- Heilongjiang Provincial Key Laboratory of Prevention and Control of Bovine Diseases, College of Animal Science and Veterinary Medicine, Heilongjiang Bayi Agricultural University, Daqing, China
We evaluated the efficacy of three vaccine formulations containing different combinations of proteins (43K OMP, leukotoxin recombinant protein PL4 and hemolysin recombinant protein H2) and killed whole cell Fusobacterium necrophorum in preventing liver abscess. Four subcutaneous vaccines were formulated: vaccine 1 (43K OMP), vaccine 2 (PL4 and H2), vaccine 3 (43K OMP, PL4 and H2), and vaccine 4 (killed whole bacterial cell). 43K OMP, PL4, and H2 proteins were produced by using recombinant protein expression. To evaluate vaccine efficacy, we randomly allocated 50 BALB/c female mice to one of five different treatment groups: PBS control group, vaccine 1, vaccine 2, vaccine 3, and vaccine 4. Mice were vaccinated three times, with 14 days between each immunization. After immunization, the mice were challenged with F. necrophorum. The three key findings of this study are as follows: (1) Vaccine 3 has enabled mice to produce higher antibody titer following bacterial challenge, (2) in the liver pathology of mice, the vaccine 3 liver showed the least pathology, and (3) all four vaccines produced high levels of antibodies and cytokines in mice, but the level of vaccine 3 was the highest. Based on our results, it has been demonstrated that a mixture of F. necrophorum 43K OMP, PL4, and H2 proteins inoculated with mice can achieve protection against liver abscess in mice. Our research may therefore provide the basis for the development of a vaccine against F. necrophorum bovine infections.
Introduction
Fusobacterium necrophorum is a Gram-negative, anaerobic bacterium. F. necrophorum can cause necrotic and purulent diseases in cattle, sheep, pigs and humans (1–5). F. necrophorum mainly cause liver abscess in beef cattle and footrot in dairy cows. Liver abscess are almost always caused by co-infection with F. necrophorum and several bacterial species, with F. necrophorum in the rumen being the main pathogen of liver abscess, followed by Trueperella pyogenes (6). In recent years, studies have found that F. necrophorum is involved in the occurrence of dairy cow mastitis, endometritis, interdigital dermatitis, and esophageal ulcers (7–11). F. necrophorum are very harmful to cattle and sheep farming, the most important of which are liver abscess and footrot caused by F. necrophorum, which hasten the culling of animals and reduce their economic value. This has led to a deeper interest in vaccine research for F. necrophorum and vaccine development has become urgent.
F. necrophorum possesses or secretes a number of virulence factors, which include the following: leukotoxin, endotoxic lipopolysaccharide (LPS), hemolysin, hemagglutinin, capsule, adhesins or outer membrane proteins, platelet aggregation factor, dermonecrotic toxin, and several extracellular enzymes (12). The main virulence factors of F. necrophorum are leukotoxin, hemolysin and outer membrane proteins (OMPs). Among them, leukotoxin is an extracellular secretory protein, F. necrophorum's most important virulence factor, which can inhibit the phagocytosis of neutrophils and Kupffer cells (13–15). Studies have shown that the amount of leukotoxin secreted by F. necrophorum correlates positively with the severity of liver abscess in cattle (16).
Erythrocytes are able to be lysed by F. necrophorum and the virulence factor that performs the main function is the secreted protein hemolysin (17–20). Studies have shown that natural F. necrophorum hemolysin can be used to immunize rabbits and has an immunoprotective effect (21). The OMPs of Gram-negative bacteria play an important role in bacterial adhesion and infection in the initial stage of bacterial infection (22–25). The functions of OMPs are mainly structural maintenance, material transport, adhesion and induction of protective immunity. Subcutaneous immunization with recombinant proteins (FimH, LKT, PLO) of three key virulence factors of E. coli, F. necrophorum, and Trueperella pyogenes was found to be effective in preventing endometritis in cattle (26). We discovered an outer membrane protein of F. necrophorum in 2013, designated as 43K OMP. The results of cell adhesion experiments showed that the protein adheres to host target cells (27, 28). Recombinant protein PL4 (60 kDa) of F. necrophorum leukotoxin alone induces good immune protection in mice against F. necrophorum infection as vaccine (18). We expressed a recombinant protein H2 (30 kDa) of F. necrophorum hemolysin that reacts with F. necrophorum polyclonal antibodies and has good antigenicity in 2015 (21). In the evaluation of vaccine immunity, cellular and humoral immunity cytokines are usually used as indicators. In our experiments, we measured the cellular immunity-related cytokines IL-2, IFN-γ, IL-1β, the humoral immunity-related cytokines IL-4, IL-10, TNF-α in order to evaluate the effectiveness of the recombinant protein 43K OMP+PL4+H2 vaccine from F. necrophorum in immunized mice.
Therefore, our aim was to immunize mice with a mixture of purified F. necrophorum recombinant protein 43K OMP, leukotoxin PL4, and hemolysin H2. The immunization effect of 43K OMP, PL4, and H2 was determined by assaying cytokines, antibody titers, liver bacterial load, and pathological findings.
Materials and Methods
Ethics Statement
In this experiment, female BALB/c mice, approximately 6 weeks old and weighing 20 g each, were purchased from Changchun Yisi Experimental Animal Technology Co., Ltd. All experiments were approved by the Heilongjiang Bayi Agricultural University, which were conducted in accordance with the regulations of the College of Animal Science & Veterinary Medicine. All efforts were made to minimize animal suffering and to reduce the number of animals used.
