- 1Department of Nutrition and Clinical Nutrition, Faculty of Veterinary Medicine, Zagazig University, Zagazig, Egypt
- 2Environment and Life Sciences Research Center, Kuwait Institute for Scientific Research, Kuwait, Kuwait
- 3Department of Clinical Laboratory Sciences, Turabah University College, Taif University, Taif, Saudi Arabia
- 4Department of Physiology, Faculty of Veterinary Medicine, Zagazig University, Zagazig, Egypt
- 5Department of Clinical Pathology, Faculty of Veterinary Medicine, Zagazig University, Zagazig, Egypt
Improving the nutritional quality of unconventional feed ingredients such as fava bean by-products can enhance their utilization by broiler chickens. Hence, the quality of fermented fava bean by-products (FFB), in addition to growth, nutrient digestibility, digestive enzyme, and intestinal barrier-related gene expression, and serum biochemical and immunological parameters were evaluated in response to different levels of FFB. A total of 500 1-day-old broiler chicks (46.00 ± 0.388 g) were allocated to five groups with 10 replicates each (100 chicks per treatment). The first group was fed a corn–soybean diet (control diet), and the other four groups were fed a diet containing 5, 15, 25, and 35% FFB for 38 days. Birds fed 25% FFB exhibited maximum body weight gain (increase by 12.5%, compared with the control group) and the most improved feed conversion ratio. Additionally, birds fed FFB at 15, 25, and 35% showed improved dry matter and crude protein digestibility. Moreover, birds fed FFB at 25 and 35% exhibited a decrease in ileal pH and an increase in fiber digestibility (p < 0.05). Upregulation of digestive enzyme genes (AMY2A, PNLIP, and CCK) was observed in groups fed with FFB. The most prominent upregulation of genes encoding tight junction proteins (claudin-1, occludin, and junctional adhesion molecules) in the duodenum was observed in chicks fed 25 and 35% FFB (increase of 0.66-, 0.31-, and 1.06-fold and 0.74-, 0.44-, and 0.92-fold, respectively). Additionally, the highest expression level of enterocyte protective genes [glucagon-like peptide (GLP-2), mucin-2 (MUC-2), and fatty acid-binding protein (FABP-6)] was detected in duodenum of chicks fed high levels of FFB. Substitution of corn–soybean diet with FFB had an inhibitory effect on cecal pathogenic microbes (Escherichia coli and Clostridium perfringens) and increased beneficial microflora (Lactobacilli and Bifidobacterium), especially at high levels. Additionally, an increase was observed in IgM and lysozyme activity, with no effect on IgA in all groups fed FFB. All levels of FFB decreased cholesterol levels. Based on our results, we concluded that substitution of corn–soybean diet with FFB can improve the growth rate and nutrient digestibility of broiler chickens, enhance their intestinal barrier functions, and increase the number of beneficial microorganisms. Using FFB at 25% had a positive effect on the growth performance of broiler chickens, and it could be utilized in poultry farms.
Introduction
Increasing demand for conventional or basic feed ingredients such as corn and soybean meal (SBM) in the biofuel industry and poultry ration leads to increased costs, which encourages poultry nutritionists to search for low-cost unconventional feed alternatives and locally cultivated food crops, especially from high-protein legumes (1, 2). Supplementation of poultry feed with native legumes and their by-products can offer additional protein, energy, and minerals that improve animal productivity (3, 4). Fava beans are an alternative legume that can partially replace SBM as a protein source (5). Previously, feeding of broiler chickens on green beans processing by-products with enzymes significantly enhanced their performance up to 16% (6). Fava beans contain a high level of proteins (~26%), carbohydrates (up to 77%), dietary fiber, niacin, folic acid, and vitamin C (7). Bean crops' by-products comprise stems, empty or partly filled pod with seeds, and leaves that vary in composition according to the ratio of each, with the highest crude protein content found in seeds and leaves (~22–25%) (8). Regardless of its high nutritional value, utilization of fava beans and their by-products as an alternative to conventional feed ingredients in poultry is limited because of the presence of many anti-nutritional factors (ANFs) (9), such as vicine, convicine, tannins, protease inhibitors, oligosaccharides, and non-starch polysaccharides (NSPs). Additionally, they have a low content of sulfur-containing amino acids that reduce their nutritional value, has a negative effect on nutrient digestibility, promotes pathogen proliferation, and reduces overall animal performance (10, 11). Various processing methods including soaking, boiling, germination, roasting, autoclaving, microwaving, micronization, and fermentation can be utilized to counteract negative effects of these ANFs (12). Solid-state fermentation with the aid of beneficial bacteria and fungi can utilize agricultural by-products as substrates for NSP-degrading microorganisms and convert them to nutritive feed ingredients (13, 14). Thus, using such an approach can improve the nutritional properties of original products (15), increase nutrient bioavailability (16), and remove undesirable components from legumes and other feed constituents (17). Moreover, microbial fermentation can enhance the proportion of digestible phosphorus, increase protein concentration and digestibility (18), and improve fiber digestibility (19). Moreover, this process helps enrich the raw material with vitamins and minerals and increase the effective release of methionine, lysine, threonine, and small peptides (20, 21). Lactic acid bacteria (LAB) including Lactobacillus, Streptococcus, Pediococcus, and Leuconostoc are important because of their unique organoleptic properties (22). Previous studies have shown that fermented soybeans, soybean by-products, and rapeseed meal have a positive effect on broiler chicken performance (21, 23). However, the influence of fermentation on the nutritional value of fava bean by-products lacks sufficient support, and there is a lack of information on the effect of fermented fava bean by-products (FFB) in broilers. Accordingly, the objective of this study was to elucidate the effects of substitution of corn–soybean diets with different levels (5, 15, 25, and 35%) of FFB on growth performance, nutrient digestibility, digestive and intestinal barrier gene expressions, cecal microbial population, and serum biochemical and immunological parameters in broiler chickens.
Materials and Methods
Preparation of FFB
Fungal and bacterial strains including Lactobacillus acidophilus (PTCC1643), Bacillus subtilis (PTCC1156), Lactobacillus plantarum (PTCC1058), and Aspergillus oryzae (PTCC5163) were used for fermentation. Each kilogram of fava bean by-products (stem, leaf, and empty or poorly filled pod by-products) as fermentation substrate was inoculated and mixed with 1 L distilled water containing 106 spores/ml of A. oryzae and 108 CFU/ml of L. acidophilus, B. subtilis, and L. plantarum in fermentation tanks fitted with a one-way valve to allow leakage of produced gases and obstructed air from entry for 7 days. The fermented samples were then dried at 50°C for 2 days. Dried samples were ground and mixed with other feed ingredients, and chemical analysis of fermented and unfermented fava beans was performed according to Latimer (24) (Table 1).
Study Animals
Five hundred 1-day-old male Ross-308 broiler chicks were obtained from a local hatchery and were weighed on arrival (46.00 ± 0.388 g). Chicks were reared in a naturally ventilated open house with sawdust as litter. Lighting, room temperature, and relative humidity were monitored according to the recommendations of Ross-308 management (25). All animal experiments were conducted following the guidelines defined in “The Guide for the Care and Use of Laboratory Animals in Scientific Investigations” and were approved by the Institutional Ethics Committee at Nutrition, Clinical Nutrition and Animal Wealth departments, Faculty of Veterinary Medicine, Zagazig University, Egypt.
Experimental Design and Diets
Broiler chicks were randomly assigned to five groups (100 chicks per group), with 10 replicates each and 10 birds per replicate. The treatment groups received a basal corn–soybean diet (control) or a diet supplemented with 5, 15, 25, and 35% FFB. The experimental period was 38 days. All chicks were allowed free access to feed and water. All experimental diets were provided in mash and formulated according to the Ross Manual Guide (25), as presented in Table 2. Proximate analysis of different nutrients [dry matter (DM), crude protein (CP), crude fiber (CF), and ether extract (EE)] in feed ingredients and diets was performed according to the standard methods of the AOAC (26).
Growth Performance
Body weights (BWs) of chicks of each replicate were determined at 1, 23, and 38 days. The average feed intake (FI) per individual in each replicate was calculated as the difference between provided feed weight and remaining feed weight, which was then divided by the number of chicks in each replicate. At each time interval, BW gain (BWG) was estimated as the difference between final and initial BW. The feed conversion ratio (FCR) was calculated during the starter, grower, finisher, and overall phases (days 1–38) [FI (g/bird)/weight gain (g/bird)].
