- Department of Epidemiology, School of Public Health, Guangdong Provincial Key Laboratory of Tropical Disease Research, Southern Medical University, Guangzhou, China
Rodents are important hosts of hantaviruses, and lungs and kidneys are known to be the preferred organs of these viruses. Recently, hantaviruses were detected in liver samples from wild rodents in Hungary and the United States, and feeder rats in the Netherlands. However, few studies have detected hantaviruses in the liver of rats from China. In this study, hantaviruses were investigated in liver samples from R. norvegicus and R. tanezumi trapped in urban areas of southern China. A total of 461 R. norvegicus and 64 R. tanezumi were trapped. Using a pan-hantavirus PCR method, hantaviruses were detected in liver, lung, and serum samples from these animals. About 7.43% of liver samples were positive for Seoul virus (SEOV). The detection rate of SEOV in liver samples from R. norvegicus (8.24%) was higher than that from R. tanezumi (1.56%), suggesting the predominant role of R. norvegicus in the transmission of SEOV in urban areas of China. Three R. norvegicus had SEOV RNA in their liver samples but not in their lung samples, suggesting that the liver might be one of the targeted organs of SEOV. The first full SEOV protein-coding sequences (CDS) of the S and M segments, and partial CDS of the L segment from R. tanezumi were amplified. Several full and partial CDS of the S, M, and L segments from R. norvegicus were also obtained. The SEOV sequences obtained from different animals were highly similar, suggesting the cross-species transmission potential of SEOV between R. norvegicus and R. tanezumi.
Introduction
Hantaviruses belong to the subfamily Mammantavirinae within the family hantaviridae (1). They are enveloped, single-stranded, negative-sense RNA viruses, with genomes composed of small (S), medium (M), and large (L) segments (2). The L segment encodes the viral RNA-dependent RNA polymerase (RdRp), while the M and S segments encode the two envelope proteins (Gn and Gc) and the nucleocapsid protein (N), respectively (2). To date, more than 50 hantavirus species have been recognized (3). Some of these viruses are not pathogenic, such as the Prospect Hill virus (4), but at least 24 species of hantaviruses are able to cause human diseases (5). Hantaviruses mainly cause two diseases in humans: hemorrhagic fever with renal syndrome (HFRS), and hantavirus cardiopulmonary syndrome (HCPS) (6).
Literature describing hantavirus infections in China dates back to the 12th century (7). Even today, hantavirus infections remain a serious health concern in China, accounting for 90% of HFRS cases globally (8). The most common hantavirus species responsible for causing HFRS are the Dobrava–Belgrade, Hantaan, Seoul, and Puumala viruses (9).
Rodents are reservoirs of HFRS-associated hantaviruses, and each hantavirus associated with a distinct rodent host species (10). Myodes rodent species usually host Puumala viruses, Apodemus rodent species commonly host Dobrava–Belgrade viruses, and Rattus and Apodemus rodent species usually host Seoul virus (SEOV) and Hantaan virus (HTNV) (11). The population densities, community composition and species distributions of rodents have important influences on HFRS transmission (12, 13). In China, SEOV and HTNV are the two dominant hantavirus species that cause HFRS in urban and agricultural areas, respectively (14). Rattus norvegicus (Norway rat) is the predominant reservoir of SEOV in urban residential areas in China (8). Other Rattus species rodents, such as Rattus tanezumi (Asian house rat), are also prevalent in urban areas of southern China, and are also hosts of SEOV (15).
Understanding the natural infection of hantaviruses to reservoir hosts is important for clarifying the pathogenesis and transmission routes of the viruses. The main transmission routes of hantaviruses are usually direct contact with rodents or inhalation of contaminated aerosols from excretions or secretions of infected animals (16). Most of the studies to date have reported hantaviruses in lung and kidney samples from rodents (17, 18), with only a few studies focusing on hantaviruses in other organs, such as the liver (1, 19). A recent study reported the detection of Dobrava–Belgrade virus, Puumala virus, and Tula virus in liver tissue samples from Apodemus, Myodes, and Microtus rodent species in Hungary (19). SEOV has been detected in liver tissue samples from feeder rats in the Netherlands and from wild R. norvegicus in New York (1, 20). Furthermore, histopathologic changes associated with SEOV infection have primarily been observed in liver samples from feeder rats (1). In addition to HFRS, SEOV can also cause other diseases in humans, such as mild hepatitis (21). Therefore, it is important to investigate SEOV in the liver of naturally infected wild reservoir hosts to understand its tropism and pathogenesis. In this study, SEOV in liver tissue samples from urban Rattus species rodents in southern China were investigated.
Materials and Methods
Sample Collection
Animals were trapped in five regions in southern China (Guangzhou City and Maoming City in Guangdong Province, Yiyang City in Hunan Province, Xiamen City in Fujian Province, and Malipo County in Yunan Province) between 2014 and 2018 (Figure 1 and Table 1). All animals were captured near human residences using cage traps. The trapped animals were anesthetized by 3% diethyl ether inhalation, with the dosage adjusted according to their heart rate, respiratory frequency, corneal reflection, and the extremity muscle tension. Trained personnel wore filtering facepiece respirators, chemical safety goggles, anti-static uniforms, and chemical protective gloves to protect them from diethyl ether. Blood was drawn by cardiac puncture and centrifuged to obtain serum samples. Animals were then executed by cervical dislocation. Liver tissue and lung tissue samples were obtained and soaked in RNAlater (Invitrogen, Carlsbad, CA, USA). All of the samples were stored at −80°C prior to processing. The species of each animal was identified by sequencing cytochrome B (cytB) gene (22).
