- 1Key Laboratory of Animal Molecular Nutrition of Education of Ministry, National Engineering Laboratory of Biological Feed Safety and Pollution Prevention and Control, Key Laboratory of Animal Feed and Nutrition of Zhejiang Province, Institute of Animal Nutrition and Feed Sciences, College of Animal Sciences, Zhejiang University, Hangzhou, China
- 2School of Life Science and Engineering, Foshan University, Foshan, China
The aim of this study was to evaluate the dietary effects of Bacillus amyloliquefaciens SC06 (SC06) instead of antibiotics on the growth performance, intestinal health, and intestinal microbiota of broilers. A total of 360 30-day-old Lingnan yellow broilers were randomly allocated into two groups with six replicates per group (30 birds per replicate). The broilers were fed either a non-supplemented diet or a diet supplemented with 108 colony-forming units lyophilized SC06 per kilogram feed for 30 days. Results showed that SC06 supplementation had no effect on the growth performance compared with that of the control group. SC06 treatment significantly (P <0.05) increased the total antioxidant capacity (T-AOC), total superoxide dismutase (T-SOD) activity in the liver, and the activities of trypsin, α-amylase (AMS), and Na+K+-ATPase in the ileum, whereas it decreased (P < 0.05) lipase, gamma glutamyl transpeptidase (γ-GT), and maltase activities in the ileum. Meanwhile, SC06 treatment also improved the immune function indicated by the significantly (P < 0.05) increased anti-inflammatory cytokine [interleukin (IL)-10] level and the decreased (P < 0.05) pro-inflammatory cytokine [IL-6 and tumor necrosis factor (TNF)-α] levels in the ileum. Furthermore, we also found that SC06 enhanced the intestinal epithelial intercellular integrity (tight junction and adhesion belt) in the ileum. Microbial analysis showed that SC06 mainly increased the alpha diversity indices in the jejunum, ileum, and cecum. SC06 treatment also significantly (P < 0.05) increased the abundances of Bacteroidetes, Bacteroidales, Bacteroides, Fusobacteria, Clostridiaceae, and Veillonellaceae in the cecum and simultaneously decreased the abundances of Planococcaceae in the duodenum, Microbacteriaceae in the jejunum, and Lachnospiraceae, [Ruminococcus] and Ruminococcus in cecum. In conclusion, these results suggested that B. amyloliquefaciens instead of antibiotics showed a potential beneficial effect on the intestinal health of broilers.
Introduction
Over the past decades, the antimicrobial growth promoters (AGPs) have enjoyed great popularity in improving growth performance, reducing pathogenic bacterial colonization, and preventing gut disease in poultry (1, 2). However, with increasing public concerns about antibiotic-resistant bacteria and antibiotic-residual animal products, AGPs have been widely prohibited in animal husbandry in many countries (3–5). Subsequently, with the strict prohibition of the in-feed AGPs, gastrointestinal pathogenic infectious diseases in food animal production and zoonotic pathogen contamination in animal products seriously threaten the health of animals and humans (6, 7). Therefore, it is very important to explore the proper alternatives to antibiotics, such as direct-fed microbes (DFMs), prebiotics, antimicrobial peptides, plant extracts, immune activators, and organic acidifiers.
As live microorganisms, probiotics do not leave residues in food animal products and have been widely used in animal husbandry to improve growth performance and overall health and can be regarded as a potential substitute for AGPs (8–10). It is reported that probiotics, such as Bacillus spp., Lactobacillus, Bifidobacterium, and yeasts, play an important role in regulating birds' intestinal microbiota, inhibiting pathogens' growth, and modulating gastrointestinal immune responses (11–13). Our previous studies found that B. amyloliquefaciens SC06 (SC06) supplementation instead of antibiotics could significantly improve the growth performance of piglets via increasing antioxidant capacity and intestinal autophagy, suggesting that it could be used as a potential alternative to antibiotics in animal husbandry (9, 14). Moreover, SC06 could effectively protect intestinal porcine epithelial cell 1 (IPEC-1) from oxidative stress by regulating reactive oxygen species (ROS) production, activating the Nrf2/Keap1 signaling pathway, and promoting the elimination of Escherichia coli in murine macrophage RAW264.7 cells by activating autophagy (15). However, the effects of B. amyloliquefaciens SC06 on broilers' performance and healthy status remain unclear. Therefore, the objective of this study was to evaluate the effects of B. amyloliquefaciens SC06 as a potential antibiotic substitute on the growth performance, intestinal health, and intestinal microbiota of broilers.
