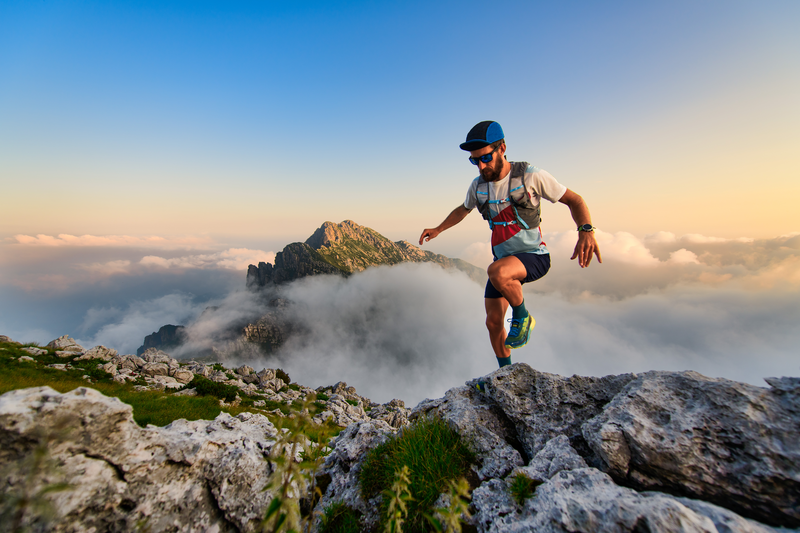
94% of researchers rate our articles as excellent or good
Learn more about the work of our research integrity team to safeguard the quality of each article we publish.
Find out more
ORIGINAL RESEARCH article
Front. Vet. Sci. , 19 August 2021
Sec. Animal Nutrition and Metabolism
Volume 8 - 2021 | https://doi.org/10.3389/fvets.2021.692389
This article is part of the Research Topic Parent-offspring Integration: Gut Health and Physiological Functions of Animals View all 15 articles
Lactobacillus delbrueckii is a Gram-positive bacterium mostly used in the dairy industry for yogurt and cheese. The present study was designed to evaluate the effects of Lactobacillus delbrueckii on serum biochemical parameters, intestinal morphology, and performance by supplementing at a dietary level of 0.1% in diets for weaned piglets. Eighty healthy weaned piglets (initial body weight: 7.56 ± 0.2 kg) were randomly divided into two feeding groups with four replicates in each group (n = 10 animals per replicate); piglets were fed with basal diet (CON) or basal diet containing 0.1% Lactobacillus delbrueckii (LAC). The results showed that dietary supplementation of Lactobacillus delbrueckii improved growth performance and increased serum HDL and insulin levels in piglets on the 28th day of the experimental time (p < 0.05). The gut microbe analysis revealed that Lactobacillus delbrueckii significantly decreased the relative abundance of the phyla Bacteroidetes, but increased the relative abundance of the phyla Firmicutes. The Lactobacillus delbrueckii also significantly increased the relative abundance of Bifidobacterium and Lactobacillus at the genus level of the bacterial community in the ileum, but decreased the relative abundance of unclassified Clostridiales. Moreover, Lactobacillus delbrueckii improved mucosal morphology by obtaining higher intestinal villus height (p < 0.05), significantly increasing the concentrations of butyrate, isobutyric acid, and isovaleric acid in colonic chyme of piglets, but decreasing the intestinal pH at the duodenum and ileum on the 28th day of the experimental time. In conclusion, dietary supplementation of Lactobacillus delbrueckii in the diet of weaned piglets can improve intestinal morphology and modulate the microbiota community to promote growth performance.
Weaning is one of the most stressful stages in pig production. It could cause intestinal and immune system dysfunctions, resulting in compromised growth performance and inferior disease resistance of piglets (1, 2). Also, it could cause drastic changes in intestinal morphology, such as shortening of the villus, elongation of the crypt (3, 4), and even chronic impairment of the mucosal barrier function (5).
Probiotics are living non-pathogenic bacteria that have beneficial effects on the host animals by modulating their intestinal microbial structure, and they are used as feed additives in animal production (6). The effects of some probiotic strains on improvements of intestinal microbial balance were observed in piglets and poultry (7, 8). The gastrointestinal system has multiple functions such as maintaining humoral balance, secreting digestive enzymes, immunoglobulins, and other components, and it is also a barrier for the host to resist harmful pathogens and antigens. Many studies have found that Lactobacillus could improve intestinal morphology and gut microbiota to prevent intestinal barrier dysfunction (9, 10). Thus, Lactobacillus has been used to promote growth and intestinal integrity in animals. However, the mechanisms of how probiotic Lactobacillus worked were not fully elucidated.
In our previous study, oral administration of Lactobacillus delbrueckii to suckling piglets improved the immune response, intestinal morphology, barrier function, and growth (11). Lactobacillus delbrueckii is a Gram-positive bacterium mostly used in the dairy industry for yogurt and cheese, exerting beneficial probiotic roles (12). In addition, our previous results showed that dietary supplementation of 0.1% Lactobacillus delbrueckii improved the growth performance of fattening pigs (13). The current study was designed to evaluate the effects of Lactobacillus delbrueckii on serum biochemical parameters, intestinal morphology, and gut microbial construction by supplementing with Lactobacillus delbrueckii at a dietary level of 0.1% of the diets for the weaned piglets.
