- 1Environmental Sciences Graduate Program, Oregon State University, Corvallis, OR, United States
- 2Department of Fisheries, Wildlife and Conservation Sciences, Oregon State University, Corvallis, OR, United States
- 3Department of Integrative Biology, Oregon State University, Corvallis, OR, United States
Emerging infectious diseases are one of the multiple factors contributing to the current “biodiversity crisis”. As part of the worldwide biodiversity crisis, amphibian populations are declining globally. Chytridiomycosis, an emerging infectious disease, caused by the fungal pathogen Batrachochytrium dendrobatidis (Bd), is a major cause of amphibian population declines. This fungus primarily affects keratinized structures in larval, juvenile, and adult amphibians as well as heart function. However, we know little about how Bd can impact embryos as well as potential latent effects of Bd exposure over ontogeny. Using two different Bd strains and multiple exposure times, we examined the effects of Bd exposure in Pacific chorus frog (Pseudacris regilla), Western toad (Anaxyrus boreas) and American bullfrog (Lithobates catesbeianus) life stages. Using a factorial experimental design, embryos of these three species were exposed to Bd at early and late embryonic stages, with some individuals re-exposed after hatching. Embryonic Bd exposure resulted in differential survival as a function of host species, Bd strain and timing of exposure. P. regilla experienced embryonic mortality when exposed during later developmental stages to one Bd strain. There were no differences across the treatments in embryonic mortality of A. boreas and embryonic mortality of L. catesbeianus occurred in all Bd exposure treatments. We detected latent effects in A. boreas and L. catesbeianus larvae, as mortality increased when individuals had been exposed to any of the Bd strains during the embryonic stage. We also detected direct effects on larval mortality in all three anuran species as a function of Bd strain, and when individuals were double exposed (late in the embryonic stage and again as larvae). Our results suggest that exposure to Bd can directly affect embryo survival and has direct and latent effects on larvae survival of both native and invasive species. However, these impacts were highly context dependent, with timing of exposure and Bd strain influencing the severity of the effects.
Introduction
In many organisms, exposure to stressors during embryonic or prenatal stages can result in both direct and latent effects on subsequent developmental stages. For example, in birds, conditions experienced at early life are determinants of fitness in adults (1); in snapping turtles (Chelydra serpentina), hatchling from eggs incubated on a wet substrate have an improved locomotor performance in comparison to hatchlings from drier substrates (2) and in fishes, embryos of pink salmon (Oncorhynchus gorbuscha) exposed to oil have a reduction in juvenile growth and survival (3). In amphibians, these effects can be on individual growth rates, behavior, locomotion or immunology (4–7). Thus, exposure to predator cues in the pinewoods tree frog Hyla femoralis slowed larval growth and development, resulting in metamorphs with relatively smaller body sizes (8). In amphibians, repeated exposure at early life stages to other environmental stressors, such as contaminants, predator cues, and pathogens, can produce latent effects in juvenile and adult amphibians (4, 9, 10). Particularly in amphibian embryos, environmental cues can cause significant changes in hatching traits (11–14). Time of hatching can change in response to risks and opportunities. For example, embryos can hatch early to escape predators and pathogens, and this life history shift can have effects that persist through later life stages (15–17). Additionally, embryos infected with water molds can suffer differential mortality rates relative to the timing of exposure to the pathogen (18). As such, the timing of pathogen exposure might play a critical role on host susceptibility to infection (19).
Host ontogeny is a key factor for examining or predicting disease dynamics. There is precedence in different systems than minimum changes in the history of exposure to pathogens during a particular developmental stage can drastically change host life history. Changes in individual susceptibility to pathogens occur throughout ontogeny in many organisms, including plants (20), insects (21), birds (22), reptiles (23), mammals (24) and amphibians (25, 26). The key, however, to understanding temporal association between pathogens and susceptibility is to empirically discern latent and direct effects within and across life history stages. We posit that amphibians can be model systems for testing these questions as they are a taxon of conservation concern, have complex life histories, and are susceptible to multiple emerging infectious diseases.
One of the most researched amphibian pathogens is the fungus Batrachochytrium dendrobatidis (Bd), which has been implicated in the decline of numerous amphibian species worldwide (27–29). Differential susceptibility to Bd has been documented across species (30–35), populations (36, 37), life stages (38–42), and Bd strains (29, 33, 43–46). However, how exposure to Bd in one developmental stage can produce latent affects in a later life stage is unclear. Information regarding direct Bd impacts on embryos is also lacking as Bd mainly affects keratinized structures, which are absent in embryos. Further, the importance of evolutionary relationships between Bd strain and the embryonic host may also have significant implications.
We explored the direct and latent effects of Bd exposure on both the embryonic and larval stages using three amphibian species with differential susceptibility to native and invasive Bd strains (33, 34, 46, 47). We posit that amphibian embryos will be susceptible to the chytrid fungus as Bd can produce enzymes that can destroy tissue (48–50). Further, the release of fungal toxin (30, 51) could impact embryos by delaying growth, triggering key transitions resulting in ontogenetic shifts or latent effects on life history trajectories. Finally, we hypothesized that Bd can impact embryos by depletion of oxygen. The lack of dissolved oxygen slows down the development in Bufo bufo (52) and hypoxia can kill early life stages (13). Direct or latent effects may also vary with Bd strain and with host species, therefore evaluating different strains is critical to disentangle intrinsic aspects of the pathogen as virulence and how it changes among hosts. We also examined the influence of Bd exposure on larval survival predicting that repeated exposure to Bd across the embryonic/larval transition would result in decreased survival.
Materials and Methods
We studied three anuran species found in the US Pacific Northwest (PNW). These are the Pacific chorus frog (Pseudacris regilla) a common species throughout its PNW range found in a variety of habitats from sea level to montane regions, the Western toad (Anaxyrus boreas) whose populations have experienced declines across much of its historic range and American bullfrogs (Lithobates catesbeianus) an introduced species in the PNW (53–55). Twenty clutches of P. regilla were collected from Little Three creeks on 19 June 2014 (44°06′03.5″ N, 121°38′34.7″ WGS84 Deschutes County, OR, elevation = 2,000 m) and 600 eggs of A. boreas were collected from 20 different egg masses at Todd Lake (44°01′44.5″ N, 121°41′07.6″W WGS84 Deschutes County, OR, elevation = 1,870 m) on 29 May 2015. We collected 600 newly laid eggs from six distinct L. catesbeianus egg masses from William L. Finley National Wildlife Refuge on 20 May 2014 (44°25′23.6″ N, 123°18′41.8″W WGS84 Benton County, OR, elevation = 276 m). After collection, eggs were immediately transported to a climate-controlled environment at Oregon State University and held under constant temperature (14°–15.5°C) and photoperiod (12L: 12D) conditions. Less than 6 h after arrival, every clutch of P. regilla or group of eggs of A. boreas and L. catesbeianus were divided into three groups and each group for P. regilla and A. boreas contained ~10 eggs (±1.95 eggs), and 20 eggs for L. catesbeianus.
Pre-hatch Exposure Regime
Bd exposure treatments were administered in either the early embryonic developmental stages or closer to hatching. Early exposure (early) corresponded to the late gastrula stages, or Gosner Developmental Stage 12 (56) while closer to hatching exposure (late) corresponded to embryos capable of muscular response, or Gosner Developmental Stage 18 (56). Bd strains (i.e., the isolate of the fungus used for the inoculation) included an endemic Bd strain (JEL 630, hereafter “West,” isolated from L. catesbeianus in Oregon), and a novel Bd strain to Oregon freshwater habitats (JEL 627, hereafter “East,” isolated from L. catesbeianus in Maine USA). These strains were identified as part of the North American clade (Bd-GPL-1) (57). We obtained cryogenically preserved culture plates from J. Longcore to prepare 1% sterile tryptone—agar media plates with 0.5 ml of stock Bd broth coming from each particular strain of the fungus (31). Bd cultures were allowed to grow for 5–8 days at 20°C before used in the experiment (31).
Using a hemocytometer, we quantified the zoospores from a pooled inoculation broth (8–12 plates per Bd strain). Five ml inoculations of the zoospore broth (30 K zoospores/ml) were then administered to experimental units (18 cm H × 10 cm OD high-density polyethylene graduated beakers) containing 800 ml of dechlorinated water. A similar dose was previously tested in larvae of P. regilla (47), A. boreas (33, 58, 59), and L. catesbeianus (32, 60). Controls were inoculated with a sham inoculum created by rinsing the same number of sterile agar plates with 5 ml of dechlorinated water.
