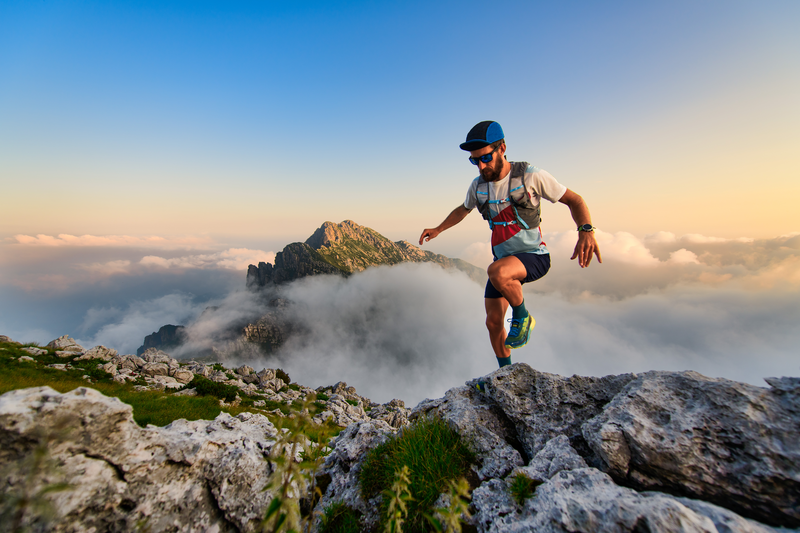
95% of researchers rate our articles as excellent or good
Learn more about the work of our research integrity team to safeguard the quality of each article we publish.
Find out more
MINI REVIEW article
Front. Vet. Sci. , 02 November 2021
Sec. Veterinary clinical, anatomical, and comparative pathology
Volume 8 - 2021 | https://doi.org/10.3389/fvets.2021.758720
This article is part of the Research Topic Biology and Pathology of Tumor Viruses in Animals View all 5 articles
A correction has been applied to this article in:
Corrigendum: Pteridium spp. and Bovine Papillomavirus: Partners in Cancer
Bovine papillomavirus (BPV) are a cause for global concern due to their wide distribution and the wide range of benign and malignant diseases they are able to induce. Those lesions include cutaneous and upper digestive papillomas, multiple histological types of urinary bladder cancers—most often associated with BPV1 and BPV2—and squamous cell carcinomas of the upper digestive system, associated with BPV4. Clinical, epidemiological and experimental evidence shows that exposure to bracken fern (Pteridium spp.) and other related ferns plays an important role in allowing viral persistence and promoting the malignant transformation of early viral lesions. This carcinogenic potential has been attributed to bracken illudane glycoside compounds with immune suppressive and mutagenic properties, such as ptaquiloside. This review addresses the role of BPV in tumorigenesis and its interactions with bracken illudane glycosides. Current data indicates that inactivation of cytotoxic T lymphocytes and natural killer cells by bracken fern illudanes plays a significant role in allowing viral persistence and lesion progression, while BPV drives unchecked cell proliferation and allows the accumulation of genetic damage caused by chemical mutagens. Despite limited progress in controlling bracken infestation in pasturelands, bracken toxins remain a threat to animal health. The number of recognized BPV types has steadily increased over the years and now reaches 24 genotypes with different pathogenic properties. It remains essential to widen the available knowledge concerning BPV and its synergistic interactions with bracken chemical carcinogens, in order to achieve satisfactory control of the livestock losses they induce worldwide.
Papillomaviruses (PVs) are small epitheliotropic viruses that contain circular double-stranded DNA with about 7,000 base pairs (bp) as genetic material and belong to the Papillomaviridae family (1, 2). PVs infect mammals, reptiles, birds and fish and can be found on healthy skin and mucous membranes, as well as in benign proliferative epithelial lesions and in invasive cancers (3–6). Structurally, PVs consist of a capsid with icosahedral symmetry composed of 72 capsomeres in forming star-shaped pentamers and measuring 55–66 nm. The viral forms a super helix with histoproteins derived from infected host cells (1, 2). The genome structure of all PVs has significant similarities. The circular genome is divided into three regions: one region containing genes that encode the initial viral proteins involved in viral replication and modulation of cellular functions called the early (“E”) region, the late (“L”) region which encodes the L1 structural protein (the main capsid protein) and the L2 minor capsid protein, and the long control region (LCR) region (1, 2, 5, 7). Difficulties in classifying PVs have been caused by their high genotypic diversity that goes far beyond those found in other virus families, and the high number of PV hosts. Recent phylogenetic studies of PVs demonstrate a variety of evolutionary processes that underlie this diversity (2, 8, 9). Currently, within the Papillomaviridae family, 53 genera are identified according to the International Committee on Taxonomy of Viruses (ICTV) (5). The inclusion of different species within each genus is done based on phylogenetic analysis, biological properties and similarity of L1 gene nucleotide sequences, due to their conserved pattern within each taxonomic genus (4, 10). Thus, PVs that share at least 60% similarities in the L1 gene are grouped into the same genus. A relationship has long been recognized between exposure to bovine papillomavirus (BPV) and certain toxic ferns—most commonly Pteridium spp.—leading to the development of malignant neoplasms, as previously reviewed (11, 12). The complex interplay between viral and chemical factors in the development of cancer in cattle is the subject of the present review.
Pteridium spp. known as bracken fern or common fern (Figure 1A) is a pteridophyte belonging to the Dennstaedtiaceae family, and comprises multiple species and strains (13, 14). Bracken fern is distributed all over the world, including bush areas, forest undergrowth and cultivated land, but it preferentially develops in acidic and deep soils and in humid and shaded areas (15, 16). Bracken fern has a negative effect at the economic level since it directly impacts animal production. Such impacts can be classified in two ways: direct losses when there is death, reproductive disorders, weight loss or reduced growth and indirect losses which involve medical costs, habitat changes and alterations in the handling of animals (17). Pteridium spp. contains many bioactive substances such as quercetin (18), shikimic acid (19), tannins (20) and a group of toxic illudane glycosides among which ptaquiloside (21–23) has been best characterized, as previously reviewed by our group (11). Ptaquiloside was first identified in 1983 (21–23) as a water-soluble, unstable glycoside, which contains a reactive cyclopropane ring. Ptaquiloside reacts with water to form highly reactive intermediates which alkylate biomolecules such as DNA, triggering pathological cellular changes, as recently reviewed (24). Other related ferns such as Cheilanthes sieberi have been studied chemically and were also found to contain illudane glycosides (25). The concentrations of ptaquiloside and other illudane glycosides in bracken fern show wide seasonal variations and also depend on bracken strains, geographical location and the parts of the pant being analyzed (e.g., rhizomes vs. fronds), reaching a maximum of 18.81 mg/g of dry plant (26). Young sprouts, known as croziers, are the parts that contain more ptaquiloside (26–28). Illudane glycosides are responsible for the immunotoxic, mutagenic and carcinogenic properties of bracken fern (24). Other compounds like quercetin, initially thought to be involved in bracken fern-induced cancers (29, 30), were later shown to be safe in vivo (31, 32). The toxic effects of bracken fern vary according to the animal species, the dose ingested and the time of consumption (17). In grazing animals, bracken ingestion is associated with multiple syndromes. These include acute bracken poisoning and others that develop upon prolonged exposure, like thiamine deficiency in horses, progressive retinal degeneration of sheep, bovine enzootic hematuria and upper alimentary tract carcinomas, as previously reviewed (11). Early works from the 17th century already mention bracken's acute gastrointestinal toxicity when ingested (33). In ruminants, acute bracken poisoning can cause necrosis of the laryngeal, pharyngeal and intestinal mucosae, as well as severe leukopenia with neutropenia or lymphopenia, anemia and thrombocytopenia (34, 35). These effects have been experimentally reproduced in laboratory rats fed bracken and in cattle administered ptaquiloside (36, 37). Another fern, Adiantopsis chlorophylla, containing caudatoside, an illudane glycoside structurally related with ptaquiloside, has also been associated with acute poisoning in cattle (38). Thiamine deficiency affects monogastric animals such as horses and, to a lesser extent, pigs that receive bracken as part of their diet. Thiamine deficiency is caused by bracken fern thiaminases and is characterized by central nervous system lesions and related symptoms (39). Progressive retinal degeneration is typically observed in sheep that graze on bracken fern and was reproduced in laboratory conditions by administering bracken or ptaquiloside (40, 41). Bovine enzootic hematuria and upper alimentary tract carcinomas are of particular interest for the purposes of this review, because their pathogenesis involves complex interactions between bracken fern toxins and BPV, revealing their partnership as chemical and biological carcinogens. These two particular syndromes will be dealt with in the following sections.
