tailed deer (Odocoileus virginianus) in the USA (18).
As ticks are ubiquitous, TBD has consequently had a global impact on human and animal health. Guam (13° 26' 39.4944” N and 144° 47' 37.4352” E) isolated within Oceania has not been spared the impact of ticks. Guam is an island territory of the US located in Micronesia in the Western Pacific Ocean ~2,600 km east of the Philippine Islands and 6,370 km southwest of the Hawaiian Islands (19). It is a tropical island with two seasons (dry and rainy), moderate to high humidity, and consistent year-round warm temperatures averaging 29°C with a range between 21 and 32°C. The dry season is typically from January through June while July through December is the rainy season (20). The human population includes over 160,000 residents to include about 10,000 rotating active-duty military personnel with their families (21). In addition, over one million tourists per year visit the island. This results in frequent travel of people and animals into and out of Guam. The international population living in Guam frequently travels to many parts of Asia and could easily bring in “hitchhiker” ticks in luggage or on their person. An example of tick movement occurred in Connecticut in the US where Hyalomma truncatum from southern Africa was found on a person with recent travel history (22). In another case, the Asian longhorned tick (Haemaphysalis longicornis) became established in the US very recently after an accidental introduction from a traveler (23).
There are five known species of ticks that have been found in Guam: four hard-bodied ticks of the family Ixodidae and one soft body tick of the family Argasidae (24). These include: the swine tick, Amblyomma breviscutatum, the soft bodied tick found on migratory seabirds, Carios capensis (previously Ornithodoros capensis) (24), the mangrove monitor (Varanus indicus) lizard tick, Amblyomma squamosum first recorded by Kohls (25), the southern cattle tick, Rhipicephalus microplus, a common worldwide parasite of livestock (26), and the most abundant tick species seen on dogs, cats, and humans in Guam, the brown dog tick (Rhipicephalus sanguineus) (24).
Several studies on ticks and TBP have previously been conducted in Guam (Supplementary Table 1). At least three of the five identified species of ticks in Guam carry TBP such as Anaplasma spp., Ehrlichia spp., and Babesia spp. (27, 28); however, a full characterization of the diseases has not been conducted. Mehrpad et al. (29) found heavy burdens of ticks in Philippine deer (Rusa marianna) and found deer that were positive for Anaplasma platys, A. marginale, and the zoonotic A. phagocytophilum. Johnson et al. (28) identified two species of ticks and found the vectors positive for A. platys, A. marginale, Coxiella burnetii (the agent of Q fever), Babesia canis vogeli, and H. canis. Cleveland et al. (27) collected ticks from wild pigs and Philippine deer and found ticks positive for Rickettsia spp., of which certain species can be zoonotic.
Tick-borne diseases are a threat to Guam's domestic animals, humans, and wildlife populations, and include the small and vulnerable populations of native and migratory birds and one bat species. At least two of the tick species present in Guam are capable of hosting pathogens such as the agents of human ehrlichiosis and spotted fevers which can cause severe autoimmune conditions and organ failure (30). Anaplasma phagocytophilum has been found in ticks in Guam (29), and both birds and bats have the potential to become infected with this pathogen (31–33). The Guam rail (Gallirallus owstoni) is highly vulnerable and was only recently upgraded from extinct in the wild to critically endangered thanks to intensive breeding and reintroduction efforts over several decades (34). The Mariana fruit bat (Pteropus mariannus) does not fare much better with a critically endangered status in Guam due to only a few colonies of <50 individuals (35). In addition, Guam is a seasonal home to over 100 species of migrating sea birds with either transient or semi-permanent colonies as Micronesia is part of an oceanic migratory route (24).
Guam's biodiversity has been severely and, in many cases, irreversibly diminished mainly due to invasive species such as wild pigs, dogs, the brown tree snake, and rodents where numbers of individuals can be in the tens of thousands and even millions as in the case of the brown tree snake. Although several of these species have caused irreparable damage to Guam's wildlife, they do afford the opportunity for sentinel surveillance. Looking at sentinel animals can be an important step in understanding the impact of diseases, especially vector-borne diseases. Sentinels such as dogs, cats, or wild pigs have shown to be effective in extrapolating disease threats to native wildlife, humans, and other domestic animals (36), and sentinels are useful for determining abundance and species of ticks (37). Wild pigs, as a larger sentinel host, have more expansive home ranges, travel longer distances, and may visit more diverse habitats than smaller species. This increases their likelihood of acquiring a variety of pathogens (38). Wild pigs are used as sentinels for tuberculosis in New Zealand (39), and in the Pantanal in Brazil, the tick species Amblyomma sculptum from wild pigs is used to extrapolate disease risk in humans (40). Sentinels can often provide a better sampling method compared with dragging or flagging in areas where vegetation is variable, and the abundance of ticks is not uniformly distributed. Sentinel monitoring can detect newly introduced tick species and changes in ranges of a species (37). Finally, sampling of sentinel species such as domestic dogs and feral pigs is easier, both from a logistic and ethical standpoint, compared with most wildlife and humans (41).
With the importance of ticks and their realized and potential impacts on animal and human health, this project was conducted to improve our understanding of ticks and TBD in Guam. The specific goals of this research project are to identify tick species observed in Guam using samples collected from the environment (off-host) and animal hosts (dogs, cats, and wild pigs). We also tested hosts for selected TBP.
Materials and Methods
Sample collection was divided into off-host environmental sampling and animal sampling. Environmental sampling locations were selected to include a variety of vegetation types and different seasons; however, sampling sites and timing was by convenience, and not randomized. All attempts were made to ensure that the sampling procedure was uniform in distance and time. Sampling of animals was also convenience sampled. All ticks collected from the environment and animals were preserved in 95% ethanol. Ticks were kept at room temperature until shipping to the Southeastern Cooperative Wildlife Disease Study (SCWDS) at the University of Georgia (Athens, GA) for identification.
Environmental Tick Sample Collection
Environmental sampling was conducted from May 2019 to January 2020. Due to various limitations, the areas selected for sampling were based on ability to access the land area, favorable weather (no rain), and the availability of an experienced guide when needed to navigate the locations. The date, season, Global Positioning System (GPS) coordinates for the start of the sampling event, vegetation type, and number of ticks collected were recorded for every location sampled. Three of the five major landcover classes (rangeland, forest, and urban vegetation) of Guam as defined by Liu and Fischer (42) were sampled (Supplementary Table 2).
Ticks were flagged using a method that would sample leaf-litter vegetation and taller grasses (43, 44). A piece of white cotton flannel cloth ~0.5 m in width and 1 m in length with an attached 0.6-m wooden dowel and twine was used to collect ticks. The flag was dragged behind and to the sides of the researcher through vegetation elevating the flag up to 1 m as needed depending on the vegetation type. In total, each sampling event occurred for ~1 h and covered a 3 km roundtrip in distance (1.5 km each way). Flagging/dragging was stopped every 20 min (three times for each sampling event) to check the cloth for the presence of ticks by meticulously scanning both sides.
Domestic Animal Sample Collection
Samples of ticks and/or blood were collected from dogs or cats that were presented to a veterinary hospital in Guam. The inclusion criteria for this study were: (1) animals must have had at least one tick on them at the time of presentation, (2) the owner consented to be part of the study, and (3) for animals with a matching blood sample, they were already undergoing venipuncture for routine or illness related diagnostic testing. Data recorded for the individuals included in the study were physical address, season/date of collection, age, sex, species, tick burden, medical diagnosis based on laboratory and/or clinical exam findings, results of antibody testing (Ehrlichia and Anaplasma only), and results of PCR testing. Each sample was given an identifying number for usage for submission to the laboratory to anonymize the samples. The original data sheet with the above information was kept only by the lead author. Any identifying information already existed in the electronic medical record database system (EzyVet), which is password protected and administration rights are given only to the veterinarians of the clinic, one of which is the lead author.
Ticks were collected from March 2019 through November 2020 from dogs and cats. For dogs and cats, ticks were collected from different anatomical locations (most commonly ears, interdigital areas, axilla, ventral abdomen, dorsum, and face) where tick clusters were found.
