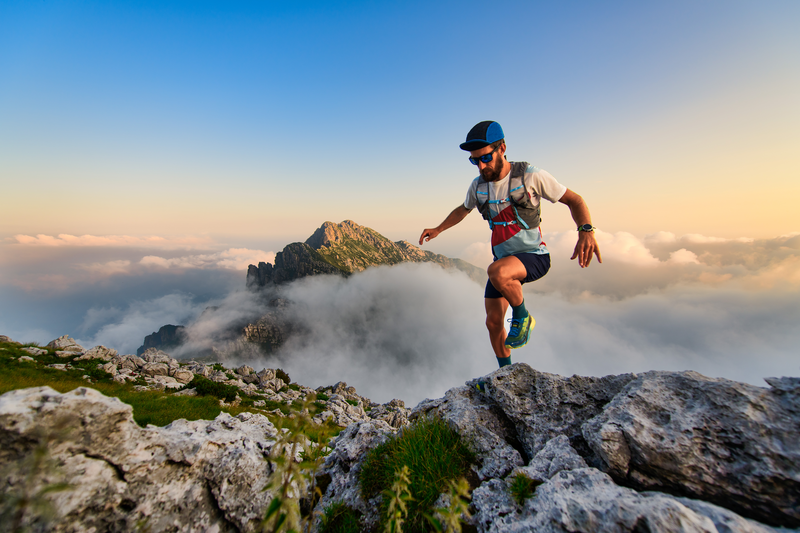
94% of researchers rate our articles as excellent or good
Learn more about the work of our research integrity team to safeguard the quality of each article we publish.
Find out more
ORIGINAL RESEARCH article
Front. Vet. Sci. , 16 September 2021
Sec. Veterinary Epidemiology and Economics
Volume 8 - 2021 | https://doi.org/10.3389/fvets.2021.698800
This article is part of the Research Topic Advancing the Development and Implementation of Regional, National Tuberculosis Control Programs in Livestock in Africa, Asia, and Latin America View all 10 articles
Local immunological responses at the site of infections, such as at the lymph nodes and lungs, do play a role in containing infection caused by Mycobacterium bovis (M. bovis). This bovine tuberculosis (bTB) study was conducted to evaluate cellular and cytokine responses in the lymph nodes and lungs of BCG-vaccinated and non-vaccinated calves that were naturally infected with M. bovis. Immunohistochemical assays were used for examination of the responses of macrophages, T cells, cytokines and chemical mediators of 40 (22 vaccinated and 18 non-vaccinated) Holstein-Friesian-zebu crossbred calves that were naturally exposed for 1 year to a known bTB positive cattle herd. The incidence rates of bTB visible lesion were 68.2% (15/22) and 89% (16/18) in vaccinated and non-vaccinated calves, respectively. The local responses of CD4+ and CD8+ T cells, and those of IFN-γ and TNF-α within the lesions, were stronger (P < 0.05) in BCG-vaccinated calves than in non-vaccinated calves. However, there was no statistically significant difference between the two groups (P > 0.05) in the response of CD68+ cells. Thus, the findings of this study indicated stronger responses of a set of immunological cells and markers at the local granulomas of BCG-vaccinated calves than in non-vaccinated calves. Furthermore, BCG vaccination may also play a role in reducing the severity of the gross pathology at the primary site of infection.
Bovine tuberculosis (bTB) is a chronic progressive disease of cattle and other animals that is caused by Mycobacterium bovis (M. bovis) and is characterized by progressive development of granulomatous lesions, termed as tubercles, in different tissues. BTB poses a major economic problem worldwide costing US$3 billion annually through infecting over 50 million cattle (1). In addition, it causes zoonotic TB in humans particularly in developing countries in Africa, Asia and Latin America (2). The conventional control measures for bTB are based on a test-and-slaughter method, which is less likely to be implemented by the developing countries because of socioeconomic reasons (3). Furthermore, the test-and-slaughter control method have been shown not to be effective in some developed countries, like the United Kingdom and New Zealand, because of the interference of reservoirs of M. bovis in infected wildlife (4, 5). Therefore, alternative control methods such as the use of vaccination are required for improved control of bTB.
BCG is the only vaccine approved against human TB (6) and animal TB (7), and it has been used in field studies in cattle yielding variable protective efficacy levels (8–11). Most of these studies evaluated immunological responses induced by BCG vaccination in peripheral blood. In addition, data on the immunological responses induced by BCG-vaccination at the local site of infection (granulomatous lesion) are useful for understanding the roles of different cell types and immunological markers (12–14).
Cell-mediated immune response is crucial in the defense against intracellular bacterial pathogens including mycobacteria (15, 16). Immune cells like CD4, CD8 and γ/δ T cells are shown to be activated when exposed to mycobacterial antigens based on studies conducted in mice, humans, and cattle (17–19). These cells are recruited to the site of infection and are capable of producing various cytokines, including interferon gamma (IFN-γ) and tumor necrosis factor alpha (TNF-α) (20).