Figure 1. It is hypothesized that Pteridium spp. and BPV synergize to drive carcinogenesis in cattle. (A) Mature Pteridium sp. fronds, Maranhão, Brazil. (B) Representative macroscopic view of a bovine urinary bladder hemangiossarcoma. (C) Illudane glycosides like ptaquiloside promote immune evasion by inactivating critical leukocytes like cytotoxic T lymphocytes and NK cells, allowing viral persistence. Genetic damage caused by bracken toxins accumulates due to unchecked cell proliferation and survival induced by BPV E5, E6 and E7 oncoproteins, leading to cancer.
BPV infections have been observed in cattle in multiple locations worldwide, often with significant effects on livestock production, as well as on the local economy, as previously reviewed by our group and others (2, 42). Among the main genes of PVs, the E2 gene has important function in neoplasms associated with PV in both animals and humans, because the interruption of E2 results in the uncontrolled expression of E6 and E7, which promotes cell transformation (2). The presence of the E1, E2, E3, E4, E5, E6, E7, and E8 genes varies depending on genera. The E1 protein is considered the primary viral replication protein, while E2 acts as a transactivator or enhancer (43). The E3 protein has no known function yet (44). The E4 protein is responsible for viral maturation and is associated with the disruption of the cytoskeleton structure (45). The E5 protein is an important BPV transforming protein and promotes the proliferation of infected cells via activation of the platelet-derived growth factor receptor beta (PDGFRβ) (46) while also promoting immune evasion by down-regulating the expression of major histocompatibility complex type I proteins in the cell surface (47). BPV E5 also interferes with cellular homeostasis by hampering the functions of cytosolic organelles, e.g., by interfering with the acidification of the Golgi complex (48). The E5 protein also forms tri-component complexes with PDGFRβ and the V1-ATPase D subunit, which are hypothesized to interfere with cellular proteostasis and autophagy (49). Furthermore, the BPV E5 protein constitutively activates c-Src (50) and activates phosphatidylinositol-3-kinase (PI3K) signaling independently of PDGFRβ (51). The E6 protein promotes the disruption of the actin cytoskeleton increasing cell motility, induces DNA breaks and neosis, p53 downregulation allowing uncontrolled proliferation in the presence of genomic instability, and contributed for metabolic deregulation, resulting in oxidative stress (52). The E7 protein promotes cell transformation by downregulating the retinoblastoma protein (pRb), deregulating the cell cycle and inducing mitosis (53). The E8 gene encodes a small hydrophobic polypeptide that contributes to cell transformation by providing anchorage-independent growth (54). The first PV genotype identified in domestic cattle (Bos taurus) (BPV-1) was described in the scientific literature in 1982 (55). Since then, research on this field brought to light other BPV genotypes and their number currently stands at 29. These genotypes are divided into five different genera: Delta (δ), Dyokappa, Yoxi, Epsilon (ε) and Xi (ξ) (56) as detailed in Table 1. There are 15 BPV genotypes classified as Xipapillomavirus that have cutaneous association (6, 57). BPV-5 and BPV-8 are classified as Epsilonpapillomavirus and are also detected in cutaneous papillomas and fibropapilomas (58). Skin papillomas induced by BPV are histologically benign and generally undergo spontaneous regression. BPV classified as Deltapapillomavirus have a unique characteristic which is their ability to cross-infect other species, including different species of animals within the Bovidae, Cervidae, Equidae, Felidae and Girafiidae families (59–66). Viruses of the genus Deltapapillomavirus are capable of causing sarcoids in distantly related hosts, such as horses, mules, African lions (67), domestic cats (65), mountain zebras, giraffes and black antelopes (68, 69). These sarcoids are locally invasive spindle-cell skin tumors, histologically characterized by epidermal hyperplasia and subepidermal proliferation of cells that are similar to fibroblasts (66). BPV-16, BPV-18 and BPV-22 are classified as Dyokappapapillomavirus and are detected in cutaneous papillomas and vulvovaginitis (57, 70, 71). BPV-7 also belongs to Dyokappapapillomavirus and has been identified in cutaneous papilloma and healthy skin. BPV-19, BPV-21 and BPV-27 currently remain unclassified (6). BPV-4 is associated with papillomas and squamous cell carcinomas in the upper digestive tract, including the oral and pharyngeal cavities, the esophagus and the rumen (72). BPV-1 and BPV-2 are associated with epithelial and mesenchymal urinary bladder tumors (Figure 1B) in cattle (73–75) and buffaloes (73).
Infection with BPV-4 has been associated with papillomas and squamous cell carcinomas of the upper digestive tract in cattle (95–98). Infection with BPV-4 is associated with oral, esophageal and ruminal papillomatosis and there have been reports of a papilloma-to-carcinoma transition (98). However, no BPV4 DNA or viral oncoproteins could be detected in malignant lesions (99, 100), raising questions about the etiological role of BPV-4 in upper digestive cancer. Exposure to bracken fern may promote the papilloma-to-carcinoma transition as previously suggested (46, 72, 98), with bracken fern toxins cooperating with BPV-4 to achieve a malignant phenotype (Figure 1C), but may also cause upper digestive cancer by itself, without BPV-4 involvement (99). Interestingly, recent findings show that ptaquiloside promotes carcinogenesis initiated by human papillomavirus type 16 (HPV-16) in the oral and pharyngeal cavities of HPV-16-transgenic mice (101). Bracken fern illudane glycosides like ptaquiloside have been proposed to contribute for carcinogenesis in two main ways. The first involves their genotoxic effects, as these compounds are able to alkylate DNA and induce point mutations, namely activating mutations at the Harvey rat sarcoma (H-ras) proto-oncogene codons 58, 58 and 61 (102, 103), which lead to increased cell proliferation. Ptaquiloside also induces chromosome aberrations (104, 105) that ultimately lead to a neoplastic phenotype, as previously reviewed (24). The second way involves their immune suppressive properties, which may facilitate the persistence of oncogenic viruses like BPV and HPV and may also allow early neoplastic lesions to evade immunological surveillance and develop into overt cancers (27). Latorre and collaborators showed that ptaquiloside is able to inactivate natural killer (NK) cells (106), which are key players in innate immunity and are essential for defending the host against viral infections and against cancer (107). When activated, NK cells are able to recognize and destroy neoplastic cells and virus-infected cells. So, by inactivating NK cells, ptaquiloside may facilitate the persistence of BPV infections and the development of early BPV-induced lesions into invasive cancers. More recently, our group showed that ptaquiloside is also able to inactivate cytotoxic T lymphocytes, another cell population involved in adaptive immunity against neoplastic and virus-infected cells (108). Interestingly, the immunotoxic effects of ptaquiloside against NK cells in mice could be reversed with selenium administration (109), suggesting that prevention of bracken fern toxicities can be achievable through dietary modifications. Very recently, the E5 oncoprotein of Delta BPVs was shown to impair the production of type I interferon and the innate antiviral response via interactions with the tripartite motif-containing 25 (TRIM25) protein (110), further establishing the immune evasion mechanisms employed by BPV.