For apparent digestibility of nutrients, TiO2 was used as an indigestible marker (3 g) and was added to each experimental diet. Chicken excreta were collected for 7 days, dried at 65°C for 72 h, and dry matter, crude protein, ether extract, and crude fiber were analyzed according to the Association of Official Agricultural Chemists (26). The TiO2 content in diet and excreta was calculated after acid digestion according to the method of Short et al. (27). The calculation was performed as follows: apparent nutrient digestibility = 100 – [100 × (indicator content (diet)/indicator content (feces) × nutrient content (feces)/nutrient content (diet)] (28).
Sample Collection
At the end of the experiment, chicks were killed by cervical dislocation (29), de-feathered, eviscerated, and weighed, after which dressing percentages were determined. Abdominal fat weight was determined and expressed as a percentage of the live BW. Blood samples (n = 10 per group) were collected from 10 randomly selected chicks of each replicate. Blood samples from the brachial vein were placed in dry sterilized tubes without anticoagulant and centrifuged at 3,000 rpm for 5 min for serum collection, for further clinico-biochemical analysis.
Pancreatic tissues (n = 10 per group) were collected for quantification of pancreatic enzyme-related genes [alpha 2A amylase genes (AMY2A) and lipase (PNLIP)]. For molecular analysis of cholecystokinin (CCK), tight junction proteins (TJPs) (occludin, junctional adhesion molecules, and claudin-1), and gut protective genes [mucin-2 (MUC-2), fatty acid-binding protein (FABP-6), glucagon-like peptide (GLP-2)], duodenal samples (n = 10 per group), ~3 cm from the distal loop, were separated, and digesta was squeezed out from it and rinsed three times in phosphate-buffered saline (PBS). After that, all molecular samples were kept in TRI reagent at −80°C until real-time polymerase chain reaction (PCR) analysis.
Clinico-Biochemical Analysis
The serum biochemical indices triglycerides (TAG), total cholesterol (TC), high density lipo-protein (HDL), low-density protein (LDL), alanine aminotransferase (ALT), and aspartate amino transferase (AST) were quantified using diagnostic kits (Spinreact, Santa Coloma, Spain). Serum lysozyme concentrations were measured according to the method of Lie et al. (30). The concentrations of immunoglobulins (IgA and IgM) were determined using ELISA kits for chickens (ABCAM Co. UK, cat. no. AB157692), according to the manufacturer's instructions.
RNA Extraction and Reverse-Transcription PCR
RNA was isolated from pancreatic and duodenal tissues (~3 cm from the distal loop) using the QIAamp RNeasy Mini Kit (Qiagen GmbH, Hilden, Germany). RNA concentration was measured using a NanoDrop™ 2000 spectrophotometer (Thermo Fisher Scientific Inc., Waltham, MA, USA) at an optical density of 260 nm.
With the use of SYBR Green for RT-PCR, the amplifications of PCR were achieved in 25-μl reactions containing 0.25 μl RevertAid reverse transcriptase (Thermo Fisher Scientific, Germany), 12.5 μl 2× QuantiTect SYBR Green PCR Master Mix (Qiagen), 0.5 μl of each primer, 8.25 μl RNase-free water, and 3 μl of the RNA template. Real-time PCR amplification was performed using a Rotor-Gene Q2 plex (Qiagen Inc., Valencia, CA, USA). The primer sequences of digestive enzymes (AMY2A, PNLIP, and CCK), TJPs (occludin, junctional adhesion molecules, and claudin-1), and gut protective genes (MUC-2, FABP-6, and GLP-2) (31, 32) are listed in Table 3. GAPDH was used as an internal control to normalize the target gene expression levels.
Bacteriological Assay
At the end of the experimental period, the spread plate technique was used for counting selected microbes in cecal contents. Serial 10-fold dilution from 1 g of cecal content (n = 10 per group) was prepared in sterile saline. De Man, Rogosa, and Sharpe (MRS, CM1153, Oxoid, UK) agar medium was utilized for Lactobacilli counting, Bifidus selective agar was exploited to verify the Bifidobacterium population (BSM agar, 88517, Sigma, St. Louis, MO, USA). For counting Escherichia coli, violet-red bile glucose agar (VRBG, CM485, Oxoid) was used. Following incubation of bacteria Lactobacilli and Bifidobacteria under anaerobic conditions for 72 h at 37°C and E. coli under aerobic conditions for 48 h at 39°C, colonies were enumerated on the plates and expressed as log10 CFU/g of cecal content.
Statistical Analysis
Data were analyzed using the general linear model (GLM) procedure of SPSS (SPSS Inc., Chicago, Illinois, USA) after confirming the homogeneity among experimental groups using Levene's test and normality using Shapiro–Wilk's test. The significant difference between the mean values was tested using Tukey's test, and the variation in the data was expressed as the standard error of the mean (SEM). The significance level was set at 0.05. Relative fold changes in the expression of target genes were calculated by the 2−ΔΔCt method (33).
Results
Chemical Analysis of Unfermented FFB and FFB
Crude protein and fat contents were significantly increased (p < 0.05) after fermentation of fava bean by-products; however, crude fiber, lignin, tannins, saponins, and cyanogenic glycosides significantly decreased (p < 0.05) in fermented fava beans compared to UFFB (Table 1).
Growth Performance
Growth performance parameters of the broilers are presented in Table 4. During the starter period, substitution of corn–soybean diet with 5, 15, 25, and 35% FFB had no effect on BW and BWG, whereas substitution with 15 and 25% FFB decreased (p < 0.05) FI and FCR, compared to the corn–soybean control diet. Throughout the grower period, broilers fed 15, 25, and 35% FFB showed an increase (p < 0.05) in BWG, whereas broilers fed 5% FFB did not exhibit a significant difference in BWG, compared to the control treatment. Moreover, FI was significantly (p < 0.05) decreased in the 15% FFB group. The FCR was significantly improved (p < 0.05) in broilers fed 15 and 25% FFB-substituted diets. During the finisher period, the use of different proportions of FFB increased (p < 0.05) BWG and FI and improved FCR. Overall performance results showed that the most significant BWG and lowest FCR occurred in the group fed 25% FFB.
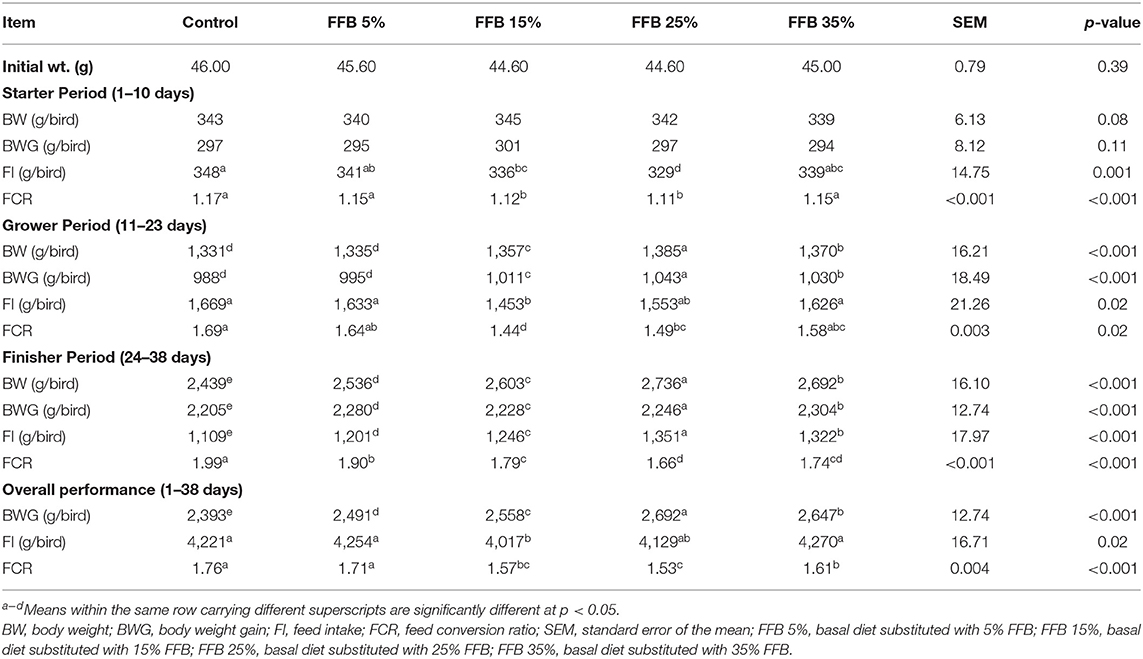
Table 4. The effect of substitution of corn–soybean diet with FFB on the growth performance parameters of broiler chickens.
