- Institute of Animal Nutrition, College of Animal Science and Technology, Northeast Agricultural University, Harbin, China
This study intended to explore the effect and mechanism of different doses of dietary quercetin on calcium and phosphorus metabolism to provide an experimental basis for preventing leg disease in broilers. A total of 480 1-day-old healthy Arbor Acre broilers were randomly allotted into four groups (0, 0.02, 0.04, 0.06%) for 42 days. Compared with control, 0.06% quercetin significantly increased the unit weight and the relative weight of tibia in broilers (P < 0.05). Meanwhile, phosphorus content and bone mineral density (BMD) were significantly increased by 0.06% dietary quercetin supplementation in tibia (P < 0.05). Ash of tibia was significantly increased by 0.04 and 0.06% quercetin in broilers (P < 0.05). In addition, 0.06% quercetin significantly increased the content of serum calcium-binding protein (CB), estradiol (E2), osteocalcin (OC), alkaline phosphatase (ALP), and calcitonin (CT) (P < 0.05); 0.04% quercetin significantly increased 1,25-dihydroxyvitamin D3 (1,25-(OH)2D3) (P < 0.05) content in serum of broilers. The content of serum parathyroid (PTH) was significantly decreased by 0.02 and 0.06% quercetin (P < 0.05) in broilers. Gene Ontology (GO) functional annotation and Kyoto Encyclopedia of Genes and Genomes (KEGG) enrichment analysis showed that the Wnt signaling pathway was a key signaling pathway of calcium and phosphorus metabolism in broilers which was significantly regulated by quercetin. The differentially expressed genes (DEGs) from transcriptome sequencing were validated with real-time quantitative PCR (RT-qPCR). In conclusion, 0.06% dietary quercetin supplementation improved calcium and phosphorus metabolism by regulating the Wnt signaling pathway in broilers.
Introduction
The poultry industry is vital for creating the national economy. Poultry-provided animal protein is widely consumed by humans, namely chicken and eggs. Poultry was the largest source of protein worldwide in 2019 (1). However, intensive production has increased the level of modern broiler production, and has also greatly increased the incidence of leg disease in broilers (2, 3). Tibial dyschondroplasia (TD) is a common bone disease in rapidly growing poultry throughout the world that causes movement and standing difficulties in broilers (4). In modern feeding patterns, broilers grow faster but are at higher risk of TD due to fast movement and weight gain, resulting in weak bones and lameness, and the disease incidence is as high as 40–60% in the flocks (5, 6). Therefore, leg diseases are considered to be the biggest economic threats to the poultry industry and may not be ignored in the breeding industry of broilers (7, 8). The production cycle of chickens is short, most drugs with serious drug residues have a long metabolic cycle, and the treatment cost is high, which severely restricts the initiative of TD treatment (9). In the modern breeding industry, the deficiency or low utilization rate of calcium and phosphorus in feed or the imbalance of calcium and phosphorus may further induce bone malnutrition in broilers. The balance between calcium and phosphorus in the feed is vital to leg health of broilers (10–12). To effectively improve the metabolism of calcium and phosphorus and reduce the occurrence of TD in broilers, functional feed additives to prevent leg disease in broilers need to be studied (13, 14).
Flavonoids have estrogen-like effects (15). Estrogen may promote the intestinal absorption of calcium (16), and flavonoids cooperate with estrogen to affect calcium and phosphorus metabolism. Quercetin, a type of flavonoid, is abundant in various tea, fruits, and leaves, and has antioxidant, anti-inflammatory, anti-allergic, antibacterial, and antiviral activities (17–19). Therefore, rational use of quercetin will produce huge economic benefit. In addition, quercetin may improve the absorption of calcium in the small intestine, and enhance the activity of vitamin D receptor (VDR) (20). One study confirmed the positive effects of proanthocyanidins and cannabinoids on bone health, which improved bone weight loss, bone length and diameter, content of calcium and phosphorus in ashes, and bone mineral density (BMD) in rats (21). VDR expression of bone cells was low in ovariectomized rats, after estrogen treatment, VDR expression was significantly increased, this implied that estrogen may enhance VDR expression in bone (22). It suggested that quercetin may also promote calcium absorption in the small intestine, thereby regulating bone calcium metabolism. However, the specific mechanism of action remains unclear.
The intestine plays a major role in the digestion and absorption of calcium and phosphorus. Ca2+ was mainly absorbed in the small intestine, especially in the duodenum (23, 24). 1,25-dihydroxyvitamin D3 (1,25-(OH)2D3) combined with parathyroid (PTH) and calcitonin (CT) forms the most important hormone in the body that maintains calcium homeostasis (25). Absorption of calcium in the small intestine by 1,25-(OH)2D3 was mainly concentrated in the transcellular transport pathway and bound to specific receptors in the small intestinal mucosa, and increased the synthesis of calcium-binding protein (CB), thus promoting Ca2+ absorption (26, 27). Flavonoids were mainly absorbed and utilized in the small intestine, while the absorption of calcium in the intestine mainly depends on hormones such as CB, 1,25-(OH)2D3, and PTH. Then, hormones may be a potential target for flavonoids to regulate calcium and phosphorus metabolism. The calcium and phosphorus metabolism was mainly transported in the duodenum. However, no examination was done on the mechanism of quercetin regulating calcium and phosphorus metabolism in specific intestinal segments, and quercetin regulating calcium and phosphorus metabolism at the molecular level in duodenum of broilers has not been reported.
The aim of this study was to evaluate the mechanism of quercetin regulating calcium and phosphorus metabolism in broilers. Therefore, the effects of quercetin on tibia development, calcium deposition, serum biochemical index, and transcriptome changes of duodenal mucosa in broilers were studied to elucidate the mechanism of dietary quercetin supplementation regulating calcium and phosphorus metabolism. The results of this experiment will provide the scientific basis for researching the use of quercetin in practical production to improve broiler tibia development in the future.
Materials and Methods
Animal and Diets
A total of 480 (1-day-old) healthy Arbor Acre broilers (similar in body weight, 47.17 ± 1.26 g) were obtained from a commercial company (Yinong Poultry, Harbin, China). The chickens were randomly divided into four experimental groups with six replicates per group and 20 broilers per replicate.
Broilers were raised in a netted stainless steel cage (526 × 423 × 381 mm) with ad libitum access to feed and fresh water, and controlled ventilation. All chickens experienced 16 h of continuous light every day for 42 days. Temperature was maintained at 32–34°C for the first 3 days and decreased to 24°C by the end.
The experimental diets were formulated based on corn and soybean meal according to Chinese Broiler Feeding Standards (GB/T5916-2020) to meet the nutrient requirements of broilers (Table 1). And four concentrations of quercetin were added to the diet: 0.00, 0.02, 0.04, and 0.06%. Feeding was divided into two phases: the starter phase from 1 to 21 days and the grower phase from 22 to 42 days. Quercetin dihydrate powder with 97% purity (Sigma-Aldrich, United States) was mixed in basal diet.
Sample Collection and Preparations
At 42 days of age, broilers were randomly slaughtered from six replicates in four groups. After 12 h of fasting, broilers were weighed and euthanized by cervical dislocation. The jugular vein blood samples (10 ml) were collected and placed on ice. The tibia was quickly separated, the adherent tissue was removed, then cleaned with saline, and stored at −20°C for further analysis. About 5 g of duodenal mucosa was placed in liquid nitrogen and then preserved at −80°C. All procedures used in this study were approved by the Animal Welfare Committee of Northeast Agricultural University (Harbin, China). Housing, management, and care of the birds were carried out in accordance with the guidelines for the Agricultural Animal in Agricultural Research and Teaching of Heilongjiang Province (HEI Animal Management Certificate No. 11928).