BTB granulomas are characterized by different developmental stages that are influenced by the interaction between the host immunological responses and virulence of M. bovis isolates (21, 22). The granuloma, the hallmark lesions of TB disease, is characterized by the accumulation of activated macrophages and a variety of other immune cells against the mycobacteria. The formation of the granuloma have long been regarded as the critical host-protective structures that wall off the bacteria, creating an immune microenvironment in which the infection can be controlled. Within the granuloma the mycobacteria are thought to undergo replicative arrest and in response to stress such as hypoxia (23). However, if immunological responses are weakened, the granulomas fail to contain the pathogen, and as a result, the bacilli disseminate to secondary sites of infection, producing multiple granulomas (24). Thus, the interaction between the immune response and the mycobacteria at the local lesion is determining the outcome of the disease. It can also be observed that granuloma protect the mycobacteria from the immune response and is probably responsible for the persistent infection (25, 26). Microscopically, bTB granulomas have been classified into four different stages; stage I being the least severe stage while stage IV being the most severe stage (27, 28). The present study was conducted along a BCG efficacy study (29), to evaluate the cellular and cytokine responses induced by BCG-vaccination in the granulomas of Holstein-Friesian (H-F) and zebu crossbred calves that were naturally exposed to M. bovis in Ethiopia.
A BCG efficacy study against bovine TB conducted at the National Animal Health Diagnostic and Investigation Centre (NAHDIC) under natural challenge conditions was utilized for this study to generate immunohistochemical (IHC) information in the same animals. In short, male HF-zebu crossbreed calves were recruited within 2 weeks of age from known bTB free cattle farms as confirmed by the Single Intradermal Comparative Cervical Tuberculin (SICCT) test for which PPD-A and PPD-B were sourced from Thermo Fisher (Lelystad, the Netherlands). Calves were randomly assigned to either the unvaccinated control or the BCG vaccinated group. The latter group was vaccinated subcutaneously with 1 × 106 CFU BCG (InterVax, Canada), a vaccine based on the Russian type of Mycobacterium bovis BCG. Six weeks after vaccination, the two groups of calves were introduced into a herd of SICCT test positive cows at a ratio of 2:1 (Experimental calves: Reactor cows). After completion of 12 months exposure, 22 BCG vaccinated and 18 non-vaccinated calves had completed the experiment and they were slaughtered for post mortem examination and collection of tissue samples.
Gross and microscopic pathological examinations as well as immunohistochemical examination were performed by a pathologist who was blinded to the vaccination status of the calves. The pathologist was trained on IHC at the Pathology Laboratory of Surrey University (UK) by Dr. Francisco J. Salguero before performing this experiment. All lymph nodes in the head and neck region (left and right lateral retropharyngeal, left and right medial retropharyngeal, left and right mandibular, and left and right parotid), thoracic (mediastinal, cranial and caudal, right and left bronchial and tracheobronchial) and abdominal (hepatic and mesenteric) cavities, together with the lungs were carefully examined for gross lesions of TB and for mycobacterial culturing by Bayissa et al. (29). For the present study, all TB-like gross lesions were collected into either 10% neutral buffered formalin or zinc salt fixative solution, depending on the type of markers, for further processing for IHC staining.
All tissue specimens were processed and embedded into paraffin wax in the histopathology laboratory at NAHDIC. Tissues for the investigation of CD68, IFN-γ, TNF-α, and iNOS were fixed in 10% neutral buffered formalin while tissues for the investigation of CD4 and CD8 cells were fixed in zinc salt by following the procedure used previously (30). All formalin fixed tissues were processed within 7 days as previously described (31) whereas zinc solution fixation was carried out for 72 h. Specimens were trimmed and processed using a SHANDON Citadel 1000 tissue processor, dehydrated in six rounds using graded ethyl alcohol (at concentrations 70, 95, 95, and 3 × 100%) to remove excess water from the tissue, and cleared in three passes of xylene to clean alcohol from the tissue and to make the tissue clear/translucent. The tissue samples were then impregnated in melted paraffin (two passes) and embedded using the paraffin wax embedding method by the TEC2900 modular Tissue Embedding Center (Histo-line laboratories). Sections of 4μm were prepared and placed on Vectabond-treated slides (Vector Laboratories, Peterborough, UK).
Lymph nodes and lung tissue sections from each animal were processed by IHC for cell types and chemical mediators using primary antibodies to label CD68, CD4, CD8, IFN-γ, TNF-α, or iNOS. The reagents and antibodies against each of these immunological markers are presented in Table 1 and the staining was conducted as previously described (32). Enzymatic digestion was used as an epitope demasking technique for the formalin fixed material. Trypsin solution was prepared by measuring 0.5 g of trypsin, 0.5 g of chymotrypsin and 1 g of CaCl2 and dissolving in 1 L of distilled water. A biotinylated secondary antibody (ABC Vector Elite; Vector Laboratories) was used at 1/200 (Ab + PBS) dilution for CD4 and CD8, and for IFN-γ and CD68 a biotinylated secondary antibody was used by adding 3 drops (135 μl) to 10 ml of normal horse serum (ABC Vector Elite; Vector Laboratories). For detection of TNF-α 1 drop (45 μl) of biotinylated secondary antibody from the ABC kit (ABC Vector Elite; Vector Laboratories) was added to 10 ml of PBS. For iNOS biotinylated Secondary antibody, the same dilution was prepared but instead of normal horse serum, normal goat serum was used from the ABC kit. A horseradish peroxidase-labeled avidin-biotin-complex was used with DAB as a chromogen (brown color) and Mayer's haematoxylin was used as counter-stain.