Vaccine Preparation
Fusobacterium necrophorum subsp. necrophorum A25286 (purchased from ATCC Company, ATCC 25286, Manassas, VA, USA) (Hereafter referred to as A25) was added to the Fastidious Anaerobe Broth (Qingdao Hope Bio-Technology Co., Ltd, China, FAB) medium at a ratio of 1:100 and cultured in an anaerobic incubator for 24–36 h at 37°C, and grown to an absorbance of 0.6–0.7 at OD600nm. Neutral formaldehyde was added to the cultivated F. necrophorum to a final concentration of 0.2%, and the inactivation continued for 24 h at 37°C in an anaerobic incubator. The killed whole bacterial cell suspension was inoculated in FAB medium at 1:100 and cultured under anaerobic conditions for 24 h to determine whether bacterial growth occurred. If the bacteria did not grow, the inactivation was considered successful.
The recombinant proteins used in our vaccine preparation include: F. necrophorum outer membrane protein 43K OMP, leukotoxin PL4, and hemolysin H2. The results of prokaryotic expression and purification were reported in our previous study (18, 21, 28).
Immunized Mice
Female BALB/c mice weighing approximately 20 g each were randomly divided into five groups: vaccine 1 (43K OMP), vaccine 2 (PL4 and H2), vaccine 3 (43K OMP, PL4 and H2), vaccine 4 (killed whole bacterial cell) and PBS control group (phosphate buffer saline), with ten mice per group. Animals were housed in individual ventilated caging system (IVC) with wood chip bedding. With SPF class housing conditions, all males were housed in 5 animals/cage. The temperature was maintained at 24 ± 1°C and free access to water and rodent food (Beijing Keao Xieli Feed Co., Ltd., China). Each animal was immunized by multi-point dorsal subcutaneous injection with a volume of 0.25 mL and Immunity 25 μg total protein (Table 1). The first immunization was emulsified with Freund's complete adjuvant, and the second and third immunizations were emulsified with Freund's incomplete adjuvant. The interval between each immunization was 2 weeks.
Bacterial Challenge
The F. necrophorum A25 strain was cultivated, bacterial cells were inoculated into pre-reduced, anaerobically sterilized brain-heart infusion (Qingdao Hope Bio-Tcehnology Co., Ltd, China, BHI) broth. The bacterial cells were centrifuged at 3,000 × g for 25 min and suspended to 1 × 107 CFU/mL with physiological saline (Short-term buffering in saline would not cause F. necrophorum death). The treated F. necrophorum was injected intraperitoneally in mice at a dose of 0.25 mL/mouse. After the mice were challenged, the death of the mice was observed and recorded. Blood is collected from mice, which are euthanized on the 7 days after the challenge. Mice were aseptically dissected at a sterilized ultra-clean workbench.
ELISA Detection of Mouse Antibody Titer
Vaccine 1 (43K OMP), vaccine 2 (PL4 and H2), vaccine 3 (43K OMP, PL4 and H2), and vaccine 4 (Killed whole bacterial cell). Except for vaccine 4, the respective capture antigens for each group of vaccines were the immunized proteins. The capture antigen of vaccine 4 is the F. necrophorum. The coating protein (0.05 mol/L carbonate buffer) was used to dilute the antigen protein to 1 μg/mL. Killed whole bacterial cell was diluted with the coating buffer, and the diluted substrate was added to an ELISA plate at 100 μL per well. The plate was sealed with film and incubated overnight at 4°C.
After coating overnight, the remaining liquid in the well was discarded, and 300 μL of washing buffer (0.05% PBS-T) was added to each well. After washing for 5 min by shaking, the washing buffer was discarded; the washing was repeated five times, and the liquid in the well was patted away after the last wash. Then 200 μL of blocking buffer (5% skim milk) was added to each well, and the plate was sealed and placed in a 37°C incubator for 2 h.
After blocking, excess blocking buffer was discarded, and the plate was washed with washing buffer five times, and excess liquid was patted away. Mouse serum was diluted in 2-fold gradient multiples with PBS and added to the enzyme-labeled plate. Then 100 μL of the diluted mouse serum was added to each well. The plate was sealed and incubated at 37°C for 1 h. Excess liquid was discarded. The plate was washed with washing buffer five times and patted dry. Specific secondary antibodies (dilution 1:5 000), coupled with HRP, were added to wells. For total IgG determination, goat anti-mouse IgG (H+L) -HRP antibodies (Labgic Technology Co., Ltd., China) were buffered into PBS. A volume of 100 μL was added to each well, and the plate was incubated for 1 h at 37°C.
After the secondary antibody incubation, the liquid was discarded, the plate was washed five times, and the liquid in the wells was patted away. Then 100 μL of TMB single-component color developing buffer was added to each well, and the plate was incubated at room temperature for 10–15 min in the dark. Finally, 100 μL of stop buffer (2 mol/L H2SO4) was added. The absorbance was measured with a microplate reader and when OD450nm>1.0, the serum dilution at this point was the antibody titer.
Mouse Cytokine Detection
Cytokines (IL-4, IL-10, TNF-α) were estimated in mouse serum to study the humoral mediated immune responses and Cytokines (IL-2, IFN-γ, IL-1β) were estimated in mouse serum to study the cell mediated immune responses. Cytokines associated with the promotion of inflammation were detected. To determine whether mixed immunization with F. necrophorum leukotoxin PL4, hemolysin H2, and outer membrane protein 43K OMP can induce both cellular and humoral immunity in mice, we assayed the above six cytokines with ELISA kit (Kete Biotechnology Co., China) (Table 2).
Bacterial Loads in Mouse Liver
Mice liver collected aseptically was weighed and placed in a tissue grinder, and 3 mL of physiological saline was added. The tissue was ground into homogenate, which was diluted with normal saline at ratios of 1: 10, 1: 100, 1: 1 000, and 1: 10 000. A 20 μL volume of the diluted tissue homogenate was spread on anaerobic solid medium and incubated for 24 h. The number of single colonies in the plate was recorded, with the number of colonies between 30 and 200, and the bacterial load of the mouse liver was calculated.