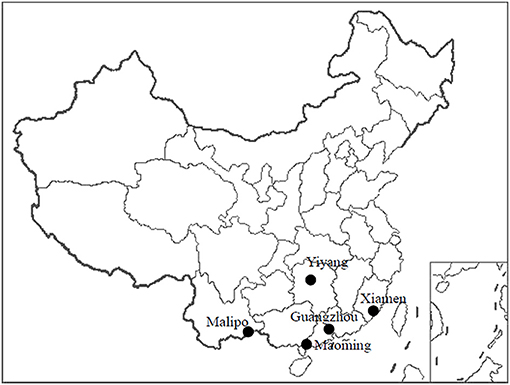
Figure 1. Locations of the animal trapping sites in China. Map source: https://image.so.com/view?q=%E4%B8%AD%E5%9B%BD%E5%9C%B0%E5%9B%BE&src=srp&correct=%E4%B8%AD%E5%9B%BD%E5%9C%B0%E5%9B%BE&ancestor=list&cmsid=50712c46325496ee4c7fbfbded18f06e&cmras=0&cn=0&gn=0&kn=50&crn=0&bxn=20&fsn=130&cuben=0&pornn=0&manun=50&adstar=0&clw=233#id=812433445ba11b77cba553308beab1d9&currsn=0&ps=136&pc=136.
Extraction of Nucleic Acid and Hantavirus Detection
Total RNA and DNA were extracted from ~20 mg of liver tissue samples or 200 μL aliquot of serum samples using the MiniBEST Viral RNA/DNA Extraction Kit (TaKaRa, Kusatsu, Japan). Given the previous report that lung was the most preferred organ for the detection of SEOV (1), total RNA and DNA were also extracted from ~20 mg of lung tissue samples from animals with SEOV-positive liver tissue samples using the MiniBEST Viral RNA/DNA Extraction Kit (TaKaRa).
A nested PCR assay designed based on a conserved region within the L segment of hantaviruses was used to detect currently known and possible novel members within the genus Orthohantavirus (23). The amplified products were separated on a 1.5% agarose gel, and positive samples were sent to the Beijing Genomics Institute (Shenzhen, China) for sequencing. All the PCR screening sequences were aligned with the sequences in the NCBI nucleotide sequence (NCBI NT) database (https://www.ncbi.nlm.nih.gov/nucleotide) by using BLASTN.
Genomic Sequencing
Based on the reference sequences (L segment: KX079474.1, KM948596.1; M segment: KM948597.1, M34882.1; S segment: NC_005236.1, EF192308.1), primers were designed to amplify the near full-length genome of SEOV (Additional File 1). After sequencing all of the fragments, the amplified sequences were aligned with the sequences in the NCBI nucleotide sequence (NCBI NT) database (https://www.ncbi.nlm.nih.gov/nucleotide) by using BLASTN. Fragments that did not represent SEOV were excluded. Then the Lasergene SeqMan software (DNASTAR, Madison, WI, USA) was used to assemble the sequences with reference-based approach (assemble method: pro assemble). Unassembled singletons were verified by amplification using different primers that designed based on the SEOV sequences to ensure they represent SEOV.
Phylogenetic Analysis
All of the screening sequences, and the full and partial protein-coding sequences (CDS) obtained in this study were aligned with hantavirus genomic sequences from GenBank (http://www.ncbi.nlm.nih.gov/genbank/) using the ClustalW multiple sequence alignment program in MEGA (version 7.0; Oxford Molecular, Cambridge, UK). Phylogenetic trees were constructed based on the PCR screening sequences, and full and partial CDS of the S, M, and L fragments via MrBayes (version 3.2; https://www.nbisweden.github.io/MrBayes/). Using the GTR + G + I nucleotide substitution matrix, two million Markov chain Monte Carlo (MCMC) iterations were sampled every 100 steps to obtain 20,000 trees, with the burn-in generally being 25% of the tree replicates (24). The identities of the amino acid and nucleotide sequences were estimated using the Sequence Identity Matrix program in BioEdit (version 7.2.5; https://file.org/free-download/bioedit). Recombination events were investigated using SimPlot (version 3.5.1; https://sray.med.som.jhmi.edu/SCRoftware/SimPlot/).
Selective Pressure Analysis
Selective pressure analyses were performed based on the full and partial CDS of the S, M, and L segments. The neighbor-joining method (Kimura 2-parameter model) in MEGA (version 7.0; Oxford Molecular, Cambridge, UK) was used to construct phylogenetic trees. The free-ratio model in CODEML in EasyCodeML (version 1.21; https://github.com/BioEasy/EasyCodeML) was used to calculate the dN (nonsynonymous substitution), dS (synonymous substitution), and ω-values (dN/dS) for each branch (25). The ω-values of >1, 1, or <1 indicating positive selection, neutral evolution, or negative selection, respectively. Site models (M0, M1a, M2a, M3, M7, M8, and M8a) were applied to detect potential selection among sites. We also used likelihood-ratio tests (LRTs) to assess these models and the Bayes Empirical Bayes (BEB) to evaluate the posterior probability of positive selection sites (26). Positive selection sites were identified as those with a BEB score larger than 0.95.
Statistical Analysis
Statistical analysis was performed using the Statistical Product and Service Solutions software (SPSS, version 13.0; IBM, Armonk, NY, USA). Descriptive statistics (Crosstabs) were used to assess detection rates. Chi-square tests were performed to test differences in detection rates across different animals and different sampling locations. P < 0.05 was considered to be statistically significant.
Ethical Guidelines
The study protocol was approved by the Animal Ethics and Welfare Committee of the School of Public Health, Southern Medical University and adhered to the guidelines for the Rules for the Implementation of Laboratory Animal Medicine (1998) from the Ministry of Health, China. All surgical procedures were performed under anesthesia in efforts to minimize the suffering of the animals. Endangered or protected animal species were not included in this study.
Results
Detection of SEOV in Liver, Serum, and Lung Samples From Urban Rattus Species Rodents
From 2014 to 2018, a total of 525 Rattus species rodents were trapped, including 461 Rattus norvegicus and 64 Rattus tanezumi (Figure 1 and Table 1). Viral RNA and DNA were extracted from 525 liver tissue samples, while only 190 serum samples (more than 200 μL) were sufficient for nucleic acid extraction.
All of the PCR screening sequences obtained in our study belonged to SEOV (accession numbers: OL364860–OL364936). A total of 7.43% (39/525) of the liver tissue samples were positive for SEOV, with the detection rates of 8.24% (38/461) and 1.56% (1/64) in R. norvegicus and R. tanezumi, respectively (χ2 = 3.647, P > 0.05; Table 1). R. norvegicus trapped in Guangzhou City had the highest detection rate of SEOV (13.43%), followed by R. norvegicus trapped in Xiamen City (8.57%) and Maoming City (4.65%). All of the SEOV-positive serum samples were from R. norvegicus (1.76%, 3/170; Table 2), and the liver tissue samples from these three animals were also positive for SEOV.