Stained sections were subjected to digital image analysis to ascertain the percentage area of the tissue section positively labeled (brown color) for each marker. Images were captured using light microscopy (Nikon ECLIPSE E200 LED) and digital image analysis software (Nikon-NIS Br, Nikon, Japan). Consecutive sections were used and all granulomas observed within the tissue section were analyzed. The whole area of the granuloma was selected as Region of Interest (ROI), and the area with immunohistochemically-positive reaction within the ROI was calculated by the software after setting the thresholds. The results are expressed as the percentage of positively stain area within the total area of the granuloma. Necrotic or mineralized areas were not included in the ROI to be analyzed as previously described (32).
Chi-square test for trend was used to compare the distribution of granulomas within the head and neck, thoracic, and abdominal lymph nodes as well as the lung sections. The presence of the different markers and cytokines were compared between the granulomas of vaccinated and non-vaccinated calves using the Mann-Whitney's U-test by Graph Pad Prism 8.0.2.263. This test was used to compare the medians and for all statistical analysis P < 0.05 was considered statistically significant.
BTB visible lesions were detected in 68.0% (15/22) of the BCG vaccinated and 89.0% (16/18) of the unvaccinated calves. Seven calves from the BCG vaccinated group and two calves from the BCG non-vaccinated group were found to be free from bTB visible lesions. Most of the visible lesions were found in the thoracic lymph nodes of both BCG vaccinated and non-vaccinated calves. In addition, the 31 animals with visible lesions were all confirmed by culture to be M. bovis infected (29).
The histopathological examination was used to identify different stages of granulomas of both the 15 BCG-vaccinated and the 16 non-vaccinated calves that had TB lesions in lymph nodes and/or lung tissues. In total, 79 lymph nodes and lung tissues were taken forward and investigated by histopathology and IHC, of which 34 were from BCG-vaccinated and 45 were from non-vaccinated calves. The majority of granulomas were found within the thoracic lymph nodes, with more stage IV granulomas present in the control group when compared to the BCG vaccinated (P < 0.05). A similar trend was observed for the lung sections, with many granulomas observed (all stages) in the control group while only few stage II and III granulomas were found in animals from the BCG vaccinated group. In the head lymph nodes, only stage I granulomas were found in the BCG vaccinated group while granulomas of all developmental stages were found in the control group (Supplementary Table 1). In contrast, more granulomas were observed in the abdominal cavity lymph nodes from BCG vaccinated animals, including several stage IV granulomas, while control animals only showed a few stage I and II granulomas (P < 0.05).
IHC was used to detect CD68+ macrophages, CD4+ and CD8+ T cells, TNF-α, IFN-γ, and iNOS within tissue sections. The responses of these immunological markers were compared between controls and vaccinated calves and further stratified by different granuloma stages. The positive labeling was expressed as a fraction of the total area examined in each slide. The following results of cellular and cytokine responses were quantified:
CD4+ cells. Immunohistochemical staining of CD4 demonstrated an increase in the number of stained cells in the BCG vaccinated calves compared to non-vaccinated calves in different stages of granuloma. The fraction of immunolabeled CD4+ cells among vaccinated calves was significantly higher (P < 0.05) in all four granuloma stages when compared with the non-vaccinated group (Figure 1).
CD8+ cells. Similarly, immunohistochemical staining of CD8 showed higher numbers of CD8+ cells in BCG vaccinated calves than in non-vaccinated calves and the difference was significant (P < 0.05) between the groups in stages II-IV (Figure 2).
Macrophages (CD68+). The immunohistochemical staining revealed no significant differences in CD68+ cell distribution in the different granuloma stages of the BCG vaccinated and non-vaccinated calves (Figure 3). The vaccinated group indicated a higher fraction of immune-labeled cells than the non-vaccinated group for stage I granulomas, but there was no statistically significant difference between the two groups (P > 0.05).
TNF-α+. The TNF-α+ immunohistochemical staining showed higher TNF-α+ response in BCG vaccinated as compared to the level of response in non-vaccinated calves (Figure 4), and that was the case in all four stages of granuloma (P < 0.05).
IFN-γ. Immunohistochemical staining for IFN-γ indicated an increased expression of IFN-γ in BCG vaccinated compared to non-vaccinated calves in all four granuloma stages, however, only in stage I-III were this increase statistically significant between the groups (P < 0.05) (Figure 5).
iNOS+. Positive staining for iNOS+ was also present at all stages of granulomas in both BCG vaccinated and non-vaccinated calves, and the analysis further demonstrated a statistically higher expression in BCG vaccinated calves at stage I, stage III, and stage IV (Figure 6).
Figure 1. Immunolabeling for CD4+ cells (ABC IHC stain, DAB brown chromogen). (A) Comparison of CD4+ cell distribution in different granuloma stages of BCG vaccinated (Vac, vaccinated) and non-vaccinated (Con, control) calves. (B) Stage I granuloma from non-vaccinated calves, showing stained CD4+ cells (arrows). (C) Stage I granuloma from BCG vaccinated calf showing more CD4+ cells (arrows). (D) Stage IV granuloma from non-vaccinated calf showing stained CD4+ cells (arrows). (E) Stage IV granuloma from BCG vaccinated calf showing stained CD4+ cells (arrows). Bar equals 50μm in (B–E). n, necrotic core.