Histopathology of Liver
Collected liver was soaked in 4% formaldehyde solution for later use. Tissue blocks were trimmed to 1 × 1 × 0.5 cm to make paraffin sections and all liver lobes processed for histological study and stained with Hematoxylin-Eosin staining (H&E) stain. After dyeing, the slices were dehydrated, rendered transparent, mounted and observed under a microscope after drying.
Statistical Analysis
All experiments were repeated at least three times with similar results. Graphs and data analysis were performed using performed using one-way analysis of variance (ANOVA) using GraphPad Prism 9 (GraphPad Software), using Tukey's post-hoc test. The data are expressed as the mean ± standard error of the mean (SEM); p < 0.05, p < 0.01, and p < 0.001 were considered statistically significant.
Results
Clinical Symptoms
We recorded the deaths of F. necrophorum A25 strain infected mice. The mice were immunized and observed to be free of abnormalities. However, after F. necrophorum challenge, all groups showed various degrees of clinical symptoms, including less severe symptoms in the vaccine 3 group compared to vaccine 1 and vaccine 2, with only erected fur that returned to normal after 3 days; Vaccine 4 presents with depression and decreased desire to eat and drink, but returns to normal after a few days; In the PBS group, in additional to the above conditions, the abdomens on both sides were sunken inwards, movement was difficult and three mice died.
Cytokine Level Detection
To determine the pattern of humoral and cellular immune responses following immunization in mice. Cytokines related to cellular (IL-2, IFN-γ, IL-1β) and humoral immunity (IL-4, IL-10) showed an upward trend in the four groups of vaccine immunization (Figure 1). The difference in TNF-α was not particularly significant between several groups while IL-1β showed an increasing trend (Figure 1). After the third immunization, the levels of cytokines in the vaccine 3 were higher than those in the vaccine 1 and the vaccine 2. The difference between the vaccine group and the control group was significant (** P ≤ 0.01).
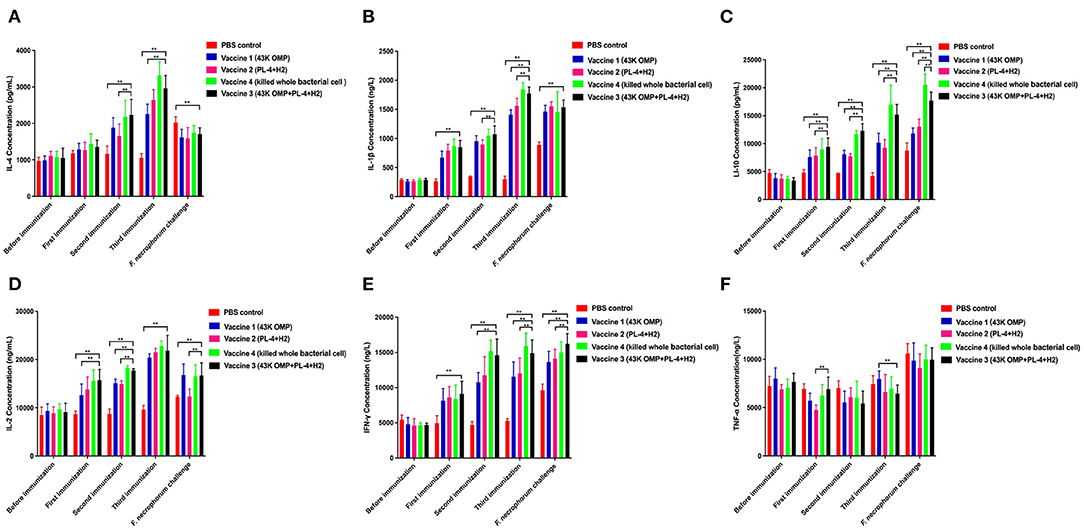
Figure 1. Detection results of cytokines in mouse serum. (A) Detection of IL-4 in mice. (B) Detection of IL-1β in mice. (C) Detection of IL-10 in mice. (D) Detection of IL-2 in mice. (E) Detection of INF-γ in mice. (F) Detection of TNF-α in mice. Data are represented as mean ± SEM of three replicates. *A result significantly different (*P ≤ 0.05 and **P ≤ 0.01) from their respective control. All groups of mice serum were collected the day before the next immunization for cytokine detection.
Antibody Titer Test
In past studies for PL4 protein we found that antibody titer reached more than 1:12,800 after 48 days of immunization in mice (18) and the H2 protein has been shown to have excellent antigenicity and can be used in studies of F. necrophorum vaccines (21). Based on the above results, the vaccine was immunized in mice BALB/c and the antibody titer was measured after 48 days as follows. After each immunization, mouse serum was collected for antibody titer detection. The results are shown in Figure 2A. The vaccine group produced higher antibody levels against F. necrophorum. After 48 days of immunization, Antibody titers reached 1:128 000 in the vaccine 1 (43K OMP), 1:120 000 in the vaccine 2 (PL4 + H2) and 1:120 000 in the vaccine 3 (43K OMP + PL4 + H2) titers in the vaccine 4 (Killed whole cell F. necrophorum) were 1:25 600, while antibody titer in the PBS control group was below 1: 400. The 43K OMP protein immunization in mice had a role in immune protection and stimulated the body's immune response. When 43K OMP was combined with PL4 and H2, the immune protection effect was improved.