Using a factorial experimental design, each group of eggs was assigned to a time of exposure treatment (early, late) and a Bd strain treatment (West, East, Control) (Figure 1, Pre-Hatching). Sixty experimental units (581 total eggs) were assigned for P. regilla (10 replicates per Early and Late treatment groups), 51 experimental units (506 total eggs) for A. boreas (8 replicates per Early exposure treatment, 9 replicates per Late exposure treatment), and 30 experimental units (600 total eggs) for L. catesbeianus (5 replicates for all groups, except for the East and West/Late exposure groups, which had 6 and 4 replicates, respectively) (Table 1). The length of the pre-hatching phase varied by species, lasting 19 days for P. regilla and A. boreas, and 22 days for L. catesbeianus. Embryos that died were preserved individually in 2.0 ml Eppendorf tubes with 95 % ethanol. No water changes were performed during the pre-hatching phase as movement associated with water changes can induce hatching, thus influencing our results. Upon hatching at Gosner stage 21, water changes were conducted weekly. We quantified the time of hatching by direct observation, and hatchling events and survival were recorded twice per day.
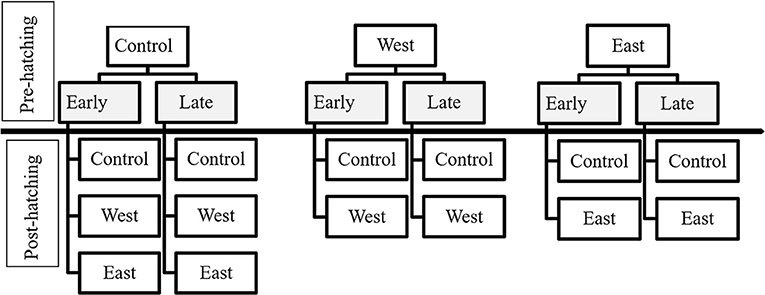
Figure 1. Exposure treatments of egg masses and tadpoles according to the time of exposure and chytrid strain. Pre-hatching treatments are indicated in gray and above the separation line. Treatments for tadpoles (post-hatching) are listed below the separation line.
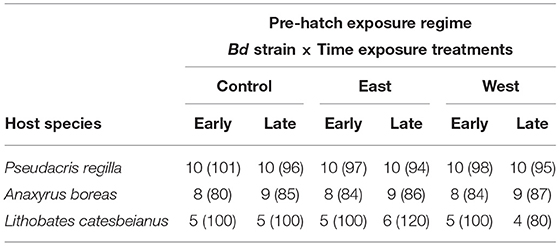
Table 1. Number of replicates per treatment per species followed by total number of eggs per treatment between parentheses.
To analyze differences in hatching time, we compared proportions between treatments of eggs being exposed to Bd and control treatments (no exposure to the pathogen) using quasibinomial generalized linear models (GLM) performed independently per species. All analyses were run in R (61). To evaluate differences among strains and controls we calculated pairwise comparison using a Tukey HSD test.
Post-hatch Exposure Regime
Upon hatching, survivors were pooled within pre-hatch treatment groups (Early or Late; East, West, Control) to standardize sample sizes for the post-hatch phase of the experiment. In this phase, larvae were either re-exposed to the same pre-hatch Bd strain or held as controls to estimate latent effects (Figure 1, Post-Hatch). For P. regilla, we had a total of 328 surviving hatchlings distributed across the larval exposure treatments for a total of 82 experimental units, resulting in 33 control replicates, 21 East strain replicates, and 28 West strain replicates. Less than 10% of eggs hatched from the East/Late pre-hatch exposure treatment group; as such, there was no continuation of this treatment in the post-hatch phase. For A. boreas, we ran 42 control replicates, 28 East strain replicates, and 26 West strain that contained a total of 384 surviving hatchlings for a total of 96 experimental units. For L. catesbeianus, we ran 23 control replicates, 17 East strain replicates, and 16 West strain using a total of 228 surviving hatchlings with a total of 56 experimental units. Due to complete mortality in the East/Early and the West/Early pre-hatch phase, these treatments were not continued in the post-hatch phase (Table 2).
Larvae were held individually and those that were re-exposed to Bd were re-inoculated once a week (every 7 d) for the duration of the experiment. Individuals were held in rectangular plastic containers (31 × 18 × 8 cm) filled with 2,000 ml dechlorinated water. Water changes occurred concurrently with re-inoculation using 5 ml of 50 K zoospores/ml. Animals that died during the experiment were preserved in 95% ethanol. At the end of the experiment, animals remaining alive were humanely euthanized in accordance with institutional animal care protocol in MS-222 (Tricaine methanesulfonate) and then preserved in 95% ethanol. The experimental trials for each species lasted until individuals reached Gosner stage 30–31 (distinctive rear limb bud) or death. Total duration for the experiment was 65 days for P. regilla, 59 days for A. boreas and 19 days for L. catesbeianus.
We monitored survival twice per day and quantified developmental differences through time by staging all larvae (Gosner stage) every week during water changes. At the end of the post-hatch phase, we sampled a subset of all Bd-exposed animals of each species and also randomly sampled 5 control animals of each species to confirm no contamination happened. To assess infection load at the termination of the experiment, we dissected larvae mouthparts for P. regilla individuals, and we swabbed mouthparts using fine tipped sterile rayon swabs (Medical Wire and Equipment MW&E 113) for A. boreas and L. catesbeianus. Both protocols, swabbing and cutting mouthparts, are recommended as adequate protocols for assessing infection loads. Excised mouthparts and swabbing are similar in the likelihood of detecting Bd infection regardless of developmental stage and larval size (62–64).
Each sample was analyzed using quantitative polymerase chain reaction (qPCR) following the methods of (65). A small modification of the amount of Prepman Ultra (Applied Biosystems®, Life Technologies) was used to extract the DNA; we used 60 μL instead of 40 μL (66). Our extractions were diluted 1:10 and each sample was analyzed in triplicate to quantify the average number of genome equivalents per animal (7,500 real-time PCR Applied Biosystems instrument). To analyze infection loads, we log transformed the qPCR results as log (genome equivalents per individual + 1) to normalize data.
Effect of exposure on survivorship was analyzed independently by species using odds ratios calculated with a generalized linear mixed model, family: binomial (logit). The values of the ratios represent the likelihood or the risk of mortality due to exposure to the pathogen in comparison to the controls. Therefore, odds ratios higher than 1 represent an increased risk after exposure, odds ratios equal to one represent no difference in the risk, and odds ratios lower than 1 represent a lower risk of the exposed group. All analyses were run in R (version 4.0.3).
Results
Pre-hatching Phase
Pseudacris regilla embryos exposed to both the East and West Bd strains in the Early exposure groups had a lower proportion of hatchlings relative to controls (East strain: t = −4.40, p < 0.001; West strain: t = 1.99, p = 0.04). A post hoc Tukey test showed that this proportion was different in embryos exposed to the East strain in contrast to the control (z = −4.45, p < 0.001) and the West strain (z = 6.14, p < 0.001), with approximately a 50% hatching rate (Figure 2A). Reduced hatching was also found in the Late/East treatment group (t = −11.03, p < 0.001) relative to the Late/West treatment (t = −1.29, p = 0.19). In fact, <10% of embryos hatched after being exposed late in development to the non-native East strain (Figure 2B). In A. boreas, the proportion of embryos that hatched was similar across both strains in comparison to controls across the Early (East strain: t = –0.49, p = 0.62; West strain: t = 0.62, p = 0.53) (Figure 2C) and Late exposure treatments (East strain: t = 1.31, p = 0.19; West strain: t = 0.73, p = 0.46) (Figure 2D).