Carcass Traits and Nutrient Digestibility
Data regarding the effect of FFB on dressing percentage and nutrient digestibility are shown in Table 5. The dressing percentage increased (p < 0.05), whereas the percentage of abdominal fat decreased (p < 0.05) in all experimental treatments. Substitution of the corn–soybean diet with 25 and 35% FFB significantly decreased the ileal pH. Nutrient digestibility of dry matter and crude protein was significantly (p < 0.05) increased in groups fed 15, 25, and 35% FFB, whereas the nutrient digestibility of crude fiber was increased (p < 0.05) in groups fed 25 and 35% FFB.
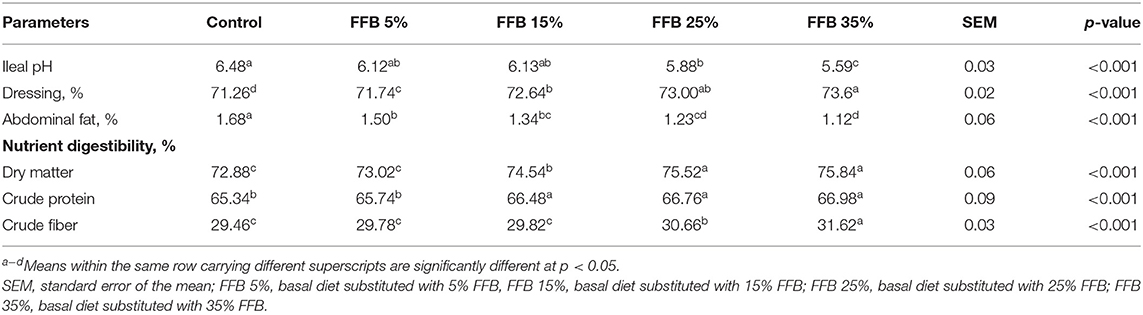
Table 5. The effect of substitution of corn–soybean diet with FFB on some carcass traits and nutrient digestibility of broiler chickens.
Expression of Intestinal Barrier, Gut Protective, and Digestive Enzyme Genes
mRNA expressions of genes encoding occludin, junctional adhesion molecules (JAM), and claudin were significantly upregulated (p < 0.05) in the duodenum with increasing levels of fermented FFB. The most prominent upregulation was observed in the 35% FFB group (increased by 0.74-, 0.44-, and 0.92-fold, respectively, vs. the control group) (Figure 1). Feeding broiler chickens with higher substitution levels of FFB (25% and 35%) significantly upregulated (p < 0.05) the expression of GLP-2 and FABP genes, compared with the control group. Moreover, the group fed 35% FFB showed the most significant level of MUC-2 (Figure 2). mRNA expression of the AMY2A gene was significantly upregulated (p < 0.05) in all groups fed FFB, whereas increasing the inclusion levels from FFB significantly upregulated PNLIP and CCK gene expressions (Figure 3).
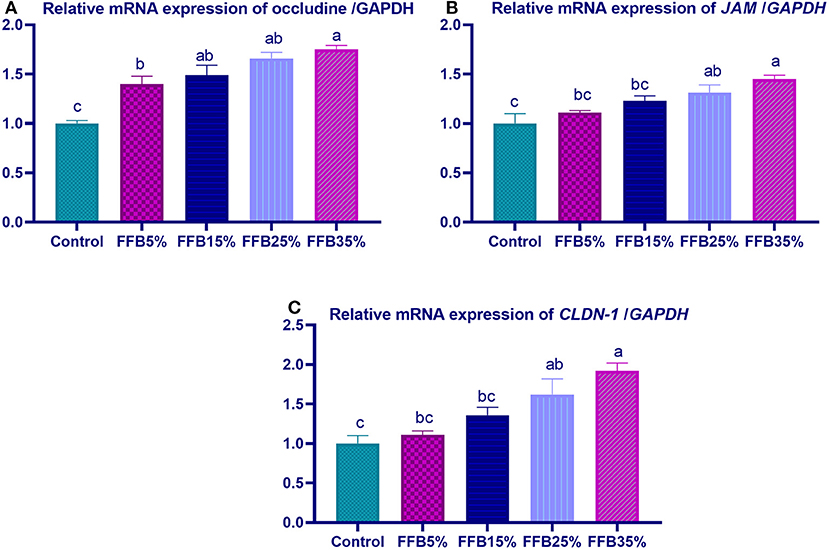
Figure 1. The effect of substitution of corn–soybean diet with fermented fava beans by-products on the expression of occludine (A), junction adhesion molecule (B; JAM), and Claudin-1 (CLDN-1; C) in the duodenum. FFB5% (basal diet substituted with 5% fermented fava beans by-products), FFB15% (basal diet substituted with 15% fermented fava beans by-products), FFB25% (basal diet substituted with 25% fermented fava beans by-products), FFB35% (basal diet substituted with 35% fermented fava beans by-products). a−cMeans within the same column carrying different superscripts are significantly different at p < 0.05.
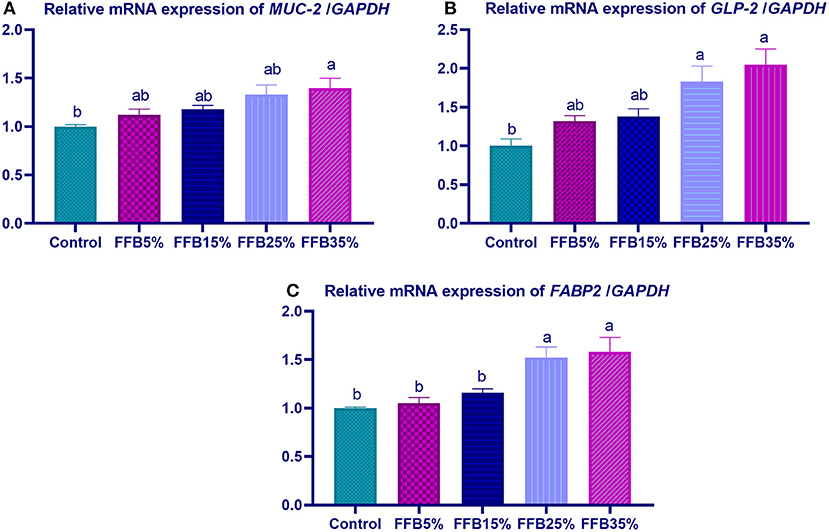
Figure 2. The effect of substitution of corn-soybean diet with fermented fava beans by-products on the expression of mucin-2 (MUC-2; A), glucagon-like peptide (GLP-2; B) and fatty acid binding proteins (FABP2; C) in the duodenum. FFB5% (basal diet substituted with 5% fermented fava beans by-products), FFB15% (basal diet substituted with 15% fermented fava beans by-products), FFB25% (basal diet substituted with 25% fermented fava beans by-products), FFB35% (basal diet substituted with 35% fermented fava beans by-products). a, bMeans within the same column carrying different superscripts are significantly different at p < 0.05.
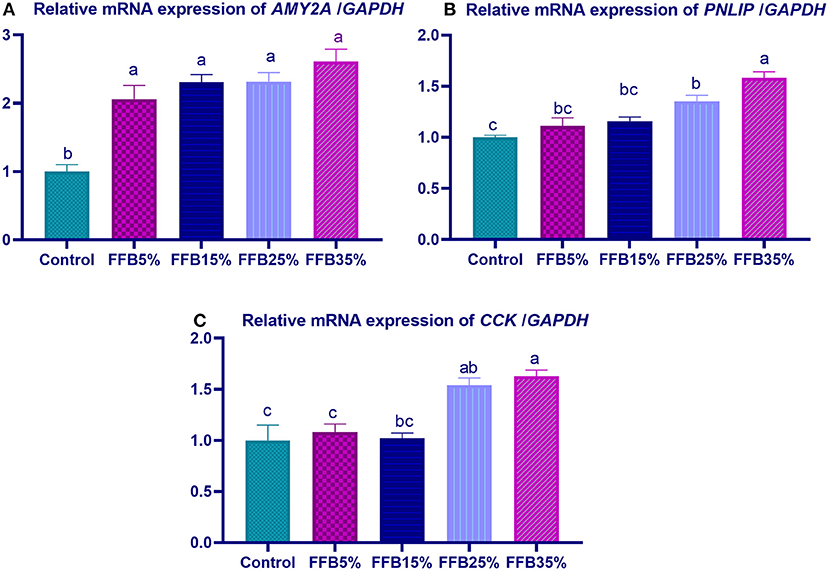
Figure 3. The effect of substitution of corn–soybean diet with FFB on the expression of pancreatic alpha 2A amylase (AMY2A; A) and lipase (PNLIP; B) genes in the pancreas and cholecystokinin (CCK; C) gene in in duodenum. FFB 5% (basal diet substituted with 5% FFB), FFB 15% (basal diet substituted with 15% FFB), FFB 25% (basal diet substituted with 25% FFB), FFB 35% (basal diet substituted with 35% FFB). a−cMeans within the same column carrying different superscripts are significantly different at p < 0.05.