Eighty healthy weaned piglets (Landrace × Yorkshire × Duroc) of mixed sex were randomly divided into two groups with four replicates (pens), each pen with 10 pigs (male and female half). The experiment started when the piglets reached 28 days of age after weaning. The control (CON) group was fed a basal diet (without antibiotic); the Lactobacillus delbrueckii (LAC) group was fed a basal diet containing 0.1% L. delhrueckii (1.0 × 1010CFU/g). Feed and water were provided ad libitum throughout the experiment period. The experimental lasted 28 days and was divided in two phases (phase I from d 1 to 14, and phase II from d 15 to 28). The basal diets were formulated based on the nutrient requirements of swine (NRC, 2012) (Table 1).
The Lactobacillus delhrueckii CCTCC M207096 was obtained from the microbiology laboratory of the College of Animal Science and Technology, Hunan Agricultural University, and prepared as microcapsule granules (1.0 × 1010 CFU/g) by the Hunan Pufeike Biotechnology Company. The Lactobacillus delhrueckii was enveloped by the binder, microcrystalline cellulose, and other excipients, followed by water granulation, shot blasting and drying, and finally prepared into particles. The Lactobacillus delhrueckii was heated during the processing. The number of Lactobacillus delhrueckii (1.0 × 1010 CFU/g) was measured by viable bacteria.
Feed intake per pen was recorded daily. The growth rate and feed conversion ratio (feed/gain) for two different experimental periods were calculated. At the 14th and 28th day of the experiment, four piglets (one per replicate) with medium body weights in each pen were selected. The blood samples for each selected piglet were collected by venipuncture into 15-ml tubes and centrifuged at 3,000 × g for 10 min at 4°C. The supernatants (serum) were collected for serum biochemical analyses. After blood collection, the piglets were slaughtered by exsanguination after electrical stunning.
About 2 g of digesta were taken from the middle of the ileum, cecum, and colon, respectively, after the weaned piglets were euthanized for analysis of microbial diversity. Feces (~1 g) were collected for short-chain fatty acid (SCFA) determination. Digesta and feces samples were separately stored at −80°C. The small intestinal sections, including the duodenum from the pyloric sphincter to the duodenojejunal bend, the jejunum, which ended at the attachment of the plica ileocecalis, and the ileal, which ended at the ileocecal opening (14), were quickly removed and divided into three parts. A segment (2 cm) of each intestinal section was collected for mucosal morphology analysis.
The concentrations of total protein (TP), triglycerides (TG), total cholesterol (TC), high-density lipoprotein (HDL), low-density lipoprotein (LDL), and the activities of alanine aminotransferase (ALT) and aspartate aminotransferase (AST) in the serum of the piglets were analyzed using the BS-200 automatic blood biochemical analyzer (Mindray, Shengzhen, China).
The 2-cm intestinal tissue samples were stained with hematoxylin and eosin (HE) as described previously (11). Villus height (from the villi tip to the villus–crypt joint) and crypt depth (from the villus–crypt joint to the base of the crypt) were measured under an Olympus Van-Ox S microscope (Opelco, Washington, DC, USA) using an image analysis software (Image-Pro, Media Cybernetics, Inc., Silver Springs, MD, USA). Ten sections were taken from each slice for the measurements. The villus height/crypt depth (V/C) value was calculated.
Immediately after slaughtering, the pH values of the basal glandular areas of the small intestine, cecum, colon, and rectum were measured in situ. The pH values of the small intestine were measured at fixed points, which were at 1 cm distal from the pylorus, and at 1/16, 1/8, 1/4, 1/2, 3/4, and 4/4 of the length of the small intestinal sections. All pH measurements were tested by inserting the pH probe into the cavities through a small incision on the gut wall (portable Sentron pH meter type Argus with Lancefet probe, The Netherlands, Sentron Europe B.V., Roden,).
Total microbial genomic DNA samples were extracted using the QIAamp DNA Stool Mini Kit (QIAGEN, Inc., Netherlands) and stored at −20°C prior to further analysis. The concentration and quality of the extracted DNAs were determined by the NanoDrop ND-1000 spectrophotometer (Thermo Fisher Scientific, Waltham, MA, USA) and agarose gel electrophoresis, respectively. The V3–V4 hypervariable region of the bacterial 16S rRNA gene was amplified by PCR with the forward primer 338F: 5′-ACTCCTACGGGAGGCAGCAG-3′ and the reverse primer 806R: 5′-GGAC- TACHVGGGTWTCTAAT-3′. Sample-specific 7-bp barcodes were incorporated into the primers for multiplex sequencing. The PCR components contained 5 μl of Q5 reaction buffer (5 ×), 5 μl of Q5 high-fidelity GC buffer (5 ×), 0.25 μl of Q5 high-fidelity DNA polymerase (5 U/μl), 2 μl (2.5 mM) of dNTPs, 1 μl (10 uM) of each forward and reverse primer, 2 μl of DNA template, and 8.75 μl of ddH2O. Thermal cycling consisted of initial denaturation at 98°C for 2 min, followed by 25 cycles consisting of denaturation at 98°C for 15 s, annealing at 55°C for 30 s, and extension at 72°C for 30 s, with a final extension of 5 min at 72°C. PCR amplicons were purified with Agencourt AMPure Beads (Beckman Coulter, Indianapolis, IN, USA) and quantified using the PicoGreen dsDNA Assay Kit (Invitrogen, Carlsbad, CA, USA). After the individual quantification step, amplicons were pooled in equal amounts, and paired-end 2′ 300-bp sequencing was performed using the Illlumina MiSeq platform with MiSeq Reagent Kit v3 at the Shanghai Personal Biotechnology Co., Ltd. (Shanghai, China) (15, 16). The OTUs, alpha diversity, beta diversity analysis, and the microbiota structure analysis were done according to previously described procedures (17). OTU taxonomy was assigned by the Greengene database. For α-diversity analysis, Chao 1 index was calculated by Mothur, and the Shannon index was calculated by the R package “vegan” to estimate the bacterial community richness within each sample. β-diversity was assessed by MANOVA and principal coordinate analysis (PCoA).