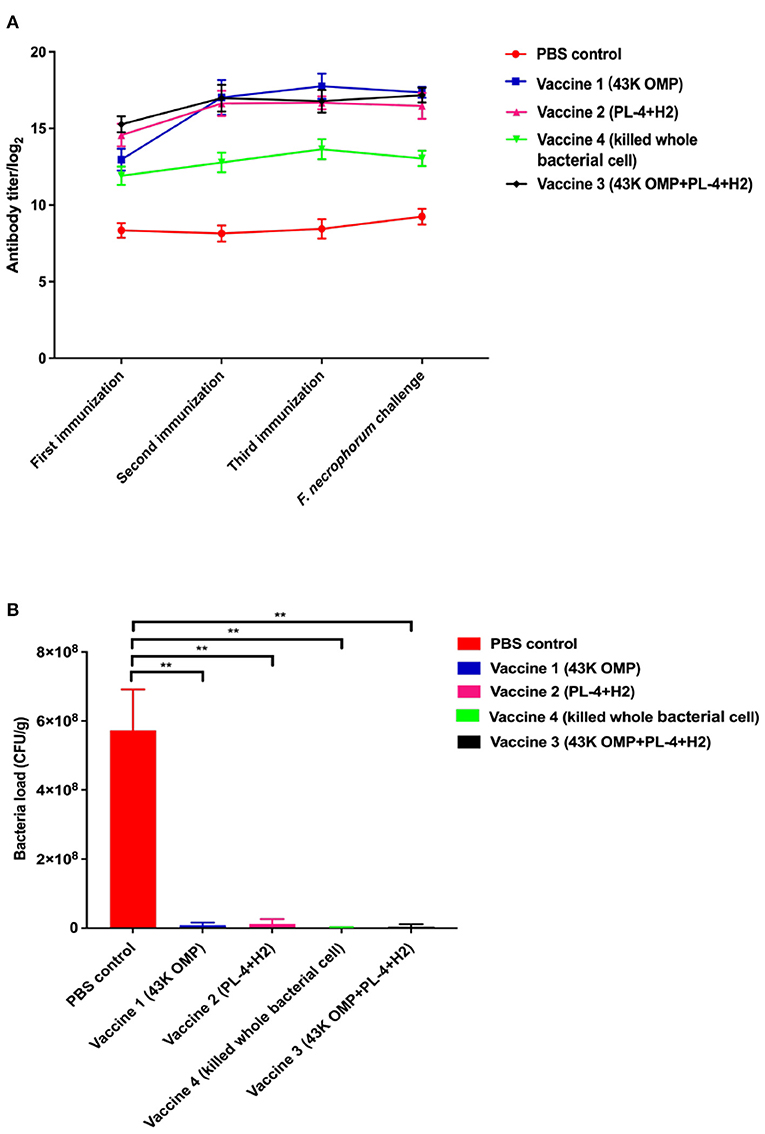
Figure 2. (A) Antibody levels in mouse serum. (B) Number of bacteria in mouse liver. Data are represented as mean ± S.E.M. of three replicates. *A result significantly different (*P ≤ 0.05 and **P ≤ 0.01) from their respective control.
Bacterial Load in Mouse Liver
Eight days after infection, the mice were euthanized, and the livers were collected for liver bacterial load experiments. In this experiment, two mice died in the PBS group after the mice were challenged. The results of the mouse liver bacterial load experiments are shown in Figure 2B. The PBS control group had a significantly higher bacterial load of F. necrophorum liver compared to the vaccine groups. Of these five groups, vaccine 4 had the lowest liver bacterial load. The bacterial loads among the groups are shown in Table 3.
Mouse Liver Histopathology
In this experiment, the mice were euthanized, and the livers were collected. Paraffin sections were made and stained with H&E. The pathological changes in mouse liver were observed under a microscope (Figure 3). The livers in the PBS control group showed severe congestion and bleeding, and clear cellular degeneration. Liver cells in the 43K OMP + PL4 + H2 group, 43K OMP group, and PL4 + H2 group showed slight granular degeneration. Compared with the four vaccines group, the PBS control group had more severe liver lesions.
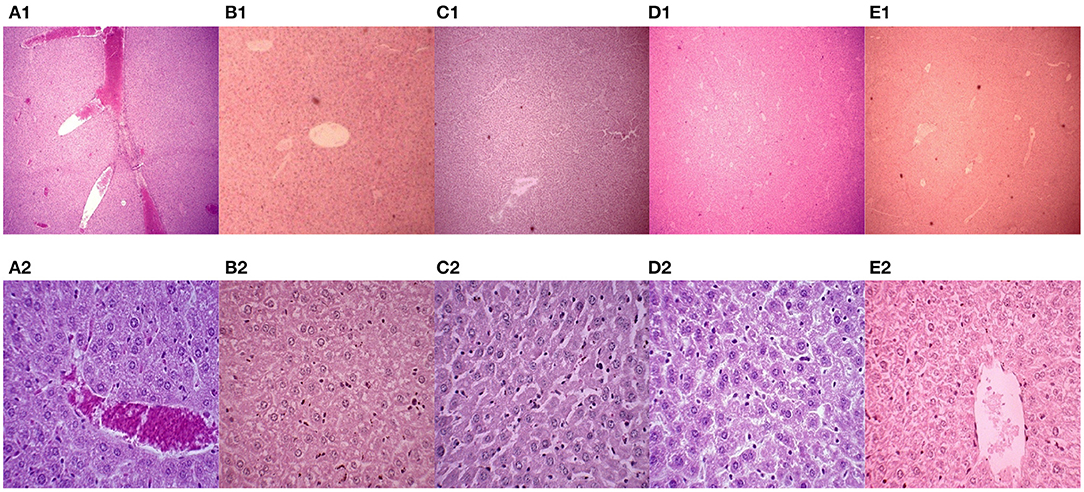
Figure 3. Mouse liver pathology (H.E. 40 ×). (A1–E1) Mouse liver pathology (H.E. 40 ×); (A2-E2) Mouse liver pathology (H.E. 400 ×). (A) PBS control group. (B) vaccine 1 (43K OMP). (C) vaccine 2 (PL4 and H2). (D) vaccine 4 (killed whole cell F. necrophorum). (E) vaccine 3 (43K OMP, PL4 and H2).