Figure 2. Immunolabelling for CD8+ cells (ABC IHC stain, DAB brown chromogen). (A) Comparison of CD8+ cell distribution in different granuloma stages of BCG vaccinated (Vac) and non-vaccinated (Con) calves. (B) Stage I granuloma from non-vaccinated calf showing very few stained CD8+ cells (arrows). (C) Stage I granuloma from BCG vaccinated calf showing stained CD8+ cells (arrows). (D) Stage III granuloma from non-vaccinated calf showing few stained CD8+ cells (arrows). (E) Stage III granuloma from vaccinated calf showing abundance of CD8+ cells (arrows). Bar equals 50μm in (B,C) and 100μm in (D,E).
Figure 3. Immunolabelling for CD68+ cells. Comparison of CD68+ cell distribution in granuloma stages I and IV of BCG vaccinated (Vac) and non-vaccinated (Con) calves.
Figure 4. Immunolabelling for TNF-α+ (ABC IHC stain, DAB brown chromogen). (A) Comparison of TNF-α+ distribution in different granuloma stages of BCG vaccinated (Vac) and non-vaccinated (Con) calves. (B) Stage I granuloma from non-vaccinated calf with few number of TNF-α stained macrophages (arrows), (C) Stage I from BCG vaccinated calf with more positively stained macrophages (arrows). (D) Stage IV granuloma from non-vaccinated calf with moderate number of positive cells (arrows). (E) Stage IV granuloma from BCG vaccinated calf with abundance of positively stained cells (arrows). Bar equals 100μm in (B,C), and 50μm in (D,E). n, necrotic core.
Figure 5. Immunolabelling for IFN-γ+. (A) Comparison of IFN-γ+ cell distribution in different granuloma stages of BCG vaccinated (Vac) and non-vaccinated (Con) calves. (B) Stage I granuloma from non-vaccinated calf with some IFN-γ+ positively stained cells (arrows). (C) Stage I granuloma from BCG vaccinated calf has more lymphocytes stained for IFN-γ+ (arrows). (D) Stage IV granuloma showing IFN-γ+ stained cells from non-vaccinated calf (arrows). (E) Stage IV granuloma showing IFN-γ+ stained cells from BCG vaccinated calf (arrows). 50μm for (B,C), Bar equals 100μm in (D,E).
Figure 6. Immunolabelling for iNOS+. (A) Comparison of iNOS+ cell distribution in different granuloma stages of BCG vaccinated (Vac) and non-vaccinated (Con) calves. (B) Stage I granuloma from non-vaccinated calf with some iNOS+ positively stained cells (arrows). (C) Stage I granuloma from BCG vaccinated calf, many cells stained for iNOS+ (arrows). (D) Stage III granuloma from non-vaccinated calf showing stained cells with iNOS+ (arrows). (E) Stage III granuloma showing cells stained positively with iNOS+ from BCG vaccinated calf (arrows). (F) Stage IV granuloma from non-vaccinated calf showing cells stained with iNOS+ (arrows). (G) Stage IV granuloma from BCG vaccinated calf showing many cells stained with iNOS+ (arrows). Bar equals in (B,C) 50 μm, Bar equals 100μm (D–G). n, necrotic core.
In the present study, immunological responses were evaluated at local granuloma level in 15 BCG-vaccinated and 16 non-vaccinated calves that had been naturally infected with M. bovis and that showed gross visible lesions at the post-mortem examination. On the other hand, nine calves (seven from the vaccinated and two from the non-vaccinated group) had either not become infected or had no visible lesions. In the present study, more stage IV granuloma was observed in the mesenteric lymph node of vaccinated calves than in control calves. This observation can be considered as misleading, as such difference was more likely associated with the sampling procedure and preparation of tissue sections of the two groups of calves. As observed during post mortem examination, gross lesions were detected in the mesenteric lymph nodes of one vaccinated and one control calves. In both calves, the pathology score was 3, which is considered as the most severe for the lymph node score. This indicates that the severity of the gross pathology was similar in both the vaccinated and non-vaccinated calves. However, in microscopic pathological comparison, stage IV (the most severe microscopic pathology) was observed in the mesenteric lymph node of the vaccinated calf than in the mesenteric lymph node of the control calf. As the two calves had similar severity of gross pathology, the difference in microscopic pathology between the two calves is mainly attributed to the difference in the sampling of the two lymph nodes and or the difference in the sections of the two lymph nodes used for the evaluation of the microscopic lesions. In the case of the vaccinated calf, the part of the mesenteric lymph node that was sampled and examined was severely affected while in the case of the non-vaccinated calf, the part of the mesenteric lymph node that was sampled was less severely affected and could the peripheral part of the lymph node. But in general, it was observed that both gross and microscopic pathology were relatively less severe in vaccinated calves than in non-vaccinated calves. Substantiating this observation, a recently conducted meta-analysis suggested that BCG vaccination may help in accelerate control of bTB in endemic settings by inducing immunity against M. bovis and also limiting the progress TB lesion at the site of infection (33).
In the current study, the immunohistochemical staining of CD68, CD4, CD8, IFN-γ, TNF-α, and iNOS were evaluated and compared in BCG-vaccinated and non-vaccinated calves. The presence of these immunological markers was also compared between the different stages of granulomas using IHC staining on histological sections of granulomas from lymph nodes and lungs. The results showed stronger responses of CD4+ and CD8+ T cells in granulomas of the BCG vaccinated calves when compared to those from the unvaccinated group. This observation is consistent with the results in a study conducted by Hope et al. (34) who also reported higher induction of CD4+ and CD8+ T cell responses in BCG-vaccinated Holstein cattle compared to non-vaccinated (34). Studies have shown that the majority of CD8+ T cells in the local immunity outside the peripheral circulation are γδ T cells (35). In this regard, it is important to look into the expressions of γδ T cells at local lesion sites during natural M. bovis infection.