Gas chromatography (Agilent 7890 A, Agilent Technologies, Santa Clara, CA, USA) was used to determine the concentrations of acetate, propionate, butyrate, i-butyrate, i-valerate, and valeric acid in colon contents according to the procedures described previously (18). One microliter of sample was injected into a 7890 Agilent gas chromatograph. Nitroterephthalic acid-modified polyethylene glycol column (DB-FFAP) was used for the gas chromatograph. The column temperature was operated at 250 to 280°C, and the carrier gas was 0.8 ml/min of high-purity N2. The minimum detectable thresholds for all volatile fatty acids (VFA) were 0.1 mmol/L.
Data in tables and figures were expressed as means ± SEM and means ± SD, respectively, and data were analyzed by T-test using SPSS 20.0 (SPSS, Inc., Chicago, IL, USA). Significance was defined as a p-value < 0.05%. The statistical analysis used in the assessment of the microbial community structure of α-diversity and OTU counts were determined by T-test using SPSS 20.0 as well.
The results of Table 2 shows the growth performance in the weaned piglets. For the phase I (1 to 14 d), phase II (15 to 28 d), and the entire experimental period, piglets in the LAC group had higher ADG and BW when compared with the CON group (p < 0.05). There were no differences (p > 0.05) in ADFI and FCR between the LAC group and the CON group at phase I and the phase II, but the LAC group had higher ADFI and lower FCR values than the CON group throughout the entire experimental period (p < 0.05).
The results of serum biochemical parameters are shown in Table 3. Although no significant differences on the serum concentrations of AST, LDL, TC, and TG were observed among groups throughout the entire experimental period (p > 0.05), the ALT concentration of piglets in the LAC group was lower than that in the CON group on the 28th day (p < 0.05). The TP concentration of piglets in the LAC group was higher than that in the CON group on the 14th day (p < 0.05). The HDL concentration of piglets in the LAC group was higher than that in the CON group on the 28th day (p < 0.05).
Table 3. Effects of Lactobacillus delbrueckii on serum biochemistry parameters in the weaned piglets.
The results of Figure 1 shows the morphologies of the intestinal mucosa in the duodenum, jejunum, and ileum. The villus height and V/C in the duodenum of the LAC group were not significantly affected throughout the entire experimental period (p > 0.05). The villus height, crypt depth, and V/C in the jejunum of the LAC group were not significantly affected on the 14th day (p > 0.05), but the crypt depth was lower, and the V/C was higher in the LAC group on the 28th day (p < 0.05). The villus height, crypt depth, and V/C in the ileum of the LAC group were not significantly affected on the 14th day, but the villus height and the V/C were significantly affected on the 28th day (p < 0.05).
Figure 1. Effects of Lactobacillus delbrueckii on the intestinal mucosal morphology of the weaned piglets. (A) Intestinal (a: duodenum; b: jejunum; c: ileum) mucosal morphology was observed (40 ×) in weaned piglets on the 14th day. (B) The villus lengths, the crypt depths, and the V/C of the intestinal sections on the 14th day. (C) Intestinal (a: duodenum; b: jejunum; c: ileum) mucosal morphology was observed (40 ×) in weaned piglets on the 28th day. (D) The villus lengths, the crypt depth, and the V/C of the intestinal sections on the 28th day. N = 4. Label (*) mean significant difference between the CON group and the LAC group (p < 0.05). CON, basal diet; LAC, basal diet with 0.1% Lactobacillus delbrueckii.
Figure 2 shows the pH values of the duodenum, jejunum, ileum, cecum, and colon. There were no significant differences in the intestinal pH between the two groups on the 14th day (p > 0.05), but the LAC group had lower pH values in the duodenum and ileum compared with the CON group on the 28th day (p < 0.05).
Figure 2. Effects of Lactobacillus delbrueckii on intestinal pH in weaned piglets. N = 4. Label (*) mean significant difference between the CON group and the LAC group (p < 0.05). CON, basal diet; LAC, basal diet with 0.1% Lactobacillus delbrueckii.
To assess whether the gut microbiota was influenced by Lactobacillus delhrueckii, we performed α-diversity analyses on the digesta of the ileum, cecum, and colon. The results show that the dietary supplementation of Lactobacillus delhrueckii did have effects on Chao 1 and Shannon of the alpha diversity indexes of the microbial communities in the ileum, cecum, and colon (Table 4). At 14 days, piglets in the LAC group exhibited higher Chao 1 in the ileum, cecum, and colon (p < 0.05) when compared with piglets in the CON group. At 28 days, piglets in the LAC group exhibited higher Shannon indexes in the ileum (p < 0.05). Additionally, piglets in the LAC group exhibited higher Chao 1 in the cecum (p < 0.05) and higher Shannon indexes in the colon compared with the CON group (p < 0.05). However, β-diversity was not significantly different between the groups in the ileum, cecum, and colon (p>0.05), as shown in Figures 3A–C.
Table 4. Effects of Lactobacillus delbrueckii on the α-diversity of the microbiota in the weaned piglets.