Tibia Index
The tibial length was measured with a vernier caliper, the wet weight was weighed by electronic balance, and the unit weight and relative weight of tibia were calculated.
Unit weight of tibia (g/cm) = fresh weight of tibia (g)/length of tibia (cm).
Relative tibia weight (%) = [weight of tibia (g)]/[weight of broiler (g)] × 100
Calcium and Phosphorus Deposition
Calcium concentration in tibia was determined by an atomic absorption spectrophotometer, and P was determined by the vanadium molybdate colorimetric method. The ash content of tibia was expressed as ash per unit tibial length, and expressed as a percentage of dry weight (28). BMD in tibia was measured using a Qdr-4500w dual energy X-ray bone mineral density detector.
Serum Biochemical Index
Serum biochemical indices were assessed according to the content of alkaline phosphatase (ALP), calcium (Ca), phosphorus (P), osteocalcin (OC), estradiol (E2), CB, PTH, 1,25-(OH)2D3, and CT by an ELISA assay kit (Nanjing Jiancheng Bioengineering Institute, Nanjing, Jiangsu, P. R. China).
High-Throughput RNA Sequencing
In this endeavor, 12 samples of duodenal mucosa were frozen in dry ice and sent to Genesis (Beijing) Co. Ltd. for sequencing using the HiSeq 2000 System (Illumina, Inc, USA). The results were compared with the database and the markers of each gene were prepared for the subsequent experimental analysis.
Real-Time Quantitative PCR
The mRNA expression of genes in the duodenal mucosa of broilers was determined using real-time quantitative polymerase chain reaction (RT-qPCR) (7500 Real-Time PCR System, Singapore). Primers were designed using Primer 5.0 software based on mRNA sequences of broiler genes published on GenBank, and Primers were synthesized (Sangon Biological Co. Ltd., Shanghai, China). The sequences of primers are showed in Table 2. After samples from the frozen duodenal mucosa were ground into fine powder in a liquid nitrogen environment, total RNA was extracted using the TRIZOL (TaKaRa, Japan) reagent. Genomic DNA was removed from total RNAs by the DNA-free kit (TaKaRa Biotechnology, Co., Ltd. Dalian, China). The integrity of RNA was detected on 1.5% agarose gel and shown by ethidium bromide staining. The 260/280 nm absorbance ratio of all RNA samples of duodenal mucosa was between 1.8 and 2.2. First strand cDNA was synthesized from 2 μg total RNA by RNA reverse transcription. The reaction mixtures were incubated in the following conditions: reverse transcription at 37°C for 15 min and inactivation of reverse transcriptase at 85°C for 5 min, until the temperature was decreased to 4°C. Reverse transcription was performed to create cDNA (2 μl) from the total RNA to use as a template for RT-qPCR. The cDNA was amplified by PCR in 20 μl of reaction mixture. The RT-qPCR reaction was carried out according to the instructions of the SYBR Real-Time PCR kit (TaKaRa Biotechnology, Co., Ltd. Dalian, China). The PCR condition was as follows: initial denaturation at 95°C for 30 s, followed by PCR reaction at 40 cycles of 95°C for 5 s and 60°C for 34 s, and melting curve analysis at 95°C for 15 s, 60°C for 2 s, and 95°C for 15 s. The specificity of the qPCR reaction was monitored by melting curve analysis and gel electrophoresis. β-actin was used as the internal control in this study (Table 2). All experiments were repeated in triplicate. The data were analyzed with the comparative cycle threshold method (2−ΔΔCT) in Microsoft Excel software.
Statistical Analysis
All data from this experiment were analyzed by one-way ANOVA as a completely randomized design with four treatments and six replicates for each treatment using SPSS 21.0 statistical software (SPSS Inc., Chicago, IL). All the results were expressed as the “mean values ± standard deviation”. Calculated ΔCt (corrected sample) = mean value of target gene − mean value of internal reference gene, ΔΔCt = ΔCt-mean value of control group. Differences with treatment means with a possibility of P < 0.05 were considered as statistically significant.
Results
Effect of Quercetin on Tibia Development in Broilers
The unit weight of tibia was significantly increased with increasing quercetin (P < 0.05). The relative weight of tibia was significantly increased by 0.06% quercetin (P < 0.05). Dietary quercetin supplementation at the level of 0.04% also significantly increased the unit weight of tibia (P < 0.05). However, quercetin did not affect tibia length (P > 0.05), compared with control (Table 3).
Effect of Quercetin on Calcium and Phosphorus Deposition in Broilers
Compared with control, the content of phosphorus and BMD in tibia was significantly increased by 0.06% quercetin (P < 0.05). Dietary quercetin supplementation at levels of 0.04 and 0.06% also significantly increased ash of tibia (P < 0.05). The content of calcium in tibia tended to increase (P = 0.079) (Table 4).

Table 4. Effect of quercetin on content of calcium and phosphorus and bone density in tibia of broilers.
Effect of Quercetin on Serum Biochemical Indexes of Broilers
Compared with control, the content of serum CB, OC, and ALP in broilers was significantly increased with increasing quercetin (P < 0.05). Dietary quercetin supplementation at the level of 0.06% significantly increased the content of serum E2 and CT in broilers (P < 0.05). The content of serum E2 and 1,25-(OH)2D3 in broilers was significantly increased by 0.04% quercetin (P < 0.05). The content of serum of PTH in broilers was significantly decreased by 0.02 and 0.06% quercetin (P < 0.05). However, dietary quercetin supplementation did not affect the content of serum P and Ca in broilers (P > 0.05) (Table 5).
Overall Assessment for Mapping Statistics
Sequence data from the 12 samples were mapped to the reference genome (Gallus gallus, 5.0). The RNA-seq libraries of the 12 samples were sequenced on the Illumina HiSeq 2500 platform. After the adaptors were removed and low quality reads from raw data were filtered, 6.22 Gb of raw paired-end reads was generated. A total of 116,922,726 cleaned reads were obtained from the four groups. Meanwhile, 77.92–78.84% reads and 60.74–61.76% unique reads were successfully aligned to the chicken reference genomes. The average Q30 value was higher than 94.33% (Table 6). These results indicated that the sequencing results were reliable enough for further analysis.
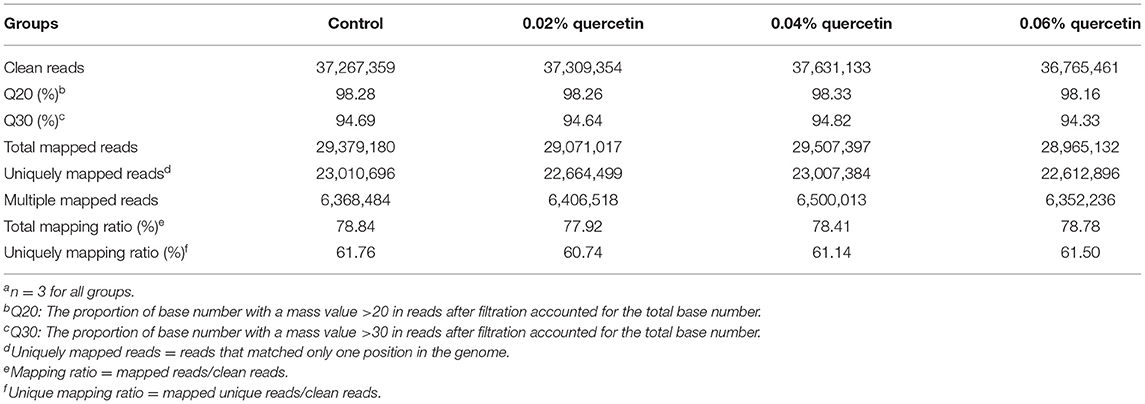
Table 6. Summary statistics for sequence quality and alignment information of 12 samples from duodenal mucosaa.