Lung tissue samples from rats with SEOV-positive liver samples were used to detect SEOV RNA. Aside from the missing lung tissue sample from animal MM86, a total of 38 lung tissue samples were obtained. Among these samples, three lung tissue samples tested negative for SEOV (Table 3).
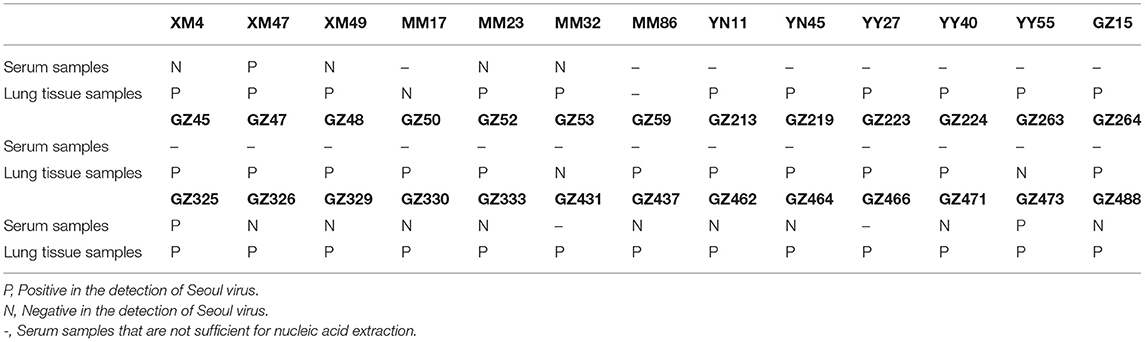
Table 3. Seoul virus in the lung tissue and serum samples from animals with Seoul virus-positive liver tissue samples.
Genomic Amplification and Phylogenetic Analysis
The sequences detected in different samples from the same animal were highly similar (>99% at the nucleotide level; Additional File 2), and the sequences from animals trapped in the same sampling location usually clustered together (Figure 2).
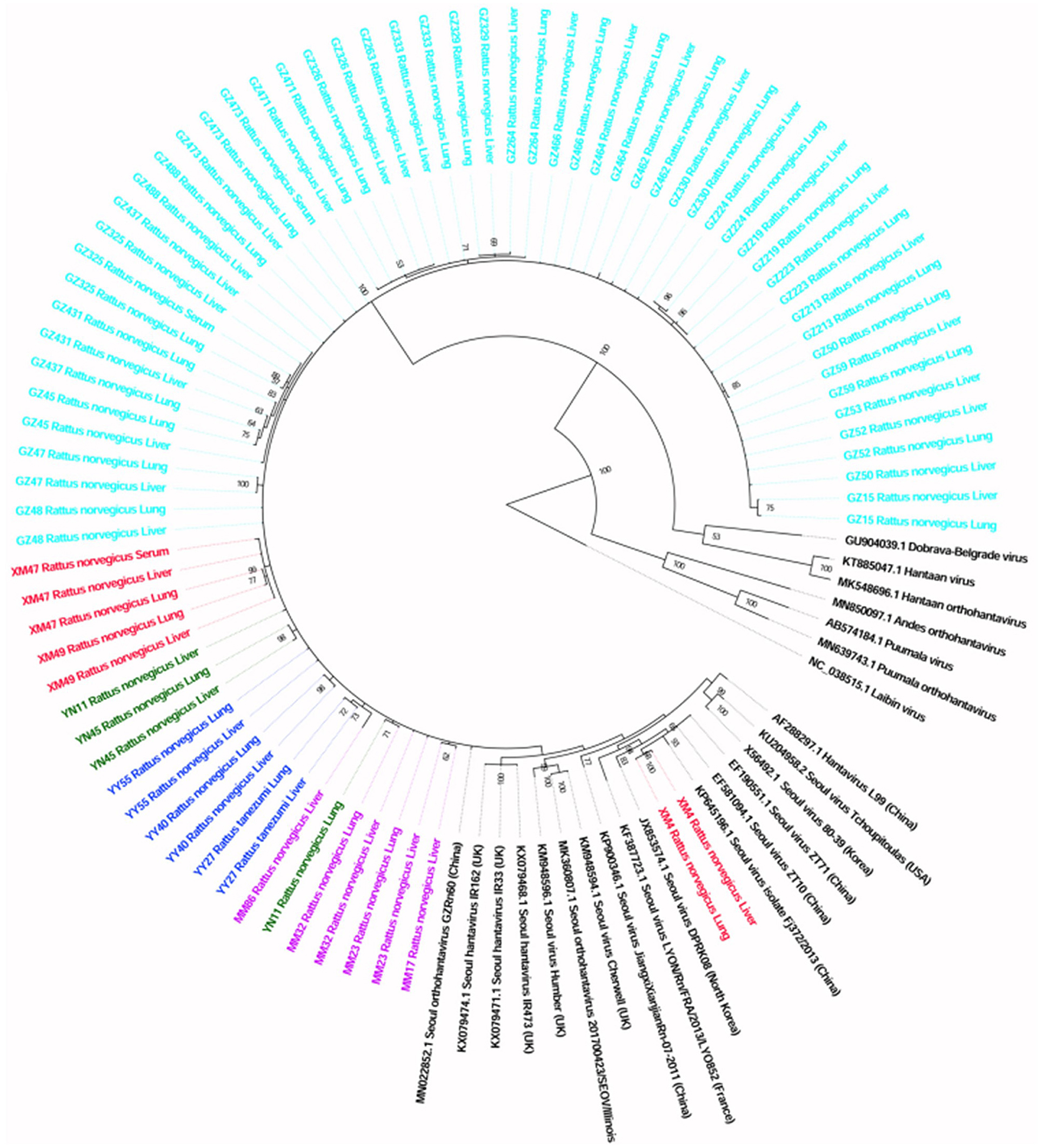
Figure 2. Phylogenetic tree constructed using PCR screening nucleotide sequences of Seoul virus obtained from rats. Twenty-two representative orthohantavirus sequences were included for comparison, and one Laibin virus sequence was set as the outgroup. The percentages of the posterior probability (PP) values are indicated.