Cecum Microbes
Table 6 shows that substitution of corn–soybean diet with different levels of FFB significantly (p < 0.05) increased the abundance of Bifidobacterium spp. and decreased (p < 0.05) Clostridium perfringens counts, compared with the controls. Lactobacillus spp. significantly (p < 0.05) increased groups fed 25% or 35% FFB diets. E. coli counts were significantly decreased (p < 0.05) in groups fed 15, 25, or 35% FFB.
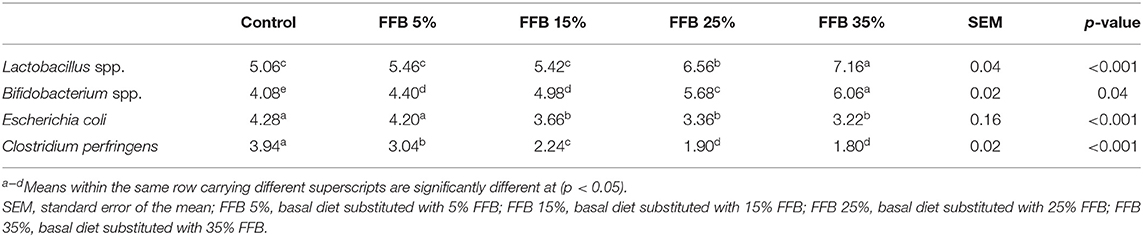
Table 6. The effects of substitution of corn–soybean diet with FFB on cecal beneficial and pathogenic microorganisms (log10 CFU/g fresh digesta).
Serum Biochemical and Immunological Parameters
The effects of FFB on liver enzymes, the lipid profile, and immunological parameters of broiler chickens are presented in Table 7. Compared to the control, different substitution levels of FFB had no effect on AST, ALT, uric acid, and creatinine (p > 0.05). Additionally, substitution of corn–soybean diet with different levels of FFB significantly decreased (p < 0.05) cholesterol and increased (p < 0.05) HDL. TAG and VLDL decreased (p < 0.05) in broilers fed diets substituted with 15, 25, and 35% FFB. Groups fed different levels of FFB showed an increase in IgM (p < 0.05); however, they showed no effect (p > 0.05) on IgA. Moreover, lysozyme activity increased (p < 0.05) in broilers fed diets containing FFB.
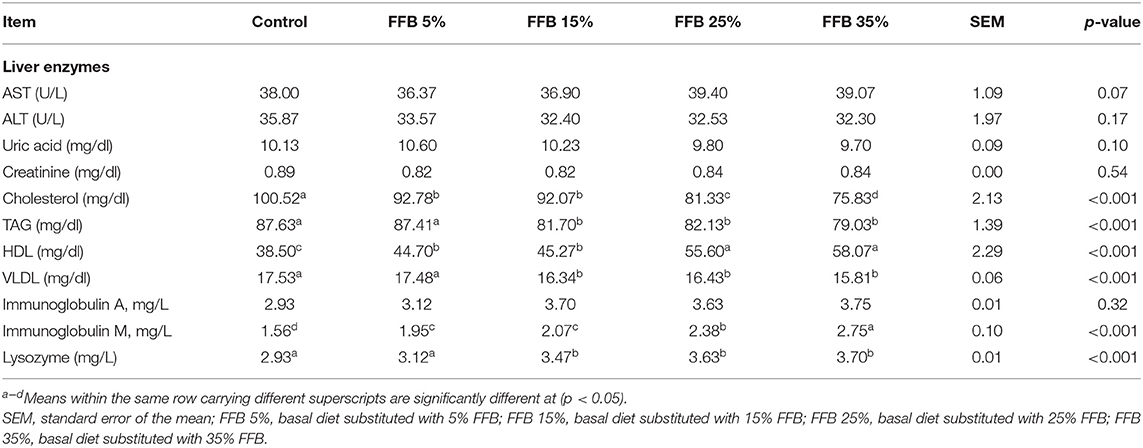
Table 7. The effect of substitution of corn–soybean diet with FFB on some serum biochemical and immunological parameters at 38 days.
Discussion
This study examined the application of microbial fermentation to an unconventional feed, fava bean by-products, and showed that it could be considered a successful tool to decrease ANFs and enhance their nutritive value. These beneficial effects may be related to the enrichment of feed with fermentation products such as probiotics and other bioactive functional ingredients. The benefit of using A. oryzae was the removal of oxygen from the fermentation media and achieving anaerobic conditions for L. acidophilus, B. subtilis, and L. plantarum growth and development. Once these bacteria were activated, the conditions for growth and proliferation of LAB were improved (34) with a subsequent reduction in feed pH that reduces viability and growth of pathogens. The pH of fermented FFB was 1.5-fold lower than that of unfermented fava beans. Previous studies have shown similar outcomes of decreased pH and increased LAB populations in fermented products (35, 36). Additionally, in our study, FFB had higher concentrations of crude protein and fat and lower levels of crude fiber and ANFs such as lignin, tannins, saponins, and cyanogenic glycosides, compared to raw fava bean by-products. Ashayerizadeh et al. (37) reported that fermentation of rapeseed meal with L. acidophilus, B. subtilis, and Aspergillus niger significantly reduced ANFs such as glucosinolates, phytic acid, total tannin, and phenolic compounds. Similarly, it was found that fermentation improved the nutritional quality of legumes by increasing crude protein and reducing crude fiber content (38, 39). The increased protein content in FFB may arise from proteases produced from some microbial strains during fermentation, which decompose proteins to peptides and free amino acids in fermented products (40). Additionally, an increase in protein and fat content may be caused by a decrease in carbohydrate content during fermentation; subsequently, microorganisms can consume carbon and energy to produce microbial proteins (17). Moreover, higher crude protein content may also be derived from microbial protein synthesis accompanied by an increased microbial population at the time of fermentation (37, 41). Moreover, a reduction in fiber content after fermentation was caused by production of fiber-degrading enzymes (42), reducing lignin and indigestible polyphenolic constituent levels (43) and decreasing NDF content (44). The decrease in ANFs in fava beans in the current study may be attributed to fungal and microbial enzymes degrading these compounds, which is in accordance with Soumeh et al. (45) who described similar benefits from microbial fermentation of SBM. Lactobacillus and B. subtilis have been described to produce phytase, xylanase, cellulase, and glucanase enzymes (46, 47), which are responsible for degradation of non-beneficial components (48). Thus, subjecting legume feeds such as fava beans to fermentation may be a sound alternative to improve the nutritive value of legumes. Moreover, the results of growth performance parameters indicated that feeding fermented fava beans with improved nutritional value had a positive effect on BWG and FCR of broiler chickens, which allowed its application to formulated diets up to 35%. Furthermore, the maximum BWG and feed efficiency were observed in the group fed FFB at 25%. Overall improvement in broiler performance may be due to the improvement in nutritional quality of fava beans and nutrient apparent digestibility, activity of gut protective and digestive enzyme gene expressions, gut microbiology, and immunity of broiler chickens. The improvement effect of FFB on growth performance of broilers may be attributed to the lowering of ANFs in legumes after fermentation and increasing nutrient absorption and utilization (49). Similar studies have shown that microbial fermentation is an efficient process to eliminate ANFs and tannins in legume feed, thereby enhancing the nutritional quality and improving the performance of broilers (50). With fermentation, fava bean oligosaccharides and NSPs become more available for cecal microbes that facilitate their digestion (51). Substitution of the diet with 25 and 35% FFB significantly decreased ileal pH, because the fermentation process was associated with the production of organic acids and enhanced growth of LAB, leading to reduced gastric pH (34). Similarly, Drazbo et al. (44) reported that feeding turkeys fava beans led to lower ammonia levels in cecal digesta and lowered the pH of the intestinal digesta. Higher abundance of probiotic lactobacilli and bifidobacterial counts in the ileum was in accordance with the findings of Yamauchi and Suetsuna (52), who showed that fermented SBM (FSBM) increased the populations of yeasts, LAB, and Bacillus, which can improve the microecology balance and health of broiler gut and their growth performance.