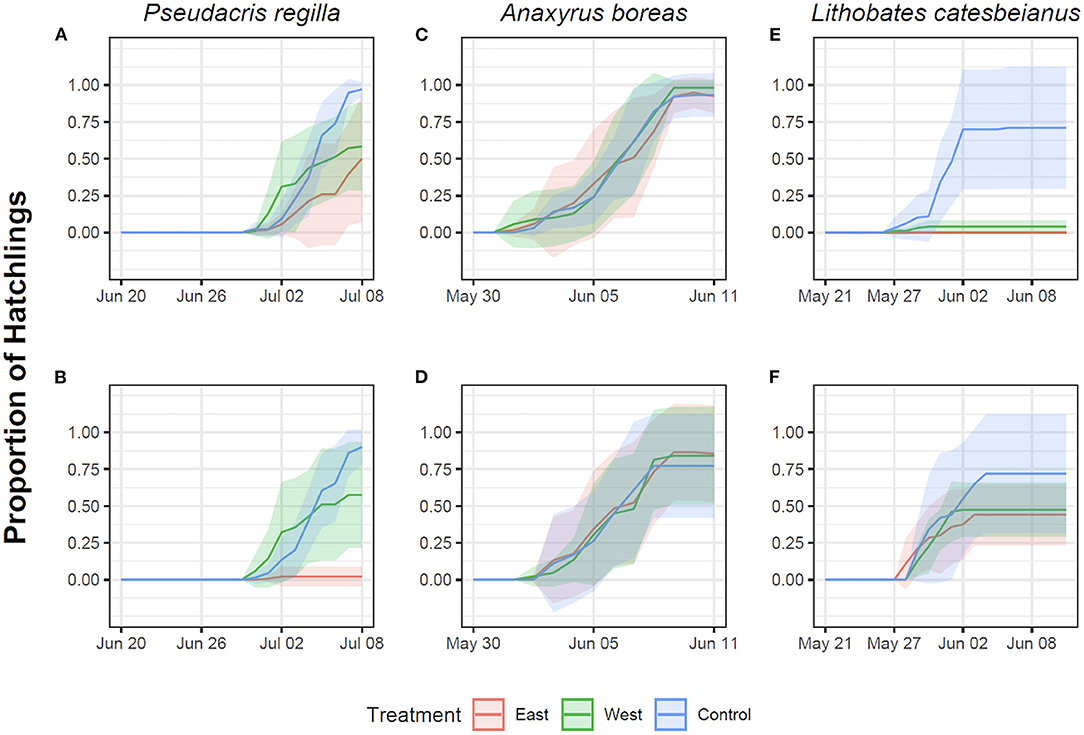
Figure 2. Proportion of hatchlings in Pseudacris regilla, Anaxyrus boreas, and Lithobates catesbeianus after exposure of eggs to different chytrid treatments. Red represents exposure to the East strain, green represents exposure to the West strain, and controls are indicated in blue. First row represents Early exposure (Gosner stage 12) for Pseudacris regilla (A), Anaxyrus boreas (C) and Lithobates catesbeianus (E). Second row represents Late exposure (Gosner stage 18) for Pseudacris regilla (B), Anaxyrus boreas (D) and Lithobates catesbeianus (F).
The proportion of L. catesbeianus embryos that hatched was low when embryos were exposed early in development, with lower survival in the West Bd strain treatment relative to controls (West strain: t = 3.58, p < 0.001) (Figure 2E). There were no survivors in the East strain exposure treatment. The estimate of Bd strain as a factor in our model was high (5,329), potentially due to the 100% mortality, making the t and p-value not significant (t = 0.003, p = 0.99). The proportion of embryos that hatched in the Late exposure treatment was lower across both Bd strains in comparison to the controls (East strain: t = 2.89, p < 0.01; West strain: t = 2.13, p = 0.03) (Figure 2F). A post hoc Tukey test showed that this proportion was different in embryos exposed to the East strain in contrast to the control (z = 2.89, p < 0.01), but it was not different for embryos exposed to the West strain (z = 2.13, p = 0.08).
Post-hatching Phase
Our generalized linear mixed model quantified as odds ratios (OR) the effects of exposure to a particular strain on larvae mortality in comparison to the controls given their history of exposure as embryos. As such, results are reported as an increase or decrease in odds of mortality.
Direct Effects on Larvae -Only Exposed to Bd as Larvae (Control-Bd)
We found evidence for direct effects of Bd exposure on larval mortality for the three species. In P. regilla, post-hatching exposure to the East strain increased the odds of mortality (OR Early/Control−East 8.88, p = 0.01, CI. 1.43–54.85, Figure 3, Left panel). For A. boreas, we found that individuals exposed during the post-hatch phase to the West strain had lower odds of mortality relative to controls (OR Early/Control−West 0.12, p = 0.03, CI: 0.018–0.84, Figure 4, Left panel). In contrast, larvae coming from the Late control group and exposed post-hatch to East or West had higher odds of mortality than controls (OR Late/Control−East 14.38, p = 0.03, CI: 1.19–173.65; OR Late/Control−West 19.56, p = 0.03, CI: 1.32–288, Figure 4, Right panel). Larvae of L. catesbeianus increased their odds of mortality when exposed to either East or West strain (OR East 9.9, p = 0.04, CI: 1.06–92; OR West 539, p < 0.001, CI: 29.64–9,801) (Figure 5).
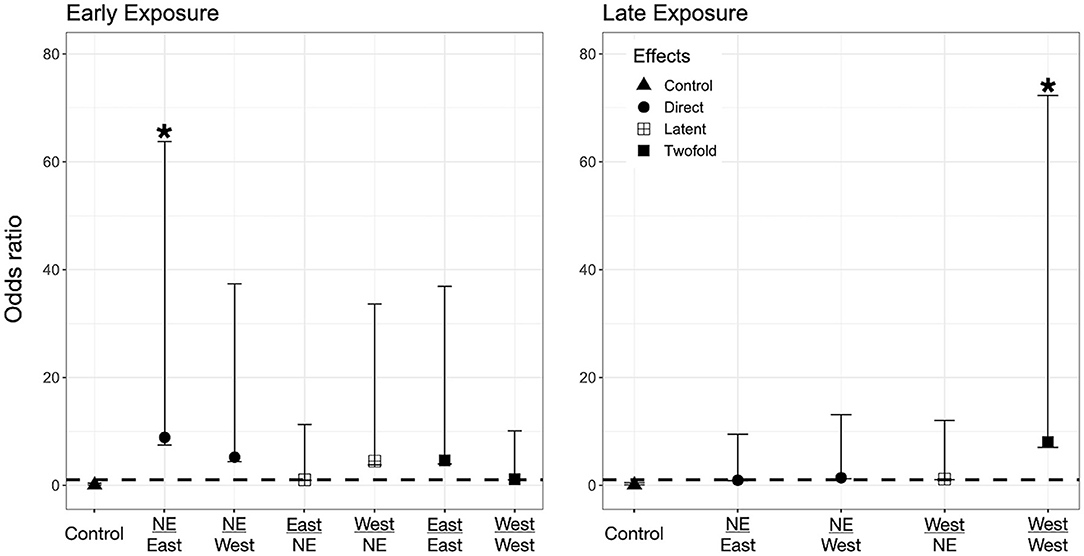
Figure 3. Odds ratio (OR) for P. regilla tadpoles according to their exposure regimes. Label of x-axis includes a fraction that indicates in the numerator the exposure regime as embryo and in the denominator exposure regime post-hatching (no exposure = NE, East or West for Bd chytrid strain). A dashed line indicates value 1. OR > 1 higher risk after exposure, OR = 1 no risk difference, OR < 1 lower risk after exposure. A star (*) indicates treatments with significant odds ratios. Effects are represented as follows: Direct effects –only exposed to Bd as larvae– are represented by circles, latent effects –only exposed as embryos– are represented as four squares, and 2-fold effects –exposed as embryos and larvae– are represented with filled squares; controls are represented by triangles.
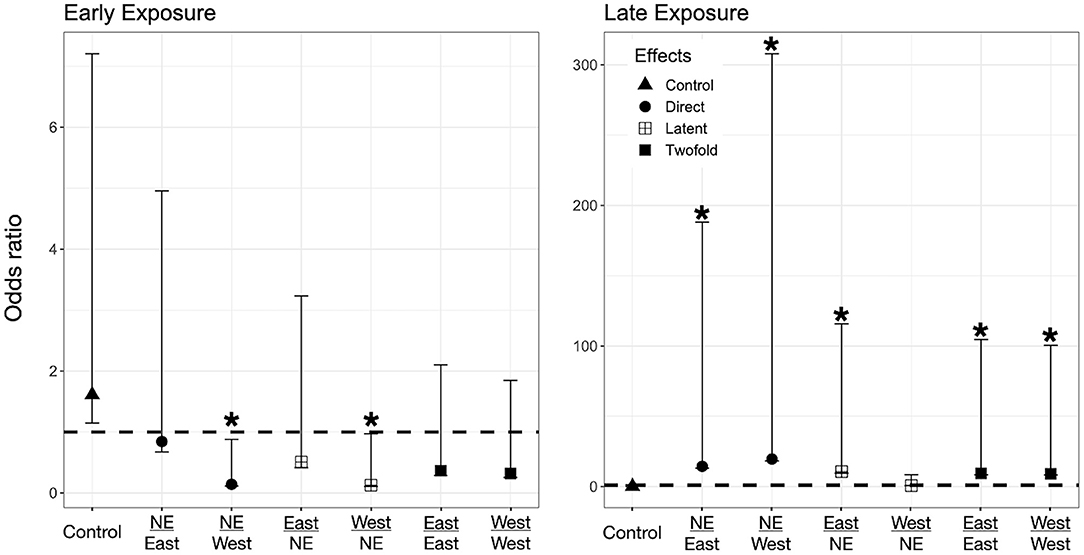
Figure 4. Odds ratio (OR) for A. boreas tadpoles according to their exposure regimes. Label of x-axis includes a fraction that indicates in the numerator the exposure regime as embryo and in the denominator exposure regime post-hatching (no exposure = NE, East or West for Bd chytrid strain). A dashed line indicates value 1. OR > 1 higher risk after exposure, OR = 1 no risk difference, OR < 1 lower risk after exposure. A star (*) indicates treatments with significant odds ratios. Effects are represented as follow: Direct effects –only exposed to Bd as larvae– are represented by circles, latent effects –only exposed as embryos– are represented as four squares, and 2-fold effects –exposed as embryos and larvae– are represented with filled squares; controls are represented by triangles.