Figure 3. Effects of Lactobacillus delbrueckii on the β-diversity of the weaned piglets. (A) Microbial community β-diversity (unweighted Unifrac, p > 0.05) in the ileum of the piglets at 14 and 28 days, which was demonstrated using principal coordinates analysis (PCoA) of the unweighted Unifrac distance matrices. (B) Microbial community β-diversity (unweighted Unifrac, p > 0.05) in the cecum of the piglets at 14 and 28 days, which was demonstrated using principal coordinates analysis (PCoA) of the unweighted Unifrac distance matrices. (C) Microbial community β-diversity (unweighted Unifrac, p > 0.05) in the colon of the piglets at 14 and 28 days, which was demonstrated using principal coordinates analysis (PCoA) of the unweighted Unifrac distance matrices. Each dot represented one sample, and each group was denoted by a different color and shape. CON, basal diet; LAC, basal diet with 0.1% Lactobacillus delbrueckii.
The microbial compositions in the ileum, cecum, and colon of the weaned piglets are shown at the phylum level in Figure 4. The two main bacteria phyla were Firmicutes and Bacteroidetes in the ileum, cecum, and colon, with Actinobacteria, Proteobacteria, Cyanobacteria, and Tenericutes also presented in the three sections. The relative abundances of the different phylum in the gut are shown in Table 5. There were no significant differences in the relative abundances of bacterial phylum in the ileum between the LAC group and the CON group throughout the entire experimental period (p > 0.05). On the 28th day, piglets in the LAC group exhibited higher Firmicutes content in the cecum compared with the piglets in the CON group (p < 0.05). On the 14th day, the piglets in the LAC group exhibited higher relative abundance of Firmicutes but lower relative abundance of Bacteroidetes in the cecum compared with the CON group (p < 0.05). However, on the 28th day, the piglets in the LAC group exhibited higher relative abundances of Firmicutes and Fibrobacteres, but lower relative abundances of Bacteroidetes and Actinobacteria in the cecum compared with the piglets in the CON group (p < 0.05).
Figure 4. Effects of Lactobacillus delbrueckii on the bacterial community at the phylum level in the guts of the weaned piglets. AHM, AMM, and AJM represent ileum, cecum, and colon samples of the CON group, respectively; BHM, BMM, and BJM represent ileum, cecum, and colon samples of the LAC group, respectively; 14 and 28 represent the 14 and 28 days of the trial period, respectively.
Changes in the bacterial communities at the genus level were noticed in the ileum, cecum, and colon of weaned piglets between the two groups, which are shown in Figure 5. On the 14th day, piglets in the LAC group exhibited higher relative abundance of Bifidobacterium and Lactobacillus, but lower relative abundance of unclassified_Clostridiales in the ileum (p < 0.05). Moreover, piglets in the LAC group exhibited higher relative abundance of 02d06 and unclassified Clostridiales in the colon (p < 0.05). At the 28th day, piglets in the LAC group exhibited higher relative abundance of Lactobacillus, but lower relative abundance of unclassified_Clostridiales and p-75-a5 in the ileum (p < 0.05). Additionally, piglets in the LAC group exhibited higher relative abundance of unidentified_RF16, but lower relative abundance of 02d06 in the cecum on the 28th day (p < 0.05). Finally, the piglets in the LAC group exhibited higher relative abundance of unidentified Christensenellaceae, unidentified Lachnospiraceae, Ruminococcus, unidentified Mogibacteriaceae, Oscillospira, unidentified Ruminococcaceae, but lower relative abundance of Blautia in the colon on the 28th day (p < 0.05).
Figure 5. Effects of Lactobacillus delbrueckii on the intestinal bacterial community at the genus level in the weaned piglets. CON, basal diet; LAC, basal diet group with 0.1% Lactobacillus delbrueckii; 14 and 28 represent the 14 and 28 days of the trial period, respectively. N = 4. Label (*) mean significant difference between the CON group and the LAC group (p < 0.05).
The SCFA concentrations in the colonic chyme are shown in Figure 6. No significant differences were observed in the total SCFA concentration in the colonic chyme between the LAC group and the CON group on the 14th day (p > 0.05). On the 28th day, the concentrations of butyrate, isobutyric acid, and isovaleric acid in the colonic chyme increased significantly in the LAC group (p < 0.05). However, there were no differences in the concentrations of acetate, propionate, valeric acid, and total SCFA in the LAC group compared with the CON group.
Figure 6. Effects of Lactobacillus delbrueckii on the concentrations of the short-chain fatty acids in the colon digesta of the weaned piglets. CON, basal diet; LAC, basal diet group with 0.1% Lactobacillus delbrueckii; SCFA, short-chain fatty acid, including acetic acid, propionic acid, and butyric acid BCFA, branched-chain fatty acid, including isobutyric acid, isovaleric acid, and valeric acid. Total SCFA, total short-chain fatty acid, including acetic acid, propionic acid, butyric acid, isobutyric acid, isovaleric acid, and valeric acid. N = 4. Label (*) mean significant difference between the CON group and the LAC group (p < 0.05); 14 and 28 represent the 14 and 28 days of the trial period, respectively.
Probiotics have beneficial effects on the host by enhancing the growth performance and providing the immunological protection (19). Lactobacillus species are one taxon of the resident bacteria in the gastrointestinal tract of most animals, which are usually used as probiotics (20, 21). The results of the present study showed that dietary supplementation of Lactobacillus delbrueckii enhanced the ADG of the weaned piglets, which was consistent with the results of previous research that oral administration of Lactobacillus delhrueckii during the suckling period increased the ADG of pre-weaning piglets (11). One possible reason for the result could be the improvement in nutrient absorption. The phyla Firmicutes and Bacteroidetes were known for polysaccharide fermentation. It was reported that when the ratio of Firmicutes/Bacteroidetes improved, the host was able to absorb more energy from the diet, and the ability of energy storage was strengthened (22). Our results showed that Lactobacillus delhrueckii significantly increased the relative abundance of Firmicutes, but decreased the relative abundance of Bacteroidetes in the colon of the piglets. Additionally, there were more beneficial bacteria and less potential pathogenic bacteria in the Lactobacillus delhrueckii-treated group, which might contribute to the higher daily weight gain (22). In contrast, it was reported that dietary supplementation of multiple Lactobacillus (containing six strains of the genus Lactobacillus) has no effect on the daily weight gains of the weaning pigs (23). Such variable results might probably be caused by the variations in the genetic background, health condition, diet composition, and feeding method of the piglets.