Differentially Expressed Genes of Quercetin in the Duodenum of Broilers
Compared with control, 7,035 differentially expressed genes (DEGs) were downregulated and 3,084 DEGs were upregulated among 10,119 significant DEGs in the 0.02% quercetin group; 7,687 DEGs were downregulated and 3,204 DEGs were upregulated among 10,891 significant DEGs in the 0.04% quercetin group; And 8,699 DEGs were downregulated and 2,801 DEGs were upregulated among 11,500 significant DEGs in the 0.06% quercetin group (Figure 1).
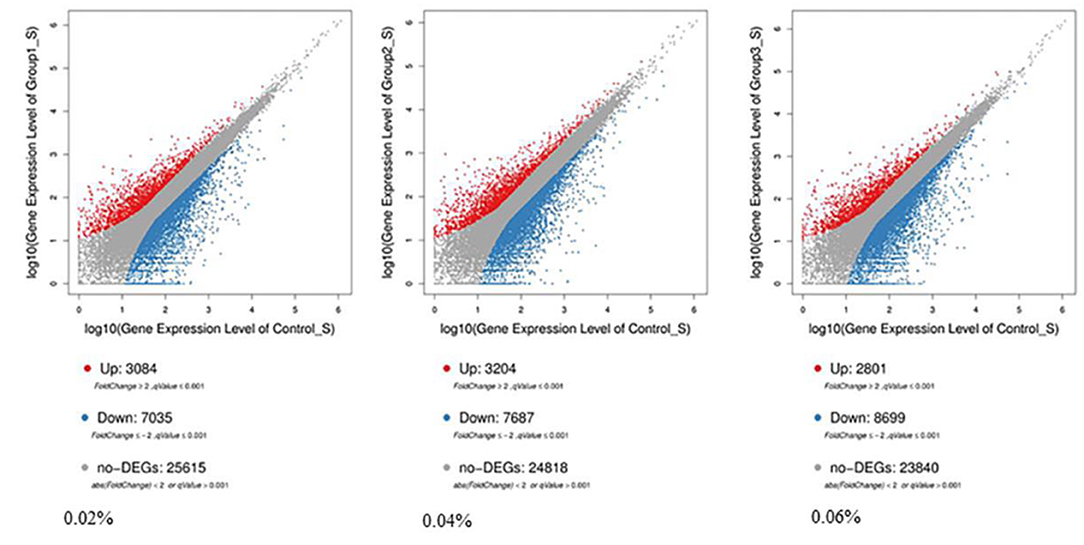
Figure 1. Comparison of differentially expressed genes between control and quercetin (0.02, 0.04, and 0.06%). The volcano plot shows correlations in the gene-rich dimension. The red and blue dots represent significantly upregulated and downregulated genes by quercetin, respectively.
Functional Annotation and Signaling Pathway Enrichment of Differentially Expressed Genes
The Gene Ontology (GO) knowledgebase is the largest source of information on the functions of genes in the world, which is divided into three parts, including biological process, cellular component, and molecular function. Among them, the metabolic process in biological processes ranked the fourth among the most abundant GO terms (Figure 2).
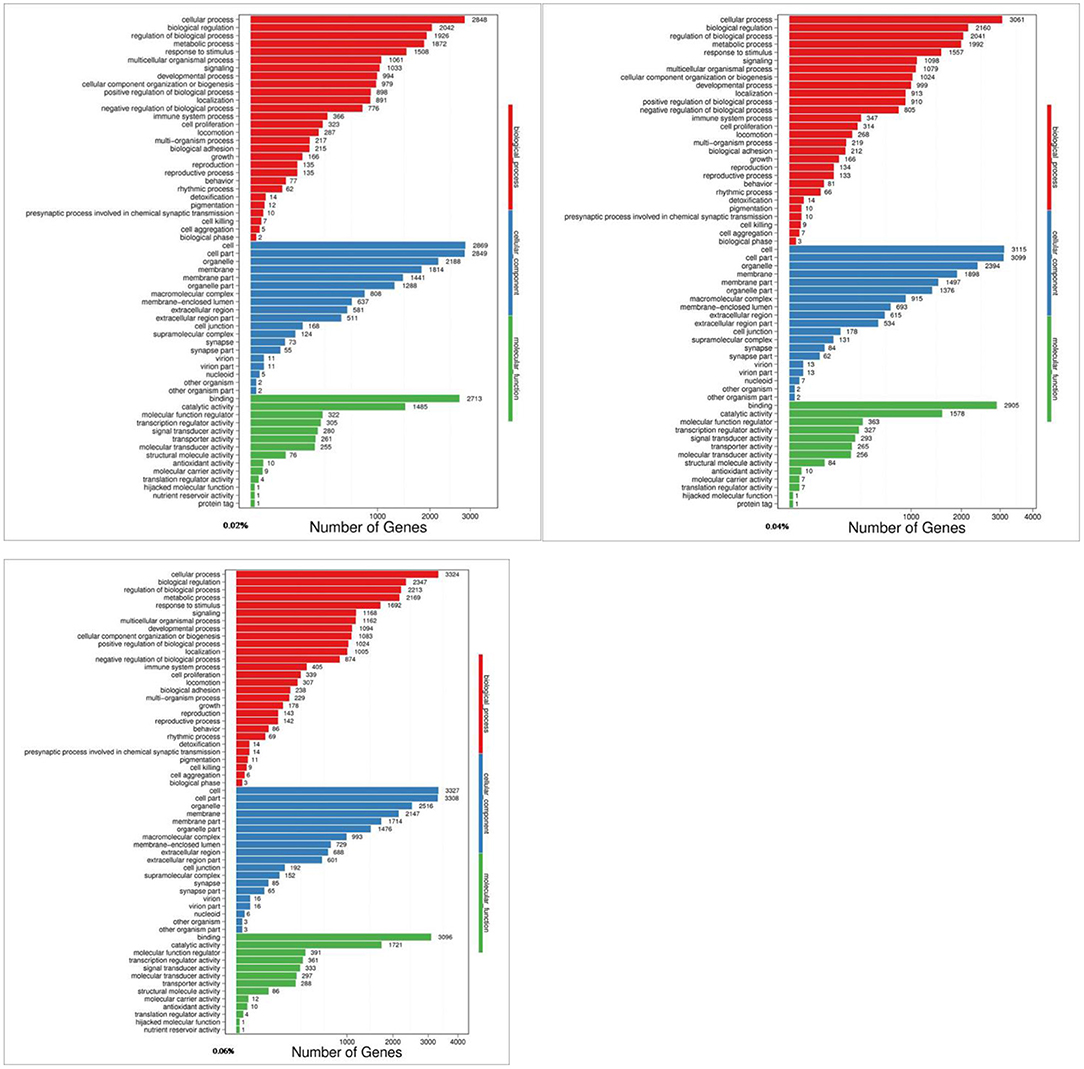
Figure 2. GO analyses of quercetin affecting differentially expressed genes in the duodenal mucosa of broilers (0.02, 0.04, and 0.06%).
The present results showed that 9,231 DEGs of control/0.02% quercetin were annotated into 334 pathways, 9,899 DEGs of control/0.04% quercetin were annotated into 334 pathways, and 10,544 DEGs of control/0.06% quercetin were annotated into 337 pathways (Table 7).
Kyoto Encyclopedia of Genes and Genomes (KEGG) enrichment analysis showed that the Wnt signaling pathway was the main signaling pathway of calcium and phosphorus metabolism in broilers, which was significantly regulated by quercetin. Signal transduction accounted for the largest proportion in KEGG analysis, and the Wnt signaling pathway was included in signal transduction (Figure 3). Among them, q ≤ 0.01 and ||≥1 were selected as the conditions for filtering DEGs. The main DEGs in the Wnt signaling pathway were as follows: Wnt family member 5a (Wnt-5a), calcium/calmodulin-dependent protein kinase II (CAMK2G, CAMK2D, CAMK2B), phospholipase C, beta 4 (PLCB4), protein kinase C alpha (PRKCA), and nuclear factor of activated T cells-1 (NFATC1) (Table 8).