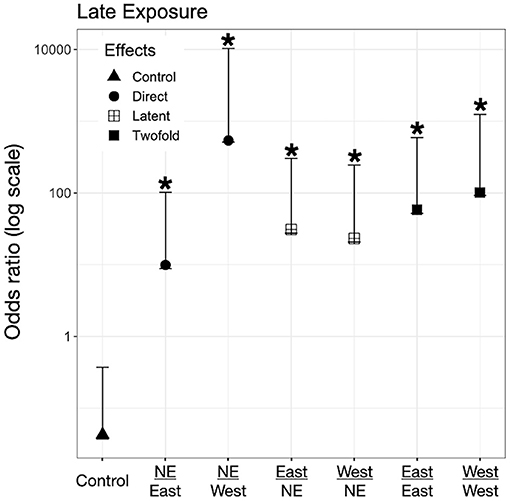
Figure 5. Odds ratio (OR) for L. catesbeianus tadpoles according to their exposure regimes. Label of x-axis includes a fraction that indicates in the numerator the exposure regime as embryo and in the denominator exposure regime post-hatching. A star (*) indicates treatments with significant odds ratios. Effects are represented as follow: Direct effects –only exposed to Bd as larvae– are represented by circles, latent effects –only exposed as embryos– are represented as four squares, and 2-fold effects –exposed as embryos and larvae– are represented with filled squares; controls are represented by triangles.
Latent Effects on Larvae -Only Exposed to Bd as Embryos (Bd-Control)
We did not find evidence for latent effects in P. regilla. In A. boreas odds of mortality changed according to the time of exposure and Bd strain. Odds of mortality for larvae decreased when embryos were exposed early in development to the West strain of Bd (OR Early/West−control 0.14, p = 0.02, CI: 0.026–0.73, Figure 4, Left panel). On the contrary, individuals exposed Late as embryos to the East strain had higher odds of mortality than controls (OR Late/East−control 10.62, p = 0.04, CI: 1.07–105, Figure 4, Right panel). In L. catesbeianus, we found higher odds of mortality than controls for both Bd strains (OR Late/East−control 31, p = 0.001, CI: 3.5–272, OR Late/West−control 23.21, p = 0.006, CI: 2.42–222.14, Figure 5).
Double Exposed Treatments -Exposed to Bd as Both Embryos and Larvae (Bd-Bd)
We found evidence that exposure to Bd in both the embryonic and larval stages affect the larval odds of mortality in all three species. We found P. regilla that were re-exposed to the West strain, (Late exposure group) increased the odds of mortality (OR Late/West−West 8.05, p = 0.04, CI 1.01–64.22, Figure 3, Right panel). In A. boreas odds of mortality increased in re-exposed individuals to both the East and West strains (Late exposure groups) (OR Late/East−East 9.37, p = 0.05, CI: 0.92–95; OR Late/West−West 9.12, p = 0.05, CI: 0.91–91.32) (Figure 4, Right panel). In L. catesbeianus, odds of mortality were high for re-exposed animals to either Bd strain (OR East/Late−East 58.3, p = 0.0003, CI: 6.35–534.6, OR West/Late−West 101.29, p < 0.001, CI: 8.9–1,145) (Figure 5).
Infection Loads
We confirmed Bd infection using real-time PCR analyses of tadpole mouthparts for P. regilla and swabs for A. boreas and L. catesbeianus. All tadpoles from control treatments tested negative for Bd. Individuals from the three species only exposed as larvae (direct effects) were positive for the East strain. From individuals only exposed as embryos (latent effects), we found that A. boreas tested positive for Bd when exposed late as embryos with the East strain. In L. catesbeianus, individuals tested positive when exposed late to either of the strains (Table 3). In the group of re-exposed individuals (2-fold effects), P. regilla was reported Bd positive for both East and West strain when exposed early as embryos. In A. boreas, individuals were positive for Bd when exposed early to the West strain and late when exposed to the East strain (Table 3).
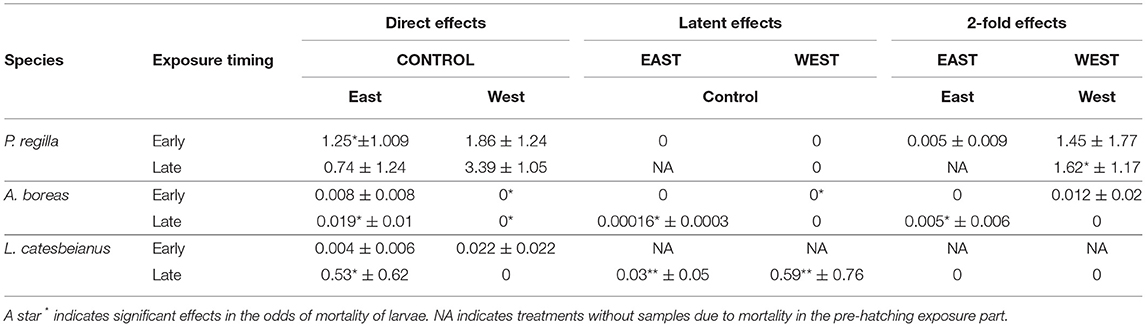
Table 3. Mean Bd loads (genome equivalents ± SD) at the end of experiment for P. regilla, A. boreas and L. catesbeianus exposed early (Gosner developmental stage 12) and late (Gosner developmental stage 18) during embryonic development.
Discussion
Life stage, time of exposure, and Bd strain influenced susceptibility to Bd in the embryo-larvae life history transition for three anuran species: P. regilla, A. boreas, and L. catesbeianus. We detected direct effects of Bd on embryonic and larval mortality, latent effects across the embryo/larval transition, and additive effects when double-exposed to Bd across both life stages. Exposure of embryos to Bd resulted in direct impacts on hatchling survivorship. We found direct, negative impacts of Bd strain and time of exposure on embryonic survival and proportion of hatching success for P. regilla and L. catesbeianus. Embryos of P. regilla were drastically affected by the non-native East Bd strain, resulting in more than 90% mortality when exposed later in embryonic development. Interestingly, embryos of invasive L. catesbeianus died when exposed to either Bd strain (East or West). When exposed early in embryonic development to the East strain, the number of viable hatchlings was zero and we detected a mortality of 90% in hatchlings after early exposure to the West Bd strain. When exposed later in development (East or West strains), only 50% of embryos hatched. The influence of time of exposure may be explained by changes in the thickness of the jelly layer surrounding the embryo through development. This jelly layer becomes thinner over development, thus late-stage embryos may be more affected by exposure to a pathogen (15, 18). Amphibian species-specific egg deposition forms (films, strings, clusters), as well as morphology of egg structure, provide differential protection from pathogens (67).
Post-hatching exposure resulted in both direct and latent impacts on larval survivorship. Direct effects on larvae are reported mainly as an increase in the odds of mortality for all three anuran species. P. regilla was negatively affected by exposure to the non-native East Bd strain, while A. boreas and L. catesbeianus were affected by both strains (East and West). Odds of mortality in A. boreas were higher when exposed to the West strain (19.56) than when exposed to the East strain (14.38). On the contrary, the odds of mortality in L. catesbeianus were higher when exposed to the East strain (9.9) than when exposed to the West strain (5.39). This result was not wholly unexpected as larvae mortality has been reported in experimental studies exposing these same species to Bd. A. boreas has been identified as particularly susceptible to Bd (30, 46) while P. regilla and L. catesbeianus larvae have relatively high survivorship (30, 33, 46, 68). In this study, we found a direct effect of Bd on larval survivorship for all three species. The increase in the odds of mortality in P. regilla and L. catesbeianus larvae can be explained by the origin and characteristics of the East strain. Isolated from L. catesbeianus in Maine (USA), this strain has been identified as hypervirulent (57, 69, 70) and categorized as part of the North American clade in the Global Pandemic Lineage (GPL) (57). As such, we anticipated an increase in larval mortality due to a lack of evolutionary relationship with this strain. However, L. catesbeianus larvae were also susceptible to the East strain even though it was isolated from their conspecifics within their native range.