The present study showed that dietary supplementation of Lactobacillus delhrueckii had limited effects on the serum biochemical parameters. Basal diet with 0.1% Lactobacillus delbrueckii did not affect the serum levels of AST, LDL, TC, and TG. These data indicated no negative actions of L. delbruecki on the lipid metabolism of the piglets. The HDL concentration of piglets in the LAC groups was higher on the 28th day than the CON group, indicating that supplementation of Lactobacillus delhrueckii enhanced the lipid utilization on the 28th day of the trial period. On the other hand, the TP concentration of piglets in the LAC group was higher on the 14th day. Changes in the serum level of TP reflected the utilization efficiency of proteins, that the serum total protein could be inhibited when the feed intake decreased (24). Our result indicated that supplementation of Lactobacillus delhrueckii could improve ADFI, representing the enhancement of the protein synthesis ability. The increased serum concentration of TP was suggested to be an indicator of enhanced immune capability, which was considered to be direct reference to the body's immune function (25). Lactobacillus delbrueckii did not affect the serum levels of AST and ALT of the piglets in the LAC group on the 28th day. AST and ALT were principally found in the liver and were considered to be biomarkers for liver cell damages (26). The lower ALT values in the piglets treated with Lactobacillus delhrueckii indicated that Lactobacillus delhrueckii might have protective effects on the liver functions. It was consistent with the previous result that Lactobacillus supplementation decreased the serum ALT levels in lactating sows (27). The effects of Lactobacillus delhrueckii on serum parameters might be related to the supplemented rate of Lactobacillus delhrueckii and the time range of the experiment.
In this study, we found that dietary supplementation of Lactobacillus delhrueckii had little effects on the intestinal mucosal morphology of piglets on the 14th day, but increased the villus height and V/C value in the ileum on the 28th day. A reduced number of proteobacteria and pH of the small intestine could improve the morphology of intestinal mucosa (28, 29). Increasing villus height and V/C value were widely recognized to improve the growth performance of piglets. The supplement of probiotics to weaning piglets and broilers caused higher villi in the small intestine and higher proliferation of the endothelial cell (30). However, the villus length, crypt depth, and goblet cell number in the small intestine of the weaned pig were not affected by the probiotic treatments (31). Such variable results might be attributed to the variations in the types of the probiotics, animals, and experiment times. Similarly, previous research demonstrated that oral administration of Lactobacillus delbrueckii during the suckling period improved the villus height (on day 21) and the V/C ratio (on days 21 and 28) of the jejunum in piglets after weaning (11). Hence, our results indicate that dietary supplement of Lactobacillus delbrueckii plays a beneficial role in improving the intestinal morphology and pH of the weaned piglets.
As observed in the present study, dietary Lactobacillus delhrueckii had significant effects on the Chao 1 and Shannon of alpha diversity indexes of the bacteria in the ileum, cecum, and colon. This result suggested that the diversity of microbial community changed with Lactobacillus delhrueckii supplementation. Dietary Lactobacillus delbrueckii had no effect on the phylum's abundance in the ileum of the weaned piglets. Basal diet with 0.1% Lactobacillus delbrueckii significantly increased the relative abundance of Firmicutes but decreased the relative abundance of Bacteroidetes in the colon. Taxonomic profiling data demonstrated that LAC increased the level of Firmicutes on the cecum and colon, but reduced the level of Actinobacteria on the colon. Supplementation of Lactobacillus-based fermentation product (1 × 1011 CFU Lactobacillus casei/kg) in the diet of weaned piglets was reported to reduce the population of E. coli in the cecum and Salmonella spp. in the ileum (32). Additionally, it was reported that the relative abundance of Firmicutes increased, and the relative abundance of Bacteroidetes decreased with an increasing body mass index (BMI) in humans (33). The dietary supplementation of Lactobacillus delbrueckii could increase the ADG of the weaned piglets. A possible explanation was that Firmicutes could promote carbohydrate absorption more effectively compared with the Bacteroidetes, therefore, causing more weight gain of the host (34, 35). The analysis of the bacterial community at the genus level in the ileum revealed that Lactobacillus delbrueckii significantly increased the relative abundance of the genus of Bifidobacterium and Lactobacillus, but decreased the relative abundance of the genus of unclassified_Clostridiales. It was reported that Lactobacillus was one of the main bacterial groups found in the gastrointestinal tract (36). The genus Bifidobacteria existed in the gastrointestinal tract in both animals and humans, and it helped to maintain the balance of microorganisms in the gastrointestinal tract by reducing the pathogenic microbes. Therefore, bifidobacteria was associated with good health status of the host (37, 38). Similarly, previous researches indicated that the addition of Lactobacilli isolated from gastrointestinal tract in piglets (Lactobacillus gasseri, L. reuteri, L. acidophilus, L. fermentum, L. johnsonii, and Lactobacillus mucosae) increased the numbers of lactobacilli and Bifidobacterium, and reduced the numbers of E. coli and aerobic bacteria in the jejunum, ileum, cecum, and colon mucosa (37, 39). The present experiment showed that the weaned piglets receiving the Lactobacillus delbrueckii diet had increased number of unidentified_RF16, Ruminococcus, unidentified_Ruminococcaceae, unidentified_lachnospiraceae, and unidentified Mogibacteriaceae, which were SCFA producers (40, 41). These results indicated that Lactobacillus delbrueckii could robust a more symbiotic intestinal microflora, which was a benefit to the host.
