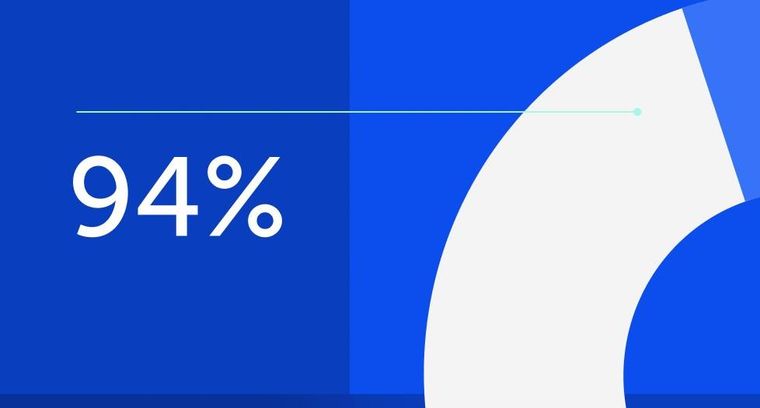
94% of researchers rate our articles as excellent or good
Learn more about the work of our research integrity team to safeguard the quality of each article we publish.
Find out more
ORIGINAL RESEARCH article
Front. Vet. Sci., 23 November 2021
Sec. Comparative and Clinical Medicine
Volume 8 - 2021 | https://doi.org/10.3389/fvets.2021.777579
The effects of cardiac disease on the intestine have been reported in humans but not in dogs. We investigated the effects of myxomatous mitral valve disease (MMVD), which is capable of causing congestion and tissue hypoperfusion, on the intestine in Chihuahuas, a breed frequently encountered in clinical practice as the preferred breed for MMVD. In this study, 69 Chihuahuas were divided into four groups based on echocardiography and chest radiography: 19 healthy Chihuahuas (H) and 50 Chihuahuas with MMVD classified according to the ACVIM consensus (stage B1, B2, C/D). In all the cases, serum intestinal fatty acid-binding protein (I-FABP) and D/L-lactate concentrations, markers of intestinal mucosal injury, were measured. I-FABP was significantly higher in stage C/D Chihuahuas than in other groups (p < 0.05), and stage B2 was significantly higher than H (p < 0.05). D-lactate was significantly increased in stages B2 and C/D compared to H and stage B1 (p < 0.05). L-lactate was significantly higher in stage C/D Chihuahuas than in any other group (p < 0.05), and stage B2 was significantly higher than that in H and stage B1 (p < 0.05). Intestinal mucosal injury risk was significantly higher in Chihuahuas with heart failure due to MMVD, suggesting that the risk could increase with worsening heart disease. This is the first study to investigate the intestinal complications of MMVD, and further investigations a needed in the future.
The heart is a vital organ, and many associations between cardiovascular diseases and other organs, such as the liver (1) and kidney (2, 3), have been reported. Among these, the heart and intestine are recognized as being closely relatssed (1, 4, 5). In patients with heart failure, ischemia owing to hypoperfusion attributed to decreased cardiac output to the mesenteric arteries and intestinal mucosa (6), and intestinal edema owing to venous congestion (7) cause increased intestinal mucosal permeability. As a result, the lipotoxin (LPS) contained in the cell walls of gram-negative bacteria in the gut translocates into the blood (8), causing systemic inflammation and multi-organ damage (9–12). Myxomatous mitral valve disease (MMVD) is the most common cause of congestive heart failure in small dogs and is characterized by progressive atrioventricular valve degeneration (13). Prolonged heart failure can result in reduced blood flow in the body, causing ischemic damage to vital organs, such as the kidneys (14) and pancreas (15, 16). While the association between cardiac disease and the intestine has been widely reported in human medicine, it has not yet been studied and is still unknown in veterinary medicine.
Intestinal fatty acid-binding protein (I-FABP), which is a 15 kD protein responsible for the transport of long-chain fatty acids from the intestinal lumen (17, 18), is localized to the small intestinal mucosal epithelial cells. Since it is a relatively low-molecular weight, renally excreted, soluble cytoplasmic protein, it is rapidly released and translocated into the bloodstream when the small intestinal mucosal epithelial cells are injured (19, 20). I-FABP elevations have been observed in patients with intestinal diseases and systemic inflammatory response syndromes (21–25) and have been recognized as useful markers of intestinal mucosal injury (23, 24).