Blood samples were collected from dogs and cats from July 2019 to November 2020. The samples were collected from both pets and stray animals that came to the hospital for routine or illness-related diagnostics and treatment. Animals were designated sick or healthy based on the presence or absence of clinical and/or laboratory abnormalities. Animals were designated as sick if they had one or more of the following abnormalities not attributable to another illness: fever, lethargy, anorexia, thrombocytopenia (<150,000 platelets/μl), anemia (<35% hematocrit), or any evidence of a potential coagulopathy such as petechiae, epistaxis, seizures, hematuria, melena, hematochezia, and hematemesis. Animals were safely restrained by Certified Veterinary Technicians and a blood sample was obtained from the jugular, cephalic, or saphenous veins. Blood was placed in both an ethylenediaminetetraacetic acid (EDTA) tube and in a tube without additive or with a serum separator to obtain serum after centrifugation. A small portion from the clinical sample obtained (~0.5–2 ml) of both the whole blood and serum sample was used for this study. Whole blood and sera were frozen at −20°C until shipping SCWDS for testing.
Wild Pig Sample Collection
Samples were only able to be collected opportunistically from wild pigs from November 2019 to January 2020. Trapping is done throughout the year by the USDA Wildlife Services across the island based on requests by private landowners or local mayors. All pigs were humanely euthanized by gunshot. Pigs were carefully checked for ticks with special emphasis on the ears, axilla, and interdigital areas.
Blood samples were taken as part of the USDA's routine testing of captured wild pigs in Guam. Serum and whole blood from wild pigs trapped and euthanized from November 2019 to January 2020 were provided for testing. Samples were obtained opportunistically based on the presence of successfully trapped pigs and depending on the location that the USDA teams were currently working. Blood samples (2 ml) were taken from the jugular vein and immediately placed in a plastic EDTA tube. The sample was kept on ice for 2–4 h before storage at −20°C.
In-house Diagnostic Laboratory Testing of Dogs
Whole blood or serum was tested for the presence of antibodies against Anaplasma spp. (A. platys and A. phagocytophilum) and Ehrlichia spp. (E. canis, E. ewingii, and E. chaffeensis) using the VETSCAN® Canine Anaplasma Rapid Test and VETSCAN® Canine Ehrlichia Rapid Test (Zoetis, Inc.).
Reference Diagnostic Laboratory Testing
All ticks were identified to genus or species based on morphology and classified by life stage and sex using identification keys from Keirans and Litwak (45) and Walker et al. (46). Any ticks that could not be identified due to damage were characterized using sequence analysis of the 16s rRNA gene as described by Lv et al. (47). A subset of R. sanguineus ticks were submitted for sequencing to determine lineage using a fragment of the 16S rRNA gene as described by Lv et al. (47).
Molecular assays were used to screen for infections with Babesia spp., E. canis, Hepatozoon spp., A. platys, and A. phagocytophilum. DNA was extracted from 100 microliters of whole blood using the DNAeasy Blood and Tissue kit, (Qiagen, Hilden, Germany). PCR was conducted using a BioRad DNA Engine Peltier Thermal Cycler (Bio-Rad Laboratories Incorporated, Foster City, CA) and published protocols (Supplementary Table 3). Additional details on laboratory methods are provided in Garrett et al. (48).
Statistical Analysis
IBM SPSS® Statistics was used for all statistical analysis. All proportions were determined along with 95% confidence interval under the non-parametric testing category using the Clopper Pearson Exact test with a 95% confidence level. A binary logistic regression univariable analysis was conducted at a 95% confidence level for the categorical variables assessed with presence of ticks (dependent variable) included season (dry/rainy) and vegetation landcover (rangeland/forest since urban only accounted for four sites). A binary logistic regression with the same confidence level was also done to assess any association between TBP prevalence and other variables. Categorical variables assessed with each positive pathogen status (dependent variable) were season and health status. A univariable analysis was conducted on both season and health status with the dependent variable separately for each of the five pathogens. From the SPSS output of the logistic regression models, beta coefficient, standard error, p-value, exp (b) (odds ratio-OR) and 95% confidence interval were recorded and evaluated for any associations among the variables. A p-value of < 0.05 was considered statistically significant.
Ethical Approval
This animal study was approved by the Royal (Dick) School of Veterinary Studies Veterinary Ethical Review Committee (VERC) of the University of Edinburgh for animal usage in clinical research with VERC Reference number 89.19. Written informed consent was obtained from the owners for the participation of their animals in this study.
Results
Environmental Tick Sampling
A total of 27 different locations of the three major vegetation landcover class were sampled (forest, rangeland, and urban) across the island of Guam. In total, 99 ticks were collected during 17 sampling events, a 63% success rate (95% CI: 42.4–80.6). Sixteen locations recovered ticks identified as R. sanguineus larvae while one location recovered an adult male R. sanguineus. The greatest number of sampling events and the most successful sampling events occurred in the dry season with a success in 11 of the 14 sampling events. while the rainy season resulted in only 6 of 13 sampling events being successful (Table 1). The most common landcover type that was sampled was the rangeland which included savanna complex vegetation (mostly sword grass over volcanic fields and coastal strand vegetation found along the beaches). Ticks were recovered in 8 of the 12 sampling events of this landcover type. The second greatest number of sites were included in the forest landcover category and was successful in 6 of 11 sampling events, The least sampled landcover class was urban but was successful in three of the four sampling events.

Table 1. Number of positive (ticks collected) environmental sampling events by different vegetation landcover classes and seasons, N = 27.
A binary logistic regression univariable analysis was used to determine if there was any relationship between a successful sampling event and season or vegetation landcover class. This analysis showed that the prevalence of positive drags was significantly higher in the dry season compared to the rainy season (p = 0.032, odds ratio 8.49 (95% CI: 1.19–60.29)]. No difference was noted for rangeland vs. forest (p = 0.73, odds ratio 1.4 (95% CI: 0.21–9.51).
Animal Tick Surveillance
In total, 1,956 ticks were collected and identified from 190 dogs, 11 wild pigs, and six cats. Most ticks (1,906, 97.4%) were identified as R. sanguineus. Thirty R. sanguineus were sequence confirmed to be tropical lineage (all were 100% identical to each other and 100% identical to a R. sanguineus (AY883868) specimen collected from southeast Asia). Fewer numbers of ticks were identified as R. microplus (15, 0.77%), A. breviscutatum (15, 0.77%), unidentified Rhipicephalus sp. (10, 0.51%), and a Haemaphysalis sp. (10, 0.51%) (Table 2).
Dogs were the most frequent sentinels sampled, and the ones contributing the greatest quantities of ticks. In total, 6,614 dogs were seen at the local animal hospital during the research project, and 833 (12.6%; 95% CI: 11.8–13.4) were infested with at least one tick. In contrast, 1,112 cats were seen during the same period, and only six (0.54%; 95% CI: 0.20–1.1) were infested with at least one tick. Sixty-four wild pigs were captured and 11 (17.2%; 95% CI: 9.5–27.8) were infested with at least one tick. Of ticks found on dogs, 99% (1,901/1,919) were R. sanguineus. For ticks from wild pigs, 100% were identified as A. breviscutatum. Although cats with ticks present were infrequent, the six cats sampled had four different species of ticks including one each with A. breviscutatum, Haemaphysalis sp., and R. microplus, and two cats with R. sanguineus. Because cats are an unusual host for R. microplus, one specimen was sequence confirmed using the same lineage PCR protocol for R. sanguineus (100% identical to an R. microplus (KM246883) collected from Malaysia).
Animal Blood Tick-Borne Pathogens Testing
Serum and whole blood samples were obtained from 136 dogs, 64 wild pigs, and four cats. For wild pigs, whole blood was tested for the presence of Babesia spp. and A. phagocytophilum. All samples were negative. For cats, only four were tested for all TBP, and one sample was positive for E. canis. This cat had one R. microplus tick attached and was excluded from the statistical analysis because of the small sample size.
The prevalence and confidence intervals for the following TBP found in dogs are shown in Table 3. Antibodies against Anaplasma spp. was the most common positive test with almost 1/3 of samples testing positive. For PCR testing, A. phagocytophilum, A. platys, B. canis vogeli, E. canis, H. canis were detected with A. platys being the most common TBP found with a prevalence of 19.1%. Overall, 43.4% (59/136; 95% CI: 34.9–52.1) of dogs had at least one TBP (Table 4) while almost 15% of dogs had co-infections with two or more TBP.