To further elucidate the underlying mechanisms of the administered Lactobacillus delbrueckii altering the gut health in piglets, the intestinal pH and the concentrations of SCFA in the colon were analyzed. Lactobacillus species could tolerate oxygen for short periods and alter the gut microbiota composition and the SCFA productions (42). As observed in the current study, dietary Lactobacillus delbrueckii significantly increased the relative abundance of the butyric acid, iso-butyric acid, and iso-valeric acid at the colon in phase II. The acetate produced by gut bacteria helped in reducing the permeability of the intestinal mucosal (39). In this study, the weaned piglets fed with a diet containing Lactobacillus delbrueckii had lower digesta pH in the duodenum and ileum, with a tendency toward a lower jejunum pH in phase II. In general, all Lactobacillus species could reduce pH by producing lactic acid as the final product in the carbohydrate fermentation, thus, inhibiting the colonization of the pathogenic bacteria (43). This finding was confirmed by our data showing that application of Lactobacillus delbrueckii produced higher SCFA in the colon and lowered the digesta pH in the duodenum and ileum of the piglets in phase II. Therefore, we concluded that Lactobacillus delbrueckii could improve the intestinal health of the piglets.
The present study demonstrates that dietary supplementation of 0.1% Lactobacillus delbrueckii could improve the mucosal morphology and cecal microflora of the weaned piglets, thus, improving the growth performance of the weaned piglets. These results indicate that Lactobacillus delbrueckii could be used as a potential feed supplement for weaned piglets.
The original contributions presented in the study are included in the article/Supplementary Material, further inquiries can be directed to the corresponding author/s.
The animal study was reviewed and approved by All the experimental procedures were approved by the Institutional Animal Care and Use Committee of Hunan Agricultural University (Changsha, China) (No. 2017-09).
X-LW and X-GH conceptualized the study and performed the validation. X-LW, Y-LL, and Y-HL formulated the methodology. L-YY provided the software. JY, X-LW, and Z-YL performed the formal analysis. Data curation was done by X-LW and Z-YL. X-LW wrote the original draft. D-XH, Z-YL, and J-HH reviewed and edited the manuscript. All authors contributed to the article and approved the submitted version.
This study was supported by the National Natural Science Foundation of China (31772617 and 31802074), the Hunan Innovation Technology Investment Project (2018GK5009), the Hunan Natural Science Foundation Youth Fund Project (2019JJ50264), and the Changsha Science and Technology Major Project (kq1902034).
Y-LL was employed by the company Hunan Pufeike Biotechnology.
The remaining authors declare that the research was conducted in the absence of any commercial or financial relationships that could be construed as a potential conflict of interest.
All claims expressed in this article are solely those of the authors and do not necessarily represent those of their affiliated organizations, or those of the publisher, the editors and the reviewers. Any product that may be evaluated in this article, or claim that may be made by its manufacturer, is not guaranteed or endorsed by the publisher.
The Supplementary Material for this article can be found online at: https://www.frontiersin.org/articles/10.3389/fvets.2021.692389/full#supplementary-material
1. McCracken BA, Spurlock ME, Roos MA, Zuckermann FA, Gaskins HR. Weaning anorexia may contribute to local inflammation in the piglet small intestine. J Nutr. (1999) 129:613–9. doi: 10.1093/jn/129.3.613
2. Xie C, Wang Q, Li G, Fan Z, Wang H, Wu, et al. Dietary supplement with nucleotides in the form of uridine monophosphate or uridine stimulate intestinal development and promote nucleotide transport in weaned piglets. J Sci Food Agric. (2019) 99:6108–13. doi: 10.1002/jsfa.9850
3. Montagne L, Boudry G, Favier C, Huërou-Luron IL, Lallès JP, Sève, et al. Main intestinal markers associated with the changes in gut architecture and function in piglets after weaning. Br J Nutr. (2007) 97:45–57. doi: 10.1017/S000711450720580X
4. Qin L, Ji W, Wang J, Li B, Hu J, Wu, et al. Effects of dietary supplementation with yeast glycoprotein on growth performance, intestinal mucosal morphology, immune response and colonic microbiota in weaned piglets. Food Funct. (2019) 10:2359–71. doi: 10.1039/C8FO02327A
5. Smith F, Clark JE, Overman BL, Tozel CC, Huang JH, Rivier JE, et al. Early weaning stress impairs development of mucosal barrier function in the porcine intestine. Am J Physiol. (2010) 298:352–63. doi: 10.1152/ajpgi.00081.2009