A total of six full and two partial CDS of the S segment (GenBank accession numbers: MZ031957-MZ031964), eight partial CDS of the M segment (GenBank accession numbers: MZ031948-MZ031955), and eight partial CDS of the L segment were obtained from R. norvegicus (GenBank accession numbers: MZ031938- MZ031944, MZ031946). In addition, the first full CDS of the S (GenBank accession number: MZ031956) and M (GenBank accession number: MZ031947) segments, and partial CDS of the L (GenBank accession number: MZ031945) segment were also amplified from R. tanezumi. High level of similarity was found between the full and partial CDS obtained from R. norvegicus and R. tanezumi, indicating the cross-species transmission potential of SEOV between these species (Figures 3–5 and Additional Files 3–5). Most of the CDS clustered with SEOV sequences obtained from rats in China, while the partial CDS of the L segment from R. tanezumi was most similar to one SEOV sequence detected in R. norvegicus from the United Kingdom. Further analyses revealed no recombination events in the sequences obtained in our study.
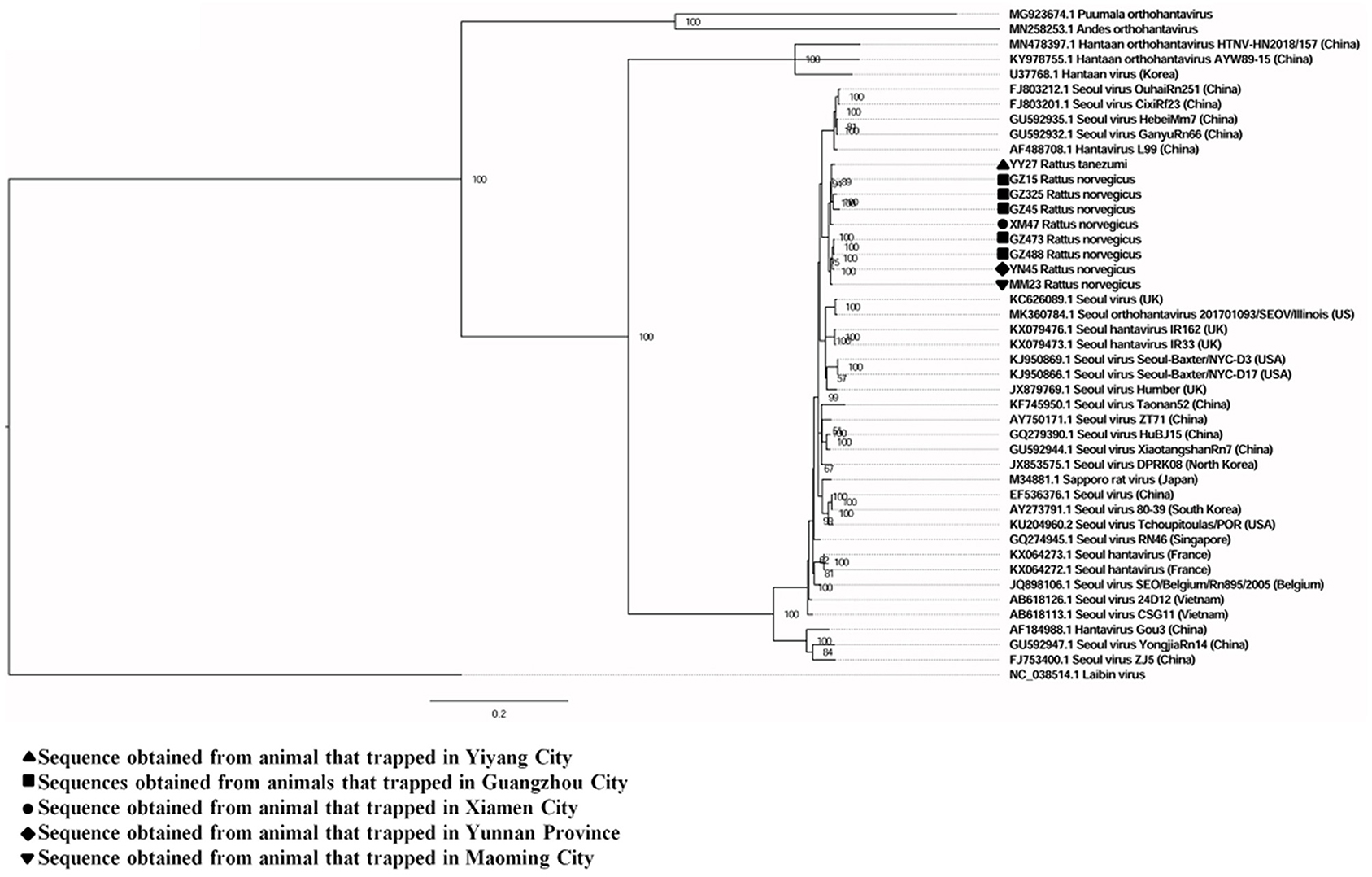
Figure 3. Phylogenetic tree constructed using nucleotide sequences of the Seoul virus S gene obtained from rats. Thirty-five representative orthohantavirus sequences were included for comparison, and one Laibin virus sequence was set as the outgroup. The percentages of the posterior probability (PP) values are indicated.