Discussion
In this study, we evaluated the effects of four subcutaneous vaccine formulations on liver health in mice based on the results of previous studies on the efficacy of recombinant leukotoxin vaccines from F. necrophorum by our class research group. Although the leukotoxin single-component vaccine of Sun et al. (18) had good immune protection, we found that the antibody titers of the multi-component vaccine (43K OMP+PL4+H2 group) were higher than those of the leukotoxin single-component vaccine. This may be due to the fact that F. necrophorum can secrete multiple virulence factors, and immune protection is more comprehensive after immunization of mice with recombinant subunit vaccines associated with multiple virulence factors. F. necrophorum leukotoxin was shown to prevent metritis with virulence proteins of Escherichia coli and Trueperella pyogenes as a multi-component vaccine (9, 26, 29). Aeromonas outer membrane protein A (ompA) and hemolysins (hly) were assembled into poly (lactic-co-glycolic) acid (PLGA) carrier vaccines with a strong and stable IgG response (30), which is the protective immune carrier system of Aeromonas. In our study, three recombinant proteins, 43K OMP, PL4, and H2, were purified as vaccine components for immunized mice, and regardless of the 43K OMP group, PL4+H2 group, and 43K OMP+PL4+H2 group, all produced high antibody titers in mice, which were significantly higher than those of killed whole cell F. necrophorum group and PBS control group. High levels of a total of IgG help the body resist and clear F. necrophorum infections. Such results demonstrated that the multi-component recombinant protein vaccine (43K OMP group, PL4+H2 group, 43K OMP+PL4+H2 group) was able to stimulate the increase of IgG level in mice, and the antibody titers reached the peak after 48 days of immunization.
We measured the levels of the immune-related cytokines IL-4, IL-2, IL-10, IL-1β, IFN-γ, and TNF-α. After the third immunization, IL-4 levels in the 43K OMP + PL4 + H2 group were significantly different compared to the PBS control group (** P ≤ 0.01) and showing an upward trend. It has been shown that IL-4 induces differentiation to Th2 (31, 32), gene transcription and anti-apoptosis, and activates B cells to produce more specific antibodies to IgG (33). Elevated IgG levels in the vaccine 4 group were also found in our study. It has been shown that IL-4 induces differentiation to Th2 (30, 31)], gene transcription and anti-apoptosis, and activates B cells to produce more specific antibodies to IgG.
The IL-2 levels in each of the three immunization tended to increase, and the 43K OMP+PL4+H2 group was significantly higher than the PBS control group, which may be due to the fact that the components in 43K OMP+PL4+H2 group stimulate the production of high levels of IL-2 by CD4+ and CD8+ T cells, which are known to produce high levels of IL-2 under the activation of immune antigens (34–36), in terms of immune stimulation in terms of IL-2, it promotes the proliferation and survival of CD4+ and CD8+ effector T cells, the differentiation of naive T cells, the growth and differentiation of activated B cells, and the proliferation and function of natural killer (NK) cells (37–41). Thus, mouse T cells may be stimulated by the 43K OMP+PL4+H2 group to produce the corresponding immune response. We suspect that the decrease in IL-4 levels after bacterial challenge, except in PBS control group, is due to the suppression of Th2 cell responses after bacterial infection. IL-10 is a cytokine with anti-inflammatory properties that plays a central role in infections by limiting the immune response to pathogens, thereby preventing damage to the host [42]. In our experiment, the reason for the persistent elevation of IL10 levels after challenge may be that pathogenic bacteria and antigens can activate dendritic cells (DCs), macrophages and neutrophils to trigger IL-10 expression in vivo (42–44). IL-4 and IL-10 are produced by Th2 cells and mainly mediate the humoral immune response. In the results of the study, IL-10, INF-γ and TNF-α, all showed an upward trend after challenging bacteria on mice, probably due to bacterial invasion stimulating the expression of organism-related cytokines. IFN-γ in mouse serum was consistently elevated during three immunizations and bacterial challenge. IFN-γ alone cannot fight infection and requires IL-2, IL-4, and GM-CSF to act synergistically (45, 46). Moreover, activated macrophages can release TNF-α, and IL-2 and IFN-γ synergistically can also induce TNF-α secretion (47). IL-1β is mainly produced by monocytes and macrophages, and is overexpressed in chronic local inflammation. Cytokines do not function in isolation in the body; instead, they regulate one another's synthesis and secretion and mutually regulate receptor expression. In this experiment, the levels of cytokines in the vaccine 1, vaccine 2, vaccine 3, and vaccine 4 showed an upward trend after immunization.
After we performed the F. necrophorum challenge, Vaccine 3 showed the best immune protection, although no mice died in any group other than the PBS group. This was evidenced by little change in clinical signs and liver pathology in Vaccine 3 and a sustained increase in antibody titers following immunization. In the histopathological findings of the liver, the liver of the PBS control group after challenge showed massive hemorrhage and marked hepatocyte degeneration. The immunized 43K OMP + PL4 + H2 group showed little pathological changes compared to the 43K OMP group, PL4 + H2 group and killed whole bacterial cell group. The PBS control group had the highest liver bacterial load within these groups at 5.91 ×108 CFU/g after 7 days of challenge. The above results demonstrate that 43K OMP + PL4 + H2 group, 43K OMP group, PL4 + H2 group, and killed whole cell F. necrophorum group stimulate the immune response in mice. At present, studies on subunit vaccines for F. necrophorum are still in the preliminary stage, and since Gram-negative bacteria secrete outer membrane vesicles (OMVs) containing multiple virulence factors, we suspect that significant immune effects may be achieved if the OMVs of F. necrophorum can be extracted and prepared as vaccines.