Ingestion of bracken fern has been associated with enzootic hematuria of grazing cattle herds since the 1960s (111, 112). Since then, the occurrence of bladder tumors in cattle grazing on bracken fern has been described by different teams in multiple continents (72, 96, 113–116). The morphology of bovine urinary bladder tumors is varied, includes multiple histological types of epithelial, mesenchymal and mixed lesions, and has been previously described in detail (114–116). The experimental administration of bracken fern to cows reproduced the urinary lesions in controlled conditions (117). In a seminal work that largely sparked research in bracken fern-related carcinogenesis, Evans and Mason (19) showed that bladder neoplasia can also be induced in rats by a diet containing bracken (19). These findings were later reproduced by other teams (17, 118, 119) and also by researchers working with guinea pigs (120). Mutations of the Harvey rat sarcoma virus (H-ras) oncogene were found in rats exposed to ptaquiloside (102, 103), but do not seem to be frequent in bovine bladder tumors (114). Interestingly, the E-ras protein is overexpressed in bovine bladder tumors, binds to PDGFRβ and the available experimental data suggest it may contribute for tumorigenesis via Akt signaling (121). Additional studies are warranted to clarify the role of Ras in bovine bladder carcinogenesis. In mice, exposure to ptaquiloside or bracken fern is mainly associated with lymphoid malignancies (122, 123), but bladder urothelial dysplasia was observed upon ptaquiloside administration (123, 124). BPV-1 and BPV-2 (55, 125) have long been associated with urinary bladder tumors (126–129). More recently, BPV-13 (86, 130) and BPV-14 (131) have also been found in bovine urinary bladder tumors. Interestingly, recent data indicate that specific major histocompatibility complex class II alleles may protect against BPV infection and reduce the risk of bladder cancer in cattle (132). The BPV-1 E5 oncoprotein shows transforming properties in vitro and is able to induce cytoskeletal remodeling of murine and bovine cells (133). Unlike in human papillomavirus and other BPVs, the E5 oncoprotein is considered the main transforming protein of delta BPV genotypes, and is able to exert important cell functions such as down-regulating major histocompatibility complex proteins promoting immune evasion (47) and of activating the platelet-derived growth factor receptor beta, driving cell proliferation (134), as previously reviewed (12). Although papillomavirus in general show a tropism for stratified squamous keratinizing epithelia of the skin and mucosae, BPVs are believed to establish non-productive infections of the bladder urothelium. Expression of BPV E5 and E6 has been demonstrated in urinary bladder tumors (75, 97, 131), further supporting BPV's etiological role. Besides its established carcinogenic roles discussed in previous sections, the E5 oncoprotein has recently been shown to interact with E-ras and increase mitophagy in bovine bladder cancer (135), The fact that delta BPVs are able to transform stromal cells also lends support to their etiological role in bladder carcinogenesis, which is frequently characterized by mesenchymal or mixed epithelial-mesenchymal tumors. In fact, BPV-1-transgenic mice develop sarcoid-like mesenchymal lesions of the skin (136–138), but there are no reports of bladder lesions in this mouse model. However, BPV DNA may be detected in non-humoral bladder samples and BPV-negative cases of bladder cancer do occur (74), suggesting that bracken fern is a critical etiological factor. These observations call for additional studies on the interplay between BPV and Pteridium sp. in bladder carcinogenesis.
The 29 BPV types currently recognized induce a variety of lesions in different anatomic sites, some of which are associated with significant economic losses. In the case of BPV-4 and upper digestive tumors, the bracken toxins, in particular illudane glycosides, seem to act as tumor promoters, both by causing gene mutations and by suppressing the host's immune response, allowing viral persistence and cancer development. Accumulating data shows how ptaquiloside inactivates key immune cell populations involved in innate and adaptive immunity and synergizes with papillomavirus to promote upper digestive carcinogenesis. The co-occurrence of BPV infection and bracken exposure in cattle with bladder tumors is striking, but the precise mechanisms underlying the cooperation of these two factors remain are less clear. Bracken fern seems able to induce bladder tumors by itself, in the absence of BPV, but BPV is likely to enhance the carcinogenic effects of bracken illudane glycosides. On the one hand, the immune suppressive properties of bracken are likely to contribute for viral persistence. On the other hand, BPV can sustain cell proliferation via PDGFβ in the presence of genetic damage induced by bracken illudanes, allowing the accumulation of gene mutations and contributing for carcinogenesis. However, the precise gene targets of bracken toxins, initially thought to include H-ras, require additional clarification.
All authors listed have made a substantial, direct and intellectual contribution to the work, and approved it for publication.
This work was supported by the Research Center of the Portuguese Oncology Institute of Porto (project no. PI86-CI-IPOP-66-2017); by European Investment Funds by FEDER/COMPETE/POCI-Operational Competitiveness and Internationalization Program, and National Funds by FCT-Portuguese Foundation for Science and Technology under projects UID/AGR/04033/2020, UIDB/CVT/00772/2020 and by Base Funding-UIDB/00511/2020 of the Laboratory for Process Engineering, Environment, Biotechnology, and Energy LEPABE-funded by national funds through the FCT/MCTES (PIDDAC); Project 2SMART-engineered Smart materials for Smart citizens, with reference NORTE-01-0145-FEDER-000054, supported by Norte Portugal Regional Operational Programme (NORTE 2020), under the PORTUGAL 2020 Partnership Agreement, through the European Regional Development Fund (ERDF). BM-F was supported by FCT, 2020.07675.BD.
The authors declare that the research was conducted in the absence of any commercial or financial relationships that could be construed as a potential conflict of interest.
All claims expressed in this article are solely those of the authors and do not necessarily represent those of their affiliated organizations, or those of the publisher, the editors and the reviewers. Any product that may be evaluated in this article, or claim that may be made by its manufacturer, is not guaranteed or endorsed by the publisher.