Lactate, which is an end product of anaerobic metabolism, is considered a biomarker for the diagnosis and prognosis of shock in humans and in veterinary medicine, and for detecting hypoperfusion (26). Lactate exists in the L- and D-body optical isomers. L- lactate is also produced by anaerobic metabolism associated with intestinal ischemia; however, it is not specific to the intestinal tract, since it increases in hypoxia related to all organs. In contrast, D-lactate is not produced in mammalian tissues but is produced by the methylglyoxal pathway and the enteric bacterial pathway; therefore, elevated D-lactate levels in the blood are considered to be of bacterial origin (26, 27); in humans, blood D-lactate is known to be elevated in patients with diabetes, infection, ulcerative colitis, intestinal surgery, and intestinal necrosis (28–30). D-lactate could be considered a specific marker for intestinal mucosal injury since it flows into the portal bloodstream when intestinal mucosal epithelial injury occurs owing to intestinal ischemia, and abnormal intestinal bacterial growth occurs (31). Hence, it is expected to be a potential intestinal mucosal injury marker (32–34).
Previous reports have used the lactulose-mannitol test and catheter- based right atrial pressure measurement to investigate intestinal permeability and venous congestion (7); however, reproducibility in dogs is difficult. In this study, we investigated the risk of intestinal mucosal injury using I-FABP and D/L-Lactate in healthy dogs and dogs with MMVD, limiting the study breed to clinically commonly encountered Chihuahuas. We hypothesized that intestinal mucosal injury could be a complication of MMVD in dogs and that the markers of intestinal mucosal injury would be significantly increased in dogs with MMVD.
This study was approved by the Rakuno Gakuen University, School of Veterinary Medicine Institutional Animal Care and Use Committee (approval No. VH19A10). All the cases were sampled between April 2019 and May 2020. Samples were collected at two institutions: Yokohama Yamate Dogs and Cats Medical Center and Tokyo Veterinary Cardiology Center. The sample was limited to Chihuahuas only, and included cases with MMVD or healthy dogs. Most of the healthy Chihuahuas visited the hospital for checkups and preventive medical care such as rabies vaccines. All Chihuahuas that met the inclusion criteria during the study period were included in the sample collection. The inclusion criteria were an adult dog, consent to the study, and absence of any urgent or fatal comorbidities. Patients with diseases secondary to MMVD (e.g., pulmonary hypertension or chronic kidney disease) were included; however, patients with other cardiac diseases (e.g., cardiac tumor or epicardial disease) were excluded. After obtaining the owner's consent, physical examination (weight and body condition score [BCS]), auscultation (presence and intensity of heart murmur), medical history, current medical history, medications, antibiotics, and the usual diet were recorded in all cases. In all the cases, chest radiography and echocardiography were performed to evaluate the heart. Based on the information obtained, healthy cases (H group) and cases with MMVD were classified into three groups according to the severity grade (stage B1, B2, and C/D) based on the ACVIM guidelines (35). Patients without cardiac disease were classified as healthy. We also performed systemic screening tests, including blood tests and radio/echography, and referred to past medical records to assess comorbidities. Serum samples were also collected to measure IFABP and L/D lactate concentrations by enzyme-linked immunosorbent assay (ELISA).
Echocardiography and chest radiography were performed in all dogs. Echocardiography was performed according to standard techniques (36), using Xario (TOSHIBA, probe: PST-50AT, 5 MHz) at the Yokohama Yamate Dogs and Cats Medical Center and Vivid e95 (GE Healthcare Japan, probe: 6S) at the Tokyo Veterinary Cardiology Center. Data were collected by two examiners; all cases were examined in a quiet room. Echocardiography included the subjective evaluation of the valve structure and function; mitral regurgitation (MR) jets on color Doppler examination in four-lumen cross-sectional images, left atrium to aorta ratio (LA/Ao) at diastole, left ventricular septal wall thickness at diastole (IVSd), left ventricular posterior wall thickness at diastole (LVPWd), left ventricular internal diameter at diastole (LVIDd), normalized left ventricular internal diameter (LVIDDN) (37), left ventricular internal diameters in systole (LVIDs), fractional shortening (FS), left ventricular ejection fraction (LVEF), and left ventricular inflow velocity waves (Evel, Avel) were evaluated and recorded.
Chest radiographs were taken at maximal right lateral inspiration for the presence of pulmonary edema and for recording the vertebral heart scale (VHS) and vertebral left atrial score (VLAS). The VHS and VLAS were measured using a previously described method (38, 39).
The disease severity was determined as cardiac enlargement (Stage B2) at LA/Ao>1.6 and LNIDDN>1.7 using ACVIM guidelines (35). Although the normal references of VHS and VLAS reportedly vary depending on the breed of dog, a previous report (40) reveals the standard for Chihuahuas; hence, we followed that report and used VHS > 10.0 v and VLAS > 1.8 v as the standard for cardiac enlargement. Patients with current or past pulmonary edema were defined as stage C; patients with refractory pulmonary edema were defined as stage D.