Additionally, reduced abundance of enteric pathogenic bacteria including C. perfringens and E. coli after feeding on higher levels of FFB may be related to the higher concentration of organic acids associated with the fermentation process, which enhanced LAB growth and proliferation, leading to reduced gastric pH, thereby inhibiting pathogen growth (34). Yin et al. (53) confirmed that FSBM feed increased the number of beneficial microbes and inhibited pathogen proliferation, and a more acidified environment in the intestine promotes proliferation of more desirable microbial taxa. Fermented feed inhibits growth of pathogenic bacteria and increases the population of desirable microbes by reducing the pH of the digestive tract (34, 54). Fermentation increases the efficiency of feed and improves the growth rate by controlling the growth of non-pathogenic and pathogenic microbes in the intestinal tract of broilers (55).
Nutrient digestibility of dry matter and crude protein was increased in all groups fed different levels of FFB, except for the 5% FFB-fed group, whereas nutrient digestibility of crude fiber increased with increasing levels of FFB (25 and 35%). In accordance with our results, dietary supplementation with FSBM increased crude protein utilization in fodder and reduced the molecular size of peptides (56). Fermentation has also been shown to enhance the digestibility of different nutrients such as organic matter, nitrogen, amino acids, and fiber (57). Additionally, during L. acidophilus fermentation of fava bean by-products, a significant amount of proteases, phytases, amylases, and β-glucanases can be generated and activated and improve the nutrient digestibility in animals (58). Jeong et al. (59) demonstrated that FSBM supplementation improved nutrient digestibility and productive performance of pigs. Moreover, fermentation with B. subtilis can improve the taste of feed, secrete digestive enzymes, stimulate digestion and absorption of nutrients (60), and produce bacitracin, polymyxin, nystatin, and gramicidin, which inhibit pathogen growth (61). Addition of FSBM to feed increased the activity of digestive enzymes (trypsin, lipase, and protease), improved the FCR and growth of weaned piglets (62), and increased the average daily gain (ADG) of finishing pigs (63). Similarly, using fermented feed, such as SBM, improved the morphological parameters of the intestine, increased nutrient absorption, and increased BWG in broilers (64). Additionally, Usayran et al. (65) found that broilers fed 30% tannin-free bean diets had better weight gain and FCRs than those fed a soybean control diet. Additionally, substituting SBM with fava beans had no adverse effect on the growth performance of guinea fowl broilers and broiler chickens (66, 67). Farrell et al. (68) showed that fava beans can be added at up to 36% in broiler diets, where they partially replaced SBM without reducing bird performance. Moreover, Chachaj et al. (64) found that feeding turkeys 9 or 10% FSBM resulted in increased BWG, compared to the control group.
In line with the results of growth performance and nutrient digestibility, expression of digestive enzyme genes (amylase, lipase, and cholecystokinin) was upregulated after 38 days of feeding FFB. Moreover, with increasing levels of FFB, expression of these enzymes was more prominent. Similarly, activities of pancreatic enzymes in broilers were enhanced after feeding on FSBM (45). Microbial fermentation of cottonseed meal with the aid of B. subtilis has been shown to increase the activities of amylase and protease enzymes (69), which may result from B. subtilis, contributing to the production of protease and amylase enzymes. Moreover, increasing the consumption of carbohydrates can enhance mRNA expression levels of glucose transmitters, thereby increasing glucose absorption (70). Accordingly, feeding broiler chickens with higher levels of microbially fermented dried brewer grain enhanced pancreatic gene expression (amylase, protease, and lipase) and GLUT2 expression (71). Additionally, Lee et al. (72) showed that B. subtilis-based supplemented feed upregulated pancreatic lipase and carboxypeptidase genes in the gut.
Tight junctions (TJs), intercellular junctional complexes, consist of unique proteins including occludin, claudin, and JAM, which maintain epithelial cell integrity, allow nutrient transportation, and represent a barrier between the lumen and the host to inhibit bacterial invasion (73, 74). TJ disruption may impair intestinal function, triggering gut leaking, which increases intestinal permeability and leads to systemic bacterial invasion, affecting animal health and growth performance (75, 76). The MUC-2 gene is expressed by goblet cells, which form a mucus layer that prevents pathogen invasion, along with TJs (77). In the current study, maintaining the integrity of the intestinal barrier was evidenced by elevated TJPs and MUC-2 expression after feeding FFB. These results are in agreement with those of Lin and Lee (78), who reported that feeding Laetiporus sulphureus-fermented products elevated zonula occludens-1, claudin-1, and mucin-2 expression. Fermented feeds were reported to enhance the intestinal barrier and immune function in poultry (79). Herein, improving the function of TJs may be attributed to the production of fermentation bioactive components such as oligosaccharides, isoflavones, and peptides, which protect intestinal cells and support their recovery (80). In addition to the presence of probiotic bacteria in fermented feed, TJ integrity and mucus secretion occurred in the gastrointestinal tract of broiler chicks. Moreover, dietary supplementation with B. subtilis and L. plantarum elevated mRNA expression of barrier function-related genes in broiler intestines (81, 82). In the current study, substitution of corn–soybean diet with 5, 15, 25, and 35% FFB significantly decreased the abdominal fat percentage, while increasing the dressing percentage. Similarly, in previous reports, abdominal fat percentage was decreased by inclusion of 30% fava beans (65).
In the present study, substitution of corn–soybean control diet with fermented fava beans decreased the concentration of cholesterol and TAG, while increasing the concentration of HDL. Meanwhile, no considerable alterations were detected in AST, ALT, uric acid, and creatinine among different experimental groups. These results were consistent with those of Usayran et al. (65), who found that feeding on 30% tannin-free fava bean diets decreased the concentrations of cholesterol and TAG in broiler chicks, with no effect on AST, unlike the control diet. Additionally, Moschini et al. (83) found that AST, ALT, and plasma urea concentrations of birds fed 25 or 50% FFB were similar to those fed corn–SBM diets. High levels of HDL were found in the serum of turkeys fed 7 or 9% FSBM (64). Moreover, pigs fed FSBM diets had significantly lower creatinine concentrations than those fed control diets (63). The reduction of cholesterol and TAG levels after feeding on FFB may be attributed to the role of probiotic produced during fermentation in inhibiting 3-hydroxy-3-methylglutaryl CoA reductase enzyme incorporated in cholesterol biosynthesis (84).
In our study, the use of FFB in chicken diets significantly improved the immune response, which was represented by an increased concentration of IgM and lysozyme activity. Fermented feed can affect immune responses, which may be caused by the high content of LAB and bioactive peptides, as well as antioxidant compounds, compared to unfermented meals (85), and live microbes in fermented meals may act as probiotics and improve the humoral immune response of birds (36). In addition to bacteria in fermented feed producing lactic and acetic acid, which creates an acidic environment at pH 4, acidic molecules can penetrate cell membranes of bacteria and increase their acidity, which interferes with enzymatic processes and kills the bacteria (86). Our results were in line with those of Chachaj et al. (64), who reported that feeding turkeys FSBM at 7, 9, or 10% increased IgM levels, with no effect on IgA. Dietary supplementation with FSBM also plays an important role in relieving diarrhea and generating immune-related effectors, such as IgA and haptoglobin (87). In addition to Feng et al. (62), Fazhi et al. (88) showed that FSBM elevated the levels of serum IgA and IgM in broiler chickens and ducks. Additionally, FSM lowers the level of soy allergens (glycinin, β-conglycinin, and trypsin inhibitors) and reduces the risk of food hypersensitivity reactions (89).
Conclusion
Application of microbial fermentation, as novel processing technologies, for non-conventional feed resources such as fava bean by-products can enhance their nutritional value and utilization. Herein, feeding of broiler chickens on FFB can promote their growth performance by boosting digestive and intestinal barrier functions. These findings encourage the poultry feed industry to recommend FFB as an alternative nutritious unconventional feed ingredient, consequently minimizing the dependence on conventional feed sources and ensuring profitable broiler production.
Data Availability Statement
The original contributions presented in the study are included in the article/supplementary material, further inquiries can be directed to the corresponding author/s.