Materials and Methods
Bacterial Preparation
Bacillus amyloliquefaciens SC06 was isolated from soil and deposited in the China Center for Type Culture Collection (CCTCC No. M 2012280). Bacteria were cultured in Luria–Bertani broth overnight at 37°C and then harvested by centrifugation at 5,000 rpm for 15 min. After washing twice with sterile phosphate-buffered saline (PBS; pH = 7.4), SC06 was resuspended in PBS and the concentration was constantly checked by the spreading plate method (16).
Birds, Diet, and Management
Three hundred sixty 30-day-old Lingnan yellow broilers were randomly allocated into two groups with six pens per group (30 birds per pen, stocking density 0.38 m2/bird). Broilers in the control group were fed with the basal diet containing colistin sulfate (10 g/ton) and zinc bacitracin (40 g/ton). Broilers in the SC06 group were fed with the same basal diet (without zinc bacitracin) supplemented with B. amyloliquefaciens SC06 (1 × 108 cfu/kg feed). The broiler experiment lasted for 30 days. All birds were allowed ad libitum to access water and diets. Feed consumption was recorded every day, and body weight was recorded on days 30 and 60. The composition of the basic diet (Table 1) was formulated to meet nutrient requirements of Chinese yellow-feathered broilers (17). Daylight was eliminated during this study, and 18-h lighting was provided from incandescent bulbs. Mortality was checked daily, and dead birds were weighed to adjust estimates of body weight gain, feed intake, and feed conversion ratio.
Sample Collection
At the 60th day of the trial, birds were deprived of feed for 4 h (05:00~09:00 a.m.) but not water. Six birds from each group were then chosen randomly and weighed. Then, the birds were electrically stunned, exsanguinated, and scalded to enable to collect tissues. The mucosa of ileum segments was gently scraped, along with the liver, snap frozen in liquid nitrogen, and stored at −80°C for further experiments.
Antioxidant Capacity Assay
The liver samples were homogenized with ice-cold sterile saline solution (1:9, w/v) and centrifuged at 5,000 rpm for 25 min at 4°C. Then, the collected supernatant was stored at −80°C for further enzyme activity assays. Briefly, after thawing the homogenates, adjusting to room temperature, the capacity of total antioxidant capacity (T-AOC), the activities of total superoxide dismutase (T-SOD), glutathione peroxidase (GSH-Px), xanthine oxidase (XOD), and superanion oxide (O2−) and content of glutathione (GSH) were analyzed by a SpectraMax M5 (Molecular Devices, USA) using assay kits (Nanjing Jiancheng Bioengineering Institute, Nanjing, China) according to the instructions of the manufacturer.
Digestive Enzyme Activity Assay
The ileum mucosa samples were homogenized with ice-cold sterile saline solution (1:9, w/v) and centrifuged at 5,000 rpm for 25 min at 4°C. Then, the collected supernatant was stored at −80°C for enzyme assays. Briefly, after thawing the homogenates, adjusting to room temperature, the activities of trypsin, lipase, α-amylase (AMS), gamma glutamyl transpeptidase (γ-GT), Na+K+ ATPase, sucrase, and maltase were analyzed by a SpectraMax M5 (Molecular Devices, USA) using assay kits (Nanjing Jiancheng Bioengineering Institute, Nanjing, China) according to the instructions of the manufacturer.
ELISA
The cytokine levels of interleukin (IL)-6, tumor necrosis factor (TNF)-α, IL-10, transforming growth factor (TGF)-β, interferon (IFN)-γ, IFN-α, and secretory immunoglobulin A (sIgA) in the ileum mucosa homogenates were determined by enzyme-linked immunosorbent assay (ELISA) kits (Bio-function Technology Co., Ltd., Beijing, China) according to the instructions.
Transmission Electron Microscopy
After fixing in 2.5% buffered glutaraldehyde, the ileum tissues were washed three times in cold 0.1 M phosphate buffer at every 15-min interval. The tissues were post-fixed in cold 0.1% buffered osmium tetroxide (OsO4) for 2 h and washed again in phosphate buffer. After rapidly dehydrating in an ascending serial ethanol solution (30%, 50%, 70%, 95%, and 100%), the tissues were then transferred to a 1:1 mixture of propylene oxide and epoxy araldite. After embedding, ultrathin sections (60–100 nm) were cut with an LKB Nova ultra-microtome (Leica Microsystems, Buffalo Grove, IL) and stained with uranyl acetate. Electron micrographs of intestinal mucosal cells and microvilli were captured by the transmission electron microscope (JEOL, Tokyo, Japan) operating at 80 kV.