Blood samples were collected in all cases. Blood was collected from the jugular vein or the external saphenous vein. The blood sample was placed in a serum separator tube, meticulously inverted, and mixed. The clots were left at room temperature for 15–30 min until the coagulation was complete and then centrifuged at 1,100 g for 10–15 min following clot formation. The serum obtained by centrifugation was transferred to microtubes and stored at −80°C for I-FABP, L-lactate, and D-lactate measurement using ELISA.
I-FABP levels were measured using the canine intestinal fatty acid binding protein ELISA kit (Bioassay Technology Laboratory), as in the previous study (41). The assay was performed according to the manufacturer's recommendations. The serum was allowed to warm up naturally at room temperature, and the serum was then diluted. Serum samples and ELISA reagents were placed in each well and incubated at 37°C for 1 h. The plate was washed five times and then incubated at 37°C for 10 min with substrate solution added, followed by the addition of stop solution and color development, and absorbance measurement at 450 nm. For the controls, we measured what was included in the manufacturer's manual. Measurements were taken in duplicate, and the cut-off was 2 standard deviations. No samples were below the lower detection limit or above the upper detection limit.
D/L-lactate was measured using the EnzyChoromTM D-lactate assay kit (ECLC-100) (BioAssay Systems) and the EnzyChoromTM L-Lactate Assay Kit (EDLC-100) (BioAssay Systems). ECLC-100 and EDLC-100 were not used for antibody measurement. These kits were used for all animals. The assay was performed according to the manufacturer's recommendations. Serum was diluted 2- fold with dH2O before the assay, and the absorbance was measured at 565 nm.
Data were computed into a spreadsheet, and statistical analysis was performed using SPSS software (SPSS statistics ver 24.0 IBM Japan, Ltd., Tokyo, Japan). Histograms were made for continuous variables to assess normality and equivariance. There were no significant deviations as a parametric method for all variables, and the results were judged to be within a tolerable range. ELISA (I-FABP, D-lactate, L- Lactate) results were subjected to a one-way ANOVA using the Tukey– Kramer post-hoc test. Results are presented as mean ± standard deviation. Differences were considered significant at P < 0.05.
We collected a sample of 69 cases, ranging in age from 3 to 12 years, which were divided into four groups: H (n = 19), B1 (n = 21), B2 (n = 15), and C/D (n = 14) (Table 1). Of the 14 cases in Group C/D, three samples were collected on presentation to the hospital in a hypoxemic crisis of fulminant pulmonary edema. The remaining 11 cases were sampled while stable on medication at a scheduled recheck. No cases were excluded after sample collection. Group H had a predominantly lower mean age compared to the other groups; however, no age difference was observed in the other groups. One case each in groups B1 and C/D was on antimicrobial medication. They were administered erythromycin and enrofloxacin, respectively. The following comorbidities were observed in each group. Group H: canine atopic dermatitis (CAD) (n = 2), chronic kidney disease (CKD) (n = 1), epileptic seizures (n = 1), and food allergy (n = 1). Group B1: CAD (n = 1), cholelithiasis (n = 1), hypoadrenocorticism (n = 1), and hypothyroidism (n = 2). Group B2: CAD (n = 1), CKD (n = 2), diabetes mellitus (n = 1), tracheal collapse (n = 2), and hypothyroidism (n = 1). Group C/D: CKD (n = 7) and tracheal collapse (n = 2). Owing to worsening cardiac disease, many patients were taking cardiac medications, and all the patients in Group C/D were taking angiotensin-converting enzyme inhibitors (ACI) and pimobendan. The three patients in Group B2 on loop diuretics (furosemide: 1.1 ± 0.6 mg/kg/day) had no history of heart failure and, were being prescribed for cough reduction. Nine patients in Group C/D were receiving loop diuretics (torasemide: 0.33 ± 0.23 mg/kg/day).
Table 1. Patient characteristics of healthy Chihuahuas (H) and Chihuahuas with myxomatous mitral valve disease divided into three groups (B1, B2, and C/D).
Echocardiography, VHS, and VLAS findings are presented in Table 2. LVIDd was higher in groups B2 and C/D than in groups H and B1; however, there was no significant difference between groups B2 and C/D. The E wave also increased with severity, with group C/D having the highest increase. VHS and VLAS were also higher with worsening cardiac disease, with Group C/D having higher values than the other groups.
Table 2. Echocardiographic and radiographic data in healthy Chihuahuas (H) and Chihuahuas with myxomatous mitral valve disease divided into three groups (B1, B2, and C/D).