We detected an increase in the odds of mortality in P. regilla when exposed early in embryonic development to Bd while the odds ratio for larval mortality were lower for later exposure. We could not evaluate potential latent effects after late exposure of P. regilla embryos to the East strain. Effects of Bd differed in embryos according to the timing of exposure. However, these effects of timing of exposure and duration of stressors such as pathogens and its relation to critical window and disease is not broadly tested (71, 72). We found an increase in the odds of larval mortality of both A. boreas and L. catesbeianus as a function of Bd strain and timing of embryonic exposure. In A. boreas, embryos exposed early to the West strain showed a decrease in the odds of mortality. Conversely, when A. boreas were exposed to the East strain late in embryonic development, larvae were almost 10 times more likely to die than control individuals. There was a similar increase in the odds of larval mortality in L. catesbeianus when exposed as embryos to any of the Bd strains. The high mortality rates in L. catesbeianus when exposed early to Bd prevented us from understanding potential latent effects for this invasive species. Our results support the hypothesis that timing of pathogen exposure is a major factor that influences host survivorship. Exposures of amphibian embryos to stressors such as pathogens at early stages of development can trigger effects over ontogeny (73). This exposure can later modify other characteristics, such as growth rates (74), mortality rates (75), mass (5), and development of immune response (76).
We also found effects of double Bd exposure (exposed in both the embryonic and larval stages) in all three anuran species. All species showed an increase in the odds of larval mortality when the first Bd exposure occurred at a later embryonic developmental stage (Gosner stage 18). In P. regilla, odds of mortality increased after double exposure to the West strain. Exposure to either strain (East or West) increased the odds of mortality in A. boreas and L. catesbeianus. Double exposure effects have been reported in experiments examining the larval/metamorph transition (77), thus our experiment provides additional information concerning other life history transitions providing a comprehensive view of the jeopardy through life.
The differential response of A. boreas to Early/Late and East/West Bd treatments may be explained by the presence of a potential critical window of vulnerability for this species and by the virulence of the Bd strain. Late exposure of A. boreas embryos to the East strain increased the odds of larval mortality of this species. Fernandez-Beneitez et al. (18) found that embryos of toads (Bufo calamita and Pelobates cultripes) exposed to Saprolegnia spp. at an early developmental stage (Gosner stage 12) suffered no increase in mortality, while embryos challenged at later stages of embryonic development (Gosner stages 15 and 19) were sensitive to the pathogen dying 72 h after exposure. Understanding which species experience latent effects will help target management efforts by identifying how exposure in particular life history stages can change host response. If a species is identified as being particularly susceptible to exposure to Bd as embryos, actions such as ex situ protection could be useful for its conservation.
Our findings complement the information on susceptibility of P. regilla to Bd as larvae of this species had previously been reported to be tolerant to certain Bd strains (30, 46). Interestingly, we found that this tolerance can change with an individual's previous exposure regime to non-native strains. Our experimental evaluation revealed that Bd strains from an invasive species can have harmful consequences on native and even invasive conspecific hosts. Our findings for A. boreas support previous work showing species as being susceptible to both the East and West strains of Bd (30, 46, 47). In L. catesbeianus, larvae and adults have been reported as able to withstand infection loads of the chytrid in different regions (78) and this species is suggested as an asymptomatic carrier or reservoir of Bd (79, 80). Our results indicate that larvae can also be susceptible to Bd but this response will be mediated by previous exposure in an early life stage. Individuals that were re-exposed to Bd were about 50 times more likely to die than individuals kept as controls. This contrasts with previous experimental studies reporting this species as a carrier of Bd (30, 32, 60). Generally, those studies directly exposed individuals in the larval stage [Gosner stage (26–30)] without considering previous exposure regimes. In our study, L. catesbeianus were vulnerable to Bd exposure in response to direct exposure and across life history transitions.
We found species-specific embryonic mortality after exposure to Bd. Many pathogens impact anuran embryos, including ranavirus (76), oomycetes (81–84), filamentous ascomycetes (85) and microsporidia (86). The mechanisms of protection offered by the vitelline membrane against ranavirus are unknown. However, bacteriostatic activity of the egg membrane or the capsule (87) or just its role as structural barrier when exposed to contaminants have been proposed (88, 89). In the case of Oomycetes, zoospores are chemotactic and move toward suitable substrates where they can germinate and grow. Similarly, ascomycetes are able to grow through the jelly. Few studies have suggested how Bd affects anuran embryos. Bd enzymatic action is one mechanism that could explain this result, as it can cause damage in skin tissue of hosts after exposure (30, 49, 90, 91). A complex mix of proteolytic and hydrolyzing enzymes (esterases) that degrade amphibian tissue have been described from different Bd isolates (90–92). In addition, many hatching anurans release enzymes to assist with degradation of the egg capsule at the moment of hatching (93, 94); this could potentially facilitate the enzymatic action of Bd to degrade tissues. Recently, dose-dependent mortality and proliferation in zebrafish (Danio rerio) tissue was reported with toxins secreted after the establishment of Bd sporangia (95).
The present study offers useful information about the complexity of host response to a pathogen, particularly with multiple exposures across life stages. Our study provides information about direct effects of Bd on anuran embryos, with significant impacts on mortality and the proportion of hatching success. Our results also quantified latent effects of Bd exposure over ontogeny (96). Despite being a relatively brief period, exposure to Bd in the egg led to increased mortality after hatching and species-specific differences were due to the timing of embryonic exposure and re-exposure in the larval stage. As we increase our understanding of how Bd impacts amphibians through direct and even latent effects, we are recognizing that the effects Bd may have on population dynamics and conservation of amphibians have been underestimated in wild populations.
Additional research exploring the mechanisms protecting the embryos is needed to better understand the susceptibility of this developmental stage to disease. Characteristics such as jelly thickness and composition, or size of the capsule, can be involved in resistance to chytrid. As eggs received material from their parents during oviposition, evaluating the role of parents in the immune response of their offspring can help us to understand more about embryonic immunity. As continued survey efforts have located Bd in populations of amphibians around the world, there is growing evidence that the risk of Bd cannot be simplified to species susceptibility. Instead, Bd risk includes strain, host life-stage, and specific exposure scenarios. Further studies are also required to better understand how variation in other environmental and biological parameters can affect the outcome of repeated Bd exposure in anuran species. Our results add information to the growing body of evidence concerning differential susceptibility to pathogens among amphibian species and across life stages.
Data Availability Statement
The raw data supporting the conclusions of this article will be made available by the authors, without undue reservation.
Ethics Statement
The animal study was reviewed and approved by Institutional Animal Care and Use committee Oregon State University.
Author Contributions
JU, AB, and TG conceptualized the manuscript. JU and EB performed the field work, experiments and data analysis. JU, EB, AB, and TG have made a significant, direct and intellectual contribution to the work. All authors contributed to the article and approved the submitted version.
Funding
Publication of this paper was supported, in part, by the Henry Mastin Graduate Student Fund (Department of Fisheries, Wildlife and Conservation Sciences, Oregon State University) and the US Department of Agriculture, Forest Service, Pacific Northwest Research Station through Deanna H. Olson.
Conflict of Interest
The authors declare that the research was conducted in the absence of any commercial or financial relationships that could be construed as a potential conflict of interest.
Publisher's Note
All claims expressed in this article are solely those of the authors and do not necessarily represent those of their affiliated organizations, or those of the publisher, the editors and the reviewers. Any product that may be evaluated in this article, or claim that may be made by its manufacturer, is not guaranteed or endorsed by the publisher.
Acknowledgments
We thank N. Soria, L. Londono, and G. Mayorga for assistance measuring and staging tadpoles and assistance with animal care. D. Olson, C. Harjoe, and L. Thurman for help during egg masses collection, Spatafora Lab., Tanguay Lab., and the Center for Genome Research and Biocomputing at Oregon State University for providing laboratory resources for analysis.