Validation of the Wnt Signaling Pathway by RT-qPCR
To verify the accuracy of the RNA-seq results in the transcriptome, the main DEGs in the Wnt signaling pathway were selected as follows: Wnt-5a, NFATC1, CAMK2G, CAMK2D, CAMK2B, PLCB4, and PRKCA, and β-actin was used as a house-keeping gene. The results showed that the mRNA expression by RT-qPCR was consistent with the transcriptome RNA-seq results, it indicated that the sequencing results were reliable (Figures 4, 5).
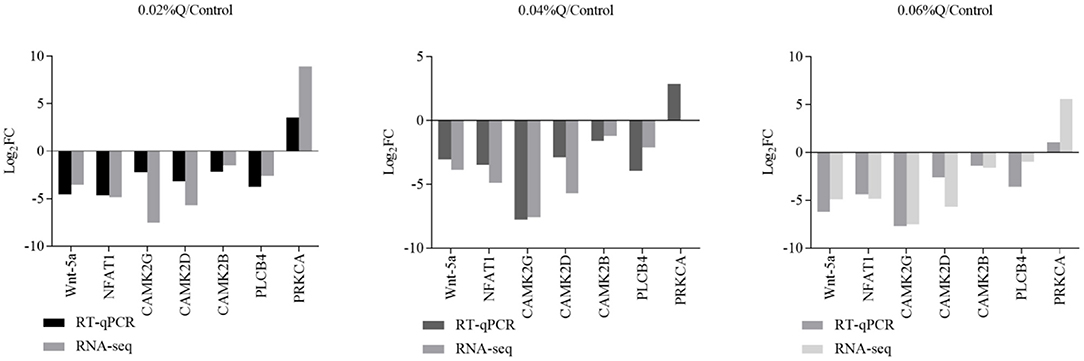
Figure 4. Correlations of mRNA expression level of seven random DEGs between the level of calcium and phosphorus using RNA-seq and RT-qPCR. The X-axis represents the seven selected genes for RT-qPCR assays and the Y-axis represents the derived from RNA-seq. Values are mean ± SEM (n = 6). 0.02, 0.04, and 0.06%Q represent 0.02% quercetin, 0.04% quercetin, and 0.06% quercetin, respectively.
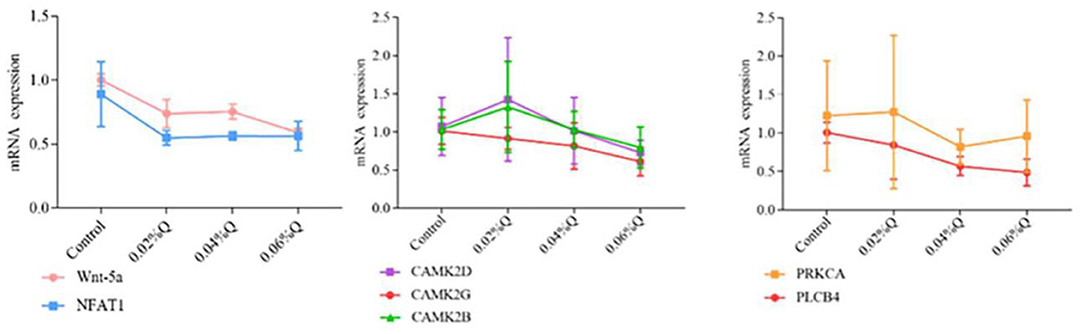
Figure 5. Effect of quercetin on mRNA expression of genes related to calcium and phosphorus metabolism in duodenal mucosa. (1) The results of relative quantification are expressed as 2−ΔΔCT. The quantification of control is 1, namely 2−ΔΔCT = 1. The value 2−ΔΔCT of the treatment group is a multiple of control. n = 6. (2) Mean values without a common letter are significantly different, P < 0.05. Values are mean ± SEM (n = 6). 0.02, 0.04, and 0.06%Q represent 0.02% quercetin, 0.04% quercetin, and 0.06% quercetin, respectively.
Discussion
Quercetin is the major representative of plant flavonoids, which ubiquitously found in fruits, kales, and cherries, as well as onions and red wine, is one of the most common flavonoids in the diet (29–31). Modern research has shown that quercetin may prevent various diseases, such as osteoporosis (32), tumors (33), and lung diseases (34). At present, there are no reports about quercetin preventing TD and regulating calcium and phosphorus metabolism. Induced TD diet combined with cholecalciferol at 1,500 IU/kg improved tibia ash, performance, and prevented TD in 1-day-old broilers (35). BMD, bone weight index, tended to increase with dietary quercetin (100 mg/kg/day) supplementation in rats (P < 0.05) (36). Diet supplemented with 2.50% quercetin increased bone density in ovariectomized rats (P < 0.05) (37). In addition, icariin prevented TD in broiler chickens, tibia weight was increased with the increasing icariin, and icariin inhibited bone resorption and stabilized bone bioapatite, it indicated that the BMD of growing rats supplemented with total flavone capsules from icariin was significantly increased (P < 0.05) (38). Meanwhile, compared with control, the tibia length of the low genistein group was longer (P < 0.05), and a high genistein level may stimulate the subchondral bone of the mandible, thereby it is beneficial to bone formation in rats (39, 40). Moreover, in the heat neutral and heat stress groups, supplementation of 25-hydroxycholecalciferol (25-OH-D3) and soy isoflavones linearly increased BMD, content of ash, Ca, and P in tibia (P < 0.05) (28). Our results showed that dietary supplementation of quercetin at the level of 0.06% significantly increased relative weight, unit weight, P, BMD, and ash of tibia (P < 0.05). The present results were supported by previous studies on the effect of flavonoids on bone development and calcium and phosphorus deposition in broilers (41).
To further study the development of the tibia and the deposition of Ca and P in the tibia of broilers, the serum biochemical indicators of broilers were determined and a duodenal transcriptomic study was carried out. Calcium provides the power to support movement for bone, however, it is also a reservoir for maintaining serum calcium levels (42, 43). Calcium metabolism is achieved by bone, kidney, and intestine, and intestinal calcium metabolism is the key. Calcium transport in the intestine is regulated by a complex homeostatic hormone system, mainly including 1,25-(OH)2D3 and serum Ca (44). Among them, 1,25-(OH)2D3 is one of the important hormones regulating bone metabolism. 1,25-(OH)2D3 reaches the intestine and bone tissue through the blood, and binds to specific receptors in the small intestinal mucosa, thereby increasing the synthesis of calcium-binding protein, which in turn promotes the absorption of Ca2+ and the process of bone calcification (45). Furthermore, soy isoflavone supplementation significantly improved levels of serum OC, vitamin D, Ca, P, and ALP activity in quail at the later laying stage (46). In addition, dietary supplementation of daidzein and Chinese herbs (CH) at the level of 0.02% significantly increased the content of serum Ca, P, and OC in laying hens (47). Similarly, in laying hens, serum calcium was linearly increased with the addition of daidzein, and serum phosphorus had a significantly conic response to the addition of daidzein (48). Compared with control, dietary supplementation of genistein (400 mg/kg) increased the content of serum CT and ALP, which was consistent with the increase in levels of calcium and phosphorus in the tibia of menopausal women (15). E2 may upregulate the expression of ATP-dependent Ca pump in the human uterine plasma membrane (49). Therefore, the present results showed that dietary supplementation of quercetin at the level of 0.06% significantly increased the content of serum E2, OC, CB, ALP, and CT (P < 0.05), and decreased the content of serum PTH (P < 0.05) in broilers. Results of serum ALP in our experiment were supported by Chen et al. who found that alfalfa flavonoids extraction increased serum ALP level in Yangzhou geese (50).