6. Fuller R. Probiotics in man and animals. J Appl Bacteriol. (1989) 66:365–78. doi: 10.1111/j.1365-2672.1989.tb05105.x
7. Giang HH, Viet TQ, Ogle B, Lindberg JE. Growth performance digestibility, gut environment and health status in weaned piglets fed a diet supplemented with potentially probiotic complexes of lactic acid bacteria. Livestock Sci. (2010) 129:95–103. doi: 10.1016/j.livsci.2010.01.010
8. He T, Zhu YH, Yu J, Xia B, Liu X, Yang GY, et al. Lactobacillus johnsonii L531 reduces pathogen load and helps maintain short-chain fatty acid levels in the intestines of pigs challenged with Salmonella enterica Infantis. Vet Microbiol. (2019) 230:187–94. doi: 10.1016/j.vetmic.2019.02.003
9. Giang HH, Viet TQ, Ogle B, Lindberg JE. Effects of Supplementation of Probiotics on the Performance, Nutrient Digestibility and Faecal Microflora in Growing-finishing Pigs. Asian Aust J Anim Sci. (2011) 24:655–61. doi: 10.5713/ajas.2011.10238
10. Liu H, Zhang J, Zhang S, Yang F, Thacker PA, Zhang G, et al. Oral administration of lactobacillus fermentumi5007 favors intestinal development and alters the intestinal microbiota in formula-fed piglets. J Agric Food Chem. (2014) 62:860–6. doi: 10.1021/jf403288r
11. Li Y, Hou S, Chen J, Peng W, Wen W, Chen F, et al. Oral administration of Lactobacillus delbrueckii during the suckling period improves intestinal integrity after weaning in piglets. J Funct Foods. (2019) 63:103591. doi: 10.1016/j.jff.2019.103591
12. Li Y, Hou S, Peng W, Lin Q, Chen F, Yang L, et al. Oral administration of lactobacillus delbrueckii during the suckling phase improves antioxidant activities and immune responses after the weaning event in a piglet model. Oxid Med Cell Longevity. (2019) 2019:6919803. doi: 10.1155/2019/6919803
13. Hou GF, Li R, Liu M, Wang HJ, Peng W, Huang G, et al. Effect of Lactobacillus delbrueckii on growth performance nutrient digestibilit, serum biochemical indexes and intestinal structure of fattening pigs. Chin J Anim Nutr. (2015) 27:2871–7. doi: 10.3969/j.issn.1006-267x.2015.09.026
14. Van Ginneken C, Van MF, Weyns A. Stereologic characteristics of pig small intestine during normal development. Dig Dis Science. (2002) 47:868–78. doi: 10.1023/a:1014768806773
15. Caporaso JG, Kuczynski J, Stombaugh K, Bittinger FD, Bushman EK, Costello N, et al. QIIME allows analysis of high-throughput community sequencing data. Nat Methods. (2010) 7:335–6. doi: 10.1038/nmeth.f.303
16. Gill SR, Pop M, DeBoy RT, Eckburg PB, Turnbaugh PJ, Samuel BS, et al. Metagenomic analysis of the human distal gut microbiome. Science. (2006) 312:1355–9. doi: 10.1126/science.1124234
17. Liu Z, Wang X, Ou S, Arowolo M, Hou D-X, He J, et al. Effects of achyranthes bidentata polysaccharides on intestinal morphology, immune response, and gut microbiome in yellow broiler chickens challenged with escherichia coli K88. Polymers. (2018) 10:1233. doi: 10.3390/polym10111233
18. Shen YB, Piao XS, Kim SW, Wang L, Liu P, Yoon I, et al. Effects of yeast culture supplementation on growth performance, intestinal health, and immune response of nursery pigs. J Anim Sci. (2009) 87:2614–24. doi: 10.2527/jas.2008-1512
19. Dowarah R, Verma AK, Agarwal N. The use of Lactobacillus as an alternative of antibiotic growth promoters in pigs: A review. Anim Nutr. (2017) 3:1–6. doi: 10.1016/j.aninu.2016.11.002
20. Chang YH, Kim JK, Kim HJ, Kim WY, Kim YB, Park, et al. Selection of a potential probiotic Lactobacillus strain and subsequent in vivo studies. Antonie Van Leeuwenhoek. (2001) 80:193–9. doi: 10.1023/A:1012213728917
21. Liu H, Ji HF, Zhang DY, Wang SX, Wang J, Shan DC, et al. Effects of Lactobacillus brevis preparation on growth performance, fecal microflora and serum profile in weaned pigs. Livestock Sci. (2015) 178:251–4. doi: 10.1016/j.livsci.2015.06.002
22. Ley RE, Backhed F, Turnbaugh P, Lozupone CA, Knight RD, Gordon, et al. Obesity alters gut microbial ecology. Proc Natl Acad Sci. (2005) 102:11070–5. doi: 10.1073/pnas.0504978102
23. Lähteinen T, Lindholm A, Rinttilä T, Junnikkala S, Kant R, Pietilä TE, et al. Effect of a multispecies lactobacillus formulation as a feeding supplement on the performance and immune function of piglets. Livestock Sci. (2015) 180:164–71. doi: 10.1016/j.livsci.2015.07.016
24. Zou Y, Wang Y, Deng Y, Cao Z, Li S, Wang, et al. Effects of feeding untreated, pasteurized and acidified waste milk and bunk tank milk on the performance, serum metabolic profiles, immunity, and intestinal development in Holstein calves. J Anim Sci Biotechnol. (2017) 8:53. doi: 10.1186/s40104-017-0182-4
25. Zhou H, Zhao Y, Liu. X. Path analysis of the relationship between serum protein contents and the growth traits in chicks. Acta veterinariaet zootechnica sinica (in Chinese). (1994) 25:301–5.