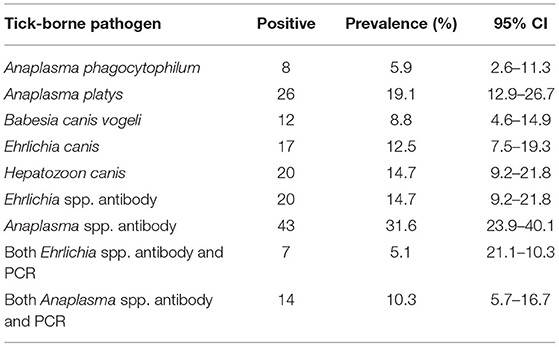
Table 3. Overall prevalence for each tick-borne pathogen found in the blood samples of dogs via molecular and antibody testing (Ehrlichia spp. and Anaplasma spp. only), N = 136.
Dog and cat sampling was conducted in both the rainy and dry seasons from both sick and healthy individuals. Table 5 shows the prevalence of each TBP by season and health status. For serology, antibodies against Anaplasma and Ehrlichia spp. were more prevalent in sick dogs, and antibodies against Anaplasma spp. were more prevalent in the dry season while antibodies against Ehrlichia spp. were more prevalent in the rainy season. For PCR testing, A. phagocytophilum, A. platys, B. canis vogelii, and H. canis were more prevalent in the dry season while E. canis was more prevalent in the rainy season. A. platys, B. canis vogeli, E. canis, and H. canis were also more prevalent in sick dogs, but A. phagocytophilum was more prevalent in healthy dogs although sample size was smallest for this pathogen.
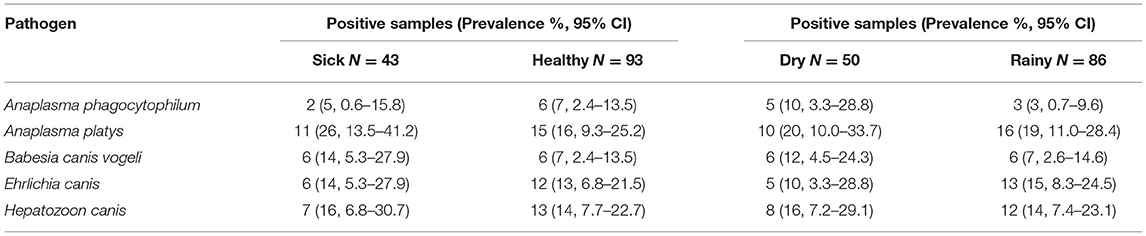
Table 5. Prevalence for each tick-borne pathogen found in blood samples of dogs by season and health status.
Results of a binary logistic regression univariable analysis to determine any relationship between TBP positivity for each of the five pathogens, season, and health status are listed in Tables 6, 7. The likelihood of infection was greater in the dry season for A. phagocytophilum, A. platys, B. canis vogeli, and H. canis. In contrast, E. canis, antibodies against Ehrlichia spp., and antibodies against Anaplasma spp. indicated that the likelihood of infection was lower in the dry season. The OR for sick health status was greater than one for antibodies against Anaplasma spp., A. platys, B. canis vogeli, antibodies against Ehrlichia spp., E. canis, and H. canis indicating blood samples were more likely to be positive if the patient was sick. The OR was less than one for A. phagocytophilum. Results with statistical significance (p < 0.05) were A. platys positivity with a greater likelihood of being positive with an Anaplasma spp. antibody test; Anaplasma spp. antibody test with a greater likelihood of being positive in a sick animal; Anaplasma spp. antibody test with a greater likelihood of being positive with a positive molecular test for A. platys; and, Ehrlichia spp. antibody test with a much greater likelihood of being positive with a positive E. canis molecular test.
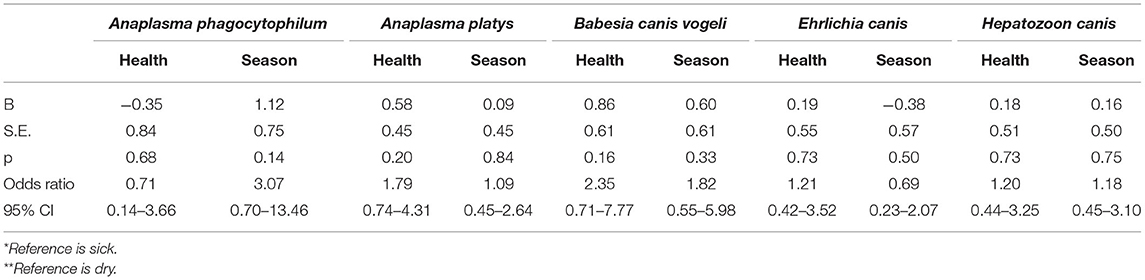
Table 6. Relationship between PCR positivity for each tick–borne pathogen with health* status and season** (univariable analysis).
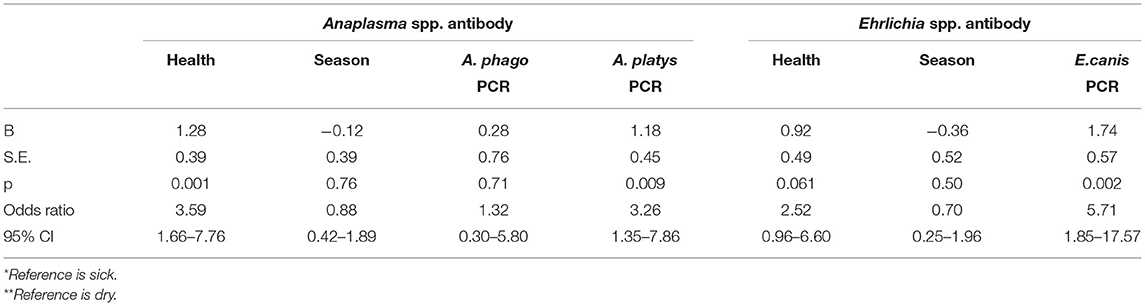
Table 7. Relationship between positivity for antibodies against Ehrlichia spp. or Anaplasma spp. with health*, season**, or corresponding positive PCR test (univariable analysis).
Discussion
This study used two different methods to collect ticks in Guam. Environmental sampling was not nearly as fruitful and efficient as animal sampling for ticks. In this study, ticks were successfully recovered using environmental sampling techniques in 63% of events, but only low numbers of one tick species were found. The season, daily weather pattern, and location all contributed to the success of a given sampling event. Thus, it would be difficult to discover a newly introduced species of tick via environmental sampling methods unless the tick species had already become widespread. Interestingly, R. sanguineus is usually found closer to urban dwellings (49) but it was widespread in Guam. Although rural areas have more deer, wild pigs, water buffalo, and cattle which host R. microplus, large populations of feral and stray dogs may be sufficient to maintain R. sanguineus in these rural areas. As with most tick species, there were specific habitats where R. sanguineus was detected with environmental sampling. The southern part of Guam, which has large regions of rangeland landcover (savanna complex habitat specifically) was where R. sanguineus was most often recovered. This could be because this vegetation type is easier to conduct dragging and flagging compared with rockier and heavily forested areas, or this could be a preferred habitat type for this tick.
The dry season, which includes the months from January through June, was the most common season for recovering R. sanguineus from the environment. Although it is called the dry season, rain still falls regularly; however, this will vary from year to year. Flagging and dragging success is dependent on the weather for that day, and every effort was made to only conduct sampling when there was no recent overnight rain. Sampling is difficult in the rain since it was harder to acquire ticks on the cotton flag when it was soaked. The likelihood of finding ticks (positive sampling event) was roughly eight times greater in the dry season compared to the rainy season (p = 0.032).
In contrast, collection of ticks from animals was much more successful in regard to numbers of ticks and species. Dogs, wild pigs, and some cats are effective sentinels since they travel long distances and roam in both isolated wildlife habitat and near human dwellings. Animal tick infestations are also not dependent entirely on weather and terrain (37, 50). From these three sentinel species, four different tick species were found. Rhipicephalus sanguineus was the most prevalent tick species found, which is unsurprising as it is the most widespread tick in Guam and can live in a variety of habitats from tropical forests to urban dwellings. Rhipicephalus sanguineus is predominantly an ectoparasite of the domestic dog and is believed to have been involved in the transmission of pathogens for over 2,000 years (51); however, it can attach and feed on other hosts such as cats, various birds, rodents, and humans (49). The most important concern with R. sanguineus is its role as a vector of numerous pathogens (30, 52–56). There are two clades or lineages, temperate and tropical, which are significant in that these lineages are associated with different pathogens (57). In Guam, we only detected the tropical lineage of R. sanguineus which is not surprising given how common E. canis was in dogs and this lineage is more likely to transmit E. canis (58). However, only a limited number of ticks were genotyped so additional work is needed to determine if both lineages are present in Guam.