Serum I-FABP concentration in Chihuahuas with MMVD increased in proportion to MMVD severity (Figure 1). Although Group B1 (2.32 ± 0.43 ng/ml) was not significant difference compared to Group H (2.32 ± 0.43 ng/ml), Group B2 (3.93 ± 0.25 ng/ml) showed predominantly higher serum levels than group H. Group C/D (5.50 ± 0.57 ng/ml) showed predominantly higher serum concentrations than the other groups. Serum D-lactate and L-lactate concentrations are shown in Figures (Figures 2A,B). Considering D-lactate, group B2 (0.55 ± 0.08 mmol/L) had increased serum concentrations compared to groups H (0.36 ± 0.06 mmol/L) and B1 (0.37 ± 0.04 mmol/L). Group C/D (0.82 ± 0.09 mmol/L) showed an overall increase in serum concentration compared to the other groups. The concentrations of serum L-lactate (H: 0.2 ± 0.08 mmol/L; B1: 0.37 ± 0.07 mmol/L; B2: 0.57 ± 0.06 mmol/L; 0.92 ± 0.07 mmol/L) were shown as the same phenomenon as the results of D-lactate.
Figure 1. Serum concentrations of intestinal fatty-acid binding protein in healthy Chihuahuas (H) and Chihuahuas with myxomatous mitral valve disease divided into three groups (B1, B2, and C/D). One-way ANOVA with post hoc Turkey-Kramer test. Significant difference was considered P < 0.05.
Figure 2. Serum concentrations of D-Lactate (A) and L-Lactate (B) in healthy Chihuahuas (H) and Chihuahuas with myxomatous mitral valve disease divided into three groups (B1, B2, and C/D). One-way ANOVA with post hoc Turkey-Kramer test. Significant difference was considered P < 0.05.
The main objective of our study was to clarify the relationship between MMVD and intestinal mucosal injury using intestinal mucosal injury markers such as I-FABP and D-lactate.
We found that serum I-FABP, D-lactate, and L-lactate levels were increased in Chihuahuas with heart failure attributed to MMVD, and there was a certain correlation between worsening heart disease and increased I-FABP, D-lactate, and L-lactate levels. I-FABP was significantly higher in group B2 than in group H, and in group C/D more than in any other group. D-lactate was significantly increased in the groups with cardiac enlargement (B2, C/D) than in groups without cardiac enlargement (H, B1). L-lactate was significantly higher in group B2 than in groups H and B1, and the C/D group showed an increase over the other groups. These results suggest that the risk of intestinal mucosal injury could increase with worsening heart disease, and dogs with heart failure (Group C/D) had a significantly higher risk of intestinal mucosal injury than dogs without heart failure (Group H, B1, B2).
Kitai et al. (42) reported that the cut-off value of I-FABP in human patients with heart failure was 853 pg/ml, suggesting that a case group with >853 pg/ml could have a poor prognosis with a short survival time. In the present study, I-FABP was significantly increased in Group C/D to 5.5 ( ± 0.6) ng/ml, and the difference in value was the difference between humans and dogs. In the future, if I-FABP is studied in dogs and a cut-off value can be established, it could become a new prognostic factor for heart failure in dogs. L-Lactate was significantly increased in heart failure, which was consistent with previous reports (43). There are no reports on the association between D- lactate levels and heart failure. However, the intestinal-specific increase in D-lactate suggests intestinal mucosal injury, as reported by Montagna et al. (33). The significant increase in D-lactate levels in dogs with heart failure in this study suggests that dogs with heart failure are at risk for intestinal mucosal injury. A study reported by Venn et al. (31) demonstrated that D-lactate was significantly elevated in dogs with parvoviral enteritis; therefore, D-lactate possesses the potential for use as a biomarker in dogs. However, Memet et al. (32) reported that D/L-Lactate is often increased at the end of severe intestinal injury and is considered inferior to I-FABP as a marker of intestinal mucosal injury. In this study, D/L-Lactate was similar to I-FABP; however, further studies are warranted to determine its usefulness in dogs.