Ethics Statement
The animal study was reviewed and approved by Institutional Animal Care and Use Committee of Zagazig University, Egypt.
Author Contributions
All authors shared in the study design, methodology, data collection and analysis, statistical analysis, and writing of the manuscript.
Funding
This study was supported by Taif University researchers supporting project number (TURSP-2020/134), Taif University, Taif, Saudi Arabia.
Conflict of Interest
The authors declare that the research was conducted in the absence of any commercial or financial relationships that could be construed as a potential conflict of interest.
Acknowledgments
The authors would like to thank Taif University researchers supporting project number (TURSP-2020/134), Taif University, Taif, Saudi Arabia.
References
1. Crépon K. Nutritional value of legumes (pea and faba bean) and economics of their use. Recent Adv Anim Nutr. (2007) 2006:331–66. doi: 10.5661/recadv-06-331
2. Ravindran G, Nalle CL, Molan A, Ravindran V. Nutritional and biochemical assessment of field peas (Pisum sativum L.) as a protein source in poultry diets. J Poult Sci. (2010) 47:48–52. doi: 10.2141/jpsa.009071
4. Abdelnour SA, El-Hack A, Mohamed E, Ragni M. The efficacy of high-protein tropical forages as alternative protein sourcesfor chickens: a review. Agriculture. (2018) 8:86. doi: 10.3390/agriculture8060086
5. Nalle C, Ravindran V, Ravindran G. Nutritional value of faba beans (Vicia faba L.) for broilers: apparent metabolisable energy, ileal amino acid digestibility and production performance. Anim Feed Sci Technol. (2010) 156:104–11. doi: 10.1016/j.anifeedsci.2010.01.010
6. Abbel-Monein MA. Effect of using green beans processing by-products with and without enzyme supplementation on broilers performance and blood parameters. J Agrobiol. (2013) 30:43. doi: 10.2478/agro-2013-0005
7. Hayat I, Ahmad A, Masud T, Ahmed A, Bashir S. Nutritional and health perspectives of beans (Phaseolus vulgaris L.): an overview. Crit Rev Food Sci Nutr. (2014) 54:580–92. doi: 10.1080/10408398.2011.596639
8. Ujowundu C, Kalu F, Emejulu A, Nkwonta C, Nwosunjoku E. Evaluation of the chemical composition of Mucuna utilis leaves used in herbal medicine in Southeastern Nigeria. Afr J Pharm Pharmacol. (2010) 4:811–6.
9. Teguia A, Fru SF. The growth performances of broiler chickens as affected by diets containing common bean (Phaseolus vulgaris) treated by different methods. Trop Anim Health Prod. (2007) 39:405–10. doi: 10.1007/s11250-007-9028-y
10. Lai L, Lee M, Chen C, Yu B, Lee T. Effects of co-fermented Pleurotus eryngii stalk residues and soybean hulls by Aureobasidium pullulans on performance and intestinal morphology in broiler chickens. Poult Sci. (2015) 94:2959–69. doi: 10.3382/ps/pev302
11. Hejdysz M, Kaczmarek S, Rutkowski A. Extrusion cooking improves the metabolisable energy of faba beans and the amino acid digestibility in broilers. Anim Feed Sci Technol. (2016) 212:100–11. doi: 10.1016/j.anifeedsci.2015.12.008
12. Khattab R, Arntfield S, Nyachoti C. Nutritional quality of legume seeds as affected by some physical treatments, part 1: protein quality evaluation. LWT Food Sci Technol. (2009) 42:1107–12. doi: 10.1016/j.lwt.2009.02.008
13. Hölker U, Höfer M, Lenz J. Biotechnological advantages of laboratory-scale solid-state fermentation with fungi. Appl Microbiol Biotechnol. (2004) 64:175–86. doi: 10.1007/s00253-003-1504-3
14. Lee M, Lin W, Lin L, Wang S, Chang S, Lee T. Effects of dietary Antrodia cinnamomea fermented product supplementation on antioxidation, anti-inflammation, and lipid metabolism in broiler chickens. Asian Austr J Anim Sci. (2020) 33:1113. doi: 10.5713/ajas.19.0392
15. Frias J, Song YS, Martínez-Villaluenga C, De Mejia EG, Vidal-Valverde C. Immunoreactivity and amino acid content of fermented soybean products. J Agric Food Chem. (2008) 56:99–105. doi: 10.1021/jf072177j
16. Hotz C, Gibson RS. Traditional food-processing and preparation practices to enhance the bioavailability of micronutrients in plant-based diets. J Nutr. (2007) 137:1097–100. doi: 10.1093/jn/137.4.1097
17. Mukherjee R, Chakraborty R, Dutta A. Role of fermentation in improving nutritional quality of soybean meal—a review. Asian Austr J Anim Sci. (2016) 29:1523. doi: 10.5713/ajas.15.0627
18. Teng D, Gao M, Yang Y, Liu B, Tian Z, Wang J. Bio-modification of soybean meal with Bacillus subtilis or Aspergillus oryzae. Biocatal Agric Biotechnol. (2012) 1:32–8. doi: 10.1016/j.bcab.2011.08.005
19. Adetuyi F, Ibrahim T. Effect of fermentation time on the phenolic, flavonoid and vitamin C contents and antioxidant activities of okra (Abelmoschus esculentus) seeds. Nigerian Food J. (2014) 32:128–37. doi: 10.1016/S0189-7241(15)30128-4
20. Zhang H, Yi J, Piao X, Li P, Zeng Z, Wang D, et al. The metabolizable energy value, standardized ileal digestibility of amino acids in soybean meal, soy protein concentrate and fermented soybean meal, and the application of these products in early-weaned piglets. Asian Aust J Anim Sci. (2013) 26:691. doi: 10.5713/ajas.2012.12429
21. Hur SJ, Lee SY, Kim YC, Choi I, Kim B. Effect of fermentation on the antioxidant activity in plant-based foods. Food Chem. (2014) 160:346–56. doi: 10.1016/j.foodchem.2014.03.112
22. Liu SN, Han Y, Zhou ZJ. Lactic acid bacteria in traditional fermented Chinese foods. Food Res Int. (2011) 44:643–51. doi: 10.1016/j.foodres.2010.12.034
23. Feng J, Liu X, Xu Z, Wang Y, Liu J. Effects of fermented soybean meal on digestive enzyme activities and intestinal morphology in broilers. Poult Sci. (2007) 86:1149–54. doi: 10.1093/ps/86.6.1149
24. Latimer J. Official Methods of Analysis of AOAC International. Washington, DC: Association of Official Analytical Chemists Inc. (2012).
25. Aviagen W. Ross 308: Broiler's Management and Nutrition Specification (2018). Available onlione at: https://en.aviagen.com/assets/Tech_Center/Ross_Broiler/Ross-BroilerHandbook2018-EN.pdf
26. AOAC. Official Methods of Analysis of AOAC International. Washington, DC: Association of Official Analytical Chemists (2012).
27. Short F, Gorton P, Wiseman J, Boorman K. Determination of titanium dioxide added as an inert marker in chicken digestibility studies. Anim Feed Sci Technol. (1996) 59:215–21. doi: 10.1016/0377-8401(95)00916-7
29. American Veterinary Medical Association. AVMA Guidelines for the Euthanasia of Animals: 2013 Edition. Schaumburg, IL: American Veterinary Medical Association (2013).