Immunization with 43K OMP + PL4 + H2 group stimulated the immune response in mice better than the 43K OMP group. Because the virulence factors such as leukotoxin, hemolysin, and outer membrane protein were mainly released by F. necrophorum infected mice, the recombinant protein of multiple virulence factors of mice was more effective against F. necrophorum infection.
The developed multicomponent recombinant subunit vaccine against F. necrophorum and outer membrane protein 43 kDa OMP, leukotoxin PL4 and hemolysin H2 can produce higher antibody levels compared to single component subunit vaccines and can induce elevated levels of relevant cytokines in the body to stimulate the body's immune response, with good protection in mice after F. necrophorum challenge. This study will provide a good preliminary basis for the development of F. necrophorum vaccine.
Data Availability Statement
The original contributions presented in the study are included in the article/supplementary material, further inquiries can be directed to the corresponding authors.
Ethics Statement
The animal study was reviewed and approved by Heilongjiang Bayi Agricultural University.
Author Contributions
DG contributed to research ideas. JX, JJ, and XH contributed to experimental work, manuscript writing data analysis, and revised the manuscript. SZ, ZW, FW, and LW contributed to experimental work. All authors contributed to the article and approved the submitted version.
Funding
This work was supported by the Natural Science Foundation of Heilongjiang Province, China (Grant No. LH2021C070).
Conflict of Interest
The authors declare that the research was conducted in the absence of any commercial or financial relationships that could be construed as a potential conflict of interest.
Publisher's Note
All claims expressed in this article are solely those of the authors and do not necessarily represent those of their affiliated organizations, or those of the publisher, the editors and the reviewers. Any product that may be evaluated in this article, or claim that may be made by its manufacturer, is not guaranteed or endorsed by the publisher.
Acknowledgments
The authors thank Prof. Yung-Fu Chang of the Comell University for providing the research idea.
References
1. Langworth BF. Fusobacterium necrophorum: its characteristics and role as an animal pathogen. Bacteriol Rev. (1977) 41:373–90. doi: 10.1128/br.41.2.373-390.1977
2. Reymond B, Huette P, Roger PA, Tredez E, Gagneur O, Sanguin S, et al. Fatal Fusobacterium necrophorum infection with gynecological Lemierre's syndrome. Med Mal Infect. (2019) 49:72–4. doi: 10.1016/j.medmal.2018.09.006
3. Gelbart M, Bilavsky E, Chodick G, Raveh E, Levy I, Ashkenazi-Hoffnung L. Fusobacterium necrophorum as an emerging pathogen of acute mastoiditis. Pediatr Infect Dis J. (2019) 38:12–5. doi: 10.1097/INF.0000000000002021
4. Atia J, Monaghan E, Kaler J, Purdy K, Green L, Keeling M. Mathematical modeling of ovine footrot in the UK: the effect of Dichelobacter nodosus and Fusobacterium necrophorum on the disease dynamics. Epidemics. (2017) 21:13–20. doi: 10.1016/j.epidem.2017.04.001
5. Nygren D, Holm K. Invasive infections with Fusobacterium necrophorum including Lemierre's syndrome: an 8-year Swedish nationwide retrospective study. Clin Microbiol Infect. (2020) 26:1089 e7–1089.e12. doi: 10.1016/j.cmi.2019.12.002
6. Elwakeel EA, Amachawadi RG, Nour AM, Nasser ME, Nagaraja TG, Titgemeyer EC. In vitro degradation of lysine by ruminal fluid-based fermentations and by Fusobacterium necrophorum. J Dairy Sci. (2013) 96:495–505. doi: 10.3168/jds.2012-5810
7. Francis AM, Jeon SJ, Cunha F, Jeong KC, Galvao KN. Draft genome sequences of two Fusobacterium necrophorum strains isolated from the uterus of dairy cows with metritis. Microbiol Resour Announc. (2019) 8:e00201–19. doi: 10.1128/MRA.00201-19
8. Braga Paiano R, Bonilla J, Moro de Sousa RL, Micke Moreno A, Sampaio Baruselli P. Chemical composition and antibacterial activity of essential oils against pathogens often related to cattle endometritis. J Infect Dev Ctries. (2020) 14:177–83. doi: 10.3855/jidc.12076
9. Bicalho ML, Machado VS, Oikonomou G, Gilbert RO, Bicalho RC. Association between virulence factors of Escherichia coli, Fusobacterium necrophorum, and Arcanobacterium pyogenes and uterine diseases of dairy cows. Vet Microbiol. (2012) 157:125–31. doi: 10.1016/j.vetmic.2011.11.034
10. Tosaki K, Kojima H, Akama S, Ootake Y, Inoue K, Katsuda K, et al. Bovine esophageal and glossal ulceration associated with Pseudomonas aeruginosa and Fusobacterium spp. in a 10-month-old Holstein heifer. J Vet Med Sci. (2018) 80:1174–8. doi: 10.1292/jvms.17-0616