LA/Ao > 1.7 and E vel > 1.2 m/s are reportedly poor prognostic factors in MMVD (44); in the present study, the values of LA/Ao and E vel were significantly higher in the C/D group than in the other groups, consistent with the reports of Borgarelli et al. I-FABP and D-lactate levels increased in proportion to MMVD severity, suggesting a possible correlation between intestinal mucosal injury and important echocardiographic parameters that indicate the prognosis of MMVD, such as LA/Ao and E vel. The more severe the MMVD, the greater the increase and decrease in FS and LVEF, respectively. Although no significant difference was found in this study, both FS and LVEF increased with worsening MMVD, which may be due to the influence of the many cases in Group B2 and all the cases in C/D who were taking pimobendan. Kanno et al. suggested that pimobendan administration increased FS and LVEF (45). It is difficult to relate cardiac contractile function to intestinal mucosal injury in this study. Kitai et al. (42) reported no association between the increased I-FABP levels and echocardiographic parameters. This is because, in this study, the collection of patients (healthy dogs to dogs with severe heart failure) was blinded, and subsequently classified and measured for severity. However, Kitai et al. (42) limited their study to patients with heart failure. In addition, the heart failure in humans studied by Kitai et al. (42) is due to cardiomyopathy, which differs in pathology from the heart failure due to MMVD in dogs in this study. Therefore, these results were inconsistent with our study owing to differences in the original population and pathology.
Performing VHS and VLAS is easier compared to echocardiography; VLAS, in particular, significantly correlates with LA/Ao measured by echocardiography (39). The more severe the MMVD, the greater the increase in VHS and VLAS; dogs with heart failure showed a significant increase compared to the other groups, suggesting a correlation between I-FABP and D/L-Lactate, which increased with MMVD severity. A correlation between VHS, VLAS, and intestinal mucosal injury markers, proven by further research, is desirable since it would result in a simpler test for determining the risk intestinal mucosal injury. However, the accuracy of VHS and VLAS is inferior to that of echocardiography since it depends on many factors, such as canine body position, radiographic technique, and the presence or absence of concomitant chest abnormalities. Therefore, the practicality of VHS and VLAS as a test to determine the risk of intestinal mucosal injury is uncertain.
Our study had certain limitations. First, there was a lack of uniformity in the diet of the patients. The effects of diet on the intestine and health in dogs have been reported (46, 47), and differences in diet could have affected the results of our study. Second, previous reports have used pulmonary artery catheterization to measure right atrial pressure to investigate venous stasis (42) and the lactulose-mannitol test to test intestinal permeability (7). Although it is a highly credible test, it is not an easy test to perform in veterinary medicine and does not increase the credibility of the results of this study. Third, the presence or absence of concomitant diseases may have affected the results. Group C/D had more cases using diuretics to treat cardiac disease and also showed a mild to severe increase in the renal values. The impact of an increased renal panel on the results of this study should be considered. Other gut bacteria are deeply involved in the relationship between the heart and the gut (48); however, in this study, we did not assess gut bacteria, blood LPS levels, or intestinal mucosa. Further studies are warranted to clarify the relationship between heart disease and intestines in dogs.
In human medicine, many reports exist on the effects of cardiac disease on the gut; however, no studies have been reported in dogs. This study showed that intestinal mucosal injury markers such as I-FABP and D-Lactate increased with the severity of MMVD in Chihuahuas and that Chihuahuas with MMVD who had heart failure were at particular risk for intestinal mucosal injury. This study is the first to investigate the relationship between heart disease and intestinal mucosa in Chihuahuas and demonstrate a potential relationship between MMVD morbidity and intestinal mucosal cell injury.
The raw data supporting the conclusions of this article will be made available by the authors, without undue reservation.
The animal study was reviewed and approved by the Rakuno Gakuen University, School of Veterinary Medicine Institutional Animal Care and Use Committee (Approval No. VH19A10). Written informed consent was obtained from the owners for the participation of their animals in this study.
Conceptualization, methodology, formal analysis, writing review, editing, visualization, and supervision: MI. Software, validation, data curation, and writing-original draft preparation: RA. Investigation: RA and KI. Project administration: KU and MI. All authors have read and agreed to the published version of the manuscript.
The authors declare that the research was conducted in the absence of any commercial or financial relationships that could be construed as a potential conflict of interest.
All claims expressed in this article are solely those of the authors and do not necessarily represent those of their affiliated organizations, or those of the publisher, the editors and the reviewers. Any product that may be evaluated in this article, or claim that may be made by its manufacturer, is not guaranteed or endorsed by the publisher.
We would like to thank Editage (www.editage.com) for English language editing.