30. Lie Ø, Syed M, Solbu H. Improved agar plate assays of bovine lysozyme and haemolytic complement activity. Acta Vet. Scand. (1986) 27:23–32. doi: 10.1186/BF03548556
31. Gilani S, Howarth G, Nattrass G, Kitessa S, Barekatain R, Forder R, et al. Gene expression and morphological changes in the intestinal mucosa associated with increased permeability induced by short-term fasting in chickens. J Anim Physiol Anim Nutr. (2018) 102:e653–61. doi: 10.1111/jpn.12808
32. Kheravii S, Swick RA, Choct M, Wu SB. Upregulation of genes encoding digestive enzymes and nutrient transporters in the digestive system of broiler chickens by dietary supplementation of fiber and inclusion of coarse particle size corn. BMC Genom. (2018) 19:208. doi: 10.1186/s12864-018-4592-2
33. Livak KJ, Schmittgen TD. Analysis of relative gene expression data using real-time quantitative PCR and the 2– ΔΔCT method. Methods. (2001) 25:402–8. doi: 10.1006/meth.2001.1262
34. Jazi V, Boldaji F, Dastar B, Hashemi S, Ashayerizadeh A. Effects of fermented cottonseed meal on the growth performance, gastrointestinal microflora population and small intestinal morphology in broiler chickens. Br Poult Sci. (2017) 58:402–8. doi: 10.1080/00071668.2017.1315051
35. Shi C, Zhang Y, Lu Z, Wang Y. Solid-state fermentation of corn-soybean meal mixed feed with Bacillus subtilis and Enterococcus faecium for degrading antinutritional factors and enhancing nutritional value. J Anim Sci Biotechnol. (2017) 8:1–9. doi: 10.1186/s40104-017-0184-2
36. Jazi V, Ashayerizadeh A, Toghyani M, Shabani A, Tellez G. Fermented soybean meal exhibits probiotic properties when included in Japanese quail diet in replacement of soybean meal. Poultry Sci. (2018) 97:2113–22. doi: 10.3382/ps/pey071
37. Ashayerizadeh A, Dastar B, Shargh MS, Mahoonak AS, Zerehdaran S. Fermented rapeseed meal is effective in controlling Salmonella enterica serovar Typhimurium infection and improving growth performance in broiler chicks. Vet Microbiol. (2017) 201:93–102. doi: 10.1016/j.vetmic.2017.01.007
38. Khempaka S, Thongkratok R, Okrathok S, Molee W. An evaluation of cassava pulp feedstuff fermented with A. oryzae, on growth performance, nutrient digestibility and carcass quality of broilers. J Poultry Sci. (2013) 51:71–9. doi: 10.2141/jpsa.0130022
39. Hu Y, Wang Y, Li A, Wang Z, Zhang X, Yun T, et al. Effects of fermented rapeseed meal on antioxidant functions, serum biochemical parameters and intestinal morphology in broilers. Food Agric Immunol. (2016) 27:182–93. doi: 10.1080/09540105.2015.1079592
40. Fernandez-Orozco R, Frias J, Muñoz R, Zielinski H, Piskula MK, Kozlowska H, et al. Fermentation as a bio-process to obtain functional soybean flours. J Agric Food Chem. (2007) 55:8972–9. doi: 10.1021/jf071823b
41. Chen L, Madl RL, Vadlani PV. Nutritional enhancement of soy meal via Aspergillus oryzae solid-state fermentation. Cereal Chem. (2013) 90:529–34. doi: 10.1094/CCHEM-01-13-0007-R
42. Sugiharto S, Ranjitkar S. Recent advances in fermented feeds towards improved broiler chicken performance, gastrointestinal tract microecology and immune responses: a review. Anim Nutr. (2019) 5:1–10. doi: 10.1016/j.aninu.2018.11.001
43. Rozan P, Villaum C, Bau H, Schwertz A, Nicolas J, Mejean L. Detoxication of rapeseed meal by Rhizopus oligosporus sp-T3: a first step towards rapeseed protein concentrate. Int J Food Sci Technol. (1996) 31:85–90. doi: 10.1111/j.1365-2621.1996.17-315.x
44. Drazbo A, Mikulski D, Jankowski J, Zduńczyk Z. The effect of diets containing raw and fermented faba beans on gut functioning and growth performance in young turkeys. J Anim Feed Sci. (2018) 27:65–73. doi: 10.22358/jafs/82779/2018
45. Soumeh E, Mohebodini H, Toghyani M, Shabani A, Ashayerizadeh A, Jazi V. Synergistic effects of fermented soybean meal and mannan-oligosaccharide on growth performance, digestive functions, and hepatic gene expression in broiler chickens. Poult Sci. (2019) 98:6797–807. doi: 10.3382/ps/pez409
46. Taheri H, Moravej H, Tabandeh F, Zaghari M, Shivazad M. Screening of lactic acid bacteria toward their selection as a source of chicken probiotic. Poultry Sci. (2009) 88:1586–93. doi: 10.3382/ps.2009-00041
47. Sun H, Tang JW, Yao XH, Wu YF, Wang X, Feng J. Improvement of the nutritional quality of cottonseed meal by Bacillus subtilis and the addition of papain. Int J Agric Biol. (2012) 14:563–8.
48. Li W, Bai J, Li Y, Qin Y, Yu D. Effects of Bacillus subtilis on meat quality, nutrient digestibility and serum biochemical parameters of broilers. Chin J Vet Sci. (2014) 34:1682–5.
49. Ashayerizadeh A, Dastar B, Shams SM, Sadeghi R. Effects of feeding fermented rapeseed meal on growth performance, gastrointestinal microflora population, blood metabolites, meat quality, and lipid metabolism in broiler chickens. Livest Sci. (2018) 216:183–90.
50. El-Moghazy GM, Sakr DM, Abd El Ghafar N. Effect of fermentation of faba bean (Vicia faba) on its nutritive and sensory properties. J Food Dairy Sci. (2011) 2:237–50. doi: 10.21608/jfds.2011.81949
51. Hirabayashi M, Matsui T, Yano H. Fermentation of soybean meal with Aspergillus usamii improves zinc availability in rats. Biol Trace Elem Res. (1998) 61:227–34. doi: 10.1007/BF02784033
52. Yamauchi F, Suetsuna K. Immunological effects of dietary peptide derived from soybean protein. J Nutr Biochem. (1993) 4:450–7. doi: 10.1016/0955-2863(93)90062-2
53. Yuan L, Chang J, Yin Q, Lu M, Di Y, Wang P, et al. Fermented soybean meal improves the growth performance, nutrient digestibility, and microbial flora in piglets. Anim Nutr. (2017) 3:19–24. doi: 10.1016/j.aninu.2016.11.003
54. Mathivanan R, Selvaraj P, Nanjappan K. Feeding of fermented soybean meal on broiler performance. Int J Poultry Sci. (2006) 5:868–72. doi: 10.3923/ijps.2006.868.872
55. Pacheco W, Stark C, Ferket P, Brake J. Effects of trypsin inhibitor and particle size of expeller-extracted soybean meal on broiler live performance and weight of gizzard and pancreas. Poult Sci. (2014) 93:2245–52. doi: 10.3382/ps.2014-03986
56. Hong KJ, Lee CH, Kim SW. Aspergillus oryzae GB-107 fermentation improves nutritional quality of food soybeans and feed soybean meals. J Med Food. (2004) 7:430–5. doi: 10.1089/jmf.2004.7.430
57. Canibe N, Jensen BB. Fermented liquid feed—Microbial and nutritional aspects and impact on enteric diseases in pigs. Anim Feed Sci Technol. (2012) 173:17–40. doi: 10.1016/j.anifeedsci.2011.12.021
58. Gallardo C, Dadalt J, Kiarie E, Neto MT. Effects of multi-carbohydrase and phytase on standardized ileal digestibility of amino acids and apparent metabolizable energy in canola meal fed to broiler chicks. Poult Sci. (2017) 96:3305–13. doi: 10.3382/ps/pex141
59. Jeong JS, Park JW, Lee SI, Kim IH. Apparent ileal digestibility of nutrients and amino acids in soybean meal, fish meal, spray-dried plasma protein and fermented soybean meal to weaned pigs. Anim Sci J. (2016) 87:697–702. doi: 10.1111/asj.12483
60. Gao Z, Wu H, Shi L, Zhang X, Sheng R, Yin F, et al. Study of Bacillus subtilis on growth performance, nutrition metabolism and intestinal microflora of 1 to 42 d broiler chickens. Anim Nutr. (2017) 3:109–13. doi: 10.1016/j.aninu.2017.02.002
61. Li CY, Lu JJ, Wu CP, Lien TF. Effects of probiotics and bremelain fermented soybean meal replacing fish meal on growth performance, nutrient retention and carcass traits of broilers. Livestock Sci. (2014) 163:94–101. doi: 10.1016/j.livsci.2014.02.005
62. Feng J, Liu X, Xu Z, Lu Y, Liu Y. Effect of fermented soybean meal on intestinal morphology and digestive enzyme activities in weaned piglets. Digest Dis Sci. (2007) 52:1845–50. doi: 10.1007/s10620-006-9705-0