11. Staton GJ, Sullivan LE, Blowey RW, Carter SD, Evans NJ. Surveying bovine digital dermatitis and non-healing bovine foot lesions for the presence of Fusobacterium necrophorum, Porphyromonas endodontalis and Treponema pallidum. Vet Rec. (2020) 186:450. doi: 10.1136/vr.105628
12. Nagaraja TG, Narayanan SK, Stewart GC, Chengappa MM. Fusobacterium necrophorum infections in animals:pathogenesis and pathogenic mechanisms. Anaerobe. (2005) 11:239–46. doi: 10.1016/j.anaerobe.2005.01.007
13. Emery DL, Vaughan JA, Clark BL, Stewart DJ, Stewart DJ. Virulence determinants of Fusobacterium necrophorum and their prophylactic potential in animals. (1986) 267–274
14. Tan ZL, Nagaraja TG, Chengappa MM. Fusobacterium necrophorum infections:virulence factors, pathogenic mechanism and control measures. Vet Res Commun. (1996) 20:113–40. doi: 10.1007/BF00385634
15. Narayanan SK, Nagaraja TG, Chengappa MM, Stewart GC. Leukotoxins of gram-negative bacteria. Vet Microbiol. (2002) 84:337–56. doi: 10.1016/S0378-1135(01)00467-9
16. Pillai DK, Amachawadi RG, Baca G, Narayanan SK, Nagaraja TG. Leukotoxin production by Fusobacterium necrophorum strains in relation to severity of liver abscesses in cattle. Anaerobe. (2021) 69:102344. doi: 10.1016/j.anaerobe.2021.102344
17. Narayanan S, Stewart GC, Chengappa MM, Willard L, Shuman W, Wilkerson M, et al. Fusobacterium necrophorum leukotoxin induces activation and apoptosis of bovine leukocytes. Infect Immun. (2002) 70:4609–20. doi: 10.1128/IAI.70.8.4609-4620.2002
18. Sun DB, Wu R, Li GL, Zheng JS, Liu XP, Lin YC, et al. Identification of three immunodominant regions on leukotoxin protein of Fusobacterium necrophorum. Vet Res Commun. (2009) 33:749–55. doi: 10.1007/s11259-009-9223-6
19. Shinjo T, Fujisawa T, Mitsuoka T. Proposal of two subspecies of Fusobacterium necrophorum (Flugge) Moore and Holdeman:Fusobacterium necrophorum subsp. necrophorum subsp. nov., nom. rev. (ex Flugge 1886), and Fusobacterium necrophorum subsp. funduliforme subsp. nov., nom. rev. (ex Halle 1898). Int J Syst Bacteriol. (1991) 41:395–7. doi: 10.1099/00207713-41-3-395
20. Guo DH, Sun DB, Wu R, Yang HM, Zheng JS, Fan CL, et al. An indirect ELISA for serodiagnosis of cattle footrot caused by Fusobacterium necrophorum. Anaerobe. (2010) 16:317–20. doi: 10.1016/j.anaerobe.2010.03.007
21. Sun D, Lv S, Gao J, Guo D, Wang J, Wu R, et al. Fusobacterium necrophorum hemolysin gene cloning, expression and antigenicity analysis. Heilongjiang Animal Science and Veterinary Medicine. (2015) 01:140–120 (In Chinese).
22. Soto GE, Hultgren SJ. Bacterial adhesins: common themes and variations in architecture and assembly. J Bacteriol. (1999) 181:1059–71. doi: 10.1128/JB.181.4.1059-1071.1999
23. Chen T, Duncan MJ. Gingipain adhesin domains mediate Porphyromonas gingivalis adherence to epithelial cells. Microb Pathog. (2004) 36:205–9. doi: 10.1016/j.micpath.2003.12.001
24. Pumbwe L, Skilbeck CA, Wexler HM. The Bacteroides fragilis cell envelope:quarterback, linebacker, coach-or all three? Anaerobe. (2006) 12:211–20. doi: 10.1016/j.anaerobe.2006.09.004
25. Iyer D, Anaya-Bergman C, Jones K, Yanamandra S, Sengupta D, Miyazaki H, et al. AdpC is a Prevotella intermedia 17 leucine-rich repeat internalin-like protein. Infect Immun. (2010) 78:2385–96. doi: 10.1128/IAI.00510-09
26. Machado VS, Bicalho ML, Meira Junior EB, Rossi R, Ribeiro BL, Lima S, et al. Subcutaneous immunization with inactivated bacterial components and purified protein of Escherichia coli, Fusobacterium necrophorum, and Trueperella pyogenes prevents puerperal metritis in Holstein dairy cows. PLoS One. (2014) 9:e91734. doi: 10.1371/journal.pone.0091734
27. Sun D, Zhang H, Lv S, Wang H, Guo D. Identification of a 43-kDa outer membrane protein of Fusobacterium necrophorum that exhibits similarity with pore-forming proteins of other Fusobacterium species. Res Vet Sci. (2013) 95:27–33. doi: 10.1016/j.rvsc.2013.01.016
28. He X, Wang L, Li H, Zhang S, Wang Z, Jiang J, et al. Screening of BHK-21 cellular proteins that interact with outer membrane protein 43K OMP of Fusobacterium necrophorum. Anaerobe. (2020) 63:102184. doi: 10.1016/j.anaerobe.2020.102184
29. Meira EBS Jr, Ellington-Lawrence RD, Silva JCC, Higgins CH, Linwood R, Rodrigues MX, et al. Recombinant protein subunit vaccine reduces puerperal metritis incidence and modulates the genital tract microbiome. J Dairy Sci. (2020) 103:7364–76. doi: 10.3168/jds.2019-17006
30. Gao S, Zhao N, Amer S, Qian M, Lv M, Zhao Y, et al. Protective efficacy of PLGA microspheres loaded with divalent DNA vaccine encoding the ompA gene of Aeromonas veronii and the hly gene of Aeromonas hydrophila in mice. Vaccine. (2013) 31:5754–9. doi: 10.1016/j.vaccine.2013.08.053