1. Sandaram V, Fang JC. Gastrointestinal and liver issue in Heart Failure. Circulation. (2016) 133:1696–703. doi: 10.1161/CIRCULATIONAHA.115.020894
2. Bongartz LG, Cramer MJ, Doevendas PA, Joles JA, Braam B. The severe cardiorenal. syndrome: ‘Guyton revisited'. Eur Heart J. (2005) 26:11–7. doi: 10.1093/eurheartj/ehi020
3. Ronco C, Haapio M, House AA, Anavekar N, Bellomo R. 2008. Cardiorenal syndrome. J Am Coll Cardiol. (2008) 52:1527–39. doi: 10.1016/j.jacc.2008.07.051
4. Kamo T, Akazawa H, Suzuki J, Komuro I. Novel concept of a Heart-Gut Axis in the. Pathophysiology of Heart Failture. Korean Circ J. (2017) 47:663–9. doi: 10.4070/kcj.2017.0028
5. Rogler G, Rosano G. The heart and the gut. Eur Heart J. (2014) 35:426–30. doi: 10.1093/eurheartj/eht271
6. Sandek A, Swindsinski A, Schroedl W, Watson A, Valentova M., Herrmann R, et al. Intestinal Blood Flow in Patients With Chronic Heart Failure A Link with bacterial Growth, Gastrointestinal Symptoms, and Cachexia. J Am Coll Cardiol. (2014) 64:1092–102. doi: 10.1016/j.jacc.2014.06.1179
7. Sandek A, Bauditz J, Swidsinski A, Buhner S, Weber-Eibel J., von Haehling S, et al. Altered intestinal function in patients with chronic heart failure. J Am Coll Cardiol. (2007) 50:1561–9. doi: 10.1016/j.jacc.2007.07.016
8. Sandek A, Bjarnason I, Volk HD, Crane R, Meddings JB, Niebauer J, et al. Studies on bacterial endotoxin and intestinal ansorption function in patients with chronic heart failture. Int J Cardiol. (2012) 157:80–5. doi: 10.1016/j.ijcard.2010.12.016
9. Cirera I, Bauer TM, Navasa M, Vila J, Grande L, Taurá P, et al. Bacterial translocation of enteric organisms in patients with cirrhosis J Hepatol. (2001) 34:32–7. doi: 10.1016/S0168-8278(00)00013-1
10. Deitch EA. Bacterial translocation or lymphatic drainage of toxic products from the gut: what is important in human beings? Surgery. (2002) 131:241–4. doi: 10.1067/msy.2002.116408
11. Doig CJ, Sutherland LR, Sandham JD, Fick GH, Verhoef M, Meddings JB. Increased intestinal permeability is associated with the development of multiple organ dysfunction syndrome in critically ill ICU patients American. Am J Respir Crit Care Med. (1998) 158:444–51. doi: 10.1164/ajrccm.158.2.9710092
12. Riddington DW, Venkatesh B, Boivin CM, Bonser RS, Elliott TS, Marshall T, et al. Intestinal perme- ability, gastric intramucosal pH, and systemic endotoxemia in patients undergoing cardiopulmonary bypass. JAMA. (1996) 275:1007–12. doi: 10.1001/jama.1996.03530370045029
13. Atkins C, Bonagura J, Ettinger S, Fox P, Gordon S, Haggstrom J, et al. Guidelines for the diagnosis and treatment of caninechronic valvular heart disease. J Vet Intern Med. (2009) 23:1142–50. doi: 10.1111/j.1939-1676.2009.0392.x
14. Pouchelon JL, Atkins CE, Bussadori C, Oyama MA, Vaden SL, Bonagura JD, et al. Cardiovascular-renal axis disorders in the domestic dog and cat: a veterinary consensus statement. J Small Anim Pract. (2015) 56:537–52. doi: 10.1111/jsap.12387
15. Han D, Choi R, Hyun C. Canine pancreatic-specific lipase concentrations in dogs with heart failure and chronic mitral valvular insufficiency. J Vet Intern Med. (2015) 29:180–3. doi: 10.1111/jvim.12521
16. Borgarelli M, Haggstrom J. Canine degenerative myxomatous mitral valve. disease:natural history, clinical presentation and therapy. Vet Clin North Am Small Anim Pract. (2010) 40:651–63. doi: 10.1016/j.cvsm.2010.03.008
17. Ockner RK, Manning JA. Fatty acid-binding protein in small intestine. Identification, isolation, and evidence for its role in cellular fatty acid transport. J Clin Invest. (1974) 54:326–38. doi: 10.1172/JCI107768
18. Veerkamp JH, Peeters RA., Maatman RG. Structural and functional features of different types of cytoplasmic fatty acid- binding proteins. Biochim Biophys Acta. (1991) 1081:1–24. doi: 10.1016/0005-2760(91)90244-C
19. Lieberman JM, Sacchettini J, Marks C, Marks WH. Human intestinal fatty acid binding protein: Report of an assay with studies in normal volunteers and intestinal ischemi. Surgery. (1997) 121:335–42. doi: 10.1016/S0039-6060(97)90363-9