Consistently, it is important to study the site of calcium absorption in the body. Intestinal Ca2+ absorption is an active (ATP-dependent) process, which mainly occurs in the small intestine, accounting for about 90% of Ca2+ absorption (51). The absorption of Ca2+ in the intestine mainly depends on the staying time in the intestine and the solubility of Ca2+. However, the duodenum is the site with the greatest solubility of Ca2+ (52). The rate of Ca2+ absorption in the intestine is as follows: duodenum > jejunum > ileum (53). Moreover, Ca2+ is absorbed through two pathways in the intestine: the transcellular process which primarily comes up in the duodenum and is mediated by vitamin D, and the paracellular concentration-dependent diffusional process which occurs throughout the intestine (54). Duodenal mucosa is the best site to explore the mechanism of quercetin regulating calcium and phosphorus metabolism. Hence, transcriptomic techniques were used to explore the effect of quercetin on calcium and phosphorus metabolism in the duodenal mucosa of broilers.
RNA-seq is an important means to study gene expression, RNA biosynthesis, and metabolism. Most importantly, RNA-seq directly reveals sequence consistency, which is essential for analyzing quantitative gene expression and investigating detailed transcriptomic profiles (55, 56). RNA-seq may obtain the full-length transcriptome sequence in animals, which has been widely used in studying the regulatory mechanism of metabolism and gene expression in livestock and poultry (57). RNA-seq has been applied in the heart (58), uterus (59), and ovarian tissues in chickens (60), and also revealed that quercetin regulated calcium absorption (61). Nonetheless, the precise mechanism of quercetin regulating calcium and phosphorus metabolism in the duodenum of broilers is still unclear. In this study, signaling pathways related to calcium and phosphorus metabolism were determined using RNA-seq, and the results were more accurate and effective of differential gene expression from high-quality sequencing and accurate mapping reading ratios (Table 6).
After functional enrichment analyses, most GO terms and KEGG pathways were mainly involved in metabolism processes. The results were similar to previous studies in cattle and pigs (62, 63), and implied that all identified DEGs may take part in calcium and phosphorus metabolism in the duodenum. The Wnt signaling pathway was highly correlated with calcium and phosphorus metabolism, and seven important genes of Wnt-5a, CAMK2G, CAMK2D, CAMK2B, PLCB4, PRKCA, and NFATC1 in regulating calcium and phosphorus absorption and metabolism were screened out. Meanwhile, to validate the results of RNA-seq, expression of the genes related to the calcium and phosphorus metabolism pathway were determined using RT-qPCR. Overall, RT-qPCR results were highly consistent with RNA-seq results, which were similar to some results previously obtained in rats (64) and chickens (65, 66).
The Wnt signaling pathway played a critical role in normal skeletal homeostasis and function. Wnt signaling cascades may play a centrally regulatory part in the development of calcium signal (67). The binding of Wnt-5a to the homologous Frizzled (Fz) receptor temporarily increases the concentration of inositol 1,4,5-triphosphate (IP3), Ca2+, and 1,2-diacylglycerol (DAG) intracellular signaling molecules. IP3 and DAG were derived from phospholipase C in the plasma membrane. Then IP3 underwent diffusion in the endoplasmic reticulum (ER) membrane and interacted with calcium channels, this process released Ca2+, then Ca2+ and calmodulin activate calmodulin-independent protein kinase II (CaMKII), and Ca2+ activates protein kinase C (PRK) through releasing ER (68–70). In this study, compared with control, quercetin supplementation significantly regulated the key genes of calcium and phosphorus metabolism in the Wnt signaling pathway. Wnt-5a played a crucial role in the atypical Wnt/PCP and Wnt/PKC pathways of osteoarthritis osteoblasts (71). The concentration of Ca2+ required for the activation of CAMK2B, CAMK2D, and CAMK2G depends on calmodulin level in the reaction (72). In the regulation of bone metabolism, the calcineurin-NFAT pathway acted on osteoclasts, osteoblasts, and chondrocytes (73). NFATC1 was highly expressed in RANKL-induced osteoclasts. Subsequently, calcineurin dephosphorylated the serine residues in NFATC1, which then entered the nucleus to initiate osteoclast formation (74). It was consistent with our results that NFATC1 levels were significantly downregulated. Phosphatidylinositol-4,5-diphosphate was converted to diacylglycerol and inositol-1,4,5-triphosphate by the PLCB4 enzyme, which may promote the release of intracellular calcium and activate protein kinase C, and osteoclasts were profoundly changed (75). Wnt-5a, CAMK2G, CAMK2D, CAMK2B, PLCB4, PRKCA, and NFATCI were key transcription factors regulating calcium and phosphorus metabolism. Flavonoids may affect the key regulators of calcium and phosphorus metabolism in the Wnt signaling pathway. Studies found that quercetin may protect bone and inhibit osteoclast formation, which may be involved in the regulation of the Wnt signaling pathway (76). And apigenin (API) may enhance the expression of downstream target genes in the Wnt signaling pathway, thereby improving new bone formation and accelerating fracture healing in vivo, so API may be a promising candidate for fracture treatment (77). Genistein maintained intracellular Ca2+ concentration by activating the CAMK signaling pathway (78). Neohesperidin prevented bone loss in ovariectomized mice by inhibiting NFAT (79). The anti-osteoclast activity of luteolin is mediated by blocking the activity of NFATc1, which may be a potential treatment for lytic bone diseases associated with osteoclast formation and dysfunction (80). Other results indicated that flavonoids improved tibia development and prevented osteoporosis by regulating genes related to calcium and phosphorus metabolism in the Wnt signaling pathway. Our results showed that diets supplemented with quercetin in broilers downregulated Wnt-5a, CAMK2B, CAMK2D, CAMK2G, PLCB4, and NFATC1 genes and upregulated the PRKCA gene. Therefore, quercetin used as a dietary additive potentially improved tibia development and balanced calcium and phosphorus metabolism.
Conclusion
The present results showed that dietary quercetin supplementation improved calcium and phosphorus metabolism and tibia development by the Wnt signaling pathway. Quercetin was a potential functional additive preventing leg disease in broilers. Next, we will study the effect of quercetin in diseased broilers (lameness).
Data Availability Statement
The datasets presented in this study can be found in online repositories. The names of the repository/repositories and accession number(s) can be found below: http://www.ncbi.nlm.nih.gov/sra/PRJNA781819, PRJNA781819.
Ethics Statement
The animal study was reviewed and approved by the HEI Animal Management Certificate No. 11928.
Author Contributions
BW designed the study and critically revised the first manuscript. Broilers were raised by BW, SW, and MD. HL and HW performed the experiments and participated in the statistical analysis. YL modified the manuscript and gave final approval of the version to be submitted. All authors agreed to be accountable for the content of the work and approved the submitted version.
Funding
This work was supported by the National Natural Science Foundation of China (32072749).
Conflict of Interest
The authors declare that the research was conducted in the absence of any commercial or financial relationships that could be construed as a potential conflict of interest.
Publisher's Note
All claims expressed in this article are solely those of the authors and do not necessarily represent those of their affiliated organizations, or those of the publisher, the editors and the reviewers. Any product that may be evaluated in this article, or claim that may be made by its manufacturer, is not guaranteed or endorsed by the publisher.