31. Wang X, Lupardus P, Laporte SL, Garcia KC. Structural biology of shared cytokine receptors. Annu Rev Immunol. (2009) 27:29–60. doi: 10.1146/annurev.immunol.24.021605.090616
32. Junttila IS, Creusot RJ, Moraga I, Bates DL, Wong MT, Alonso MN, et al. Redirecting cell-type specific cytokine responses with engineered interleukin-4 superkines. Nat Chem Biol. (2012) 8:990–8. doi: 10.1038/nchembio.1096
33. LaPorte SL, Juo ZS, Vaclavikova J, Colf LA, Qi X, Heller NM, et al. Molecular and structural basis of cytokine receptor pleiotropy in the interleukin-4/13 system. Cell. (2008) 132:259–72. doi: 10.1016/j.cell.2007.12.030
34. Boyman O, Kovar M, Rubinstein MP, Surh CD, Sprent J. Selective stimulation of T cell subsets with antibody-cytokine immune complexes. Science. (2006) 311:1924–7. doi: 10.1126/science.1122927
35. Granucci F, Vizzardelli C, Pavelka N, Feau S, Persico M, Virzi E, et al. Inducible IL-2 production by dendritic cells revealed by global gene expression analysis. Nat Immunol. (2001) 2:882–8. doi: 10.1038/ni0901-882
36. Setoguchi R, Hori S, Takahashi T, Sakaguchi S. Homeostatic maintenance of natural Foxp3(+) CD25(+) CD4(+) regulatory T cells by interleukin (IL)-2 and induction of autoimmune disease by IL-2 neutralization. J Exp Med. (2005) 201:723–35. doi: 10.1084/jem.20041982
37. Boyman O, Sprent J. The role of interleukin-2 during homeostasis and activation of the immune system. Nat Rev Immunol. (2012) 12:180–90. doi: 10.1038/nri3156
38. Mingari MC, Gerosa F, Carra G, Accolla RS, Moretta A, Zubler RH, et al. Human interleukin-2 promotes proliferation of activated B cells via surface receptors similar to those of activated T cells. Nature. (1984) 312:641–3. doi: 10.1038/312641a0
39. Pulliam SR, Uzhachenko RV, Adunyah SE, Shanker A. Common gamma chain cytokines in combinatorial immune strategies against cancer. Immunol Lett. (2016) 169:61–72. doi: 10.1016/j.imlet.2015.11.007
40. Siegel JP, Sharon M, Smith PL, Leonard WJ. The IL-2 receptor beta chain (p70): role in mediating signals for LAK, NK, and proliferative activities. Science. (1987) 238:75–8. doi: 10.1126/science.3116668
41. Lenardo MJ. Interleukin-2 programs mouse alpha beta T lymphocytes for apoptosis. Nature. (1991) 353:858–61. doi: 10.1038/353858a0
42. Saraiva M, O'Garra A. The regulation of IL-10 production by immune cells. Nat Rev Immunol. (2010) 10:170–81. doi: 10.1038/nri2711
43. Chang EY, Guo B, Doyle SE, Cheng G. Cutting edge: involvement of the type I IFN production and signaling pathway in lipopolysaccharide-induced IL-10 production. J Immunol. (2007) 178:6705–9. doi: 10.4049/jimmunol.178.11.6705
44. Boonstra A, Rajsbaum R, Holman M, Marques R, Asselin-Paturel C, Pereira JP, et al. Macrophages and myeloid dendritic cells, but not plasmacytoid dendritic cells, produce IL-10 in response to MyD88- and TRIF-dependent TLR signals, and TLR-independent signals. J Immunol. (2006) 177:7551–8. doi: 10.4049/jimmunol.177.11.7551
45. Nacy CA, Meierovics AI, Belosevic M, Green SJ. Tumor necrosis factor-alpha: central regulatory cytokine in the induction of macrophage antimicrobial activities. Pathobiology. (1991) 59:182–4. doi: 10.1159/000163640
46. Vila-del Sol V, Punzon C, Fresno M. IFN-gamma-induced TNF-alpha expression is regulated by interferon regulatory factors 1 and 8 in mouse macrophages. J Immunol. (2008) 181:4461–70. doi: 10.4049/jimmunol.181.7.4461
Keywords: 43K OMP, hemolysin, leukotoxin, immunoprotective, Fusobacterium necrophorum
Citation: Xiao J, Jiang J, He X, Zhang S, Wang Z, Wang F, Wang L and Guo D (2021) Evaluation of Immunoprotective Effects of Fusobacterium necrophorum Outer Membrane Proteins 43K OMP, Leukotoxin and Hemolysin Multi-Component Recombinant Subunit Vaccine in Mice. Front. Vet. Sci. 8:780377. doi: 10.3389/fvets.2021.780377
Received: 21 September 2021; Accepted: 10 November 2021;
Published: 06 December 2021.
Edited by:
Massimo Amadori, Italian Network of Veterinary Immunology, ItalyReviewed by:
T. G. Nagaraja, Kansas State University, United StatesRaghavendra G. Amachawadi, Kansas State University, United States
Copyright © 2021 Xiao, Jiang, He, Zhang, Wang, Wang, Wang and Guo. This is an open-access article distributed under the terms of the Creative Commons Attribution License (CC BY). The use, distribution or reproduction in other forums is permitted, provided the original author(s) and the copyright owner(s) are credited and that the original publication in this journal is cited, in accordance with accepted academic practice. No use, distribution or reproduction is permitted which does not comply with these terms.
*Correspondence: Donghua Guo, ZGhfZ3VvJiN4MDAwNDA7MTI2LmNvbQ==
†These authors have contributed equally to this work