Rhipicephalus microplus, the southern cattle tick, was the second most common tick found. Annual losses due to the impact of this tick species particularly in tropical countries is estimated to be 22–30 billion US dollars per year (59). In Guam, where there is no commercial livestock farming, R. microplus ticks are mostly found on Philippine deer, cattle, water buffalo, and goats (24, 27, 29). Rhipicephalus microplus is a one-host tick (8) so it was unusual to find this tick on dogs and cats as they are not the typical host; however, several studies have reported finding this tick on dogs, cats, and wild carnivores (60–64). Rhipicephalus microplus can host various TBP. Cleveland et al. (27) did find “Candidatus Rickettsia senegalensis,” of the R. felis cluster, a potential human pathogen, in R. microplus collected from Philippine deer in Guam. This pathogen has been reported most in cat fleas (Ctenocephalides felis orientis), but other arthropods such as ticks can host “Candidatus Rickettsia senegalensis” (65–67).
Several immature specimens of a Haemaphysalis sp. were detected which represents the first detection of this genus on Guam. This tick belongs to a genus that includes at least 168 different species. It is a small, inornate tick that has a three-host life cycle. Adults are found in both domestic animals and wildlife while immature stages prefer to parasitize small mammals and birds (68). Immature stages are difficult to identify to species and many species lack sufficient descriptions, so species identification was not conducted; however, they are morphologically and genetically distinct from H. longicornis, a species native to East Asia of recent notoriety because it is invasive in the United States (23, 69) and numerous countries in Oceania (70, 71). Assuming this tick has been introduced, where this Haemaphysalis sp. originally came from is unknown; however, Guam frequently has travelers, sometimes with companion animals, from East Asia where numerous Haemaphysalis spp. are endemic. Another possibility is that a migratory bird could have introduced this species as this genus has been found on migratory birds in Asia (72). With the introduction of a non-native tick species, the risk of a new TBD increases. A concerning finding is that multiple Haemaphysalis spp. in native and introduced areas can transmit numerous pathogens (73, 74).
Due to their close association with humans and interactions with wildlife, domestic canines can act as sentinels for vector and/or pathogen exposure for humans and other wildlife species (75, 76). Sampling can be easily done as part of veterinary hospital visits or as part of vaccine and preventive care campaigns in more rural areas (41). Dogs may potentially be a reservoir of certain infectious disease and be a source of infection for ticks (77). For zoonotic pathogens, dogs may serve as effective sentinels (78, 79). For example, seroprevalence of Borrelia burgdorferi in dogs may be a sensitive marker of human risk for Lyme disease as dogs are infected at a higher frequency (80, 81).
Numerous TBP were detected in blood samples of dogs and cats including A. phagocytophilum, A. platys, B. canis vogeli, E. canis, and H. canis. This is the first time these pathogens have been identified molecularly in dogs and one cat in Guam. Anaplasma platys is a mostly canine pathogen that is transmitted by R. sanguineus. Anaplasma phagocytophilum is a known zoonotic agent and infects a wide variety of species to include birds, cats, rodents, and deer species, including Philippine deer in Guam (29, 82). Babesia spp. are intraerythrocytic protozoans grouped in the Piroplasmidae family, related to Theileria spp. Babesia canis, a large piroplasm, infects dogs and is subdivided into subspecies based on unique biology and tick vectors. Babesia canis vogeli is transmitted by R. sanguineus (83, 84). Ehrlichia are gram-negative, obligate intracellular, pleomorphic organisms of the Rickettsia order. Ehrlichia canis is the agent of canine monocytic ehrlichiosis (85). Hepatozoon is a genus of protozoal parasites which includes over 300 different species. Hepatozoon canis is of primary concern for dogs (86) with infections ranging from subclinical and asymptomatic to severe (87). Anaplasma platys was the most common TBP found. This is consistent with what has been anecdotally noted in the population of dogs seen at animal hospitals in Guam. The antibody assays used in this study can cross-react with several species within these genera, but there was a statistically significant correlation between antibody results and positive corresponding molecular testing for E. canis and A. platys suggesting that serologic data corresponds with these two species.
Nearly half of the dogs (43.4%) tested were positive for at least one TBP. Another 14.7% had infections with two or three TBP. Although most pathogens were more likely to be found in the dry season and in sick individuals, none of the relationships were statistically significant. This is consistent with what is seen in the animal hospitals in Guam. Not all animals that are sick with a TBP will test positive, and many animals are positive for a TBP and lack disease. In fact, clinically ill animals often have other comorbidities that may make illness due to TBP opportunistic. A larger sample size might elucidate any relationships present although this may not be important in monitoring prevalence of TBP for a tick monitoring program.
The detection of A. phagocytophilum in dogs is an interesting finding as it confirms the recent detection of this pathogen in Philippine deer from Guam (29) despite the typical vector (Ixodes spp.) not being detected on these hosts in Guam. Although A. phagocytophilum is usually associated with an Ixodes spp. (72), there are competent vectors in other tick genera. The most common tick on deer in Guam was R. microplus and although this tick was rarely found on dogs in this study, R. microplus can transmit A. phagocytophilum (88). The predominant ticks on dogs were R. sanguineus, and several studies have detected A. phagocytophilum in R. sanguineus (89–91). In addition, H. longicornis in the Republic of Korea (South Korea) (92) and United States (74) were positive for A. phagocytophilum. There are also several Asian species of Haemaphysalis (e.g., H. megaspinosa, H. douglasii, and H. japonica) have been PCR positive for A. phagocytophilum (72).
Anaplasma phagocytophilum has also been reported from some Amblyomma spp. (e.g., in A. flavomaculatum from various imported African reptile species in Poland) (93) and in A. cajennense from dogs in Brazil) (91). In Europe, wild pigs are a host of A. phagocytophilum (94); however, A. phagocytophilum was not found in wild pigs in Guam in a recent study (27) nor in the current study. Because of the lack of A. phagocytophilum in pigs and a lack of Amblyomma spp. on deer or dogs, A. breviscutatum is not suspected to be a vector of A. phagocytophilum. Although wild pigs are a reservoir of other pathogens such as leptospirosis, there is no evidence from this study that wild pigs are a reservoir of TBP in Guam as none were positive for Babesia spp. or A. phagocytophilum. Nonetheless, in 2015, there was a reported case of infestation of humans by larval A. breviscutatum (95) so determining its vector competence for zoonotic pathogens, especially Rickettsia spp., is important. A previous study found R. amblyommatis, a potentially zoonotic pathogen, from A. breviscutatum from wild pigs in Guam (27). Wild pigs are managed by the USDA in Guam, so this is one way to easily monitor this species in Guam.
Several of the TBP that have been found in the current and previous studies in Guam are zoonotic or have the potential to cause illness in humans (A. phagocytophilum, C. burnetii, R. amblyommatis, and R. felis). The most significant is A. phagocytophilum, which causes human granulocytotropic anaplasmosis (HGA). The other Anaplasmataceae reported in Guam, A. platys, may also infect people although its exact role in disease is not completely understood. Maggi et al. (96) reported a symptomatic veterinarian with seizures and migraines who was positive for A. platys, but this patient was also infected with Bartonella henselae and “Candidatus Mycoplasma haematoparvum.” There was another report of two symptomatic people in the US who, along with their dog, were infected with A. platys, E. chaffeensis, and E. ewingii (97). In Venezuela, there were two case reports of people with symptoms such as headaches, myalgia, joint pain, and fever, and A. platys was the only TBP detected in their blood (98). In all cases, the humans lived or worked closely with dogs. Ehrlichia canis can also cause illness in humans with similar symptoms to E. ewingii or E. chaffeensis (99); however, E. canis is more likely asymptomatic as noted in a study of blood bank samples where donors had no symptoms of disease but 3.6% of people had molecular and serological evidence of E. canis infection (100).
Of note, there were several limitations to this study. The sample size for off-host sampling was small, and only revealed one statistically significant conclusion. A more robust sample size over a longer period and through multiple seasons would have been more informative. Sampling bias may be present as sampling of both animals and the environment was not randomized. It is recommended that the study be expanded upon by having a more structured means of sampling both the island and the animals. Another limitation of this study is that the ticks themselves were not tested for pathogens, and animals were not tested for other TBP such as Rickettsia spp. that have been found in Guam in previous studies.