Microbial Analysis
The DNA Isolation Kit (Tiangen, Beijing, China) was used for bacterial genomic DNA extraction from the contents of the duodenum, jejunum, ileum, and cecum, and the quality of the extracted DNA was checked by agarose gel electrophoresis and spectrophotometric analysis. All the genomic DNA samples were stored at −80°C for further experiments. Here, 16s rRNA PCR amplification and 454 pyrosequencing were performed according to a previous study (18). Sequences obtained through 454 pyrosequencing were then filtered by QIIME software (QIIME version 1.9.1) with default parameters. The operational taxonomic unit (OTU) clustering pipeline UPARSE was used to select OTU at 97% similarity. Alpha diversity and beta diversity between the samples were also analyzed by QIIME software. Beta diversity was displayed by principal coordinates analysis (PCoA) using the “ape” package of R software. Permutational multivariate analysis of variance (PERMANOVA) was calculated to determine significant differences in microbial community (based on the Bray–Curtis distance matrices). The linear discriminant analysis (LDA) effect size (LEfSe) analysis was performed online (https://huttenhower.sph.harvard.edu/galaxy/) to find out the highly dimensional intestinal microbes and characterize the differences between the two groups. The biomarkers were then analyzed and visualized by statistical analysis of taxonomic and functional profiles (STAMP) software with a two-sided Welch's t-test (19).
Co-occurrence networks of the microbial communities in the different intestinal segments between the two groups were built based on significant correlations [Spearman's R > 0.6 and false discovery rate (FDR)-adjusted P < 0.05] (20) and were visualized by Gephi software (https://gephi.org/). The complex patterns of the interrelationships were described by the topological properties of co-occurrence network calculated by Gephi software.
Statistical Analysis
The rest of the data were analyzed by two-tailed Student's t-test using SPSS 20.0 (SPSS Inc., Chicago, IL, USA), and results were expressed as mean ± standard error of the mean (SEM).
Results
Growth Performance
As shown in Table 2, compared with the control group, SC06 treatment had no (P > 0.05) effect on the growth performance, including the final body weight, the average daily feed intake, the average daily gain, and the ratio of feed conversion, of broilers.
Antioxidant Capacity in Liver
As shown in Table 3, SC06 treatment significantly (P < 0.05) increased the T-AOC capacity and T-SOD activity of liver but had no (P > 0.05) effect on the content of GSH and O2− and the activities of GSH-Px and XOD.
Digestive Enzyme Activities in the Ileum
Compared with the control group, SC06 treatment significantly (P < 0.05) increased the activities of trypsin, AMS, and Na+K+-ATPase in the ileum, whereas it significantly (P < 0.05) decreased the activities of lipase, γ-GT, and maltase (Table 4).
Cytokine Levels in the Ileum
SC06 treatment significantly (P < 0.05) increased IL-10 level and decreased the concentrations of IL-6 and TNF-α in the ileum, whereas it had no effect (P > 0.05) on the levels of TGF-β, IFN-γ, IFN-α, and sIgA (Table 5).
Transmission Electron Micrograph
TEM results showed that the ileum of the broilers fed with SC06 showed ordered arrangement, higher microvillus, and longer tight junction (TJ) and adhesion belt (AB) compared with broilers fed with antibiotics (Figure 1).