1. Zheng Z-M, Baker CC. Papillomavirus genome structure, expression, and post-transcriptional regulation. Front Biosci J Virtual Libr. (2006) 11:2286–302. doi: 10.2741/1971
2. Pal A, Kundu R. Human papillomavirus E6 and E7: the cervical cancer hallmarks and targets for therapy. Front Microbiol. (2020) 10:3116. doi: 10.3389/fmicb.2019.03116
3. Antonsson A, Hansson BG. Healthy skin of many animal species harbors papillomaviruses which are closely related to their human counterparts. J Virol. (2002) 76:12537–42. doi: 10.1128/JVI.76.24.12537-12542.2002
4. Bernard HU, Burk RD, Chen Z, van Doorslaer K, zur Hausen H, de Villiers EM. Classification of Papillomaviruses (PVs) based on 189 PV types and proposal of taxonomic amendments. Virology. (2010) 401:70–79. doi: 10.1016/j.virol.2010.02.002
5. Van Doorslaer K, Chen Z, Bernard HU, Chan PKS, DeSalle R, Dillner J, et al. ICTV virus taxonomy profile: papillomaviridae. J Gen Virol. (2018) 99:989–90. doi: 10.1099/jgv.0.001105
6. Willemsen A, van den Boom A, Dietz J, Bilge Dagalp S, Dogan F, Bravo IG, et al. Genomic and phylogenetic characterization of ChPV2, a novel goat PV closely related to the Xi-PV1 species infecting bovines. Virol J. (2020) 17:167. doi: 10.1186/s12985-020-01440-9
7. Alp Avci G. Genomic organization and proteins of human papillomavirus. Mikrobiyol Bul. (2012) 46:507–15.
8. Rector A, Van Ranst M. Animal papillomaviruses. Virology. (2013) 445:213–23. doi: 10.1016/j.virol.2013.05.007
9. Akkutay-Yoldar Z, Oguzoglu T, Dogan F, Ataseven V. Detection of bovine papillomavirus in an equine cell line as a contaminant. Vlaams Diergeneeskd Tijdschr. (2019) 88:150–6. doi: 10.21825/vdt.v88i3.16019
10. de Villiers EM, Fauquet C, Broker TR, Bernard HU, zur Hausen H. Classification of papillomaviruses. Virology. (2004) 324:17–27. doi: 10.1016/j.virol.2004.03.033
11. Gil da Costa RM, Bastos MMSM, Oliveira PA, Lopes C. Bracken-associated human and animal health hazards: chemical, biological and pathological evidence. J. Hazard. Mater. (2012) 203–204:1–12. doi: 10.1016/j.jhazmat.2011.12.046
12. Bocaneti F, Altamura G, Corteggio A, Velescu E, Roperto F, Borzacchiello G. Bovine papillomavirus: new insights into an old disease. Transbound Emerg Dis. (2016) 63:14–23. doi: 10.1111/tbed.12222
13. Der JP, Thomson JA, Stratford JK, Wolf PG. Global chloroplast phylogeny and biogeography of bracken (Pteridium; Dennstaedtiaceae). Am J Bot. (2009) 96:1041–9. doi: 10.3732/ajb.0800333
14. Wolf PG, Rowe CA, Kinosian SP, Der JP, Lockhart PJ, Shepherd LD, et al. Worldwide relationships in the fern genus Pteridium (Bracken) based on nuclear genome markers. Am J Bot. (2019) 106:1365–76. doi: 10.1002/ajb2.1365
15. Kubicka K, Samecka-Cymerman A, Kolon K, Kosiba P, Kempers AJ. Chromium and nickel in Pteridium aquilinum from environments with various levels of these metals. Environ Sci Pollut Res Int. (2015) 22:527–34. doi: 10.1007/s11356-014-3379-5
16. Senyanzobe JMV, Mulei JM, Bizuru E, Nsengimuremyi C. Impact of Pteridium aquilinum on vegetation in Nyungwe Forest, Rwanda. Heliyon. (2020) 6:e04806. doi: 10.1016/j.heliyon.2020.e04806
17. Hirono I, Shibuya C, Fushimi K, Haga M. Studies on carcinogenic properties of Bracken, Pteridium aquilinum. JNCI. (1970) 45:179–88.
18. Imperato F. Flavonol glycosides from Pteridium aquilinum. Phytochemistry. (1995) 40:1801–2. doi: 10.1016/0031-9422(95)00593-V
19. Evans IA, Mason J. Carcinogenic activity of bracken. Nature. (1965) 208:913–4. doi: 10.1038/208913a0
20. Alonso-Amelot ME, Oliveros A, Calcagno-Pisarelli MP. Phenolics and condensed tannins in relation to altitude in Neotropical pteridium spp: a field study in the Venezuelan Andes. Biochem Syst Ecol. (2004) 32:969–81. doi: 10.1016/j.bse.2004.03.005
21. Niwa H, Ojika M, Wakamatsu K, Yamada K, Hirono I, Matsushita K. Ptaquiloside, a novel norsesquiterpene glucoside from bracken, Pteridium aquilinum var. latiusculum. Tetrahedron Lett. (1983) 24:4117–20. doi: 10.1016/S0040-4039(00)88276-3
22. Niwa H, Ojika M, Wakamatsu K, Yamada K, Ohba S, Saito Y, et al. Stereochemistry of ptaquiloside, a novel norsesquiterpene glucoside from bracken, Pteridium aquilinum var. latiusculum. Tetrahedron Lett. (1983) 24:5371–2. doi: 10.1016/S0040-4039(00)87871-5
23. van der Hoeven JC, Lagerweij WJ, Posthumus MA, van Veldhuizen A, Holterman HA. Aquilide A. a new mutagenic compound isolated from bracken fern (Pteridium aquilinum (L) Kuhn). Carcinogenesis. (1983) 4:1587–90. doi: 10.1093/carcin/4.12.1587
24. O'Connor PJ, Alonso-Amelot ME, Roberts SA, Povey AC. The role of bracken fern illudanes in bracken fern-induced toxicities. Mutat Res Rev Mutat Res. (2019) 782:108276. doi: 10.1016/j.mrrev.2019.05.001
25. Agnew MP, Lauren DR. Determination of ptaquiloside in bracken fern (Pteridium esculentum). J Chromatogr A. (1991) 538:462–8. doi: 10.1016/S0021-9673(01)88870-1
26. Smith BL, Seawright A. Bracken fern (Pteridium spp.) carcinogenicity and human health–a brief review. Nat. Toxins. (1995) 3:1–5. doi: 10.1002/nt.2620030102
27. Marçal WS, Gaste L, Netto NCR, Monteiro FA. Intoxicação aguda pela samambaia (Pteridium aquilinum, L. Kuhn), em bovinos da raça aberdeen angus. Arch Vet Sci. (2002) 7:77–81. doi: 10.5380/avs.v7i1.3972
28. Cruz GD, Bracarense APFRL. Role of bracken fern (Pteridium aquilinum (L.) Kuhn) in animal and human heath. Semina Ciênc. Agrár. (2004) 25:249–258. doi: 10.5433/1679-0359.2004v25n3p249
29. Pamukcu AM, Yalçiner S, Hatcher JF, Bryan GT. Quercetin, a rat intestinal and bladder carcinogen present in bracken fern (Pteridium aquilinum). Cancer Res. (1980) 40:3468–72.