References
1. Merilä J, Svensson E. Are fat reserves in migratory birds affected by condition in early life? J Avian Biol. (1997) 28:279–86. doi: 10.2307/3676940
2. Miller K, Packard GC, Packard MJ. Hydric conditions during incubation influence locomotor performance of hatchling snapping turtles. J Exp Biol. (1987) 127:401–12. doi: 10.1242/jeb.127.1.401
3. Heintz RA, Rice SD, Wertheimer AC, Bradshaw RF, Thrower FP, Joyce JE, et al. Delayed effects on growth and marine survival of pink salmon Oncorhynchus gorbuscha after exposure to crude oil during embryonic development. Mar Ecol Prog Ser. (2000) 208:205–16. doi: 10.3354/meps208205
4. Pechenik JA. Larval experience and latent effects—metamorphosis is not a new beginning. Integr Comp Biol. (2006) 46:323–33. doi: 10.1093/icb/icj028
5. Uller T, Sagvik J, Olsson M. Pre-hatching exposure to water mold reduces size at metamorphosis in the moor frog. Oecologia. (2009) 160:9–14. doi: 10.1007/s00442-009-1280-6
6. Murillo-Rincón AP, Laurila A, Orizaola G. Compensating for delayed hatching reduces offspring immune response and increases life-history costs. Oikos. (2017) 126:565–71. doi: 10.1111/oik.04014
7. Sniegula S, Janssens L, Stoks R. Integrating multiple stressors across life stages and latitudes: combined and delayed effects of an egg heat wave and larval pesticide exposure in a damselfly. Aquatic Toxicology. (2017) 186:113–22. doi: 10.1016/j.aquatox.2017.02.029
8. LaFiandra EM, Babbitt KJ. Predator induced phenotypic plasticity in the pinewoods tree frog, Hyla femoralis: necessary cues and the cost of development. Oecologia. (2004) 138:350–9. doi: 10.1007/s00442-003-1412-3
9. Richter-Boix A, Orizaola G, Laurila A. Transgenerational phenotypic plasticity links breeding phenology with offspring life-history. Ecology. (2014) 95:2715–22. doi: 10.1890/13-1996.1
10. Garcia TS, Urbina J, Bredeweg E, Ferrari MCO. Embryonic learning and developmental carry-over effects in an invasive anuran. Oecologia. (2017) 184:623–31 doi: 10.1007/s00442-017-3905-5
11. Sih A, Moore RD. Delayed hatching of salamander eggs in response to enhanced larval predation risk. Am Nat. (1993) 142:947–60. doi: 10.1086/285583
12. Warkentin KM. Adaptive plasticity in hatching age: a response to predation risk trade-offs. Proc Nat Acad Sci. (1995) 92:3507–10. doi: 10.1073/pnas.92.8.3507
13. Warkentin KM. Plasticity of hatching in amphibians: evolution, trade-offs, cues and mechanisms. Integr Comp Biol. (2011) 51:111–27. doi: 10.1093/icb/icr046
14. Chivers DP, Kiesecker JM, Marco A, Devito J, Anderson MT, Blaustein AR. Predator-induced life history changes in amphibians: egg predation induces hatching. Oikos. (2001) 92:135–42. doi: 10.1034/j.1600-0706.2001.920116.x
15. Gomez-Mestre I, Touchon JC, Warkentin KM. Amphibian embryo and parental defenses and a larval predator reduce egg mortality from water mold. Ecology. (2006) 87:2570–81. doi: 10.1890/0012-9658(2006)87[2570:AEAPDA]2.0.CO;2
16. Touchon JTJ, Gomez-Mestre IG-MI, Warkentin KWK. Hatching plasticity in two temperate anurans: responses to a pathogen and predation cues. Can J Zool. (2006) 84:556–63. doi: 10.1139/z06-058
17. Touchon JC, McCoy MW, Vonesh JR, Warkentin KM. Effects of plastic hatching timing carry over through metamorphosis in red-eyed treefrogs. Ecology. (2013) 94:850–60. doi: 10.1890/12-0194.1
18. Fernández-Benéitez M, Ortiz-Santaliestra M, Lizana M, Diéguez-Uribeondo J. Differences in susceptibility to Saprolegnia infections among embryonic stages of two anuran species. Oecologia. (2011) 165:819–26. doi: 10.1007/s00442-010-1889-5
19. Rumschlag SL, Boone MD. How time of exposure to the amphibian chytrid fungus affects Hyla chrysoscelis in the presence of an insecticide. Herpetologica. (2015) 71:169–76. doi: 10.1655/HERPETOLOGICA-D-13-00070
20. Develey-Rivière M-P, Galiana E. Resistance to pathogens and host developmental stage: a multifaceted relationship within the plant kingdom. New Phytol. (2007) 175:405–16. doi: 10.1111/j.1469-8137.2007.02130.x
21. Brutscher LM, Daughenbaugh KF, Flenniken ML. Antiviral defense mechanisms in honey bees. Curr Opin Insect Sci. (2015) 10:71–82. doi: 10.1016/j.cois.2015.04.016
22. Mast J, Goddeeris BM. Development of immunocompetence of broiler chickens. Vet Immunol Immunopathol. (1999) 70:245–56. doi: 10.1016/S0165-2427(99)00079-3
23. Holgersson MCN, Nichols WA, Paitz RT, Bowden RM. How important is the eggshell as a source for initial acquisition of Salmonella in hatchling turtles? J Exp Zool. (2016) 325:142–8. doi: 10.1002/jez.2004
24. Valkenburg SA, Venturi V, Dang THY, Bird NL, Doherty PC, Turner SJ, et al. Early priming minimizes the age-related immune compromise of CD8+ T cell diversity and function. PLoS Pathog. (2012) 8:e1002544. doi: 10.1371/journal.ppat.1002544
25. Rohr JR, Raffel TR, Hall CA. Developmental variation in resistance and tolerance in a multi-host–parasite system. Functional Ecology. (2010) 24:1110–21. doi: 10.1111/j.1365-2435.2010.01709.x
26. Echaubard P, Pauli BD, Trudeau VL, Lesbarrères D. Ranavirus infection in northern leopard frogs: the timing and number of exposures matter. J Zool. (2016) 298:30–6. doi: 10.1111/jzo.12281
27. Hatcher MJ, Dick JTA, Dunn AM. Disease emergence and invasions. Funct Ecol. (2012) 26:1275–87. doi: 10.1111/j.1365-2435.2012.02031.x
28. Olson DH, Aanensen DM, Ronnenberg KL, Powell CI, Walker SF, Bielby J, et al. The Bd mapping group. Mapping the global emergence of Batrachochytrium dendrobatidis, the amphibian chytrid fungus. PLoS ONE. (2013) 8:e56802. doi: 10.1371/journal.pone.0056802
29. Berger L, Roberts AA, Voyles J, Longcore JE, Murray KA, Skerratt LF. History and recent progress on chytridiomycosis in amphibians. Fungal Ecol. (2016) 19:89–99. doi: 10.1016/j.funeco.2015.09.007
30. Blaustein AR, Romansic JM, Scheessele EA, Han BA, Pessier AP, Longcore JE. Interspecific variation in susceptibility of frog tadpoles to the pathogenic fungus Batrachochytrium dendrobatidis. Conservation Biology. (2005) 19:1460–8. doi: 10.1111/j.1523-1739.2005.00195.x
31. Searle CL, Gervasi SS, Hua J, Hammond JI, Relyea RA, Olson DH, et al. Differential host susceptibility to Batrachochytrium dendrobatidis, an emerging amphibian pathogen. Conservation Biology. (2011) 25:965–74. doi: 10.1111/j.1523-1739.2011.01708.x
32. Gahl MK, Longcore JE, Houlahan JE. Varying responses of northeastern north american amphibians to the chytrid pathogen Batrachochytrium dendrobatidis. Conservation Biology. (2012) 26:135–41. doi: 10.1111/j.1523-1739.2011.01801.x
33. Gervasi S, Gondhalekar C, Olson DH, Blaustein AR. Host identity matters in the amphibian-Batrachochytrium dendrobatidis system: fine-scale patterns of variation in responses to a multi-host pathogen. PLoS ONE. (2013) 8:e54490. doi: 10.1371/journal.pone.0054490
34. Gervasi SS, Stephens PR, Hua J, Searle CL, Xie GY, Urbina J, et al. Linking ecology and epidemiology to understand predictors of multi-host responses to an emerging pathogen, the amphibian chytrid fungus. PLoS ONE. (2017) 12:e0167882. doi: 10.1371/journal.pone.0167882
35. Bielby J, Fisher MC, Clare FC, Rosa GM, Garner TWJ. Host species vary in infection probability, sub-lethal effects, and costs of immune response when exposed to an amphibian parasite. Sci Rep. (2015) 5:10828. doi: 10.1038/srep10828
36. Tobler U, Schmidt BR. Within- and among-population variation in chytridiomycosis-induced mortality in the toad Alytes obstetricans. PLoS ONE. (2010) 5:e10927. doi: 10.1371/journal.pone.0010927