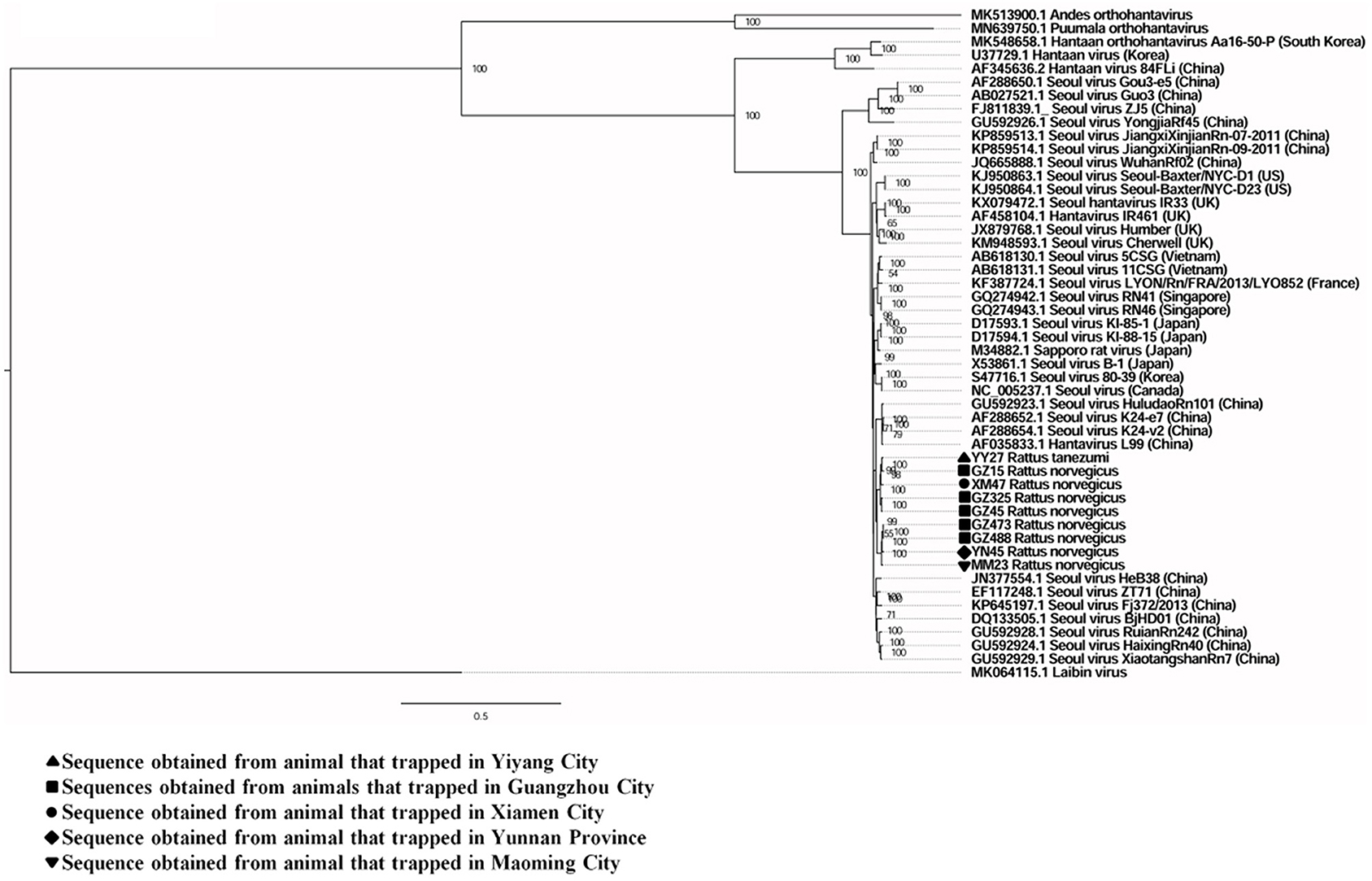
Figure 4. Phylogenetic tree constructed using nucleotide sequences of the Seoul virus M gene obtained from rats. Forty representative orthohantavirus sequences were included for comparison, and one Laibin virus sequence was set as the outgroup. The percentages of the posterior probability (PP) values are indicated.
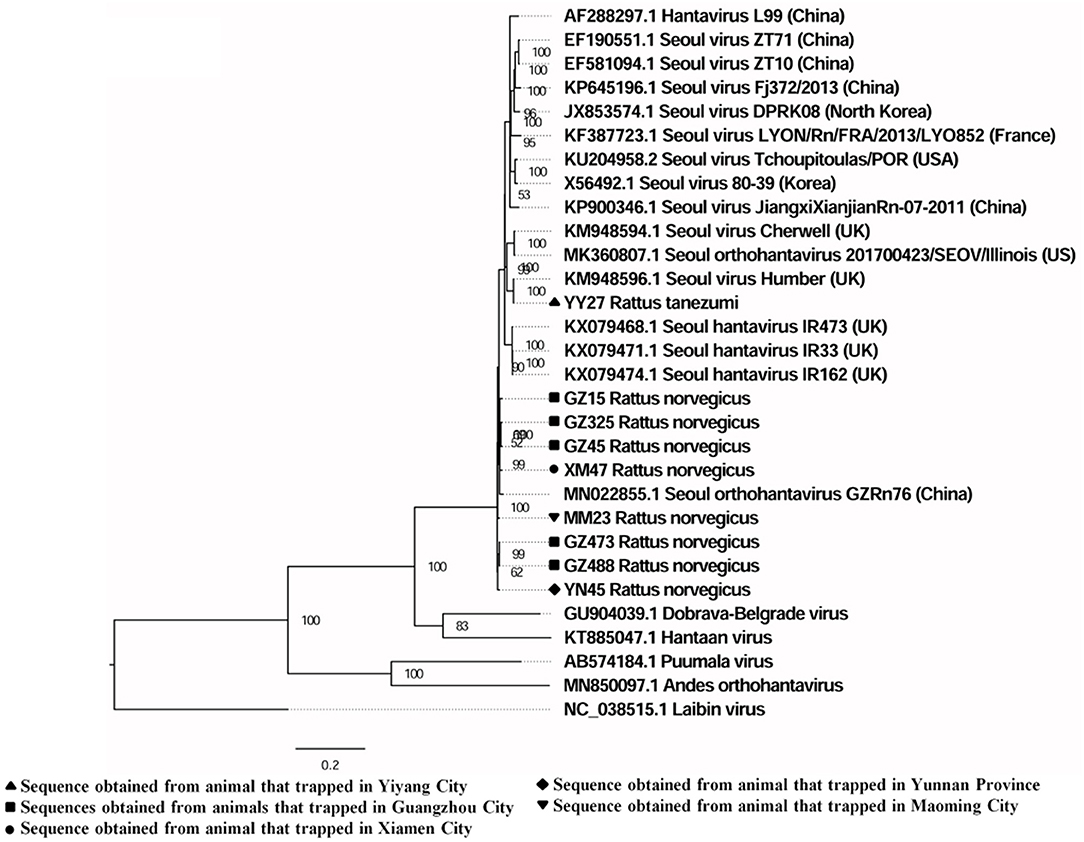
Figure 5. Phylogenetic tree constructed using nucleotide sequences of the Seoul virus L gene obtained from rats. Twenty representative orthohantavirus sequences were included for comparison, and one Laibin virus sequence was set as the outgroup. The percentages of the posterior probability (PP) values are indicated.