30. Beniston RG, Morgan IM, O'Brien V, Campo MS. Quercetin, E7 and p53 in papillomavirus oncogenic cell transformation. Carcinogenesis. (2001) 22:1069–76. doi: 10.1093/carcin/22.7.1069
31. Zhu BT, Ezell EL, Liehr JG. Catechol-O-methyltransferase-catalyzed rapid O-methylation of mutagenic flavonoids. Metabolic inactivation as a possible reason for their lack of carcinogenicity in vivo. J Biol Chem. (1994) 269:292–9. doi: 10.1016/S0021-9258(17)42348-9
32. Utesch D, Feige K, Dasenbrock J, Broschard TH, Harwood M, Danielewska-Nikiel B, et al. Evaluation of the potential in vivo genotoxicity of quercetin. Mutat Res. (2008) 654:38–44. doi: 10.1016/j.mrgentox.2008.04.008
33. Culpeper N. The English Physician: Or, an Astrologo-physical Discourse of the Vulgar Herbs of this Nation. Benefit of the Commonwealth of England. (London: Peter Cole) (1652). doi: 10.5962/bhl.title.153561
34. Moon FE, Raafat MA. The experimental production of bracken “poisoning” in sheep. J Comp Pathol. (1951) 61:88–100. doi: 10.1016/S0368-1742(51)80009-2
35. Cushnie GH, Naftalin JM. Haematology of experimental bracken poisoning of cattle. I Changes in blood and bone marrow II Attempts to modify the course of the bone marrow damage. J Comp Pathol. (1956) 66:354–72. doi: 10.1016/S0368-1742(56)80038-6
37. Hirono I, Kono Y, Takahashi K, Yamada K, Niwa H, Ojika M, et al. Reproduction of acute bracken poisoning in a calf with ptaquiloside, a bracken constituent. Vet Rec. (1984) 115:375–8. doi: 10.1136/vr.115.15.375
38. de Oliveira LGS, Boabaid FM, Kisielius V, Rasmussen LH, Buroni F, Lucas M, et al. Hemorrhagic diathesis in cattle due to consumption of Adiantopsis chlorophylla (Swartz) Fée (Pteridaceae). Toxicon X. (2020) 5:100024. doi: 10.1016/j.toxcx.2020.100024
39. Meyer P. Thiaminase activities and thiamine content of Pteridium aquilinum, Equisetum ramosissimum, Malva parviflora, Pennisetum clandestinum and Medicago sativa. Onderstepoort J Vet Res. (1989) 56:145–6.
40. Watson WA, Terlecki S, Patterson DS, Sweasey D, Herbert CN, Done JT. Experimentally produced progressive retinal regeneration (bright blindness) in sheep. Br Vet J. (1972) 128:457–69. doi: 10.1016/S0007-1935(17)36785-4
41. Hirono I, Ito M, Yagyu S, Haga M, Wakamatsu K, Kishikawa T, et al. Reproduction of progressive retinal degeneration (bright blindness) in sheep by administration of ptaquiloside contained in bracken. J Vet Med Sci. (1993) 55:979–83. doi: 10.1292/jvms.55.979
42. He Z, Meng Q, Qiao J, Peng Y, Xie K, Liu Y, et al. Mixed nipple infections caused by variant of BPV3 and a putative new subtype of BPV in cattle. Transbound Emerg Dis. (2016) 63:e140–143. doi: 10.1111/tbed.12238
43. Stephens PE, Hentschel CC. The bovine papillomavirus genome and its uses as a eukaryotic vector. Biochem J. (1987) 248:1–11. doi: 10.1042/bj2480001
44. Rivoire WA Corleta HVE Brum IS Capp E. SciELO - Brazil - biologia molecular do câncer cervical biologia molecular do câncer cervical. Rev Bras Saúde Materno Infant. (2006) 6:447–51. doi: 10.1590/S1519-38292006000400012
45. Mazzuchelli-de-Souza J, Carvalho RF, Ruiz RM, Melo TC, Araldi RP, Carvalho E, et al. Expression and in silico analysis of the recombinant bovine papillomavirus E6 protein as a model for viral oncoproteins studies. BioMed Res. Int. (2013) 2013:421398. doi: 10.1155/2013/421398
46. Borzacchiello G, Roperto F. Bovine papillomaviruses, papillomas and cancer in cattle. Vet Res. (2008) 39:45. doi: 10.1051/vetres:2008022
47. Ashrafi GH, Tsirimonaki E, Marchetti B, O'Brien PM, Sibbet GJ, Andrew L, et al. Down-regulation of MHC class I by bovine papillomavirus E5 oncoproteins. Oncogene. (2002) 21:248–59. doi: 10.1038/sj.onc.1205008
48. Schapiro F, Sparkowski J, Adduci A, Suprynowicz F, Schlegel R, Grinstein S. Golgi alcalinization by the papillomavirus E5 oncoprotein. J Cell Biol. (2000) 148:305–15. doi: 10.1083/jcb.148.2.305
49. Roperto S, Russo V, Russo V, Borzacchiello G, Urraro C, Lucá R, et al. Bovine papillomavirus type 2 (BPV-2) E5 oncoprotein binds to the subunit D of the V1-ATPase proton pump in naturally occurring urothelial tumors of the urinary bladder in cattle. PLoS ONE. (2014) 9:e88860. doi: 10.1371/journal.pone.0088860
50. Suprynowicz FA, Baege A, Sunitha I, Schlegel R. s-Src activation by the E5 oncoprotein enables transformation independently of PDGF receptor activation. Oncogene. (2002) 21:1695–706. doi: 10.1038/sj.onc.1205223
51. Suprynowicz FA, Sparkowski J, Baege A, Schlegel R. E5 oncoprotein mutants activate phosphoinositide-3-kinase independently of platelet-derived growth factor receptor activation. J Biol Chem. (2000) 275:5111–9. doi: 10.1074/jbc.275.7.5111
52. Tong X, Howley PM. The bovine papillomavirus E6 oncoprotein interacts with paxillin and disrupts the actin cytoskeleton. Proc Natl Acad Sci U S A. (1997) 94:4412–7. doi: 10.1073/pnas.94.9.4412
53. DeMasi J, Huh K-W, Nakatani Y, Münger K, Howley PM. Bovine papillomavirus E7 transformation function correlates with cellular p600 protein binding. Proc Natl Acad Sci U S A. (2005) 102:11486–91. doi: 10.1073/pnas.0505322102
54. Faccini AM, Cairney M, Ashrafi GH, Finbow ME, Campo MS, Pitts JD. The bovine papillomavirus type 4 E8 protein binds to ductin and causes loss of gap junctional intercellular communication in primary fibroblasts. J Virol. (1996) 70:9041–5. doi: 10.1128/jvi.70.12.9041-9045.1996
55. Chen EY, Howley PM, Levinson AD, Seeburg PH. The primary structure and genetic organization of the bovine papillomavirus type 1 genome. Nature. (1982) 299:529–34. doi: 10.1038/299529a0
56. Silva FR C, da Cibulski SP, Daudt C, Weber MN, Guimarães LLB, Streck AF, et al. Novel bovine papillomavirus type discovered by rolling-circle amplification coupled with next-generation sequencing. PLoS ONE. (2016) 11:e0162345. doi: 10.1371/journal.pone.0162345
57. Daudt C, Da Silva FRC, Lunardi M, Alves CBDT, Weber MN, Cibulski SP, et al. Papillomaviruses in ruminants: an update. Transbound Emerg Dis. (2018) 65:1381–95. doi: 10.1111/tbed.12868
58. Tomita Y, Literak I, Ogawa T, Jin Z, Shirasawa H. Complete genomes and phylogenetic positions of bovine papillomavirus type 8 and a variant type from a European bison. Virus Genes. (2007) 35:243–9. doi: 10.1007/s11262-006-0055-y
59. Löhr CV, Juan-Sallés C, Rosas-Rosas A, Parás García A, Garner MM, Teifke JP. Sarcoids in captive zebras (Equus burchellii): association with bovine papillomavirus type 1 infection. J Zoo Wildl Med. (2005) 36:74–81. doi: 10.1638/03-126
60. Literák I, Tomita Y, Ogawa T, Shirasawa H, Smíd B, Novotny L, et al. Papillomatosis in a European bison. J Wildl Dis. (2006) 82:149–53. doi: 10.7589/0090-3558-42.1.149
61. Kidney BA, Berrocal A. Sarcoids in two captive tapirs (Tapirus bairdii): clinical, pathological and molecular study. Vet Dermatol. (2008) 19:380–4. doi: 10.1111/j.1365-3164.2008.00698.x
62. Silvestre O, Borzacchiello G, Nava D, Iovane G, Russo V, Vecchio D, et al. Bovine papillomavirus type 1 DNA and E5 oncoprotein expression in water buffalo fibropapillomas. Vet Pathol. (2009) 46:636–41. doi: 10.1354/vp.08-VP-0222-P-FL
63. Nasir L, Brandt S. Papillomavirus associated diseases of the horse. Vet Microbiol. (2013) 167:159–67. doi: 10.1016/j.vetmic.2013.08.003
64. Maxie G. Jubb, Kennedy & Palmer's Pathology of Domestic Animals - E-Book: Volume 2. Amsterdam: Elsevier Health Sciences (2015).