Conclusion
Second only to mosquito-borne pathogens, TBP and their associated diseases are a significant threat to humans, domestic animals, and wildlife worldwide. Sentinel surveillance can be a useful tool in helping to understand the risk of disease from TBP in humans and wildlife (36). From this study and previous studies in Guam (27, 29) dogs and deer may be the most useful mammalian sentinels thus far for determining tick species and TBP prevalence on the island. In fact, the current sentinel surveillance discovered a novel tick species in Guam and a high prevalence of TBP in dogs with almost half of all dogs with one or more ticks infected with at least one TBP. Consistent and on-going sentinel surveillance is essential to detect new ticks and novel pathogens in Guam. Other wildlife species, including birds and reptiles, should also be included as sentinel species for future studies to increase the diversity of ticks that may be detected.
Although Guam is a seemingly isolated island in the middle of the Pacific Ocean, it has an outsized influence in this area and perhaps around the world with large numbers of transitory citizens, many tourists, and rotating military and other government personnel. Almost all residents have family members from outside the island to include the USA, Asia, Russia, and other Pacific Islands. Air travel is very frequent in this population to include movement of pets, and consequently, disease movement. Therefore, understanding the disease threats present in Guam is not only important to the current population but also to other parts of the world.
Data Availability Statement
The raw data supporting the conclusions of this article will be made available by the authors, without undue reservation.
Ethics Statement
The animal study was reviewed and approved by Royal (Dick) School of Veterinary Studies Veterinary Ethical Review Committee (VERC) of the University of Edinburgh for animal usage in clinical research with VERC Reference number 89.19. Written informed consent was obtained from the owners for the participation of their animals in this study.
Author Contributions
GW was responsible for conception of the study, manuscript writing and revisions, data analysis, and interpretation of the data. NA was responsible for conceptualization, supervision, and manuscript review and editing. AT was responsible for tick identification and review of the manuscript. KG was responsible for molecular testing and analysis of blood pathogen data and review of the manuscript. MY was responsible for study design, analysis and interpretation of data, and writing of the manuscript. All authors contributed to the article and approved the submitted version.
Funding
Experiment Crowd Funding Platform for Scientific Research and the Southeastern Cooperative Wildlife Disease Study at the University of Georgia.
Conflict of Interest
The authors declare that the research was conducted in the absence of any commercial or financial relationships that could be construed as a potential conflict of interest.
Publisher's Note
All claims expressed in this article are solely those of the authors and do not necessarily represent those of their affiliated organizations, or those of the publisher, the editors and the reviewers. Any product that may be evaluated in this article, or claim that may be made by its manufacturer, is not guaranteed or endorsed by the publisher.
Acknowledgments
The authors would like to thank Dr. Joel Joseph and the entire staff of Wise Owl Animal Hospital in Guam for sample collection and processing.
Supplementary Material
The Supplementary Material for this article can be found online at: https://www.frontiersin.org/articles/10.3389/fvets.2021.803424/full#supplementary-material
Abbreviations
TBD, tick-borne disease(s); TBP, tick-borne pathogen(s).
References
1. Brites-Neto J, Duarte KMR, Martins TF. Tick-borne infections in human and animal population worldwide. Vet World. (2015) 8:301–15. doi: 10.14202/vetworld.2015.301-315
2. Boulanger N, Boyer P, Talagrand-Reboul E, Hansmann Y. Ticks and tick-borne diseases Tiques et maladies vectorielles à tiques. Med Mal Infect. (2019) 49:87–97. doi: 10.1016/j.medmal.2019.01.007
3. Dantas-Torres F, Chomel BB, Otranto D. Ticks and tick-borne diseases: a one health perspective. Trends Parasitol. (2012) 28:37–46. doi: 10.1016/j.pt.2012.07.003
4. Inci A, Yildirim A, Duzlu O, Doganay M, Aksoy S. Tick-borne diseases in Turkey: a review based on One Health perspective. PLoS Negl Trop Dis. (2016) 10:e0005021. doi: 10.1371/journal.pntd.0005021
5. Estrada-Peña A. Ticks as vectors: taxonomy, biology and ecology. Revue Scientifique et Technique. (2015) 34:53–65. doi: 10.20506/rst.34.1.2345
6. Centers for Disease Control Prevention (CDC). Data and Surveillance | Lyme Disease. CDC (2020). Available online at: https://www.cdc.gov/lyme/datasurveillance/index.html?CDC_AA_refVal=https%3A%2F%2F%2Flyme%2Fstats%2Findex.html (accessed April 13, 2020).
7. Lee-Lewandrowski E, Chen Z, Branda J, Baron J, Kaufman HW. Laboratory blood-based testing for non-Lyme disease tick-borne infections at a National Reference Laboratory. Am J Clin Pathol. (2020) 153:139–45. doi: 10.1093/ajcp/aqz139
8. Senbill H, Hazarika LK, Baruah A, Borah DK, Bhattacharyya B, Rahman S. Life cycle of the southern cattle tick, Rhipicephalus (Boophilus) microplus Canestrini 1888 (Acari: Ixodidae) under laboratory conditions. Syst Appl Acarol. (2018) 23:1169–79. doi: 10.11158/saa.23.6.12
9. Gizzarelli M, Foglia Manzillo V, Ciuca L, Morgoglione ME, el Houda Ben Fayala N, Cringoli G, et al. Simultaneous detection of parasitic vector borne diseases: a robust cross-sectional survey in hunting, stray and sheep dogs in a Mediterranean area. Front Vet Sci. (2019) 6:1–9. doi: 10.3389/fvets.2019.00288
10. Espinaze MPA, Hellard E, Horak IG, Cumming GS. Domestic mammals facilitate tick-borne pathogen transmission networks in South African wildlife. Biol Conserv. (2018) 221:228–36. doi: 10.1016/j.biocon.2018.03.017
11. Ioannou I, Sandalakis V, Kassinis N, Chochlakis D, Papadopoulos B, Loukaides F, et al. Tick-borne bacteria in mouflons and their ectoparasites in Cyprus. J Wildl Dis. (2011) 47:300–6. doi: 10.7589/0090-3558-47.2.300
12. Dawood K, Morgan J, Busfield F, Srivastava M, Fletcher T, Sambono J, et al. Observation of a novel Babesia spp. in eastern grey kangaroos (Macropus giganteus) in Australia. Int J Parasitol. (2013) 2:54–61. doi: 10.1016/j.ijppaw.2012.12.001
13. Berggoetz M, Schmid M, Ston D, Wyss V, Chevillon C, Pretorius A-M, et al. Tick-borne pathogens in the blood of wild and domestic ungulates in South Africa: interplay of game and livestock. Ticks Tick Borne Dis. (2014) 5:166–75. doi: 10.1016/j.ttbdis.2013.10.007
14. Kim TY, Kwak YS, Kim JY, Nam S-H, Lee I-Y, Mduma S, et al. Prevalence of tick-borne pathogens from ticks collected from cattle and wild animals in Tanzania in 2012. Korean J Parasitol. (2018) 56:305–8. doi: 10.3347/kjp.2018.56.3.305
15. Paillard L, Jones KL, Evans AL, Berret J, Jacquet M, Lienhard R, et al. Serological signature of tick-borne pathogens in Scandinavian brown bears over two decades. Parasit Vectors. (2015) 8:398. doi: 10.1186/s13071-015-0967-2
16. Pulscher LA, Moore TC, Caddell L, Sukhbaatar L, von Fricken ME, Anderson BD, et al. A cross-sectional study of small mammals for tick-borne pathogen infection in northern Mongolia. Infect Ecol Epidemiol. (2018) 8:1–7. doi: 10.1080/20008686.2018.1450591
17. Soares HS, Marcili A, Barbieri ARM, Minervino AHH, Malheiros AF, Gennari SM, et al. Novel Anaplasma and Ehrlichia organisms infecting the wildlife of two regions of the Brazilian Amazon. Acta Trop. (2017) 174:82–7. doi: 10.1016/j.actatropica.2017.07.006
18. Castellaw AH, Chenney EF, Varela-Stokes AS. Tick-borne disease agents in various wildlife from Mississippi. Vector-Borne Zoonotic Dis. (2011) 11:439–42. doi: 10.1089/vbz.2009.0221
19. Karolle B. Guampedia. Geography of Guam. (2020). Available online at: https://www.guampedia.com/geography-of-guam (accessed Apr 13, 2020).
20. Guampedia. About Guam (2019). Available online at: https://www.guampedia.com/about-guam (accessed December 12, 2019).
21. United States Census. Island Area: Guam (2010). Available online at: https://www.census.gov/population/www/cen2010/island_area/guam.html (accessed April 13, 2020).