37. Bradley PW, Gervasi SS, Hua J, Cothran RD, Relyea RA, Olson DH, et al. Differences in sensitivity to the fungal pathogen Batrachochytrium dendrobatidis among amphibian populations: pathogen effects across populations. Conservation Biology. (2015) 29:1347–56. doi: 10.1111/cobi.12566
38. Briggs CJ, Vredenburg VT, Knapp RA, Rachowicz LJ. Investigating the population-level effects of chytridiomycosis: an emerging infectious disease of amphibians. Ecology. (2005) 86:3149–59. doi: 10.1890/04-1428
39. Briggs CJ, Knapp RA, Vredenburg VT. Enzootic and epizootic dynamics of the chytrid fungal pathogen of amphibians. Proc Nat Acad Sci. (2010) 107:9695–700. doi: 10.1073/pnas.0912886107
40. Garner TWJ, Walker S, Bosch J, Leech S, Marcus Rowcliffe J, Cunningham AA, et al. Life history tradeoffs influence mortality associated with the amphibian pathogen Batrachochytrium dendrobatidis. Oikos. (2009) 118:783–91. doi: 10.1111/j.1600-0706.2008.17202.x
41. Piovia-Scott J, Pope KL, Lawler SP, Cole EM, Foley JE. Factors related to the distribution and prevalence of the fungal pathogen Batrachochytrium dendrobatidis in Rana cascadae and other amphibians in the Klamath Mountains. Biol Conserv. (2011) 144:2913–21. doi: 10.1016/j.biocon.2011.08.008
42. Ortiz-Santaliestra ME, Rittenhouse TAG, Cary TL, Karasov WH. Interspecific and postmetamorphic variation in susceptibility of three north american anurans to Batrachochytrium dendrobatidis. J Herpetol. (2013) 47:286–92. doi: 10.1670/11-134
43. Retallick RWR, Miera V. Strain differences in the amphibian chytrid Batrachochytrium dendrobatidis and non-permanent, sub-lethal effects of infection. Dis Aquat Org. (2007) 75:201–7. doi: 10.3354/dao075201
44. Doddington BJ, Bosch J, Oliver JA, Grassly NC, Garcia G, Schmidt BR, et al. Context-dependent amphibian host population response to an invading pathogen. Ecology. (2013) 94:1795–804. doi: 10.1890/12-1270.1
45. Piovia-Scott J, Pope K, Joy Worth S, Rosenblum EB, Poorten T, Refsnider J, et al. Correlates of virulence in a frog-killing fungal pathogen: evidence from a California amphibian decline. ISME J. (2015) 9:1570–8. doi: 10.1038/ismej.2014.241
46. Dang T, Searle CL, Blaustein AR. Virulence variation among strains of the emerging infectious fungus Batrachochytrium dendrobatidis (Bd) in multiple amphibian host species. Dis Aquat Org. (2017) 124:233–9. doi: 10.3354/dao03125
47. Gervasi SS, Urbina J, Hua J, Chestnut T, Relyea RA, Blaustein AR. Experimental evidence for American bullfrog (Lithobates catesbeianus) susceptibility to chytrid fungus (Batrachochytrium dendrobatidis). Ecohealth. (2013) 10:166–71. doi: 10.1007/s10393-013-0832-8
48. Rosenblum EB, Fisher MC, James TY, Stajich JE, Longcore JE, Gentry LR, et al. Molecular perspective: biology of the emerging pathogen Batrachochytrium dendrobatidis. Dis Aquat Organ. (2010) 92:131–47. doi: 10.3354/dao02179
49. McMahon TA, Brannelly LA, Chatfield MWH, Johnson PTJ, Joseph MB, McKenzie VJ, et al. Chytrid fungus Batrachochytrium dendrobatidis has nonamphibian hosts and releases chemicals that cause pathology in the absence of infection. Proc Nat Acad Sci. (2013) 110:210–5. doi: 10.1073/pnas.1200592110
50. Fites JS, Ramsey JP, Holden WM, Collier SP, Sutherland DM, Reinert LK, et al. The invasive chytrid fungus of amphibians paralyzes lymphocyte responses. Science. (2013) 342:366. doi: 10.1126/science.1243316
51. Voyles J, Young S, Berger L, Campbell C, Voyles WF, Dinudom A, et al. pathogenesis of chytridiomycosis, a cause of catastrophic amphibian declines. Science. (2009) 326:582–5. doi: 10.1126/science.1176765
52. Dmitrieva EV. Influence of the concentration of dissolved oxygen on embryonic development of the common toad (Bufo bufo). Russ J Dev Biol. (2015) 46:368–80. doi: 10.1134/S1062360415060041
53. Blaustein A, Beatty J, Olson D, Storm R. The biology of amphibians and reptiles in old-growth forests in the Pacific Northwest. In: General Technical Report (GTR). Portland, OR: Pacific Northwest Research Station (1995). p.98. doi: 10.2737/PNW-GTR-337
54. Muths E, Corn PS, Pessier AP, Green DE. Evidence for disease-related amphibian decline in Colorado. Biol Conserv. (2003) 110:357–65. doi: 10.1016/S0006-3207(02)00239-2
55. Jones LLC, Leonard WP, Olson DH. Amphibians of the Pacific Northwest. Seattle, WA: Seattle Audubon Society (2005). p. 227
56. Gosner KL, A. simplified table for staging anuran embryos and larvae with notes on identification. Herpetologica. (1960) 16:183–90.
57. Schloegel LM, Toledo LF, Longcore JE, Greenspan SE, Vieira CA, Lee M, et al. Novel, panzootic and hybrid genotypes of amphibian chytridiomycosis associated with the bullfrog trade. Mol Ecol. (2012) 21:5162–77. doi: 10.1111/j.1365-294X.2012.05710.x
58. Marcum R, St-Hilaire S, Murphy P, Rodnick K. Effects of Batrachochytrium dendrobatidis infection on ion concentrations in the boreal toad Anaxyrus (Bufo) boreas boreas. Dis Aquat Org. (2010) 91:17–21. doi: 10.3354/dao02235
59. Searle CL, Belden LK, Du P, Blaustein AR. Stress and chytridiomycosis: exogenous exposure to corticosterone does not alter amphibian susceptibility to a fungal pathogen. J Exp Zool. (2014) 321:243–53. doi: 10.1002/jez.1855
60. Eskew EA, Worth SJ, Foley JE, Todd BD. American bullfrogs (Lithobates catesbeianus) resist infection by multiple isolates of Batrachochytrium dendrobatidis, including one implicated in wild mass mortality. Ecohealth. (2015) 12:513–8. doi: 10.1007/s10393-015-1035-2
61. R Core Team. R: A language and environment for statistical computing. Vienna, Au: R Foundation for Statistical Computing (2016). Available online at: http://www.R-project.org/
62. Retallick RWR, Miera V, Richards KL, Field KJ, Collins JP, A. non-lethal technique for detecting the chytrid fungus Batrachochytrium dendrobatidis on tadpoles. Dis Aquat Org. (2006) 72:77–85. doi: 10.3354/dao072077
63. Hyatt AD, Boyle DG, Olsen V, Boyle DB, Berger L, Obendorf D, et al. Diagnostic assays and sampling protocols for the detection of Batrachochytrium dendrobatidis. Dis Aquat Organ. (2007) 73:175–92. doi: 10.3354/dao073175
64. Kadekaru S, Une Y. Comparison of methods for detection of chytrid fungus (Batrachochytrium dendrobatidis) in bullfrog tadpole mouthparts. J Vet Med Sci. (2018) 80:260–2. doi: 10.1292/jvms.17-0071
65. Boyle D, Boyle D, Olsen V, Morgan J, Hyatt A. Rapid quantitative detection of chytridiomycosis (Batrachochytrium dendrobatidis) in amphibian samples using real-time Taqman PCR assay. Dis Aquat Organ. (2004) 60:141–8. doi: 10.3354/dao060141
66. Searle CL, Xie GY, Blaustein AR. Development and infectious disease in hosts with complex life cycles. PLoS ONE. (2013) 8:e60920. doi: 10.1371/journal.pone.0060920
67. Altig R, McDiarmid RW. Morphological diversity and evolution of egg and clutch structure in amphibians. Herpetol Monogr. (2007) 21:1–32. doi: 10.1655/06-005.1
68. Reeder NM, Pessier AP, Vredenburg VT. A reservoir species for the emerging amphibian pathogen Batrachochytrium dendrobatidis thrives in a landscape decimated by disease. PLoS ONE. (2012) 7:e33567. doi: 10.1371/journal.pone.0033567
69. Farrer RA, Weinert LA, Bielby J, Garner TWJ, Balloux F, Clare F, et al. Multiple emergences of genetically diverse amphibian-infecting chytrids include a globalized hypervirulent recombinant lineage. Proc Nat Acad Sci. (2011) 108:18732–6. doi: 10.1073/pnas.1111915108