Selective Constraints During the Evolution of SEOV
Selective pressure analysis was performed based on the full and partial CDS of the S, M, and L segments. The ω-values of three segments (S, M, and L) varied and all of them were <1 (ω = 0.00997, 0.01846, and 0.01302, respectively), suggesting the negative selective pressure on all of the SEOV genes. The M gene had the largest ω-value (0.01846), indicating a greater selective pressure on this gene. Site model analysis found no amino acid sites with signals of positive selection.
Discussion
Only a few studies have investigated SEOV in liver tissue samples from urban Rattus species rodents in China (27, 28). In this study, SEOV was detected in liver tissue samples from urban R. norvegicus and R. tanezumi in southern China.
A nested-PCR assay that can detect currently known and possible novel members within the genus Orthohantavirus was used for hantavirus screening, and all of the PCR screening sequences detected in our study belonged to SEOV. This is consistent with the results of previous studies, suggesting that SEOV is one of the prevalent hantavirus species in residential habitats in China (29, 30). However, different methods are still needed to confirm the presence of other hantavirus species within the samples.
In this study, SEOV RNA was detected in liver tissue samples from R. norvegicus and R. tanezumi. The presence of SEOV RNA in lung tissue samples from R. norvegicus and R. tanezumi was also reported (15). The above suggests that these animals are natural hosts of SEOV in southern China. The highest detection rate was found in R. norvegicus, which is consistent with the results of previous studies and indicates the predominant role of R. norvegicus in the transmission of SEOV (29, 31).
Detection of SEOV RNA in liver tissue, lung tissue, and serum samples from Rattus species rodents in our study confirms that SEOV has multiple targeted organs in naturally infected reservoir hosts. Previous studies have shown that the lung is the most preferred organ for detecting SEOV in feeder rats and wild R. norvegicus (1, 20). Interestingly, we found that three R. norvegicus with SEOV-positive liver tissue samples had SEOV-negative lung tissue samples, which is inconsistent with the results of previous studies (1, 20). In addition, histopathologic changes associated with SEOV infection have been primarily found in the liver of rats (1). It seems that the liver is one of the targeted organs of SEOV in naturally infected wild Rattus species rodents. Our research group is also investigating SEOV in the lung tissue samples from rats. About 200 lung tissue samples from rats have been investigated (detailed results will be reported in the future). Among these animals, three R. norvegicus with SEOV-positive lung tissue samples had SEOV-negative liver tissue samples, suggesting that the positivity within one tissue sample doesn't always correspond to the positivity in other tissue samples. When investigating SEOV in animals, different organs should be tested to fully understand the prevalence of viral infection and the risk of disease transmission. More studies are needed to investigate the tropism and pathogenesis of SEOV in its naturally infected reservoir hosts.
In this study, sequences from animals trapped in same sampling location usually clustered together, which might be explained by the limited movement of the reservoir hosts (32). Geographic clustering of SEOV has also been found in other studies (33). The full and partial CDS from R. norvegicus and R. tanezumi were highly similar, suggesting the cross-species transmission potential of SEOV between R. norvegicus and R. tanezumi. The partial CDS of the L segment from R. tanezumi obtained in this study was most similar to an L segment detected in R. norvegicus from the United Kingdom (34). Previous studies have reported that the species R. norvegicus probably originated from northern China and migrated to other countries along with human activities (29, 35). These findings suggest that human activities and cross-species transmission potential of SEOV might responsible for the worldwide distribution of SEOV and the similarity between SEOV in different species of reservoir hosts from different countries. However, further study is needed to confirm the cross-species transmission potential of SEOV among rats.
Despite previous studies have showed that the recombination events appear to be common in hantaviruses (15, 36), no recombination event was found in our study. The recombination of SEOV remains to be studied in more large-scale studies. In this study, negative selection is the principal evolutionary force on SEOV, which is consistent with the results of previous studies (33).
There were several limitations in this study. Firstly, we did not identify the age and sex of the animals, which might affect the prevalence of SEOV. Secondly, due a lack of pathological descriptions, we could not confirm the pathogenicity of SEOV in animals. Thirdly, only one R. tanezumi was infected with SEOV in this study, large scale study is needed to investigate SEOV in R. tanezumi and confirm the cross-species transmission potential of SEOV among rats. Fourthly, since we did not collect kidney samples, we were unable to include kidney analyses in this study. In addition, SEOV was only investigated in partial serum samples and the lung tissue samples of individuals with SEOV detection in the liver tissue samples. Further study is needed to investigate the tissue tropism of SEOV in rats.
Conclusions
In this study, SEOV in liver tissue samples from urban Rattus species rodents in China was investigated. In addition, liver was found to be one of the targeted organs of SEOV in naturally infected wild Rattus species rodents, which expands our knowledge of natural SEOV infection in its reservoir hosts. In the future, more rigorous studies will be needed to investigate the tissue tropism of SEOV in its naturally infected reservoir hosts. When monitoring SEOV infection in animals, it is important to include liver tissue samples to provide comprehensive information about the viral prevalence.
Data Availability Statement
The datasets supporting the conclusions of this article are included within the article and its additional files. All PCR screening sequences and full and partial CDS of SEOV are available in the NCBI database (Accession Nos: OL364860-OL364936, and MZ031938-MZ031964).