22. Molaei G, Andreadis TG, Anderson JF III. KCS An exotic hitchhiker: a case report of importation into Connecticut from Africa of the human parasitizing tick, Hyalomma truncatum (Acari: Ixodidae). J Parasitol. (2018) 104:302–5. doi: 10.1645/18-13
23. Rainey T, Occi JL, Robbins RG, Egizi A. Discovery of Haemaphysalis longicornis (Ixodida: Ixodidae) Parasitizing a sheep in New Jersey, United States. J Med Entomol. (2018) 55:757–9. doi: 10.1093/jme/tjy006
24. Vander Velde N, Vander Velde B. Known and potential ticks and tick-borne pathogens of Micronesia. Micronesica. (2013) 1:1–25. Retrieved from: https://micronesica.org/sites/default/files/2013-01_vander_velde_micronesian_ticks-1.pdf
25. Kohls GM. Notes on the ticks of Guam with the description of Amblyomma squamosum n.sp. (Acarina: Ixodidae). J Parasitol. (1953) 39:264–7. doi: 10.2307/3273948
26. Busch JD, Stone NE, Nottingham R, Araya-Anchetta A, Lewis J, Hochhalter C, et al. Widespread movement of invasive cattle fever ticks (Rhipicephalus microplus) in southern Texas leads to shared local infestations on cattle and deer. Parasites Vectors. (2014) 7:188. doi: 10.1186/1756-3305-7-188
27. Cleveland CA, Swanepoel L, Box EK, de Nicola A, Yabsley MJ. Rickettsia species in ticks collected from wild pigs (Sus scrofa) and Philippine deer (Rusa marianna) on Guam, Marianna Islands, USA. Acta Trop. (2019) 194:89–92. doi: 10.1016/j.actatropica.2019.03.010
28. Johnson J, Vice D, Nunn P, Gutierrez T, Reeves WK, Rabago R, et al. Invertebrate vectors, parasites, and rickettsial agents in Guam. Micronesica. (2012) 43:225–36. Retrieved from: https://www.micronesica.org/sites/default/files/10_micronesica_432_reeves.pdf
29. Mehrpad S, Cleveland CA, Denicola A, Dubey JP, Yabsley MJ. Survey for selected pathogens in Philippine deer (Rusa marianna) from Guam, Marianna Islands, USA. Vet Parasitol Regional Stud Rep. (2018) 11:36–40. doi: 10.1016/j.vprsr.2017.11.010
30. Stoffel RT, Mcclure JC, Butcher MM, Johnson GC, Roland W, Cheng C, et al. Experimental infection of Rhipicephalus sanguineus with Ehrlichia chaffeensis. Vet Microbiol. (2014) 172:334–8. doi: 10.1016/j.vetmic.2014.04.017
31. Hildebrandt A, Franke J, Meier F, Sachse S, Dorn W, Straube E. The potential role of migratory birds in transmission cycles of Babesia spp., Anaplasma phagocytophilum, and Rickettsia spp. Ticks Tick Borne Dis. (2010) 1:105–7. doi: 10.1016/j.ttbdis.2009.12.003
32. Hornok S, Szoke K, Meli ML, Sándor AD, Görföl T, Estók P, et al. Molecular detection of vector-borne bacteria in bat ticks (Acari: Ixodidae, Argasidae) from eight countries of the Old and New Worlds 06 Biological Sciences 0604 Genetics. Parasites Vectors. (2019) 12:1–7. doi: 10.1186/s13071-019-3303-4
33. Sparagano O, George D, Giangaspero A, Špitalská E. Arthropods and associated arthropod-borne diseases transmitted by migrating birds. the case of ticks and tick-borne pathogens. Vet Parasitol. (2015) 213:61–6. doi: 10.1016/j.vetpar.2015.08.028
34. IUCN Red List,. Species Recoveries Bring Hope Amidst the Biodiversity Crisis (2019). Available online at: https://www.iucn.org/news/species/201912/species-recoveries-bring-hope-amidst-biodiversity-crisis-iucn-red-list (accessed April 13, 2020).
35. United States Fish Wildlife Service (USFWS),. Wildlife & Habitat - Guam - U.S. Fish Wildlife Service (2018). Available online at: https://www.fws.gov/refuge/Guam/wildlife_and_habitat (accessed April 13, 2020).
36. Halliday JEB, Meredith AL, Knobel DL, Shaw DJ, Bronsvoort BM de C, Cleaveland S. A framework for evaluating animals as sentinels for infectious disease surveillance. J Royal Soc Interface. (2007) 4:973–84. doi: 10.1098/rsif.2007.0237
37. Merrill MM, Boughton RK, Lord CC, Sayler KA, Wight B, Anderson WM, et al. Wild pigs as sentinels for hard ticks: a case study from south-central Florida. Int J Parasitol. (2018) 7:161–70. doi: 10.1016/j.ijppaw.2018.04.003
38. Esser HJ, Foley JE, Bongers F, Allen Herre E, Miller MJ, Prins HHT, et al. Host body size and the diversity of tick assemblages on Neotropical vertebrates. Int J Parasitol. (2016) 5:295–304. doi: 10.1016/j.ijppaw.2016.10.001
39. Nugent G, Yockney IJ, Whitford EJ, Cross ML. Assessing the effectiveness of tuberculosis management in brushtail possums (Trichosurus vulpecula), through indirect surveillance of Mycobacterium bovis infection using released sentinel pigs. Vet Med Int. (2014) 2014:1–13. doi: 10.1155/2014/361634
40. Ramos V do N, Piovezan U, Franco AHA, Osava CF, Herrera HM, Szabó MPJ. Feral pigs as hosts for Amblyomma sculptum (Acari: Ixodidae) populations in the Pantanal, Mato Grosso do Sul, Brazil. Exp Appl Acarol. (2014) 64:393–406. doi: 10.1007/s10493-014-9832-9
41. Bryan HM, Darimont CT, Paquet PC, Ellis JA, Goji N, Gouix M, et al. Exposure to infectious agents in dogs in remote coastal British Columbia: possible sentinels of diseases in wildlife and humans. Canad J Vet Res. (2011) 5:11–7.
42. Liu Z, Fischer L Guam Vegetation Mapping Using Very High Spatial Resolution Imagery: Methodology. McClellan, CA (2006). Available online at: https://www.fs.usda.gov/Internet/FSE_DOCUMENTS/fsbdev3_046054.pdf. (accessed April 14, 2020).