26. Johnston DE. Special considerations in interpreting liver function tests. Am Fam Physician. (1999) 59:2223–30.
27. Wang J, Ji HF, Hou CL, Wang SX, Zhang DY, Liu H, et al. Effects of Lactobacillus johnsonii XS4 supplementation on reproductive performance, gut environment, and blood biochemical and immunological index in lactating sows. Livestock Sci. (2014) 164:96–101. doi: 10.1016/j.livsci.2014.03.008
28. Suo C, Yin Y, Wang X, Lou X, Song D, Wang X, et al. Effects of lactobacillus plantarum ZJ316 on pig growth and pork quality. BMC Vet Res. (2012) 8:89. doi: 10.1186/1746-6148-8-89
29. Li D, Ni K, Pang H, Wang Y, Cai Y, Jin Q. Identification and antimicrobial activity detection of lactic acid bacteria isolated from corn Stover silage. Asian Aust J Anim Sci. (2015) 28:620–31. doi: 10.5713/ajas.14.0439
30. Hutsko SL, Meizlisch K, Wick M, Lilburn M. Early intestinal development and mucin transcription in the young poultry with probiotic and mannan oligosaccharide prebiotic supplementation. Poul Sci. (2016) 95:1173–8. doi: 10.3382/ps/pew019
31. Gareau MG, Sherman PM, Walker WA. Probiotics and the gut microbiota in intestinal health and disease. Nat Rev Gastroenterol Hepatol. (2010) 7:503–14. doi: 10.1038/nrgastro.2010.117
32. Jeong YD, Ko HS, Hosseindoust A, Choi YH, Chae BJ, Yu DJ, et al. Lactobacillus-based fermentation product and lactose level in the feed for weanling pigs: effects on intestinal morphology, microbiota, gas emission, and targeted intestinal coliforms. Livestock Sci. (2019) 227:90–6. doi: 10.1016/j.livsci.2019.06.018
33. Koliada A, Syzenko G, Moseiko V, Budovska L, Puchkov K, Perederiy V, et al. Association between body mass index and Firmicutes/Bacteroidetes ratio in an adult Ukrainian population. BMC Microbiol. (2017) 17:120. doi: 10.1186/s12866-017-1027-1
34. Turnbaugh PJ, Ley RE, Mahowald MA, Magrini V, Mardis ER, Gordon, et al. I. An obesity-associated gut microbiome with increased capacity for energy harvest. Nature. (2006) 444:1027–31. doi: 10.1038/nature05414
35. Krajmalnik-Brown R, Ilhan ZE, Kang DW, DiBaise JK. Effects of Gut Microbes on Nutrient Absorption and Energy Regulation. Nutr Clin Pract. (2010) 27:201–14. doi: 10.1177/0884533611436116
36. Dibner JJ, Richards JD. Antibiotic growth promoters in agriculture: history and mode of action. Poul Sci. (2005) 84:634–43. doi: 10.1093/ps/84.4.634
37. Huang C, Qiao S, Li D, Piao X, Ren J. Effects of Lactobacilli on the Performance, diarrhea incidence, VFA concentration and gastrointestinal microbial flora of weaning pigs. Asian Aust J Anim Sci. (2004) 17:401–9. doi: 10.5713/ajas.2004.401
38. Fukuda S, Toh H, Hase K, Oshima K, Nakanishi Y, Yoshimura K, et al. Bifidobacteria can protect from enteropathogenic infection through production of acetate. Nature. (2011) 469:543–7. doi: 10.1038/nature09646
39. Chiang ML, Chen HC, Chen KN, Lin YC, Lin YT, Chen, et al. Optimizing production of two potential probiotic Lactobacilli strains isolated from piglet feces as feed additives for weaned piglets. Asian-Aust J Anim Sci. (2015) 28:1163–70. doi: 10.5713/ajas.14.0780
40. Louis P, Young P, Holtrop G, Flint HJ. Diversity of human colonic butyrate-producing bacteria revealed by analysis of the butyryl-CoA:acetate CoA-transferase gene. Environ Microbiol. (2010) 12:304–14. doi: 10.1111/j.1462-2920.2009.02066.x
41. Meehan CJ, Beiko RG. A phylogenomic view of ecological specialization in the lachnospiraceae, a family of digestive tract-associated bacteria. Genome Biol Evol. (2014) 6:703–13. doi: 10.1093/gbe/evu050
42. Derrien M, van Hylckama Vlieg JE. Fate activity, and impact of ingested bacteria within the human gut microbiota. Trends Microbiol. (2015) 23:354–66. doi: 10.1016/j.tim.2015.03.002
Keywords: Lactobacillus delbrueckii, mucosal morphology, gut microbes, short chain fatty acids, weaned piglets
Citation: Wang X-L, Liu Z-Y, Li Y-H, Yang L-Y, Yin J, He J-H, Hou D-X, Liu Y-L and Huang X-G (2021) Effects of Dietary Supplementation of Lactobacillus delbrueckii on Gut Microbiome and Intestinal Morphology in Weaned Piglets. Front. Vet. Sci. 8:692389. doi: 10.3389/fvets.2021.692389
Received: 08 April 2021; Accepted: 19 July 2021;
Published: 19 August 2021.
Edited by:
Xiangfeng Kong, Institute of Subtropical Agriculture, Chinese Academy of Sciences, ChinaReviewed by:
Siaka Seriba Diarra, University of the South Pacific, FijiCopyright © 2021 Wang, Liu, Li, Yang, Yin, He, Hou, Liu and Huang. This is an open-access article distributed under the terms of the Creative Commons Attribution License (CC BY). The use, distribution or reproduction in other forums is permitted, provided the original author(s) and the copyright owner(s) are credited and that the original publication in this journal is cited, in accordance with accepted academic practice. No use, distribution or reproduction is permitted which does not comply with these terms.
*Correspondence: Zhu-Ying Liu, bGl1emh1eWluZ0BnbWFpbC5jb20=; Xing-Guo Huang, aHhnNjg5ODlAaHVuYXUuZWR1LmNu
Disclaimer: All claims expressed in this article are solely those of the authors and do not necessarily represent those of their affiliated organizations, or those of the publisher, the editors and the reviewers. Any product that may be evaluated in this article or claim that may be made by its manufacturer is not guaranteed or endorsed by the publisher.
Research integrity at Frontiers
Learn more about the work of our research integrity team to safeguard the quality of each article we publish.