Ethics Statement
The study protocol was approved by the Animal Ethics and Welfare Committee of the School of Public Health, Southern Medical University and adhered to the guidelines for the Rules for the Implementation of Laboratory Animal Medicine (1998) from the Ministry of Health, China. All surgical procedures were performed under anesthesia in efforts to minimize the suffering of the animals. Endangered or protected species were not included in this study.
Author Contributions
WH and QC conceived of the project and QC obtained the funding. WH and QC contributed to the writing of the paper. WH and JF performed the experiment. WH, YW, and MC analyzed the data. WH, JF, YW, MC, and YM collected the samples. All of the authors have read and approved the manuscript for publication.
Funding
This work was supported by the Key-Area Research and Development Program of Guangdong Province (no. 2018B020241002) and the National Natural Science Foundation of China (no. 81373051). The funders had no role in the study design, data collection and analysis, decision to publish, or preparation of the manuscript.
Conflict of Interest
The authors declare that the research was conducted in the absence of any commercial or financial relationships that could be construed as a potential conflict of interest.
Publisher's Note
All claims expressed in this article are solely those of the authors and do not necessarily represent those of their affiliated organizations, or those of the publisher, the editors and the reviewers. Any product that may be evaluated in this article, or claim that may be made by its manufacturer, is not guaranteed or endorsed by the publisher.
Acknowledgments
We are grateful to Shujuan Ma, Lina Jiang, Wen Zhou, Zejin Ou, Yiquan Xiong, Yongzhi Li, Huan He, Fei Wu, and Xing Li for their participation in the collection of samples.
Supplementary Material
The Supplementary Material for this article can be found online at: https://www.frontiersin.org/articles/10.3389/fvets.2021.748232/full#supplementary-material
Abbreviations
BEB, Bayes Empirical Bayes; CDS, protein-coding sequences; cytB, cytochrome B; HCPS, hantavirus cardiopulmonary syndrome; HFRS, hemorrhagic fever with renal syndrome; HTNV, Hantaan virus; LRTs, likelihood-ratio tests; MCMC, Markov chain Monte Carlo; RdRp, RNA-dependent RNA polymerase; R. norvegicus, Rattus norvegicus; R. tanezumi, Rattus tanezumi; SEOV, Seoul virus; SPSS, Statistical Product and Service Solutions software.
References
1. Maas M, Van Heteren M, De Vries A, Kuiken T, Hoornweg T, Veldhuis Kroeze E, et al. Seoul virus tropism and pathology in naturally infected feeder rats. Viruses. (2019) 11:531. doi: 10.3390/v11060531
2. Plyusnin A, Vapalahti O, Vaheri A. Hantaviruses: genome structure, expression and evolution. J Gen Virol. (1999) 77:2677–87. doi: 10.1099/0022-1317-77-11-2677
3. Zeier M, Handermann M, Bahr U, Rensch B, Müller S, Kehm R, et al. New ecological aspects of hantavirus infection: a change of a paradigm and a challenge of prevention–a review. Virus Genes. (2005) 30:157–80. doi: 10.1007/s11262-004-5625-2
4. Spiropoulou CF, Albariño CG, Ksiazek TG, Rollin PE. Andes and Prospect Hill hantaviruses differ in early induction of interferon although both can downregulate interferon signaling. J Virol. (2007) 81:2769–76. doi: 10.1128/JVI.02402-06
5. Noack D, Goeijenbier M, Reusken C, Koopmans MPG, Rockx BHG. Orthohantavirus pathogenesis and cell tropism. Front Cell Infect Microbiol. (2020) 10:399. doi: 10.3389/fcimb.2020.00399
6. Johansson P, Yap G, Low HT, Siew CC, Kek R, Ng LC, et al. Molecular characterization of two hantavirus strains from different rattus species in Singapore. Virol J. (2010) 7:15. doi: 10.1186/1743-422X-7-15
7. Song G. Epidemiological progresses of hemorrhagic fever with renal syndrome in China. Chin Med J. (1999) 112:472–7.
8. Zhang S, Wang S, Yin W, Liang M, Li J, Zhang Q, et al. Epidemic characteristics of hemorrhagic fever with renal syndrome in China, 2006-2012. BMC Infect Dis. (2014) 11:384. doi: 10.1186/1471-2334-14-384
10. Dearing MD, Dizney L. Ecology of hantavirus in a changing world. Ann N Y Acad Sci. (2010) 1195:99–112. doi: 10.1111/j.1749-6632.2010.05452.x
11. Milholland MT, Castro-Arellano I, Suzán G, Garcia-Peña GE, Lee TE Jr, Rohde RE, et al. Global diversity and distribution of hantaviruses and their hosts. Ecohealth. (2018) 15:163–208. doi: 10.1007/s10393-017-1305-2
12. Wolfe ND, Daszak P, Kilpatrick AM, Burke DS. Bushmeat hunting, deforestation, and prediction of zoonoses emergence. Emerg Infect Dis. (2005) 11:1822–7. doi: 10.3201/eid1112.040789
13. Xiao H, Tong X, Huang R, Gao L, Hu S, Li Y, et al. Landscape and rodent community composition are associated with risk of hemorrhagic fever with renal syndrome in two cities in China, 2006-2013. BMC Infect Dis. (2018) 18:37. doi: 10.1186/s12879-017-2827-5
14. Hansen A, Cameron S, Liu Q, Sun Y, Weinstein P, Williams C, et al. Transmission of haemorrhagic fever with renal syndrome in china and the role of climate factors: a review. Int J Infect Dis. (2015) 33:212–8. doi: 10.1016/j.ijid.2015.02.010
15. Su Q, Chen Y, Li M, Ma J, Wang B, Luo J, et al. Genetic characterization and molecular evolution of urban seoul virus in Southern China. Viruses. (2019) 11:1137. doi: 10.3390/v11121137