43. Dantas-Torres F, Lia RP, Capelli G, Otranto D. Efficiency of flagging and dragging for tick collection. Exp Appl Acarol. (2013) 61:119–27. doi: 10.1007/s10493-013-9671-0
44. Rulison EL, Kuczaj I, Pang G, Hickling GJ, Tsao JI, Ginsberg HS. Flagging versus dragging as sampling methods for nymphal Ixodes scapularis (Acari: Ixodidae). J Vector Ecol. (2013) 38:163–7. doi: 10.1111/j.1948-7134.2013.12022.x
45. Keirans JE, Litwak TR. Pictorial key to the adults of hard ticks, family Ixodidae (Ixodida: Ixodoidea), east of the Mississippi River. J Med Entomol. (1989) 26:435–48. doi: 10.1093/jmedent/26.5.435
46. Walker Jane B, (Jane Brotherton), Keirans, James E, Horak I. The Genus Rhipicephalus (Acari, Ixodidae): A Guide to the Brown Ticks of the World. Keirans JE, Horak I, editors. Cambridge: Cambridge University Press (2000) doi: 10.1017/CBO9780511661754
47. Lv J, Wu S, Zhang Y, Zhang T, Feng C, Jia G, et al. Development of a DNA barcoding system for the Ixodida (Acari: Ixodida). Mitochondrial DNA. (2014) 25:142–9. doi: 10.3109/19401736.2013.792052
48. Garrett KB, Hernandez SM, Balsamo G, Barron H, Beasley JC, Brown JD, et al. Prevalence, distribution, and diversity of cryptic piroplasm infections in raccoons from selected areas of the United States and Canada. Int J Parasitol. (2019) 9:224–33. doi: 10.1016/j.ijppaw.2019.05.007
49. Dantas-Torres F. Biology and ecology of the brown dog tick, Rhipicephalus sanguineus. Parasites Vectors. (2010) 3:1–11. doi: 10.1186/1756-3305-3-26
50. Hernández FA, Parker BM, Pylant CL, Smyser TJ, Piaggio AJ, Lance SL, et al. Invasion ecology of wild pigs (Sus scrofa) in Florida, USA: The role of humans in the expansion and colonization of an invasive wild ungulate. Biol Invasions. (2018) 20:1865–80. doi: 10.1007/s10530-018-1667-6
51. Otranto D, Huchet J-B, Giannelli A, Callou C, Dantas-Torres F. Enigma of the dog mummy from Ancient Egypt and the origin of ‘Rhipicephalus sanguineus.’ Parasites Vectors. (2014) 7:1171. doi: 10.1186/1756-3305-7-2
52. Banović P, Díaz-Sánchez AA, Galon C, Simin V, Mijatović D, Obregón D, et al. Humans infested with Ixodes ricinus are exposed to a diverse array of tick-borne pathogens in Serbia. Ticks Tick Borne Dis. (2021) 12:101609. doi: 10.1016/j.ttbdis.2020.101609
53. Chao L-L, Shih C-M. Molecular detection of Rickettsial infection in Rhipicephalus sanguineus ticks ectoparasitized on dogs in Kaohsiung City of Southern Taiwan. Int J Infect Dis. (2019) 9:141. doi: 10.1016/j.ijid.2018.11.344
54. Ravi A, Ereqat S, Al-Jawabreh A, Abdeen Z, Abu Shamma O, Hall H, et al. Metagenomic profiling of ticks: identification of novel rickettsial genomes and detection of tick-borne canine parvovirus. PLoS Negl Trop Dis. (2019) 13:e0006805. doi: 10.1371/journal.pntd.0006805
55. René-Martellet M, Minard G, Massot R, Van VT, Moro CV, Chabanne L, et al. Bacterial microbiota associated with Rhipicephalus sanguineus (s.l.) ticks from France, Senegal and Arizona. Parasit Vectors. (2017) 10:1–10. doi: 10.1186/s13071-017-2352-9
56. Wikel SK. Ticks and tick-borne infections: Complex ecology, agents, and host interactions. Vet Sci. (2018) 5:60. doi: 10.3390/vetsci5020060
57. Zemtsova GE, Apanaskevich DA, Reeves WK, Hahn M, Snellgrove A, Levin ML. Phylogeography of Rhipicephalus sanguineus sensu lato and its relationships with climatic factors. Exp Appl Acarol. (2016) 69:191–203. doi: 10.1007/s10493-016-0035-4
58. Oliveira BCM, Ferrari ED, Viol MA, André MR, Machado RZ, de Aquino MCC, et al. Prevalence of Ehrlichia canis (Rickettsiales: Ehrlichieae) DNA in tissues from Rhipicephalus sanguineus (Acari: Ixodidae) ticks in areas endemic for canine monocytic ehrlichiosis in Brazil. J Med Entomol. (2019) 56:828–31. doi: 10.1093/jme/tjy220
59. Wang H, Corson MS, Grant WE, Teel PD. Quantitative models of Rhipicephalus (Boophilus) ticks: historical review and synthesis. Ecosphere. (2017) 8:e01949. doi: 10.1002/ecs2.1942
60. Nuchjangreed C, Somprasong W. Ectoparasite species found on domestic dogs from Pattaya District, Chon Buri Province, Thailand. Southeast Asian J Trop Med Publ Health. (2007) 38:203–7. Retrieved from: https://www.tm.mahidol.ac.th/seameo/2007-38-suppl-1/38suppl1-203.pdf
61. de Miranda RL, de Castro JR, Olegário MMM, Beletti ME, Mundim AV, O'Dwyer LH, et al. Oocysts of Hepatozoon canis in Rhipicephalus (Boophilus) microplus collected from a naturally infected dog. Vet Parasitol. (2011) 177:392–6. doi: 10.1016/j.vetpar.2011.01.044
62. Ojeda-Chi MM, Rodriguez-Vivas RI, Esteve-Gasent MD, León AAP de, Modarelli JJ, Villegas-Perez SL. Ticks infesting dogs in rural communities of Yucatan, Mexico and molecular diagnosis of Rickettsial infection. Transbound Emerg Dis. (2019) 66:102–10. doi: 10.1111/tbed.12990
63. Ramos VN, Lemos FG, Azevedo FC, Arrais RC, Lima CFM, Candeias IZ, et al. Wild carnivores, domestic dogs and ticks: shared parasitism in the Brazilian Cerrado. Parasitology. (2020) 147:689–98. doi: 10.1017/S0031182020000335
64. Szabó MPJ, de Souza LGA, Olegário MMM, Ferreira FA, de Albuquerque Pajuaba Neto A. Ticks (Acari: Ixodidae) on dogs from Uberlândia, Minas Gerais, Brazil. Transbound Emerg Dis. (2010) 57:72–4. doi: 10.1111/j.1865-1682.2010.01111.x
65. Betancourt-Ruiz P, Martínez-Díaz H-C, Gil-Mora J, Ospina C, Olaya-M L-A, Benavides E, et al. Candidatus Rickettsia senegalensis in cat fleas (Siphonaptera: Pulicidae) collected from dogs and cats in Cauca, Colombia. J Med Entomol. (2020) 57:382–7. doi: 10.1093/jme/tjz177
66. Chaorattanakawee S, Korkusol A, Tippayachai B, Promsathaporn S, Poole-Smith BK, Takhampunya R. Amplicon-based next generation sequencing for rapid identification of Rickettsia and ectoparasite species from entomological surveillance in Thailand. Pathogens. (2021) 10:215. doi: 10.3390/pathogens10020215
67. Hornok S, Baneth G, Grima A, Takács N, Kontschán J, Meli ML, et al. Molecular investigations of cat fleas (Ctenocephalides felis) provide the first evidence of Rickettsia felis in Malta and Candidatus Rickettsia senegalensis in Israel. New Microb New Infect. (2018) 5:3–6. doi: 10.1016/j.nmni.2018.05.001
68. Geevarghese G, Mishra A. Haemaphysalis Ticks of India. Mishra AC, editor. London; Waltham, Mass.: Elsevier (2011) p. 229–44. doi: 10.1016/B978-0-12-387811-3.00003-6
69. White SA, Bevins SN, Ruder MG, Shaw D, Vigil SL, Randall A, et al. Surveys for ticks on wildlife hosts and in the environment at Asian longhorned tick (Haemaphysalis longicornis)-positive sites in Virginia and New Jersey, 2018. Transbound Emerg Dis. (2021) 68:605–14. doi: 10.1111/tbed.13722
70. Heath ACG. Biology, ecology and distribution of the tick, Haemaphysalis longicornis Neumann (Acari: Ixodidae) in New Zealand. N Z Vet J. (2016) 64:10–20. doi: 10.1080/00480169.2015.1035769
71. Zhao L, Li J, Cui X, Jia N, Wei J, Xia L, et al. Distribution of Haemaphysalis longicornis and associated pathogens: analysis of pooled data from a China field survey and global published data. Lancet Planetary Health. (2020) 4:e320–9. doi: 10.1016/S2542-5196(20)30145-5
72. Stuen S, Granquist E, Silaghi C. Anaplasma phagocytophilum-a widespread multi-host pathogen with highly adaptive strategies. Front Cell Infect Microbiol. (2013) 3:31. doi: 10.3389/fcimb.2013.00031
73. Thompson AT, White S, Shaw D, Egizi A, Lahmers K, Ruder MG, et al. Theileria orientalis Ikeda in host-seeking Haemaphysalis longicornis in Virginia, U.S.A. Ticks Tick Borne Dis. (2020) 11:101450. doi: 10.1016/j.ttbdis.2020.101450