65. Munday JS, Thomson N, Dunowska M, Knight CG, Laurie RE, Hills S. Genomic characterisation of the feline sarcoid-associated papillomavirus and proposed classification as Bos taurus papillomavirus type 14. Vet Microbiol. (2015) 177:289–95. doi: 10.1016/j.vetmic.2015.03.019
66. Savini F, Gallina L, Prosperi A, Puleio R, Lavazza A, Di Marco P, et al. Bovine papillomavirus 1 gets out of the flock: detection in an ovine wart in sicily. Pathogens. (2020) 9:429. doi: 10.3390/pathogens9060429
67. Orbell GMB, Young S, Munday JS. Cutaneous sarcoids in captive African lions associated with feline sarcoid-associated papillomavirus infection. Vet Pathol. (2011) 48:1176–9. doi: 10.1177/0300985810391111
68. van Dyk E, Oosthuizen MC, Bosman A-M, Nel PJ, Zimmerman D, Venter EH. Detection of bovine papillomavirus DNA in sarcoid-affected and healthy free-roaming zebra (Equus zebra) populations in South Africa. J Virol Methods. (2009) 158:141–51. doi: 10.1016/j.jviromet.2009.02.008
69. Williams JH, van Dyk E, Nel PJ, Lane E, Van Wilpe E, Bengis RG, et al. Pathology and immunohistochemistry of papillomavirus-associated cutaneous lesions in Cape mountain zebra, giraffe, sable antelope and African buffalo in South Africa. J S Afr Vet Assoc. (2011) 82:97–106. doi: 10.4102/jsava.v82i3.59
70. Bauermann F, Joshi LR, Mohr K, Kutish G, Meier P, Chase C, et al. A novel bovine papillomavirus type in the genus Dyokappapapillomavirus. Arch Virol. (2017) 162:3225–8. doi: 10.1007/s00705-017-3443-9
71. Hamad MA, Al-Shammari AM, Odisho SM, Yaseen NY. Molecular epidemiology of bovine papillomatosis and identification of three genotypes in Central Iraq. Intervirology. (2017) 60:156–64. doi: 10.1159/000486594
72. Lucena RB, Rissi DR, Kommers GD, Pierezan F, Oliveira-Filho JC, Macêdo JTSA, et al. A retrospective study of 586 tumours in Brazilian cattle. J Comp Pathol. (2011) 145:20–4. doi: 10.1016/j.jcpa.2010.11.002
73. Roperto S, Russo V, Ozkul A, Sepici-Dincel A, Maiolino P, Borzacchiello G, et al. Bovine papillomavirus type 2 infects the urinary bladder of water buffalo (Bubalus bubalis) and plays a crucial role in bubaline urothelial carcinogenesis. J Gen Virol. (2013) 94:403–8. doi: 10.1099/vir.0.047662-0
74. Resendes AR, Roperto S, Trapani F, Urraro C, Rodrigues A, Roperto F, et al. Association of bovine papillomavirus type 2 (BPV-2) and urinary bladder tumours in cattle from the Azores archipelago. Res Vet Sci. (2011) 90:526–9. doi: 10.1016/j.rvsc.2010.02.001
75. Roperto S, Borzacchiello G, Brun R, Leonardi L, Maiolino P, Martano M, et al. A review of bovine urothelial tumours and tumour-like lesions of the urinary bladder. J Comp Pathol. (2010) 142:95–108. doi: 10.1016/j.jcpa.2009.08.156
76. Fenner F, Bachmann P. A., Gibbs E. P. J., Murphy F. A., Studdert M. J., White D. O. (1987). In: Veterinary Virology Academic Press, London. (pp. 321–328).
77. Patel KR, Smith KT, Campo MS. The nucleotide sequence and genome organization of bovine papillomavirus type 4. J Gen Virol 68 (Pt 8). (1987) 68:2117–28. doi: 10.1099/0022-1317-68-8-2117
78. Bloch N, Breen M. Bovine papillomavirus type 5: partial sequence and comparison with other bovine papillomaviruses. Virus Genes. (1997) 14:171–4. doi: 10.1023/A:1007960324424
79. da Silva FRC, Daudt C, Cibulski SP, Weber MN, Varela APM, Mayer FQ, et al. Genome characterization of a bovine papillomavirus type 5 from cattle in the Amazon region, Brazil. Virus Genes. (2017) 53:130–3. doi: 10.1007/s11262-016-1406-y
80. Jarrett WF, Campo MS, O'Neil BW, Laird HM, Coggins LW. A novel Bovine Papillomavirus (BPV-6) causing true epithelial papillomas of the mammary gland skin: a member of a proposed new BPV subgroup. Virology. (1984) 136:255–64. doi: 10.1016/0042-6822(84)90162-4
81. Ogawa T, Tomita Y, Okada M, Shirasawa H. Complete genome and phylogenetic position of bovine papillomavirus type 7. J Gen Virol. (2007) 88:1934–8. doi: 10.1099/vir.0.82794-0
82. Hatama S, Nobumoto K, Kanno T. Genomic and phylogenetic analysis of two novel bovine papillomaviruses, BPV-9 and BPV-10. J Gen Virol. (2008) 89:158–63. doi: 10.1099/vir.0.83334-0
83. Hatama S, Ishihara R, Ueda Y, Kanno T, Uchida I. Detection of a novel bovine papillomavirus type 11 (BPV-11) using xipapillomavirus consensus polymerase chain reaction primers. Arch Virol. (2011) 156:1281–5. doi: 10.1007/s00705-011-0970-7
84. Zhu W, Dong J, Shimizu E, Hatama S, Kadota K, Goto Y, et al. Characterization of novel bovine papillomavirus type 12 (BPV-12) causing epithelial papilloma. Arch Virol. (2012) 157:85–91. doi: 10.1007/s00705-011-1140-7
85. Lunardi M, de Alcântara BK, Otonel RAA, Rodrigues WB, Alfieri AF, Alfieri AA. Bovine papillomavirus type 13 DNA in equine sarcoids. J Clin Microbiol. (2013) 51:2167–71. doi: 10.1128/JCM.00371-13
86. Roperto S, Munday JS, Corrado F, Goria M, Roperto F. Detection of bovine papillomavirus type 14 DNA sequences in urinary bladder tumors in cattle. Vet Microbiol. (2016) 190:1–4. doi: 10.1016/j.vetmic.2016.04.007
87. Hu J, Zhang W, Chauhan SS, Shi C, Song Y, Zhao Y, et al. Complete genome and phylogenetic analysis of bovine papillomavirus type 15 in Southern Xinjiang dairy cow. J Vet Sci. (2020) 21:e73. doi: 10.4142/jvs.2020.21.e73
88. Daudt C, da Silva FRC, Streck AF, Weber MN, Mayer FQ, Cibulski SP, et al. How many papillomavirus species can go undetected in papilloma lesions? Sci. Rep. (2016) 6:36480. doi: 10.1038/srep36480
89. Daudt C, da Silva FRC, Cibulski SP, Streck AF, Laurie RE, Munday JS, et al. Bovine papillomavirus 24: a novel member of the genus Xipapillomavirus detected in the Amazon region. Arch Virol. (2019) 164:637–41. doi: 10.1007/s00705-018-4092-3
90. Bos taurus papillomavirus 25 isolate 14RS13/BR complete genome. (2018)Available at: http://www.ncbi.nlm.nih.gov/nuccore/MG252779.1 (accessed July 26, 2021).