16. Bouloy M, Zeller H. [Hantavirus: recent data and review of the literature]. Bull Soc Pathol Exot. (2000) 93:177–80.
17. Murphy EG, Williams NJ, Bennett M, Jennings D, Chantrey J, Mcelhinney LM. Detection of Seoul virus in wild brown rats (Rattus norvegicus) from pig farms in Northern England. Vet Rec. (2019) 184:525. doi: 10.1136/vr.105249
18. Mc ELM, Marston DA, Pounder KC, Goharriz H, Wise EL, Verner-Carlsson J, et al. High prevalence of Seoul hantavirus in a breeding colony of pet rats. Epidemiol Infect. (2017) 145:3115–24. doi: 10.1017/S0950268817001819
19. Madai M, Horváth G, Herczeg R, Somogyi B, Zana B, Földes F, et al. Effectiveness regarding hantavirus detection in rodent tissue samples and urine. Viruses. (2021) 13:570. doi: 10.3390/v13040570
20. Firth C, Bhat M, Firth MA, Williams SH, Frye MJ, Simmonds P, et al. Detection of zoonotic pathogens and characterization of novel viruses carried by commensal Rattus norvegicus in New York City. mBio. (2014) 5:e01933–14. doi: 10.1128/mBio.01933-14
21. Alves VAF. Acute viral hepatitis: beyond A, B, and C. Surg Pathol Clin. (2018) 11:251–66. doi: 10.1016/j.path.2018.02.014
22. Arai S, Bennett SN, Sumibcay L, Cook JA, Song JW, Hope A, et al. Phylogenetically distinct hantaviruses in the masked shrew (Sorex cinereus) and dusky shrew (Sorex monticolus) in the United States. Am J Trop Med And Hyg. (2008) 78:348–51. doi: 10.4269/ajtmh.2008.78.348
23. Klempa B, Fichet-Calvet E, Lecompte E, Auste B, Aniskin V, Meisel H, et al. Hantavirus in African wood mouse, Guinea. Emerg Infect Dis. (2006) 12:838–40. doi: 10.3201/eid1205.051487
24. Ronquist F, Teslenko M, Van Der Mark P, Ayres DL, Darling A, Höhna S, et al. MrBayes 3.2: efficient Bayesian phylogenetic inference and model choice across a large model space. Syst Biol. (2012) 61:539–42. doi: 10.1093/sysbio/sys029
25. Gao F, Chen C, Arab DA, Du Z, He Y, Ho S. EasyCodeML: a visual tool for analysis of selection using CodeML. Ecol Evol. (2019) 9:3891–8. doi: 10.1002/ece3.5015
26. Yang Z. PAML 4: phylogenetic analysis by maximum likelihood. (2007) 24:1586–91. doi: 10.1093/molbev/msm088
27. Kim WK, No JS, Lee SH, Song DH, Lee D, Kim JA, et al. Multiplex PCR-Based next-generation sequencing and global diversity of seoul virus in humans and rats. Emerg Infect Dis. (2018) 24:249–57. doi: 10.3201/eid2402.171216
28. Wang B, Cai CL, Li B, Zhang W, Zhu Y, Chen WH, et al. Detection and characterization of three zoonotic viruses in wild rodents and shrews from Shenzhen city, China. Virol Sin. (2017) 32:290–7. doi: 10.1007/s12250-017-3973-z
29. Lin XD, Guo WP, Wang W, Zou Y, Hao ZY, Zhou DJ, et al. Migration of Norway rats resulted in the worldwide distribution of Seoul hantavirus today. J Virol. (2012) 86:972–81. doi: 10.1128/JVI.00725-11
30. Zhang YZ, Zou Y, Fu ZF, Plyusnin A. Hantavirus infections in humans and animals, China. Emerg Infect Dis. (2010) 16:1195–203. doi: 10.3201/eid1608.090470
31. Gravinatti ML, Barbosa CM, Soares RM, Gregori F. Synanthropic rodents as virus reservoirs and transmitters. Rev Soc Bras Med Trop. (2020) 53:e20190486. doi: 10.1590/0037-8682-0486-2019
32. Jameson LJ, Newton A, Coole L, Newman EN, Carroll MW, Beeching NJ, et al. Prevalence of antibodies against hantaviruses in serum and saliva of adults living or working on farms in Yorkshire, United Kingdom. Viruses. (2014) 6:524–34. doi: 10.3390/v6020524
33. Zuo SQ, Li XJ, Wang ZQ, Jiang JF, Fang LQ, Zhang WH, et al. Genetic diversity and the spatio-temporal analyses of hantaviruses in Shandong Province, China. Front Microbiol. (2018) 9:2771. doi: 10.3389/fmicb.2018.02771
34. Jameson LJ, Logue CH, Atkinson B, Baker N, Galbraith SE, Carroll MW, et al. The continued emergence of hantaviruses: isolation of a Seoul virus implicated in human disease, United Kingdom, October 2012. Euro Surveill. (2013) 18:4–7. doi: 10.2807/ese.18.01.20344-en
35. Puckett EE, Orton D, Munshi-South J. Commensal rats and humans: integrating rodent phylogeography and zooarchaeology to highlight connections between human societies. Bioessays. (2020) 42:e1900160. doi: 10.1002/bies.201900160
Keywords: liver, rats, Seoul virus, prevalence, genetic diversity
Citation: He W, Fu J, Wen Y, Cheng M, Mo Y and Chen Q (2021) Detection and Genetic Characterization of Seoul Virus in Liver Tissue Samples From Rattus norvegicus and Rattus tanezumi in Urban Areas of Southern China. Front. Vet. Sci. 8:748232. doi: 10.3389/fvets.2021.748232
Received: 29 September 2021; Accepted: 17 November 2021;
Published: 13 December 2021.
Edited by:
Filippo Biscarini, National Research Council (CNR), ItalyReviewed by:
Maria Luisa Marenzoni, University of Perugia, ItalyBarbara Lazzari, PTP Science Park, Italy
Copyright © 2021 He, Fu, Wen, Cheng, Mo and Chen. This is an open-access article distributed under the terms of the Creative Commons Attribution License (CC BY). The use, distribution or reproduction in other forums is permitted, provided the original author(s) and the copyright owner(s) are credited and that the original publication in this journal is cited, in accordance with accepted academic practice. No use, distribution or reproduction is permitted which does not comply with these terms.
*Correspondence: Qing Chen, MTgwMDIyNzAzMDgmI3gwMDA0MDsxNjMuY29t