References
1. Jahejo AR, Rajput N, Tian WX, Naeem M, Kalhoro DH, Kaka A, et al. Immunomodulatory and growth promoting effects of basil (Ocimum basilicum) and ascorbic acid in heat stressed broiler chickens. Pak J Zoo. (2019) 51:801. doi: 10.17582/journal.pjz/2019.51.3.801.807
2. Zuidhof MJ, Schneider BL, Carney VL, Korver DR, Robinson FE. Growth, efficiency, and yield of commercial broilers from 1957, 1978, and 2005. Poultry Sci. (2014) 93:2970–82. doi: 10.3382/ps.2014-04291
3. Ipema AF, Gerrits WJJ, Bokkers EAM, Kemp B, Bolhuis JE. Provisioning of live black soldier fly larvae (Hermetia illucens) benefits broiler activity and leg health in a frequency- and dose-dependent manner. Appl Anim Behav Sci. (2020) 230:105082. doi: 10.1016/j.applanim.2020.105082
4. Mehood K, Zhang H, Yao WY, Jiang X, Waqas M, Li AY, et al. Protective effect of astragaloside IV to inhibit thiram-induced tibial dyschondroplasia. Environ Sci Pollut Res. (2019) 26:16210–9. doi: 10.1007/s11356-019-05032-1
5. Jahejo AR, Tian WX. Cellular, molecular and genetical overview of avian tibial dyschondroplasia. Res Vet Sci. (2021) 135:569–79. doi: 10.1016/j.rvsc.2020.10.002
6. Jahejo AR, Kalhoro NH, Soomro H, Yu J, Zhang CL, El-Kassas S, et al. Dietary supplementation with Celecoxib to prevent the welfare problem of tibial dyschondroplasia in broiler chickens. J Iivest Sci. (2021) 250:104568. doi: 10.1016/j.livsci.2021.104568
7. Blatchford RA, Archer GS, Mench JA. Contrast in light intensity, rather than day length, influences the behavior and health of broiler chickens. Poultry Sci. (2012) 91:1768–74. doi: 10.3382/ps.2011-02051
8. Ohara A, Oyakawa C, Yoshihara Y, Ninomiya S, Sato S. Effect of environmental enrichment on the behavior and welfare of japanese broilers at a commercial farm. J Poult Sci. (2015) 52:323–30. doi: 10.2141/jpsa.0150034
9. Zhang H, Wang Y, Mehmood K, Chang Y-F, Tang Z, Li Y. Treatment of tibial dyschondroplasia with traditional Chinese medicines: “Lesson and future directions”. Poultry Sci. (2020) 99:6422–33. doi: 10.1016/j.psj.2020.08.055
10. Fleming RH. Nutritional factors affecting poultry bone health. Proc Nutr Soc. (2008) 67:177–83. doi: 10.1017/S0029665108007015
11. Huang SC, Zhang LH, Zhang JL, Rehman MU, Tong XL, Qiu G, et al. Role and regulation of growth plate vascularization during coupling with osteogenesis in tibial dyschondroplasia of chickens. Sci Rep. (2018) 8:3680. doi: 10.1038/s41598-018-22109-y
12. Zhang J, Huang S, Tong X, Zhang L, Jiang X, Zhang H, et al. Chlorogenic acid alleviates thiram-induced tibial dyschondroplasia by modulating caspases, BECN1 expression and ECM degradation. Int J Mol Sci. (2019) 20:3160. doi: 10.3390/ijms20133160
13. Zhang M, Xie Z, Gao W, Pu L, Wei J, Guo C. Quercetin regulates hepatic cholesterol metabolism by promoting cholesterol-to-bile acid conversion and cholesterol efflux in rats. Nutr Res. (2016) 36:271–9. doi: 10.1016/j.nutres.2015.11.019
14. Jiang X, Li A, Wang Y, Iqbal M, Waqas M, Yang H, et al. Ameliorative effect of naringin against thiram-induced tibial dyschondroplasia in broiler chicken. Environ Sci Pollut Res. (2020) 27:11337–48. doi: 10.1007/s11356-020-07732-5
15. Lv ZP, Yan SJ, Li G, Liu D, Guo YM. Genistein improves the reproductive performance and bone status of breeder hens during the late egg-laying period. Poultry Sci. (2019) 98:7022–9. doi: 10.3382/ps/pez367
16. Arjmandi BH, Lucas EA, Khalil DA, Devareddy L, Smith BJ, McDonald J, et al. One year soy protein supplementation has positive effects on bone formation markers but not bone density in postmenopausal women. Nutr J. (2005) 4:8. doi: 10.1186/1475-2891-4-8
17. Zhang R, Yao Y, Wang Y, Ren G. Antidiabetic activity of isoquercetin in diabetic KK -A(y) mice. Nutr Metab. (2011) 8:85. doi: 10.1186/1743-7075-8-85
18. Jung CH, Cho I, Ahn J, Jeon TL, Ha TY. Quercetin reduces high-fat diet-induced fat accumulation in the liver by regulating lipid metabolism genes. Phytother Res. (2013) 27:139–43. doi: 10.1002/ptr.4687
19. Rezk AM, Ibrahim IAAEH, Mahmoud MF, Mahmoud AAA. Quercetin and lithium chloride potentiate the protective effects of carvedilol against renal ischemia-reperfusion injury in high-fructose, high-fat diet-fed Swiss albino mice independent of renal lipid signaling. Chembio Interact. (2021) 333:109307. doi: 10.1016/j.cbi.2020.109307
20. Lee KY, Choi HS, Choi HS, Chung KY, Lee BJ, Maeng HJ, et al. Quercetin directly interacts with vitamin D receptor (VDR): structural implication of VDR activation by quercetin. Biomol Ther. (2016) 24:191–8. doi: 10.4062/biomolther.2015.122
21. Orsolic N, Nemrava J, Jelec Z, Kukolj M, Odeh D, Terzic S, et al. The beneficial effect of proanthocyanidins and icariin on biochemical markers of bone turnover in rats. Int J Mol Sci. (2018) 19:2746. doi: 10.3390/ijms19092746
22. Duque G, El Abdaimi K, Macoritto M, Miller MM, Kremer R. Estrogens (E2) regulate expression and response of 1,25-dihydroxyvitamin D3 receptors in bone cells: changes with aging and hormone deprivation. Biochem Bioph Res Commun. (2002) 299:446–54. doi: 10.1016/S0006-291X(02)02657-8
23. Gonzalez-Vega JC, Walk CL, Liu Y, Stein HH. The site of net absorption of Ca from the intestinal tract of growing pigs and effect of phytic acid, Ca level and Ca source on Ca digestibility. Arch Anim Nutr. (2014) 68:126–42. doi: 10.1080/1745039X.2014.892249
24. Mutucumarana RK, Ravindran V, Ravindran G, Cowieson AJ. Influence of dietary calcium concentration on the digestion of nutrients along the intestinal tract of broiler chickens. J Poult Sci. (2014) 51:392–401. doi: 10.2141/jpsa.0140022
25. Lips P. Interaction between vitamin D and calcium. Scand J Clin Lab Invest Suppl. (2012) 243:60–4. doi: 10.3109/00365513.2012.681960
26. Anderson PH, Turner AG, Morris HA. Vitamin D actions to regulate calcium and skeletal homeostasis. Clin Biochem. (2012) 45:880–6. doi: 10.1016/j.clinbiochem.2012.02.020
27. Aoiadni N, Ayadi H, Jdidi H, Naifar M, Maalej S, Makni FA, et al. Flavonoid-rich fraction attenuates permethrin-induced toxicity by modulating ROS-mediated hepatic oxidative stress and mitochondrial dysfunction ex vivo and in vivo in rat. Environ Sci Pollut Res. (2020) 28:9290–312. doi: 10.1007/s11356-020-11250-9