Phagocytic cells such as macrophages and dendritic cells, and T cells including CD4+, CD8+, and γδ T cells play important roles in controlling M. bovis infection in cattle (36). The observation of responses of these cells in bTB granulomas in the lymph nodes of BCG-vaccinated calves could suggest the significance of BCG in protecting against bTB (37). In the present study, the expression levels of CD68+ macrophages in the granulomas of vaccinated and non-vaccinated calves were not significantly different. On the contrary, Salguero et al. (38) reported a significant reduction in the expression of CD68+ macrophages in granulomas of BCG-vaccinated Holstein calves as compared to its expression in non-vaccinated animals, after being experimentally inoculated intratracheally (38). On the other hand, a study conducted by Tulu et al. (39) on M. bovis naturally infected cross breed cows showed a significant increase in the immune-labeling of CD68+ macrophages as the level of granuloma increases from stage I to IV in culture positive animals as compared to culture negative animals. However, at the early stages of granuloma formation, the immune-labeling of CD68+ macrophages was significantly higher for M. bovis culture negative cows as compared to culture positive animals, which was explained in terms of the role of CD68+ macrophages geared toward the protection and elimination mycobacteria (39).
Furthermore, the result of the present study showed that the expressions of IFN-γ, TNF-α, and iNOS were stronger in granulomas of vaccinated calves than in non-vaccinated calves. The protective role of IFN-γ in the response to M. bovis infection in cattle is well-established (40). Previous experimental infection reported that IFN-γ, TNF-α, and iNOS showed increased expressions among the lymph node granulomas of BCG vaccinated cattle compared to non-vaccinated (38). Additionally, TNF-α is known to act in conjunction with IFN-γ to induce the release of reactive oxygen and nitrogen in infected macrophages, which are required for the killing of intracellular bacilli (41). On the other hand, such release can become toxic at high concentrations through an increase in necrosis and tissue damage (42). Previous studies have indicated that the macrophage and iNOS activity are more intense and sustained along the granuloma development (43, 44).
The comparison of the microscopic pathology and immunological markers between the vaccinated and non-vaccinated calves were based on the examinations of one or few sections of tissues. The results of examinations of a single section or few sections does not give realistic comparison. Several sections should have been prepared and examined the comparisons of microscopic pathology and immunological markers between the vaccinated and control calves. The other limitation of this study is the consideration of only a few markers for comparison between the two groups of calves. Additional cell and cytokine, or chemokine markers could have been included in the study. In addition to the above two limitations, the use zinc solution fixed tissues for the histopathological examination and classification of granuloma. Tissue fixation with zinc solution is recommended for the investigation of CD4+ and CD8+ cells markers, but histopathological examination of tissues fixed with zinc solution is laborious and difficult to delineate the different stages of granuloma.
BTB continues to be a major animal and public health problem worldwide and new tools are required for the control of this disease, primarily in cattle. In the present study, vaccination of calves with BCG induced strong responses at the granulomas of CD4+ and CD8+ T cells, and the two cytokines TNF-α and IFN-γ as well as for the chemical mediator iNOS. These observations could suggest the potential of BCG vaccination in containing the spread of M. bovis and in reducing the severity of gross pathology at the primary sites of infection.
The raw data supporting the conclusions of this article will be made available by the authors, without undue reservation.
The animal study was reviewed and approved by Institutional Review Board (IRB) of Aklilu Lemma Institute of Pathobiology, Addis Ababa University (Reference number IRB/ALIPB/013/1017/18).
AS and GA conceived the study. AS and BB conducted the field and laboratory aspects of the study. FS and GA supervised and lead the study. AS, BT, and FS analyzed the result of the study and interpreted the result. AS, BT, and BG drafted the manuscript while FS, SB, and GA edited the manuscript. All authors reviewed the final draft and agreed with its content and conclusions.
This study was funded by the Biotechnology and Biological Sciences Research Council (BBSRC), the Department for International Development, the Economic & Social Research Council, the Medical Research Council, the Natural Environment Research Council and the Defense Science & Technology Laboratory, under the Zoonoses and Emerging Livestock Systems (ZELS) program, ref: BB/L018977/1. SB was also partly funded by the Department for Environment, Food & Rural Affairs, United Kingdom, for this study, ref: TBSE3294.
The authors declare that the research was conducted in the absence of any commercial or financial relationships that could be construed as a potential conflict of interest.
All claims expressed in this article are solely those of the authors and do not necessarily represent those of their affiliated organizations, or those of the publisher, the editors and the reviewers. Any product that may be evaluated in this article, or claim that may be made by its manufacturer, is not guaranteed or endorsed by the publisher.