74. Thompson AT, White SA, Shaw D, Garrett KB, Wyckoff ST, Doub EE, et al. A multi-seasonal study investigating the phenology, host and habitat associations, and pathogens of Haemaphysalis longicornis in Virginia, U.S.A. Ticks Tick Borne Dis. (2021) 12:101773. doi: 10.1016/j.ttbdis.2021.101773
75. Bowser NH, Anderson NE. Dogs (Canis familiaris) as sentinels for human infectious disease and application to Canadian populations: a systematic review. Vet Sci. (2018) 5:1–24. doi: 10.3390/vetsci5040083
76. Smith FD, Ballantyne R, Morgan ER, Wall R. Estimating Lyme disease risk using pet dogs as sentinels. Comp Immunol Microbiol Infect Dis. (2012) 35:163–7. doi: 10.1016/j.cimid.2011.12.009
77. Hornok S, Dénes B, Meli ML, Tánczos B, Fekete L, Gyuranecz M, et al. Non-pet dogs as sentinels and potential synanthropic reservoirs of tick-borne and zoonotic bacteria. Vet Microbiol. (2013) 167:700–3. doi: 10.1016/j.vetmic.2013.08.011
78. Gettings JR, Self SCW, McMahan CS, Brown DA, Nordone SK, Yabsley MJ. Local and regional temporal trends (2013–2019) of canine Ehrlichia spp. seroprevalence in the USA. Parasit Vectors. (2020) 13:153. doi: 10.1186/s13071-020-04022-4
79. Self SCW, Liu Y, Nordone SK, Yabsley MJ, Walden HS, Lund RB, et al. Canine vector-borne disease: mapping and the accuracy of forecasting using big data from the veterinary community. Animal Health Res Rev. (2019) 20:47–60. doi: 10.1017/S1466252319000045
80. Gettings JR, Self SCW, McMahan CS, Brown DA, Nordone SK, Yabsley MJ. Regional and local temporal trends of Borrelia burgdorferi and Anaplasma spp. Seroprevalence in domestic dogs: contiguous United States 2013-2019. Front Vet Sci. (2020) 7:561592. doi: 10.3389/fvets.2020.561592
81. Liu Y, Nordone SK, Yabsley MJ, Lund RB, McMahan CS, Gettings JR. Quantifying the relationship between human Lyme disease and Borrelia burgdorferi exposure in domestic dogs. Geospat Health. (2019) 14:111–20. doi: 10.4081/gh.2019.750
82. Ybañez AP, Inokuma H. Anaplasma species of veterinary importance in Japan. Veterinary World. (2016) 9:1190–6. doi: 10.14202/vetworld.2016.1190-1196
83. Bilic P, Kules J, Baric R, Mrljak V. Canine babesiosis: where do we stand? Acta Veterinaria-Beograd. (2018) 68:127–60. doi: 10.2478/acve-2018-0011
84. Köster LS, Lobetti RG, Kelly P. Canine babesiosis: a perspective on clinical complications, biomarkers, and treatment. Vet Med Res Rep. (2015) 6:119–28. doi: 10.2147/VMRR.S60431
85. Mylonakis M, Theodorou K. Canine monocytic ehrlichiosis: an update on diagnosis and treatment. Acta Veterinaria. (2017) 67:299–317. doi: 10.1515/acve-2017-0025
86. Modrý D, Beck R, Hrazdilová K, Baneth G. A review of methods for detection of Hepatozoon infection in carnivores and arthropod vectors. Vector-Borne Zoonotic Dis. (2017) 17:66–72. doi: 10.1089/vbz.2016.1963
87. Bouchaour S, Azzag N, Ghalmi F, Chine F, China B. Canine hepatozoonosis a bibliographic review. Rev Med Vet. (2016) 167:201–10. Retrieved from: https://www.researchgate.net/profile/Bernard-China/publication/306179350_Canine_hepatozoonosis_a_bibliograhic_review/links/5965ce824585157fcc5eefb5/Canine-hepatozoonosis-a-bibliograhic-review.pdf
88. Matysiak A, Dudko P, Dudek K, Dudek M, Junkuszew A, Tryjanowski P. The occurrence of pathogens in Rhipicephalus microplus ticks from cattle in Madagascar. Vet Med. (2016) 61:516–23. doi: 10.17221/59/2016-VETMED
89. Ghafar MW, Amer SA. Prevalence and first molecular characterization of Anaplasma phagocytophilum, the agent of human granulocytic anaplasmosis, in Rhipicephalus sanguineus ticks attached to dogs from Egypt. J Adv Res. (2012) 3:189–94. doi: 10.1016/j.jare.2011.08.002
90. Prado-Ávila SR, Rascón-Cruz Q, Beristain-Ruiz DM, Adame-Gallegos JR. Identificación del agente etiológico de la anaplasmosis granulocítica humana en la garrapata café de perro en Chihuahua, México. Salud Pública de México. (2018) 60:377–8. doi: 10.21149/9153
91. Santos HA, Thomé SMG, Baldani CD, Silva CB, Peixoto MP, Pires MS, et al. Molecular epidemiology of the emerging zoonosis agent Anaplasma phagocytophilum (Foggie, 1949) in dogs and ixodid ticks in Brazil. Parasites Vectors. (2013) 6:348. doi: 10.1186/1756-3305-6-348
92. Bang MS, Kim C-M, Pyun S-H, Kim D-M, Yun NR. Molecular investigation of tick-borne pathogens in ticks removed from tick-bitten humans in the southwestern region of the Republic of Korea. PLoS One. (2021) 16:e0252992. doi: 10.1371/journal.pone.0252992
93. Nowak M, Cieniuch S, Stańczak J, Siuda K. Detection of Anaplasma phagocytophilum in Amblyomma flavomaculatum ticks (Acari: Ixodidae) collected from lizard Varanus exanthematicus imported to Poland. Exp Appl Acarol. (2010) 51:363–71. doi: 10.1007/s10493-009-9332-5
94. Michalik J, Stańczak J, Cieniuch S, Racewicz M, Sikora B, Dabert M. Wild boars as hosts of human-pathogenic Anaplasma phagocytophilum variants. Emerg Infect Dis. (2012) 8:998–1001. doi: 10.3201/eid1806.110997
95. Reeves WK. Tick larvae from Yigo, Anderson AFB (2015). Available online at: http://guaminsects.myspecies.info/sites/guaminsects.myspecies.info/files/Anderson%20A%20FB%20Ticks%2027%20July%202015%20Memo.pdf (accessed April 1, 2020).
96. Maggi RG, Mascarelli PE, Havenga LN, Naidoo V, Breitschwerdt EB. Co-infection with Anaplasma platys, Bartonella henselae and ‘Candidatus Mycoplasma haematoparvum'in a veterinarian. Parasites Vectors. (2013) 6:103–13. doi: 10.1186/1756-3305-6-103
97. Breitschwerdt EB, Hegarty BC, Qurollo BA, Saito TB, Maggi RG, Blanton LS, et al. Intravascular persistence of Anaplasma platys, Ehrlichia chaffeensis, and Ehrlichia ewingii DNA in the blood of a dog and two family members. Parasit Vectors. (2014) 7:298. doi: 10.1186/1756-3305-7-298
98. Arraga-Alvarado CM, Qurollo BA, Parra OC, Berrueta MA, Hegarty BC, Breitschwerdt EB. Case Report: Molecular evidence of Anaplasma platys infection in two women from Venezuela. Am J Trop Med Hyg. (2014) 91:1161–5. doi: 10.4269/ajtmh.14-0372
99. Perez M, Bodor M, Zhang C, Xiong Q, Rikihisa Y. Human infection with Ehrlichia canis accompanied by clinical signs in Venezuela. Ann N Y Acad Sci. (2006) 1078:110–7. doi: 10.1196/annals.1374.016
Keywords: Guam, ticks, tick-borne disease, tick-borne pathogen, domestic animals, dogs (Canis familiaris), wild pigs (Sus scrofa), sentinels
Citation: Weaver GV, Anderson N, Garrett K, Thompson AT and Yabsley MJ (2022) Ticks and Tick-Borne Pathogens in Domestic Animals, Wild Pigs, and Off-Host Environmental Sampling in Guam, USA. Front. Vet. Sci. 8:803424. doi: 10.3389/fvets.2021.803424
Received: 28 October 2021; Accepted: 16 December 2021;
Published: 11 January 2022.
Edited by:
Magdalena Larska, National Veterinary Research Institute (NVRI), PolandReviewed by:
Attila D. Sándor, University of Agricultural Sciences and Veterinary Medicine of Cluj-Napoca, RomaniaSara Savic, Scientific Veterinary Institute Novi Sad, Serbia
Michał Czopowicz, Warsaw University of Life Sciences, Poland
Copyright © 2022 Weaver, Anderson, Garrett, Thompson and Yabsley. This is an open-access article distributed under the terms of the Creative Commons Attribution License (CC BY). The use, distribution or reproduction in other forums is permitted, provided the original author(s) and the copyright owner(s) are credited and that the original publication in this journal is cited, in accordance with accepted academic practice. No use, distribution or reproduction is permitted which does not comply with these terms.
*Correspondence: Genevieve V. Weaver, ZHJndndlYXZlciYjeDAwMDQwO2dtYWlsLmNvbQ==