28. Sahin NTA, Onderci M, Balci TA, Cikim G, Sahin K, Kucuk O. Effects of 25-hydroxycholecalciferol and soy isoflavones supplementation on bone mineralisation of quail. Br Poult Sci. (2009) 50:709–15. doi: 10.1080/00071660903261944
29. Filipa Almeida A, Borge GIA, Piskula M, Tudose A, Tudoreanu L, Valentova KG, et al. Bioavailability of quercetin in humans with a focus on interindividual variation. Compr Rev Food Sci Food Saf. (2018) 17:714–31. doi: 10.1111/1541-4337.12342
30. Reyes-Farias M, Carrasco-Pozo C. The anti-cancer effect of quercetin: molecular implications in cancer metabolism. Int J Mol Sci. (2019) 20:3177. doi: 10.3390/ijms20133177
31. Xu D, Hu MJ, Wang YQ, Cui YL. Antioxidant activities of quercetin and its complexes for medicinal application. Molecules. (2019) 24:1123. doi: 10.3390/molecules24061123
32. Chen XH, Shi ZG, Lin HB, Wu F, Zheng F, Wu CF, et al. Resveratrol alleviates osteoporosis through improving the osteogenic differentiation of bone marrow mesenchymal stem cells. Eur Rev Med Pharmacol Sci. (2019) 23:6352–59. doi: 10.26355/eurrev_201907_18459
33. Rauf A, Imran M, Khan IA, Mujeebur R, Gilani SA, Mehmood Z, et al. Anticancer potential of quercetin: a comprehensive review. Phytother Res. (2018) 32:2109–30. doi: 10.1002/ptr.6155
34. Farazuddin M, Mishra R, Jing Y, Srivastava V, Comstock AT, Sajjan US. Quercetin prevents rhinovirus-induced progression of lung disease in mice with COPD phenotype. PLoS ONE. (2018) 13:e0199612. doi: 10.1371/journal.pone.0199612
35. Landy N, Kheiri F, Faghani M, Bahadoran R. Investigation of different levels of cholecalciferol and its metabolite in calcium and phosphorus deficient diets on growth performance, tibia bone ash and development of tibial dyschondroplasia in broilers. Acta Sci Anim Sci. (2021) 43:e48816. doi: 10.4025/actascianimsci.v43i1.48816
36. Orsolic N, Jelec Z, Nemrava J, Balta V, Gregorovic G, Jelec D. Effect of quercetin on bone mineral status and markers of bone turnover in retinoic acid-induced osteoporosis. Pol J Food Nutr Sci. (2018) 68:149–62. doi: 10.1515/pjfns-2017-0023]
37. Tsuji M, Yamamoto H, Sato T, Mizuha Y, Kawai Y, Taketani Y, et al. Dietary quercetin inhibits bone loss without effect on the uterus in ovariectomized mice. J Bone Miner Metab. (2009) 27:673–81. doi: 10.1007/s00774-009-0088-0
38. Zhang HK, Mehmood K, Jiang X, Yao W, Iqbal M, Li K, et al. Effect of Icariin on tibial dyschondroplasia incidence and tibial characteristics by regulating P2RX7 in chickens. Biomed Res Int. (2018) 2018:6796271. doi: 10.1155/2018/6796271
39. Ahn H, Park YK. Soy isoflavone supplementation improves longitudinal bone growth and bone quality in growing female rats. Nutrition. (2017) 37:68–73. doi: 10.1016/j.nut.2016.12.008
40. Li YQ, Xing XH, Wang H, Weng XL, Yu SB, Dong GY. Dose-dependent effects of genistein on bone homeostasis in rats' mandibular subchondral bone. Acta Pharmacol Sin. (2012) 33:66–74. doi: 10.1038/aps.2011.136
41. Zhang HK, Mehmood K, Li K, Rehman MU, Jiang X, Huang S, et al. Icariin ameliorate thiram-induced tibial dyschondroplasia via regulation of WNT4 and VEGF expression in broiler chickens. Front Pharmacol. (2018) 9:123. doi: 10.3389/fphar.2018.00123
43. Gao N, Dou XJ, Yin T, Yang Y, Yan D, Ma ZW, et al. Tryptophan promotes intestinal immune defense through calcium-sensing receptor (CaSR)dependent metabolic pathways. J Agr Food Chem. (2021) 69:13460–73. doi: 10.1021/acs.jafc.1c05820
44. Peacock M. Calcium metabolism in health and disease. Clin J Am Soc Nephrol. (2010) 5(Suppl. 1):S23–30. doi: 10.2215/CJN.05910809
45. Kirk B, Prokopidis K, Duque G. Nutrients to mitigate osteosarcopenia: the role of protein, vitamin D and calcium. Curr Opin Clin Nutr. (2021) 24:25–32. doi: 10.1097/MCO.0000000000000711
46. Sahin N, Balci TA, Kucuk O, Smith MO, Sahin K. The effect of soy isoflavones on egg quality and bone mineralisation during the late laying period of quail. Br Poult Sci. (2007) 48:363–9. doi: 10.1080/00071660701341971
47. Xiao YQ, Shao D, Sheng ZW, Wang Q, Shi SR. A mixture of daidzein and Chinese herbs increases egg production and eggshell strength as well as blood plasma Ca, P, antioxidative enzymes, and luteinizing hormone levels in post-peak, brown laying hens. Poultry Sci. (2019) 98:3298–303. doi: 10.3382/ps/pez178
48. Shi SR, Gu H, Chang LL, Wang ZY, Tong HB, Zou JM. Safety evaluation of daidzein in laying hens: part I. Effects on laying performance, clinical blood parameters, and organs development. Food Chem Toxicol. (2013) 55:684–8. doi: 10.1016/j.fct.2013.01.009
49. Yang H, Choi KC, Hyun SH, Jeung EB. Coexpression and estrogen-mediated regulation of TRPV6 and PMCA1 in the human endometrium during the menstrual cycle. Mol Reprod Dev. (2011) 78:274–82. doi: 10.1002/mrd.21303
50. Chen Y, Gong X, Li G, Lin M, Huo Y, Li S, et al. Effects of dietary alfalfa flavonoids extraction on growth performance, organ development and blood biochemical indexes of Yangzhou geese aged from 28 to 70 days. Anim Nutr. (2016) 2:318–22. doi: 10.1016/j.aninu.2016.09.004
51. Areco V, Rivoira MA, Rodriguez V, Marchionatti AM, Carpentieri A, Tolosa de Talamoni N. Dietary and pharmacological compounds altering intestinal calcium absorption in humans and animals. Nutr Res Rev. (2015) 28:83–99. doi: 10.1017/S0954422415000050
52. van der Velde RY, Brouwers JRBJ, Geusens PP, Lems WF, van den Bergh JPW. Calcium and vitamin D supplementation: state of the art for daily practice. Food Nutr Res. (2014) 58:21796. doi: 10.3402/fnr.v58.21796
53. Wasserman RH. Vitamin D and the dual processes of intestinal calcium absorption. Nutr J. (2004) 134:3137–9. doi: 10.1093/jn/134.11.3137
54. Cui M, Li Q, Johnson R, Fleet JC. Villin promoter-mediated transgenic expression of transient receptor potential cation channel, subfamily V member 6 (TRPV6) increases intestinal calcium absorption in wild-type and vitamin D receptor knockout mice. J Bone Miner Res. (2012) 27:2097–107. doi: 10.1002/jbmr.1662
55. Han Y, Gao S, Muegge K, Zhang W, Zhou B. Advanced applications of RNA sequencing and challenges. Bioinform Biol Insights. (2015) 9:29–46. doi: 10.4137/BBI.S28991
56. Hrdlickova R, Toloue M, Tian B. RNA-seq methods for transcriptome analysis. Wires RNA. (2017) 8:10. doi: 10.1002/wrna.1364