In addition, authors would like to thank AHRI pathology laboratory and Mr. Selfu Girma for his support during the laboratory work. The members of the Ethiopia Control of Bovine Tuberculosis Strategies (ETHICOBOTS) consortium are: Abraham Aseffa, Adane Mihret, Bamlak Tessema, Bizuneh Belachew, Eshcolewyene Fekadu, Fantanesh Melese, Gizachew Gemechu, Hawult Taye, Rea Tschopp, Shewit Haile, Sosina Ayalew, Tsegaye Hailu, all from Armauer Hansen Research Institute, Ethiopia; Rea Tschopp from Swiss Tropical and Public Health Institute, Switzerland; Adam Bekele, Chilot Yirga, Mulualem Ambaw, Tadele Mamo, Tesfaye Solomon, all from Ethiopian Institute of Agricultural Research, Ethiopia; Tilaye Teklewold from Amhara Regional Agricultural Research Institute, Ethiopia; Solomon Gebre, Getachew Gari, Mesfin Sahle, Abde Aliy, Abebe Olani, AS, Gizat Almaw, Getnet Mekonnen, Mekdes Tamiru, Sintayehu Guta, all from National Animal Health Diagnostic and Investigation Center, Ethiopia; James Wood, Andrew Conlan, Alan Clarke, all from Cambridge University, United Kingdom; Henrietta L. Moore and Catherine Hodge, both from University College London, United Kingdom; Constance Smith at University of Manchester, United Kingdom; R. Glyn Hewinson, SB, Martin Vordermeier, Javier Nunez-Garcia, all from Animal and Plant Health Agency, United Kingdom; GA, BB, Aboma Zewude, Adane Worku, Lemma Terfassa, Mahlet Chanyalew, Temesgen Mohammed, Miserach Zeleke, all from Addis Ababa University, Ethiopia.
The Supplementary Material for this article can be found online at: https://www.frontiersin.org/articles/10.3389/fvets.2021.698800/full#supplementary-material
1. Waters WR, Palmer MV, Buddle BM, Vordermeier HM. Bovine tuberculosis vaccine research: historical perspectives and recent advances. Vaccine. (2012) 30:2611–22. doi: 10.1016/j.vaccine.2012.02.018
2. WHO-FAO-OIE-The Union. Roadmap for Zoonotic Tuberculosis. Geneva: World Health Organization (2017).
3. Quevillon E.-L., Otero FD, Meza LJ, Gutierréz-Pabello JA, Cuevas FÁC, et al. Assessment of the immune response induced in neonatal calves by vaccination with Mycobacterium bovis BCG phipps under field conditions. J Vet Healthcare. (2017) 1:47. doi: 10.14302/issn.2575-1212.jvhc-17-1662
4. de LISLE GW. Bovine tuberculosis - the New Zealand problem. Proc New Zealand Grassland Assoc. (1993) 55:199–202.
5. Fitzgerald SD, Kaneene JB. Wildlife reservoirs of bovine tuberculosis worldwide: hosts, pathology, surveillance, and control. Vet Pathol. (2012) 50:488–99. doi: 10.1177/0300985812467472
6. Corner LAL, Costello E, Lesellier S, O'Meara D, Gormley E. Vaccination of European badgers (Meles meles) with BCG by the subcutaneous and mucosal routes induces protective immunity against endobronchial challenge with Mycobacterium bovis. Tuberculosis. (2008) 88:601–9. doi: 10.1016/j.tube.2008.03.002
7. Gormley E, Bhuachalla D, O'Keeffe J, Murphy D, Aldwell FE, Fitzsimons T, et al. Oral vaccination of free-living badgers (meles meles) with bacille calmette guérin (BCG) vaccine confers protection against tuberculosis. PLoS ONE. (2017) 12:e0168851. doi: 10.1371/journal.pone.0168851
8. Buddle BM, de Lisle GW, Pfeffer A, Aldwell FE. Immunological responses and protection against Mycobacterium bovis in calves vaccinated with a low dose of BCG. Vaccine. (1995) 13:1123–30.
9. Vordermeier HM, Chambers MA, Cockle PJ, Whelan AO, Simmons J, Hewinson RG. Correlation of ESAT-6-Specific gamma interferon production with pathology in cattle following Mycobacterium bovis BCG vaccination against experimental bovine tuberculosis. Infect Immun. (2002) 70:3026–32. doi: 10.1128/IAI.70.6.3026-3032.2002
10. Ameni G, Vordermeier M, Aseffa A, Young D, Hewinson G. Field evaluation of the efficacy of Mycobacterium bovis bacillus calmette-guerin against bovine tuberculosis in neonatal calves in ethiopia. Clin Vaccine Immunol. (2010) 17:1533–8. doi: 10.1128/CVI.00222-10
11. Ameni G, Tafess K, Zewde A, Eguale T, Tilahun M, Hailu T, et al. Vaccination of calves with Mycobacterium bovis bacillus calmette-guerin reduces the frequency and severity of lesions of bovine tuberculosis under a natural transmission setting in ethiopia. Transbound Emerg Dis. (2018) 65:96–104. doi: 10.1111/tbed.12618