91. González R, Murillo M, Castillo HEM, Jaén M, Villalobos-Cortés A. First report of bovine papillomavirus genotypes in cutaneous lesions by polymerase chain reaction (PCR) and direct sequencing in Panama. Afr J Biotechnol. (2019) 18:1098–104. doi: 10.5897/AJB2019.16975
92. Bos taurus papillomavirus 26 isolate 29RS14/BR complete genome. (2018). Available at: http://www.ncbi.nlm.nih.gov/nuccore/MG281846.1 (accessed July 26, 2021).
93. Bovine papillomavirus isolate ujs-21015 complete genome. (2018). Available at: http://www.ncbi.nlm.nih.gov/nuccore/MH512005.1 (accessed July 26, 2021).
94. Yamashita-Kawanishi N, Ito S, Ishiyama D, Chambers JK, Uchida K, Kasuya F, Haga T. Vet Microbiol. (2020) 250:108879. doi: 10.1016/j.vetmic.2020.108879
95. Campo MS, Moar MH, Jarrett WFH, Laird HM. A new papillomavirus associated with alimentary cancer in cattle. Nature. (1980) 286:180–2. doi: 10.1038/286180a0
96. Marrero E, Bulnes C, Sánchez LM, Palenzuela I, Stuart R, Jacobs F, et al. Pteridium aquilinum (bracken fern) toxicity in cattle in the humid Chaco of Tarija, Bolivia. Vet Hum Toxicol. (2001) 43:156–8.
97. Borzacchiello G, Iovane G, Marcante ML, Poggiali F, Roperto F, Roperto S, et al. Presence of bovine papillomavirus type 2 DNA and expression of the viral oncoprotein E5 in naturally occurring urinary bladder tumours in cows. J Gen Virol. (2003) 84:2921–6. doi: 10.1099/vir.0.19412-0
98. Tsirimonaki E, O'Neil BW, Williams R, Campo MS. Extensive papillomatosis of the bovine upper gastrointestinal tract. J Comp Pathol. (2003) 129:93–9. doi: 10.1016/S0021-9975(03)00007-0
99. Faccin TC, Cargnelutti JF, Rodrigues FdeS, de Menezes FR, Piazer JVM, et al. Bovine upper alimentary squamous cell carcinoma associated with bracken fern poisoning: clinical-pathological aspects and etiopathogenesis of 100 cases. PLoS ONE. (2018) 13:e0204656. doi: 10.1371/journal.pone.0204656
100. Munday JS. Bovine and human papillomaviruses: a comparative review. Vet Pathol. (2014) 51:1063–75. doi: 10.1177/0300985814537837
101. Gil da Costa RM, Aragão S, Moutinho M, Alvarado A, Carmo D, Casaca F, et al. HPV16 induces a wasting syndrome in transgenic mice: amelioration by dietary polyphenols via NF-κB inhibition. Life Sci. (2017) 169:11–19. doi: 10.1016/j.lfs.2016.10.031
102. Prakash AS, Pereira TN, Smith BL, Shaw G, Seawright AA. Mechanism of bracken fern carcinogenesis: evidence for H-ras activation via initial adenine alkylation by ptaquiloside. Nat Toxins. (1996) 4:221–7. doi: 10.1002/(SICI)(1996)4:5<221::AID-NT4>3.0.CO;2-Q
103. Shahin M, Moore MR, Worrall S, Smith BL, Seawright AA, Prakash AS. H-ras activation is an early event in the ptaquiloside-induced carcinogenesis: comparison of acute and chronic toxicity in rats. Biochem Biophys Res Commun. (1998) 250:491–7. doi: 10.1006/bbrc.1998.9341
104. Nagao T, Saito K, Hirayama E, Uchikoshi K, Koyama K, Natori S, et al. Mutagenicity of ptaquiloside, the carcinogen in bracken, and its related illudane-type sesquiterpenes. I Mutagenicity in Salmonella typhimurium. Mutat Res. (1989) 215:173–8. doi: 10.1016/0027-5107(89)90181-4
105. Costa RMGda, Coelho P, Sousa R, Bastos MMSM, Porto B, Teixeira JP, et al. Multiple genotoxic activities of ptaquiloside in human lymphocytes: aneugenesis, clastogenesis and induction of sister chromatid exchange. Mutat. Res. (2012) 747:77–81. doi: 10.1016/j.mrgentox.2012.04.010
106. Latorre AO, Furlan MS, Sakai M, Fukumasu H, Hueza IM, Haraguchi M, et al. Immunomodulatory effects of Pteridium aquilinum on natural killer cell activity and select aspects of the cellular immune response of mice. J Immunotoxicol. (2009) 6:104–14. doi: 10.1080/15476910902972465
107. Zhang Y, Yang Q, Zeng X, Wang M, Dong S, Yang B, et al. MET amplification attenuates lung tumor response to immunotherapy by inhibiting STING. Cancer Discov. (2021). doi: 10.1158/2159-8290.CD-20-1500
108. Santos C, Vilanova M, Medeiros R, Gil da Costa RM. HPVtransgenic mouse models: tools for studying the cancer-associated immune response. Virus Res. (2017) 235:49–57. doi: 10.1016/j.virusres.2017.04.001
109. Latorre AO, Caniceiro BD, Wysocki HL, Haraguchi M, Gardner DR, Górniak SL. Selenium reverses Pteridium aquilinum-induced immunotoxic effects. Food Chem Toxicol. (2011) 49:464–70. doi: 10.1016/j.fct.2010.11.026
110. De Falco F, Cutarelli A, Gentile I, Cerino P, Uleri V, Catoi AF, et al. Bovine delta papillomavirus E5 oncoprotein interacts with TRIM25 and hampers antiviral innate immune response mediated by RIG-I-like receptors. Front Immunol. (2021) 12:658762. doi: 10.3389/fimmu.2021.658762
111. Rosenberger G, Heeschen W. Adler-farn (Pteris aquilina) – die Ursache des Sog. Stalrotes der Rinder Deut Tierärtz Woch. (1960) 67:201–8.
112. Rosenberger G, Von LA. (Pteris aquilina) – die Ursache der chronischen vesikalen Haematurie des Rindes. Wien Tierärztl Mon. (1965) 52:415–21.
113. Dawra RK, Kurade NP, Sharma OP. Carcinogenicity of the fern Pteridium aquilinum collected from enzootic bovine haematuria-free hilly area in India. Curr Sci. (2002) 83:1005–9.
114. Sardon D, de la Fuente I, Calonge E, Perez-Alenza MD, Castaño M, Dunner S, et al. H-ras immunohistochemical expression and molecular analysis of urinary bladder lesions in grazing adult cattle exposed to bracken fern. J. Comp. Pathol. (2005) 132, 195–201. doi: 10.1016/j.jcpa.2004.09.011
115. Carvalho T, Pinto C, Peleteiro MC. Urinary bladder lesions in bovine enzootic haematuria. J Comp Pathol. (2006) 134:336–46. doi: 10.1016/j.jcpa.2006.01.001
116. Carvalho T, Elias AP, Nunes T, Peleteiro MC, Dias S. Chemo-angiogenic profile of bovine urinary bladder tumors distinguishes urothelial carcinomas from hemangiosarcomas. Vet Immunol Immunopathol. (2008) 121:344–58. doi: 10.1016/j.vetimm.2007.09.020
117. Pamukcu AM, Goksoy SK, Price JM. Urinary bladder neoplasms induced by feeding bracken fern. Cancer Res. (1967) 27:917–24.