57. Farrer RA, Kemen E, Jones JDG, Studholme DJ. De novo assembly of the Pseudomonas syringae pv. syringae B728a genome using Illumina/Solexa short sequence reads. FEMS Microbiol Lett. (2009) 291:103–11. doi: 10.1111/j.1574-6968.2008.01441.x
58. López Castel A, Joann Overby S, Artero R. MicroRNA-based therapeutic perspectives in myotonic dystrophy. Int J Mol Sci. (2019) 20:5600. doi: 10.3390/ijms20225600
59. Wang J, Zhao CC, Li J, Feng Y, Gong Y. Transcriptome analysis of the potential roles of FOXL2 in chicken pre-hierarchical and pre-ovulatory granulosa cells. Comp Biochem Physiol Part D Genomics Proteomics. (2017) 21:56–66. doi: 10.1016/j.cbd.2016.12.003
60. Yang JX, Maria TC, Zhou B, Xiao FL, Wang M, Mao YJ, et al. Quercetin improves immune function in Arbor Acre broilers through activation of NF-κB signaling pathway. Poultry Sci. (2020) 99:906–13. doi: 10.1016/j.psj.2019.12.021
61. Diaz de Barboza G, Guizzardi S, Moine L, Tolosa de Talamoni N. Oxidative stress, antioxidants and intestinal calcium absorption. World J Gastroenterol. (2017) 23:2841–53. doi: 10.3748/wjg.v23.i16.2841
62. Chen Y, Gondro C, Quinn K, Herd RM, Parnell PF, Vanselow B. Global gene expression profiling reveals genes expressed differentially in cattle with high and low residual feed intake. Anim Genet. (2011) 42:475–90. doi: 10.1111/j.1365-2052.2011.02182.x
63. Karisa B, Moore S, Plastow G. Analysis of biological networks and biological pathways associated with residual feed intake in beef cattle. Anim Sci J. (2014) 85:374–87. doi: 10.1111/asj.12159
64. Dergunova LV, Filippenkov IB, Stavchansky VV, Denisova AE, Yuzhakov VV, Mozerov SA, et al. Genome-wide transcriptome analysis using RNA-Seq reveals a large number of differentially expressed genes in a transient MCAO rat model. BMC Genomic. (2018) 19:655. doi: 10.1186/s12864-018-5039-5
65. Park W, Rengaraj D, Kil DY, Kim H, Lee HK, Song KD. RNA-seq analysis of the kidneys of broiler chickens fed diets containing different concentrations of calcium. Sci Rep. (2017) 7:11740. doi: 10.1038/s41598-017-11379-7
66. Zhuo Z, Lamont SJ, Abasht B. RNA-seq analyses identify additivity as the predominant gene expression pattern in F1 chicken embryonic brain and liver. Genes. (2019) 10:27. doi: 10.3390/genes10010027
67. Lu DS, Carson DA. Spiperone enhances intracellular calcium level and inhibits the Wnt signaling pathway. BMC Pharmacol. (2009) 9:13. doi: 10.1186/1471-2210-9-13
68. Feske S, Okamura H, Hogan PG, Rao A. Ca2+/calcineurin signalling in cells of the immune system. Biochem Bioph Res Co. (2003) 311:1117–32. doi: 10.1016/j.bbrc.2003.09.174
69. Hogan PG, Chen L, Nardone J, Rao A. Transcriptional regulation by calcium, calcineurin, and NFAT. Gene Dev. (2003) 17:2205–32. doi: 10.1101/gad.1102703
70. Kühl M, Sheldahl LC, Malbon CC, Moon RT. Ca(2+)/calmodulin-dependent protein kinase II is stimulated by Wnt and Frizzled homologs and promotes ventral cell fates in Xenopus. J Biol Chem. (2000) 275:12701–11. doi: 10.1074/jbc.275.17.12701
71. Martineau X, Abed É, Martel-Pelletier J, Pelletier JP, Lajeunesse D. Alteration of Wnt5a expression and of the non-canonical Wnt/PCP and Wnt/PKC-Ca2+ pathways in human osteoarthritis osteoblasts. PLoS ONE. (2017) 12:e0180711. doi: 10.1371/journal.pone.0180711
72. Kakkar R, Raju RV, Sharma RK. Calmodulin-dependent protein kinase II from bovine cardiac muscle: purification and differential activation by calcium. Cell Calcium. (1996) 20:347–53. doi: 10.1016/S0143-4160(96)90040-8
73. Koga T, Matsui Y, Asagiri M, Kodama T, de Crombrugghe B, Nakashima K, et al. NFAT and Osterix cooperatively regulate bone formation. Nat Med. (2005) 11:880–5. doi: 10.1038/nm1270
74. Kuroda Y, Hisatsune C, Nakamura T, Matsuo K, Mikoshiba K. Osteoblasts induce Ca2+ oscillation-independent NFATc1 activation during osteoclastogenesis. Proc Natl Acad Sci USA. (2008) 105:8643–8. doi: 10.1073/pnas.0800642105
75. Lyon AM, Tesmer JJ. Structural insights into phospholipase C-β function. Mol Pharmacol. (2013) 84:488–500. doi: 10.1124/mol.113.087403
76. Wong SK, Chin KY, Ima-Nirwana S. Quercetin as an agent for protecting the bone: a review of the current evidence. Int J Mol Sci. (2020) 21:6448. doi: 10.3390/ijms21176448
77. Pan FF, Shao J, Shi CF, Li ZP, Fu WM, Zhang JF, et al. Apigenin promotes osteogenic differentiation of mesenchymal stem cells and accelerates bone fracture healing via activating Wnt/I3-catenin signaling. Am J Physiol Endocrinol Metab. (2021) 320:E760–71. doi: 10.1152/ajpendo.00543.2019
78. Xi YD, Zhang DD, Ding J, Yu HL, Yuan LH, Ma WW, et al. Genistein inhibits a beta 25-35-induced synaptic toxicity and regulates CaMKII/CREB pathway in SH-SY5Y cells. Cell Mole Neurobiol. (2016) 36:1151–9. doi: 10.1007/s10571-015-0311-6
79. Tan Z, Cheng J, Liu Q, Zhou L, Kenny J, Wang T, et al. Neohesperidin suppresses osteoclast differentiation, bone resorption and ovariectomised-induced osteoporosis in mice. Mol Cell Endocrinol. (2017) 439:369–78. doi: 10.1016/j.mce.2016.09.026
Keywords: quercetin, broiler, calcium and phosphorus metabolism, Wnt signaling pathway, transcriptomics
Citation: Wang B, Wang S, Ding M, Lu H, Wu H and Li Y (2022) Quercetin Regulates Calcium and Phosphorus Metabolism Through the Wnt Signaling Pathway in Broilers. Front. Vet. Sci. 8:786519. doi: 10.3389/fvets.2021.786519
Received: 02 October 2021; Accepted: 22 December 2021;
Published: 27 January 2022.
Edited by:
Ewa Tomaszewska, University of Life Sciences of Lublin, PolandReviewed by:
Fazul Nabi, Lasbela University of Agriculture, Water and Marine Sciences, PakistanAli Raza Jahejo, Shanxi Agricultural University, China
Fernando Tavernari, Brazilian Agricultural Research Corporation (EMBRAPA), Brazil
Copyright © 2022 Wang, Wang, Ding, Lu, Wu and Li. This is an open-access article distributed under the terms of the Creative Commons Attribution License (CC BY). The use, distribution or reproduction in other forums is permitted, provided the original author(s) and the copyright owner(s) are credited and that the original publication in this journal is cited, in accordance with accepted academic practice. No use, distribution or reproduction is permitted which does not comply with these terms.
*Correspondence: Yao Li, bGl5YW9sencmI3gwMDA0MDsxNjMuY29t