63. Feng H, Qu H, Liu Y, Shi Y, Wu S, Bao W. Effect of fermented soybean meal supplementation on some growth performance, blood chemical parameters, and fecal microflora of finishing pigs. Rev Bras Zootecnia. (2020) 49. doi: 10.37496/rbz4920190096
64. Chachaj R, Sembratowicz I, Krauze M, Stepniowska A, Rusinek-Prystupa E, Czech A, et al. The effect of fermented soybean meal on performance, biochemical and immunological blood parameters in turkeys. Ann Anim Sci. (2019) 19:1035–49. doi: 10.2478/aoas-2019-0040
65. Usayran N, Sha'ar H, Barbour G, Yau S, Maalouf F, Farran M. Nutritional value, performance, carcass quality, visceral organ size, and blood clinical chemistry of broiler chicks fed 30% tannin-free fava bean diets. Poultry Sci. (2014) 93:2018–27. doi: 10.3382/ps.2014-03872
66. Laudadio V, Ceci E, Tufarelli V. Productive traits and meat fatty acid profile of broiler chickens fed diets containing micronized fava beans (Vicia faba L. var. minor) as the main protein source. J Appl Poultry Res. (2011) 20:12–20. doi: 10.3382/japr.2010-00173
67. Tufarelli V, Laudadio V. Feeding of dehulled-micronized faba bean (Vicia faba var. minor) as substitute for soybean meal in guinea fowl broilers: effect on productive performance and meat quality. Asian Aust J Anim Sci. (2015) 28:1471. doi: 10.5713/ajas.15.0245
68. Farrell D, Perez-Maldonado R, Mannion P. Optimum inclusion of field peas, faba beans, chick peas and sweet lupins in poultry diets. II. Broiler experiments. Br Poultry Sci. (1999) 40:674–80. doi: 10.1080/00071669987070
69. Sun H, Tang JW, Yao XH, Wu YF, Wang X, Feng J. Effects of dietary inclusion of fermented cottonseed meal on growth, cecal microbial population, small intestinal morphology, and digestive enzyme activity of broilers. Trop Anim Health Prod. (2013) 45:987–93. doi: 10.1007/s11250-012-0322-y
70. Ferraris RP. Dietary and developmental regulation of intestinal sugar transport. Biochem J. (2001) 360:265–76. doi: 10.1042/bj3600265
71. Al-Khalaifah HS, Shahin SE, Omar AE, Mohammed HA, Mahmoud HI, Ibrahim D. Effects of graded levels of microbial fermented or enzymatically treated dried brewer's grains on growth, digestive and nutrient transporter genes expression and cost effectiveness in broiler chickens. BMC Vet Res. (2020) 16:1–15. doi: 10.1186/s12917-020-02603-0
72. Lee KW, Kim DK, Lillehoj HS, Jang SI, Lee SH. Immune modulation by Bacillus subtilis-based direct-fed microbials in commercial broiler chickens. Anim Feed Sci Technol. (2015) 200:76–85. doi: 10.1016/j.anifeedsci.2014.12.006
74. Pham VH, Kan L, Huang J, Geng Y, Zhen W, Guo Y, et al. Dietary encapsulated essential oils and organic acids mixture improves gut health in broiler chickens challenged with necrotic enteritis. J Anim Sci Biotechnol. (2020) 11:1–18. doi: 10.1186/s40104-019-0421-y
75. Johansson ME, Sjövall H, Hansson GC. The gastrointestinal mucus system in health and disease. Nat Rev Gastroenterol Hepatol. (2013) 10:352. doi: 10.1038/nrgastro.2013.35
76. Robinson K, Deng Z, Hou Y, Zhang G. Regulation of the intestinal barrier function by host defense peptides. Front Vet Sci. (2015) 2:57. doi: 10.3389/fvets.2015.00057
77. Parlato M, Yeretssian G. NOD-like receptors in intestinal homeostasis and epithelial tissue repair. Int J Mol Sci. (2014) 15:9594–627. doi: 10.3390/ijms15069594
78. Lin WC, Lee TT. The Laetiporus sulphureus fermented product enhances the antioxidant status, intestinal tight junction, and morphology of broiler chickens. Animals. (2021) 11:149. doi: 10.3390/ani11010149
79. Zhang J, Zhu J, Sun J, Jiang R, Rahman MRT. The sterilized fermented feed improves intestinal barrier function and immune function in chicken. FASEB J. (2016) 30:lb247. doi: 10.1096/fasebj.30.1_supplement.lb247
80. Zhang Y, Chen S, Zong X, Wang C, Shi C, Wang F, et al. Peptides derived from fermented soybean meal suppresses intestinal inflammation and enhances epithelial barrier function in piglets. Food Agric Immunol. (2020) 31:120–35. doi: 10.1080/09540105.2019.1705766
81. Rajput I, Li L, Xin X, Wu B, Juan Z, Cui Z, et al. Effect of Saccharomyces boulardii and Bacillus subtilis B10 on intestinal ultrastructure modulation and mucosal immunity development mechanism in broiler chickens. Poult Sci. (2013) 92:956–65. doi: 10.3382/ps.2012-02845
82. Wu Y, Wang B, Zeng Z, Liu R, Tang L, Gong L, et al. Effects of probiotics Lactobacillus plantarum 16 and Paenibacillus polymyxa 10 on intestinal barrier function, antioxidative capacity, apoptosis, immune response, and biochemical parameters in broilers. Poult Sci. (2019) 98:5028–39. doi: 10.3382/ps/pez226
83. Moschini M, Masoero F, Prandini A, Fusconi G, Morlacchini M, Piva G. Raw pea (Pisum sativum), raw Faba bean (Vicia faba var. minor) and raw Lupin (Lupinus albus var. multitalia) as alternative protein sources in broiler diets. Italian J Anim Sci. (2005) 4:59–69. doi: 10.4081/ijas.2005.59
84. Huang Y, Zheng Y. The probiotic Lactobacillus acidophilus reduces cholesterol absorption through the down-regulation of Niemann-Pick C1-like 1 in Caco-2 cells. Br J Nutr. (2010) 103:473–8. doi: 10.1017/S0007114509991991
85. Feng J, Liu X, Xu Z, Liu Y, Lu Y. Effects of Aspergillus oryzae 3.042 fermented soybean meal on growth performance and plasma biochemical parameters in broilers. Anim Feed Sci Technol. (2007) 134:235–42. doi: 10.1016/j.anifeedsci.2006.08.018
86. Heres L, Wagenaar JA, van Knapen F, Urlings BA. Passage of Salmonella through the crop and gizzard of broiler chickens fed with fermented liquid feed. Avian Pathol. (2003) 32:173–81. doi: 10.1080/0307945021000071597
87. Kim MH, Yun CH, Kim HS, Kim JH, Kang SJ, Lee CH, et al. Effects of fermented soybean meal on growth performance, diarrheal incidence and immune-response of neonatal calves. Anim Sci J. (2010) 81:475–81. doi: 10.1111/j.1740-0929.2010.00760.x
88. Fazhi X, Lvmu L, Jiaping X, Kun Q, Zhide Z, Zhangyi L. Effects of fermented rapeseed meal on growth performance and serum parameters in ducks. Asian Aust J Anim Sci. (2011) 24:678–84. doi: 10.5713/ajas.2011.10458
Keywords: broiler chickens, fermented fava beans by-products, growth, cecal microflora, intestinal barrier
Citation: Omar AE, Al-Khalaifah HS, Ismail TA, Abd El-Aziz RM, El-Mandrawy SAM, Shalaby SI and Ibrahim D (2021) Performance, Serum Biochemical and Immunological Parameters, and Digestive Enzyme and Intestinal Barrier-Related Gene Expression of Broiler Chickens Fed Fermented Fava Bean By-Products as a Substitute for Conventional Feed. Front. Vet. Sci. 8:696841. doi: 10.3389/fvets.2021.696841
Received: 18 April 2021; Accepted: 09 June 2021;
Published: 15 July 2021.
Edited by:
Amlan Kumar Patra, West Bengal University of Animal and Fishery Sciences, IndiaReviewed by:
Guanhong Li, Jiangxi Agricultural University, ChinaAiwei Guo, Southwest Forestry University, China
Copyright © 2021 Omar, Al-Khalaifah, Ismail, Abd El-Aziz, El-Mandrawy, Shalaby and Ibrahim. This is an open-access article distributed under the terms of the Creative Commons Attribution License (CC BY). The use, distribution or reproduction in other forums is permitted, provided the original author(s) and the copyright owner(s) are credited and that the original publication in this journal is cited, in accordance with accepted academic practice. No use, distribution or reproduction is permitted which does not comply with these terms.
*Correspondence: Doaa Ibrahim, doibrahim@vet.zu.edu.eg; orcid.org/0000-0003-3402-1216