70. Rosenblum EB, James TY, Zamudio KR, Poorten TJ, Ilut D, Rodriguez D, et al. Complex history of the amphibian-killing chytrid fungus revealed with genome resequencing data. Proc Nat Acad Sci. (2013) 110:9385–90. doi: 10.1073/pnas.1300130110
71. Johnson PTJ, Kellermanns E, Bowerman J. Critical windows of disease risk: amphibian pathology driven by developmental changes in host resistance and tolerance. Funct Ecol. (2011) 25:726–34. doi: 10.1111/j.1365-2435.2010.01830.x
72. Kirschman LJ, Crespi EJ, Warne RW. Critical disease windows shaped by stress exposure alter allocation trade-offs between development and immunity. J Anim Ecol. (2018) 87:235–46. doi: 10.1111/1365-2656.12778
73. Rohr JR, Raffel TR, Halstead NT, McMahon TA, Johnson SA, Boughton RK, Martin LB. Early-life exposure to a herbicide has enduring effects on pathogen-induced mortality. Proc Biol Soc B. (2013) 280:20131502. doi: 10.1098/rspb.2013.1502
74. Capellán E, Nicieza A. Trade-offs across life stages: does predator induced hatching plasticity reduce anuran post-metamorphic performance? Evol Ecol. (2007) 21:445–58. doi: 10.1007/s10682-006-9133-9
75. Vonesh JR, Bolker BM. Compensatory larval responses shift trade-offs associated with predator-induced hatching plasticity. Ecology. (2005) 86:1580–91. doi: 10.1890/04-0535
76. Haislip NA, Gray MJ, Hoverman JT, Miller DL. Development and disease: how susceptibility to an emerging pathogen changes through anuran development. PLoS ONE. (2011) 6:e22307. doi: 10.1371/journal.pone.0022307
77. Fernández-Loras A, Fernández-Beaskoetxea S, Arriero E, Fisher MC, Bosch J. Early exposure to Batrachochytrium dendrobatidis causes profound immunosuppression in amphibians. Eur J Wildl Res. (2017) 63:99. doi: 10.1007/s10344-017-1161-y
78. Hanselmann R, Rodriguez A, Lampo M, Fajardo-Ramos L, Aguirre A, Kilpatrick AM, et al. Presence of an emerging pathogen of amphibians in introduced bullfrogs Rana catesbeiana in Venezuela. Biol Conserv. (2004) 120:115–9. doi: 10.1016/j.biocon.2004.02.013
79. Daszak P, Strieby A, Cunningham AA, Longcore J, Brown C, Porter D. Experimental evidence that the bullfrog (Rana catesbeiana) is a potential carrier of chytridiomycosis, an emerging fungal disease of amphibians. Herpetol J. (2004) 14:201–7. Available online at: https://www.thebhs.org/publications/the-herpetological-journal/volume-14-number-4-october-2004/1773-06-experimental-evidence-that-the-bullfrog-rana-catesbeiana-is-a-potential-carrier-of-chytridiomycosis-an-emerging-fungal-disease-of-amphibians/file
80. Garner TWJ, Perkins MW, Govindarajulu P, Seglie D, Walker S, Cunningham AA, et al. The emerging amphibian pathogen Batrachochytrium dendrobatidis globally infects introduced populations of the North American bullfrog, Rana catesbeiana. Biol Lett. (2006) 2:455. doi: 10.1098/rsbl.2006.0494
81. Kiesecker JM, Blaustein AR. Synergism between UV-B radiation and a pathogen magnifies amphibian embryo mortality in nature. Proc Natl Acad Sci USA. (1995) 92:11049–52. doi: 10.1073/pnas.92.24.11049
82. Fernández-Benéitez MJ, Ortiz-Santaliestra ME, Lizana M, Diéguez-Uribeondo J. Saprolegnia diclina: another species responsible for the emergent disease ‘Saprolegnia infections’ in amphibians. FEMS Microbiol Lett. (2008) 279:23–9. doi: 10.1111/j.1574-6968.2007.01002.x
83. Ruthig GR. The influence of temperature and spatial distribution on the susceptibility of southern leopard frog eggs to disease. Oecologia. (2008) 156:895–903. doi: 10.1007/s00442-008-1026-x
84. Ruthig G. Water molds of the genera Saprolegnia and Leptolegnia are pathogenic to the North American frogs Rana catesbeiana and Pseudacris crucifer, respectively. Dis Aquat Org. (2009) 84:173–8. doi: 10.3354/dao02042
85. Warkentin KM, Currie CR, Rehner SA. Egg-killing fungus induces early hatching of red-eyed treefrog eggs. Ecology. (2001) 82:2860–9. doi: 10.1890/0012-9658(2001)082[2860:EKFIEH]2.0.CO;2
86. Green DE, Converse KA. “Diseases of amphibian eggs and embryos.” In: Majumdar SK, Huffman JE, Brenner FJ, Panah AI, editors. Wildlife Diseases: Landscape Epidemiology, Spatial Distribution and Utilization of Remote Sensing Technology. Easton, PA: The Pennsylvania Academy of Science (2005). p. 62–71. Available online at: http://pubs.er.usgs.gov/publication/85665
87. Han Y, Yu H, Yang X, Rees HH, Liu J, Lai R, et al. serine proteinase inhibitor from frog eggs with bacteriostatic activity. Comp Biochem Physiol B Biochem Mol Biol. (2008) 149:58–62. doi: 10.1016/j.cbpb.2007.08.003
88. Berrill M, Coulson D, McGillivray L, Pauli B. Toxicity of endosulfan to aquatic stages of anuran amphibians. Environ Toxicol Chem. (1998) 17:1738–44. doi: 10.1002/etc.5620170914
89. Pauli BD, Coulson DR, Berrill M. Sensitivity of amphibian embryos and tadpoles to Mimic® 240 LV insecticide following single or double exposures. Environ Toxicol Chem. (1999) 18:2538–44. doi: 10.1002/etc.5620181122
90. Symonds EP, Trott DJ, Bird PS, Mills P. Growth characteristics and enzyme activity in Batrachochytrium dendrobatidis isolates. Mycopathologia. (2008) 166:143–7. doi: 10.1007/s11046-008-9135-y
91. Moss A, Carty N, San Francisco M. Identification and partial characterization of an elastolytic protease in the amphibian pathogen Batrachochytrium dendrobatidis. Dis Aquat Org. (2010) 92:149–58. doi: 10.3354/dao02223
92. Brutyn M, D'Herde K, Dhaenens M, Rooij PV, Verbrugghe E, Hyatt AD, et al. Batrachochytrium dendrobatidis zoospore secretions rapidly disturb intercellular junctions in frog skin. Fungal Genet Biol. (2012) 49:830–7. doi: 10.1016/j.fgb.2012.07.002
93. Carroll EJ, Hedrick JL. Hatching in the toad Xenopus laevis: morphological events and evidence for a hatching enzyme. Dev Biol. (1974) 38:1–13. doi: 10.1016/0012-1606(74)90254-1
94. Cohen KL, Seid MA, Warkentin KM. How embryos escape from danger: the mechanism of rapid, plastic hatching in red-eyed treefrogs. J Exp Biol. (2016) 219:1875. doi: 10.1242/jeb.139519
95. Liew N, Mazon Moya MJ, Wierzbicki CJ, Hollinshead M, Dillon MJ, Thornton CR, et al. Chytrid fungus infection in zebrafish demonstrates that the pathogen can parasitize non-amphibian vertebrate hosts. Nat Commun. (2017) 8:15048. doi: 10.1038/ncomms15048
Keywords: pathogen exposure, development, carry-over, embryos, larvae
Citation: Urbina J, Bredeweg EM, Blaustein AR and Garcia TS (2021) Direct and Latent Effects of Pathogen Exposure Across Native and Invasive Amphibian Life Stages. Front. Vet. Sci. 8:732993. doi: 10.3389/fvets.2021.732993
Received: 29 June 2021; Accepted: 28 September 2021;
Published: 29 October 2021.
Edited by:
Rachel E. Marschang, Laboklin GmbH & Co. KG, GermanyReviewed by:
Gabriela Parra-Olea, National Autonomous University of Mexico, MexicoJohn Abramyan, University of Michigan–Dearborn, United States
Susan C. Walls, United States Geological Survey (USGS), United States
Copyright © 2021 Urbina, Bredeweg, Blaustein and Garcia. This is an open-access article distributed under the terms of the Creative Commons Attribution License (CC BY). The use, distribution or reproduction in other forums is permitted, provided the original author(s) and the copyright owner(s) are credited and that the original publication in this journal is cited, in accordance with accepted academic practice. No use, distribution or reproduction is permitted which does not comply with these terms.
*Correspondence: Jenny Urbina, amVubnl1cmJpbmFAZ21haWwuY29t