12. Moliva JI, Turner J, Torrelles JB. Immune responses to bacillus calmette–guérin vaccination: why do they fail to protect against Mycobacterium tuberculosis? Front Immunol. (2017) 8:407. doi: 10.3389/fimmu.2017.00407
13. Tanner R, Villarreal-Ramos B, Vordermeier HM, McShane H. The humoral immune response to BCG vaccination. Front Immunol. (2019) 10:1317. doi: 10.3389/fimmu.2019.01317
14. Flynn JL, Chan J, Lin PL. Macrophages and control of granulomatous inflammation in tuberculosis. Mucosal Immunol. (2011) 4:271–8. doi: 10.1038/mi.2011.14
15. Cooper AM. Cell-mediated immune responses in tuberculosis. Annu Rev Immunol. (2009) 27:393–422. doi: 10.1146/annurev.immunol.021908.132703
16. Thakur A, Mikkelsen H, Jungersen G. Intracellular pathogens: host immunity and microbia l persistence strategies. J Immunol Res. (2019) 2019:1–24. doi: 10.1155/2019/1356540
17. Flynn JL, Chan J. Immunology of tuberculosis. Annu Rev Immunol. (2001) 19:93–129. doi: 10.1146/annurev.immunol.19.1.93
18. Hope JC, Kwong LS, Sopp P, Collins RA, Howard CJ. Dendritic cells induce CD4+ and CD8+ T-Cell responses to Mycobacterium bovis and M. avium antigens in bacille calmette guerin vaccinated and nonvaccinated cattle. Scand J Immunol. (2000) 52:285–91. doi: 10.1046/j.1365-3083.2000.00780.x
19. Pollock JM, Pollock DA, Campbell DG, Girvin RM, Crockard AD, Neill SD, et al. Dynamic changes in circulating and antigen-responsive T-cell subpopulations post-Mycobacterium bovis infection in cattle. Immunology. (1996) 87:236–41. doi: 10.1046/j.1365-2567.1996.457538.x
20. Serbina NV, Flynn JL. Early emergence of CD8(+) T cells primed for production of type 1 cytokines in the lungs of Mycobacterium tuberculosis-infected mice. Infect Immun. (1999) 67:3980–8. doi: 10.1128/IAI.67.8.3980-3988
21. Neill SD, Bryson DG, Pollock JM. Pathogenesis of tuberculosis in cattle. Tuberculosis. (2001) 81:79–86. doi: 10.1054/tube.2000.0279
22. Carrisoza-Urbina J, Morales-Salinas E, Bedolla-Alva MA, Hernández-Pando R, Gutiérrez-Pabello JA. Atypical granuloma formation in Mycobacterium bovis-infected calves. PLoS ONE. (2019) 14:e0218547. doi: 10.1371/journal.pone.0218547
23. Ramakrishnan L. The zebrafish guide to tuberculosis immunity and treatment. Cold Spring Harb Symp Quant Biol. (2013) 78:179–92. doi: 10.1101/sqb.2013.78.023283
24. Palmer MV, Waters WR, Thacker TC. Lesion development and immunohistochemical changes in granulomas from cattle experimentally infected with Mycobacterium bovis. Vet Pathol. (2007) 44:863–74. doi: 10.1354/vp.44-6-863
25. Cavaleiro JS, Trindade BC, Balderramas HA, Haanwinckel MC, Pinto JGG, Oliveira SL, et al. Immune response to Mycobacterium bovis-AN5 infection in genetically selected mice (selection IV-A). J Venomous Anim Toxins Include Trop Dis. (2007) 13:620–39. doi: 10.1590/S1678-91992007000300006
26. Bezos J, Alvarez J, Romero B, de Juan L, Domínguez L. Bovine tuberculosis: historical perspective. Res Vet Sci. (2017) 97:S3–4. doi: 10.1016/j.rvsc.2014.09.003
27. Wangoo A, Johnson L, Gough J, Ackbar R, Inglut S, Hicks D, et al. Advanced granulomatous lesions in Mycobacterium bovis-infected cattle are associated with increased expression of type I procollagen, gammadelta (WC1+) T cells and CD 68+ cells. J Comp Pathol. (2005) 133:223–34. doi: 10.1016/j.jcpa.2005.05.001
28. Palmer MV, Wiarda J, Kanipe C, Thacker TC. Early pulmonary lesions in cattle infected via aerosolized Mycobacterium bovis. Vet Pathol. (2019) 56:544–54. doi: 10.1177/0300985819833454
29. Bayissa B, Sirak A, Zewde A, Zeleke Y, Chanyalew M, Gumi B, Berg S, et al. Evaluation of the efficacy of BCG in protecting against contact challenge with bovine tuberculosis in holstein-friesian and zebu crossbred calves in ethiopia. Front Vet Sci. (2021) 8:702402. doi: 10.3389/fvets.2021.702402
30. Hicks DJ, Johnson L, Mitchell SM, Gough J, Cooley WA, La Ragione RM, et al. Evaluation of zinc salt based fixatives for preserving antigenic determinants for immunohistochemical demonstration of murine immune system cell markers. Biotech Histochem. (2006) 81:23–30. doi: 10.1080/10520290600725375
31. Suvarna KS, Layton C, Bancroft JD. Bancroft's Theory and Practice of histological Techniques, 7th ed. Elsevier: Churchill Livingstone (2012).