118. Price JM, Pamukcu AM. The induction of neoplasms of the urinary bladder of the cow and the small intestine of the rat by feeding bracken fern (Pteris aquilina). Cancer Res. (1968) 28:2247–51.
119. França T, do N, Tokarnia CH, Peixoto PV. Enfermidades determinadas pelo princípio radiomimético de Pteridium aquilinum (Polypodiaceae). Pesqui Veterinária Bras. (2002) 22:85–96. doi: 10.1590/S0100-736X2002000300001
120. Bringuier PP, Piaton E, Berger N, Debruyne F, Perrin P, Schalken J, et al. Bracken fern-induced bladder tumors in guinea pigs. A model for human neoplasia. Am J Pathol. (1995) 147:858–68.
121. Russo V, Roperto F, Esposito I, Ceccarelli DM, Zizzo N, Leonardi L, et al. ERas protein is overexpressed and binds to the activated platelet-redived growth factor receptor β receptor in bovine urothelial tumour cells associated with papillomavirus infection. Vet J. (2016) 212:44–7. doi: 10.1016/j.tvjl.2016.01.004
122. Pamukcu AM, Ertürk E, Price JM, Bryan GT. Lymphatic leukemia and pulmonary tumors in female Swiss mice fed bracken fern (Pteris aquilina). Cancer Res. (1972) 32:1442–5.
123. Gil da Costa RM, Oliveira PA, Vilanova M, Bastos MMSM, Lopes CC, Lopes C. Ptaquiloside-induced, B-cell lymphoproliferative and early-stage urothelial lesions in mice. Toxicon. (2011) 58:543–549. doi: 10.1016/j.toxicon.2011.08.016
124. Gil da Costa RM, Oliveira PA, Bastos MMSM, Lopes CC, Lopes C. Ptaquiloside-induced early-stage urothelial lesions show increased cell proliferation and intact β-catenin and E-cadherin expression: immunohistochemistry of ptaquiloside-induced lesions. Environ Toxicol. (2014) 29:763–769. doi: 10.1002/tox.21803
125. Lancaster WD, Olson C. Demonstration of two distinct classes of bovine papilloma virus. Virology. (1978) 89:372–9. doi: 10.1016/0042-6822(78)90179-4
127. Campo MS, Jarrett WF, Barron R, O'Neil BW, Smith KT. Association of bovine papillomavirus type 2 and bracken fern with bladder cancer in cattle. Cancer Res. (1992) 52:6898–904.
128. Wosiacki SR, Claus MP, Alfieri AF, Alfieri AA. Bovine papillomavirus type 2 detection in the urinary bladder of cattle with chronic enzootic haematuria. Mem Inst Oswaldo Cruz. (2006) 101:635–8. doi: 10.1590/S0074-02762006000600009
129. Campo MS. Bovine papillomavirus and cancer. Vet J Lond Engl. (1997) 154:175–88. doi: 10.1016/S1090-0233(97)80019-6
130. de Alcântara BK, Lunardi M, Agnol AMD, Alfieri AF, Alfieri AA. Detection and quantification of the E6 Oncogene in bovine papillomavirus types 2 and 13 from urinary bladder lesions of cattle. Front Vet Sci. (2021) 8:673189. doi: 10.3389/fvets.2021.673189
131. Roperto S, Russo V, Leonardi L, Martano M, Corrado F, Riccardi MG, et al. Bovine papillomavirus type 13 expression in the urothelial bladder tumours of cattle. Transbound Emerg Dis. (2016) 63:628–34. doi: 10.1111/tbed.12322
132. Longeri M, Russo V, Strillacci MG, Perillo A, Carisetti M, Cozzi MC, et al. Association between BoLA-DRB3.2 polymorphism and bovine papillomavirus infection for bladder tumor risk in Podolica cattle. Front. Vet. Sci. (2021) 8:630089. doi: 10.3389/fvets.2021.630089
133. Tsirimonaki E, Ullah R, Marchetti B, Ashrafi GH, McGarry L, Ozanne B, et al. Similarities and differences between the E5 oncoproteins of bovine papillomaviruses type 1 and type 4: cytoskeleton, motility and invasiveness in E5-transformed bovine and mouse cells. Virus Res. (2006) 115:158–68. doi: 10.1016/j.virusres.2005.08.003
134. Karabadzhak AG, Petti LM, Barrera FN, Edwards APB, Moya-Rodríguez A, Polikanov YS, et al. Two transmembrane dimers of the bovine papillomavirus E5 oncoprotein clamp the PDGF β receptor in an active dimeric conformation. Proc Natl Acad Sci USA. (2017) 114:E7262–71. doi: 10.1073/pnas.1705622114
135. De Falco F, Urraro C, Cutarelli A, Roperto S. Bovine papillomavirus E5 oncoprotein upregulates parkin-dependent mitophagy in urothelial cells ofcattle with spontaneous papillomavirus infection: a mechanistic study. Comp Immunol Infect Dis. (2020) 70:101463. doi: 10.1016/j.cimid.2020.101463
136. Lacey M, Alpert S, Hanahan D. Bovine papillomavirus genome elicits skin tumours in transgenic mice. Nature. (1986) 322:609–12. doi: 10.1038/322609a0
137. Lindgren V, Sippola-Thiele M, Skowronski J, Wetzel E, Howley PM, Hanahan D. Specific chromosomal abnormalities characterize fibrosarcomas of bovine papillomavirus type 1 transgenic mice. Proc Natl Acad Sci USA. (1989) 86:5025–9. doi: 10.1073/pnas.86.13.5025
Keywords: papillomaviruses, bovine papillomavirus, cancer, molecular epidemiology ptaquiloside, bladder
Citation: Medeiros-Fonseca B, Abreu-Silva AL, Medeiros R, Oliveira PA and Gil da Costa RM (2021) Pteridium spp. and Bovine Papillomavirus: Partners in Cancer. Front. Vet. Sci. 8:758720. doi: 10.3389/fvets.2021.758720
Received: 14 August 2021; Accepted: 06 October 2021;
Published: 02 November 2021.
Edited by:
Francisco Javier Salguero, Public Health England, United KingdomReviewed by:
John Munday, Massey University, New ZealandCopyright © 2021 Medeiros-Fonseca, Abreu-Silva, Medeiros, Oliveira and Gil da Costa. This is an open-access article distributed under the terms of the Creative Commons Attribution License (CC BY). The use, distribution or reproduction in other forums is permitted, provided the original author(s) and the copyright owner(s) are credited and that the original publication in this journal is cited, in accordance with accepted academic practice. No use, distribution or reproduction is permitted which does not comply with these terms.
*Correspondence: Rui M. Gil da Costa, cm1jb3N0YUBmZS51cC5wdA==
Disclaimer: All claims expressed in this article are solely those of the authors and do not necessarily represent those of their affiliated organizations, or those of the publisher, the editors and the reviewers. Any product that may be evaluated in this article or claim that may be made by its manufacturer is not guaranteed or endorsed by the publisher.
Research integrity at Frontiers
Learn more about the work of our research integrity team to safeguard the quality of each article we publish.