20. Robinson JW, Mirkovitch V. The recovery of function and microcirculation in small intestinal loops following ischaemia. Gut. (1972) 13:784–89. doi: 10.1136/gut.13.10.784
21. Derikx JP, Poeze M, Bijnen AA, Buurman WA, Heineman E. Evidence for intestinal and liver epithelial cell injury in the early phase of sepsis. Shock. (2007) 28:544–48. doi: 10.1097/shk.0b013e3180644e32
22. Derikx JP, Waardenburg DA, Thuijls G, Willigers HM, Koenraads M, Bijnen AA, et al. New insight in loss of gut barrier during major non- abdominal surgery. PLoS ONE. (2008) 3:3954. doi: 10.1371/journal.pone.0003954
23. Guthmann F, Borchers T, Wolfrum C, Wustrack T, Bartholomaus S., Spener F, et al. Plasma concentration of intestinal- and liver-fabp in neonates suffering from necrotizing enterocolitis and in healthy preterm neonates. Mol Cell Biochem. (2002) 239:227–34. doi: 10.1023/A:1020508420058
24. Pelsers MM, Namiot Z, Kisielewski W, Namiot A, Januszkiewicz M, Hermens WT, et al. Intestinal-type and liver-type fatty acid-binding protein in the intestine. Tissue distribution and clinical utility Clin Biochem. (2003) 36:529–35. doi: 10.1016/S0009-9120(03)00096-1
25. Relja B, Szermutzky M, Henrich D, Maier M, Haan JJ, Lubbers T, et al. Intestinal-fabp and liver-fabp: Novel markers for severe abdominal injury. Acad Emerg Med. (2010) 17:729–35. doi: 10.1111/j.1553-2712.2010.00792.x
26. Allen SE, Holm JL. Lactate: physiology and clinical utility. J Vet Emerg Crit Care. (2008) 18:123–32. doi: 10.1111/j.1476-4431.2008.00286.x
28. Hasegawa H, Fukushima T, Lee JA, Tsukamoto K, Moriya K, Ono Y, et al. Determination of serum D-lactic and L-lactic acids in normal subjects and diabetic patients by column-switching HPLC with pre-column fluorescence derivatization. Anal Bioanal Chem. (2003) 377:886–91. doi: 10.1007/s00216-003-2108-6
29. Scheijen JL, Hanssen NM, Waarenburg MP, Jonkers DM, Stehouwer CD, Schalkwijk CG. L(+) and D(-) lactate are increased in plasma and urine samples of type 2 diabetes as measured by a simultaneous quantification of L(+) and D(-) lactate by reversed- phase liquid chromatography tandem mass spectrometry. Exp Diabetes Res. (2012) 2012:234812. doi: 10.1155/2012/234812
30. Talasniemi JP, Pennanen S, Savolainen H, Niskanen L, Liesivuori J. Analytical investigation: assay of D-lactate in diabetic plasma and urine. Clin Biochem. (2008) 41:1099–103. doi: 10.1016/j.clinbiochem.2008.06.011
31. Venn EC, Barnes AJ, Hansen RJ, Boscan PL, Twedt DC, Sullivan LA. Serum D-lactate concentrations in dogs with parvoviral enteritis. J Vet Intern Med. (2020) 34:691–9. doi: 10.1111/jvim.15688
32. Memet O, Zhang L, Shen J. Serological biomarkers for acute mesenteric ischemia. Ann Transl Med. (2019) 16:394. doi: 10.21037/atm.2019.07.51
33. Montagnana M, Danese E, Lippi G. Biochemical markers of acute intestinal ischemia: possibilities and limitations. Ann Transl Med. (2018) 6:341. doi: 10.21037/atm.2018.07.22
34. Nielsen C, Lindholt JS, Erlandsen EJ, Mortensen FV. D-lactate as a marker of induced intestinal ischemia: an experimental study in pigs. Int J Surg. (2011) 9:428–32. doi: 10.1016/j.ijsu.2011.04.004
35. Keene BW, Atkins CE, Bonagura JD, Fox PR, Haggstrom J, Fuentes VL., et al. ACVIM consensus guidelines for the diagnosis and treatment of myxomatous mitral valve disease in dogs. J Vet Intern Med. (2019) 33:1127–40. doi: 10.1111/jvim.15488
36. Thomas WP, Gaber CE, Jacobs GJ, Kaplan PM, Lombard CW, Moise NS, et al. Recommendations for standards in transthoracic two-dimensional echocardiography in the dog and cat. J Vet Intern Med. (1993) 7:247–52. doi: 10.1111/j.1939-1676.1993.tb01015.x
37. Cornell CC, Kittleson MD, Della TP, Haggstrom J, Lombard CW, Pedersen HD, et al. Allometric scaling of M-mode cardiac measurements in normal adult dogs. J Vet Intern Med. (2004) 18:311–21 doi: 10.1111/j.1939-1676.2004.tb02551.x
38. Bunchanan JW, Bucheler J. Vertebral scale system to measure canine heart size in radiographs. J Vet Intern Med. (1995) 206:194–9.