32. Aranday-Cortes E, Bull NC, Villarreal-Ramos B, Gough J, Hicks D, Ortiz-Peláez Á, et al. Upregulation of IL-17A, CXCL9 and CXCL10 in early-stage granulomas induced by Mycobacterium bovis in cattle. Transbound Emerg Dis. (2013) 60:525–37. doi: 10.1111/j.1865-1682.2012.01370.x
33. Srinivasan S., Conlan AJK, Easterling LA, Herrera C, Dandapat P, Veerasami M, et al. A meta-analysis of the effect of bacillus calmette-guérin vaccination against bovine tuberculosis: is perfect the enemy of good? Front Vet Sci. (2021) 8:637580. doi: 10.3389/fvets.2021.637580
34. Hope JC, Thom ML, Villarreal-Ramos B, Vordermeier HM, Hewinson RG, Howard CJ. Vaccination of neonatal calves with Mycobacterium bovis BCG induces protection against intranasal challenge with virulent M. bovis. Clin Exp Immunol. (2005) 139:48–56. doi: 10.1111/j.1365-2249.2005.02668.x
35. Guzman E, Hope J, Taylor G, Smith AL, Cubillos-Zapata C, Charleston B. Bovine γδ T cells are a major regulatory T cell subset. J Immunol. (2014) 193:208–22. doi: 10.4049/jimmunol.1303398
36. Parlane NA, Buddle BM. Immunity and vaccination against tuberculosis in cattle. Curr Clin Microbiol Rep. (2015) 2:44–53. doi: 10.1007/s40588-014-0009-4
37. Johnson L, Gough J, Spencer Y, Hewinson G, Vordermeier M, Wangoo A. Immunohistochemical markers augment evaluation of vaccine efficacy and disease severity in bacillus Calmette-Guerin (BCG) vaccinated cattle challenged with Mycobacterium bovis. Vet Immunol Immunopathol. (2006) 111:219–29. doi: 10.1016/j.vetimm.2006.01.016
38. Salguero FJ, Gibson S, Garcia-Jimenez W, Gough J, Strickland TS, Vordermeier HM, et al. Differential cell composition and cytokine expression within lymph node granulomas from BCG-vaccinated and non-vaccinated cattle experimentally infected with Mycobacterium bovis. Transbound Emerg Dis. (2017) 64:1734–49. doi: 10.1111/tbed.12561
39. Tulu B, Martineau HM, Zewude A, Desta F, Jolliffe DA, Abebe M, et al. Cellular and cytokine responses in the granulomas of asymptomatic cattle naturally infected with Mycobacterium bovis in Ethiopia. Infect Immun. (2020) 88:e00507–20. doi: 10.1128/IAI.00507-20
40. Villarreal-Ramos B, McAulay M, Chance V, Martin M, Morgan J, Howard CJ. Investigation of the role of CD8+ T cells in bovine tuberculosis in vivo. Infection Immunity. (2003) 71:4297–303. doi: 10.1128/IAI.71.8.4297-4303.2003
41. Mohan VP, Scanga CA, Yu K, Scott HM, Tanaka KE, Tsang E, et al. Effects of tumor necrosis factor alpha on host immune response in chronic persistent tuberculosis: possible role for limiting pathology. Infection Immunity. (2001) 69:1847–55. doi: 10.1128/IAI.69.3.1847-1855.2001
42. Zganiacz A, Santosuosso M, Wang J, Yang T, Chen L, Anzulovic M, et al. TNF-alpha is a critical negative regulator of type 1 immune activation during intracellular bacterial infection. J Clin Invest. (2004) 113:401–13. doi: 10.1172/JCI18991
43. García-Jiménez WL, Fernández-Llario P, Gómez L, Benítez-Medina JM, García-Sánchez A, Martínez R, et al. Histological and immunohistochemical characterisation of Mycobacterium bovis induced granulomas in naturally infected fallow deer (Dama dama). Vet Immunol Immunopathol. (2012) 149:66–75. doi: 10.1016/j.vetimm.2012.06.010
Keywords: BCG vaccination, crossbred calves, immunological response, Mycobacterium bovis, natural infection
Citation: Sirak A, Tulu B, Bayissa B, Gumi B, Berg S, Salguero FJ, Ameni G and The ETHICOBOTS Consortium (2021) Cellular and Cytokine Responses in Lymph Node Granulomas of Bacillus Calmette Guérin (BCG)-Vaccinated and Non-vaccinated Cross-Breed Calves Naturally Infected With Mycobacterium bovis. Front. Vet. Sci. 8:698800. doi: 10.3389/fvets.2021.698800
Received: 22 April 2021; Accepted: 02 August 2021;
Published: 16 September 2021.
Edited by:
Vivek Kapur, The Pennsylvania State University (PSU), United StatesReviewed by:
Mirinda Van Kleef, Agricultural Research Council of South Africa (ARC-SA), South AfricaCopyright © 2021 Sirak, Tulu, Bayissa, Gumi, Berg, Salguero, Ameni and The ETHICOBOTS Consortium. This is an open-access article distributed under the terms of the Creative Commons Attribution License (CC BY). The use, distribution or reproduction in other forums is permitted, provided the original author(s) and the copyright owner(s) are credited and that the original publication in this journal is cited, in accordance with accepted academic practice. No use, distribution or reproduction is permitted which does not comply with these terms.
*Correspondence: Gobena Ameni, Z29iZW5hLmFtZW5pQGFhdS5lZHUuZXQ=; Z29iZW5hLmFtZW5pQHVhZXUuYWMuYWU=
†Members of the ETHICOBOTS consortium are listed in the Acknowledgments
‡Present Address: Francisco J. Salguero, National Infection Service, Public Health England, Salisbury, United Kingdom
Disclaimer: All claims expressed in this article are solely those of the authors and do not necessarily represent those of their affiliated organizations, or those of the publisher, the editors and the reviewers. Any product that may be evaluated in this article or claim that may be made by its manufacturer is not guaranteed or endorsed by the publisher.
Research integrity at Frontiers
Learn more about the work of our research integrity team to safeguard the quality of each article we publish.