39. Malcom EL, Visser LC, Phillips KL, Johonson LR. 2018. Diagnostic value of vertebral left atrial size as determined from thoracic radiographs for assessment of left atrial size in dogs with myxomatous mitral valve disease. J Vet Intern Med. (2018) 253:1038–45. doi: 10.2460/javma.253.8.1038
40. Puccineili C, Citi S, Vezzosi T, Garibaldi S, Tognetti R. A radiographic study of breed-specific vertebral heart score and vertebral left atrial size in chihuahuas. Vet Radiol Ultrasound. (2021) 62:20–6. doi: 10.1111/vru.12919
41. Gulersoy E, Ok M, Yildiz R, Koral E, Ider M, Sevinc M, et al. Assessment of intestinal and cardiac-related biomarkers in dogs with parvoviral enteritis. Pol J Vet Sci. (2020) 23:211–19.
42. Kitai T, Kim YH, Kiefer K, Morales R, Borowski AG, Grodin JL, et al. Circulating intestinal fatty acid-binding protein(I-FABP) levels in acute decompensated heart failure. Clin Biochem. (2017) 50:491–5. doi: 10.1016/j.clinbiochem.2017.02.014
43. Bosso G, Mercurio V, Diab N, Pagano A, Porta G, Allegorico E, et al. Time- weighted lactate as a predictor of adverse outcome in acute heart failure. ESC Heart Fail. (2021) 8:539–45. doi: 10.1002/ehf2.13112
44. Borgarelli M, Savarino P, Crosara S, Santilli RA, Chiavegato D, Poggi M, et al. Survival characteristics and prognostic variables of dogs with mitral regurgitation attributable to myxomatous valve disease. J Vet Intern Med. (2018) 22:120–8. doi: 10.1111/j.1939-1676.2007.0008.x
45. Kanno N, Kuse H, Kawasaki M, Hara A, Kano R, Sasaki Y. Effects of pimobendane for mitral valve regurgitation in dogs. J Vet Intern Med. (2007) 69:373–7. doi: 10.1292/jvms.69.373
46. Kim J, An J, Kim W, Lee S, Cho S. Differences in the gut microbiota of dogs (Canis lupus familiaris) fed a natural diet or a commercial feed revealed by the Illimina Miseq platform. Gut Pathog. (2017) 2017:9–68. doi: 10.1186/s13099-017-0218-5
47. Zebtek J, Marquart B, Pietrzak T, Ballevre O, Rochat F. Dietary effects on bifidobacterial and Clostridium perfringens in the canine intestinal tract. J Anim Physiol Anim Nutr. (2003) 87:397–407. doi: 10.1046/j.0931-2439.2003.00451.x
Keywords: Chihuahuas, D-lactate, intestinal complication, intestinal fatty acid-binding protein, intestinal mucosal injury markers, myxomatous mitral valve disease
Citation: Araki R, Iwanaga K, Ueda K and Isaka M (2021) Intestinal Complication With Myxomatous Mitral Valve Diseases in Chihuahuas. Front. Vet. Sci. 8:777579. doi: 10.3389/fvets.2021.777579
Received: 15 September 2021; Accepted: 28 October 2021;
Published: 23 November 2021.
Edited by:
Ryou Tanaka, Tokyo University of Agriculture and Technology, JapanReviewed by:
Corrin John Boyd, Murdoch University, AustraliaCopyright © 2021 Araki, Iwanaga, Ueda and Isaka. This is an open-access article distributed under the terms of the Creative Commons Attribution License (CC BY). The use, distribution or reproduction in other forums is permitted, provided the original author(s) and the copyright owner(s) are credited and that the original publication in this journal is cited, in accordance with accepted academic practice. No use, distribution or reproduction is permitted which does not comply with these terms.
*Correspondence: M. Isaka, bS1pc2FrYUByYWt1bm8uYWMuanA=
Disclaimer: All claims expressed in this article are solely those of the authors and do not necessarily represent those of their affiliated organizations, or those of the publisher, the editors and the reviewers. Any product that may be evaluated in this article or claim that may be made by its manufacturer is not guaranteed or endorsed by the publisher.
Research integrity at Frontiers
Learn more about the work of our research integrity team to safeguard the quality of each article we publish.