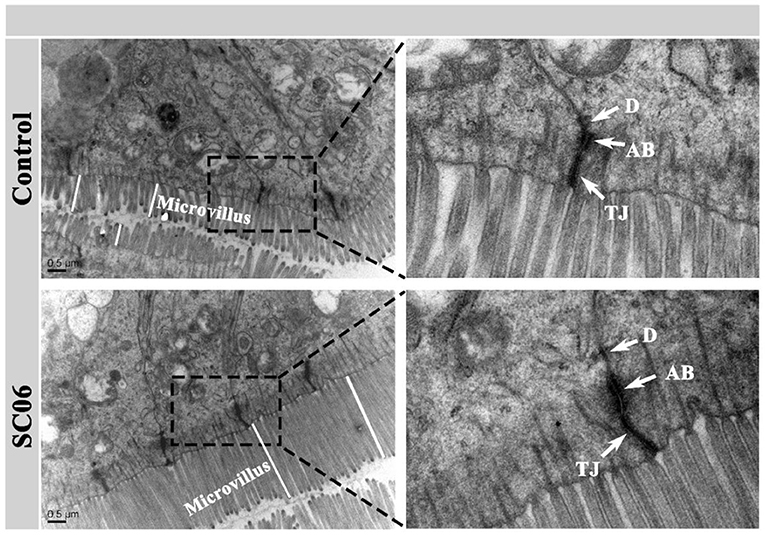
Figure 1. Transmission electron micrographs (TEMs) of the ileal microvilli in broilers. TJ, tight junction; AB, adhesion belt; D, desmosome.
Intestinal Microbiota Analysis
The intestinal microbiota plays an important role in the overall health of the broilers. We found that SC06 treatment significantly (P < 0.05) increased the alpha diversity indices (including Observed species, Chao1, PD_whole_tree, and Ace) of ileal microbiota and the indices of Observed species and PD_whole_tree in the jejunum (Figure 2). SC06 treatment also increased (P > 0.05) the alpha diversity indices of cecal and duodenal microbiota. PCoA of microbial communities based on Bray–Curtis distance revealed that bacterial communities from the ileum and cecum formed distinct clusters but had no significant differences between the two groups, which was further confirmed by PERMANOVA analysis (ileum: R2 = 0.21, P = 0.20; cecum: R2 = 0.48, P = 0.10) (Figure 3).
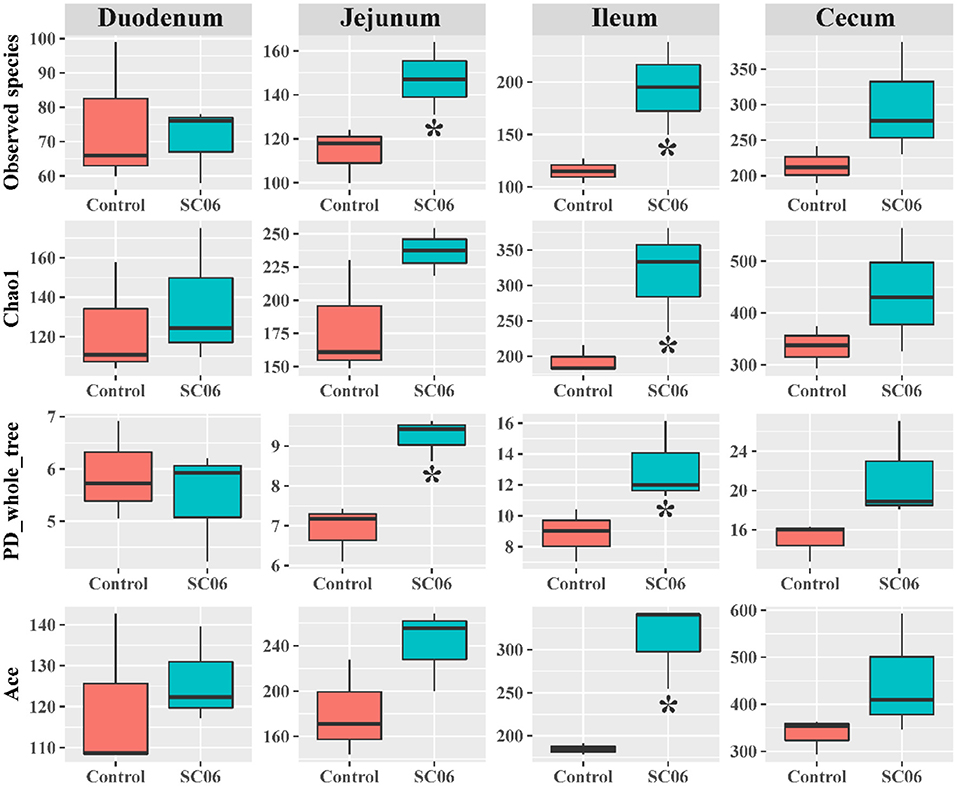
Figure 2. Alpha diversity index analysis of the intestinal microbiota between the two groups (n = 3 birds/group). *P < 0.05.
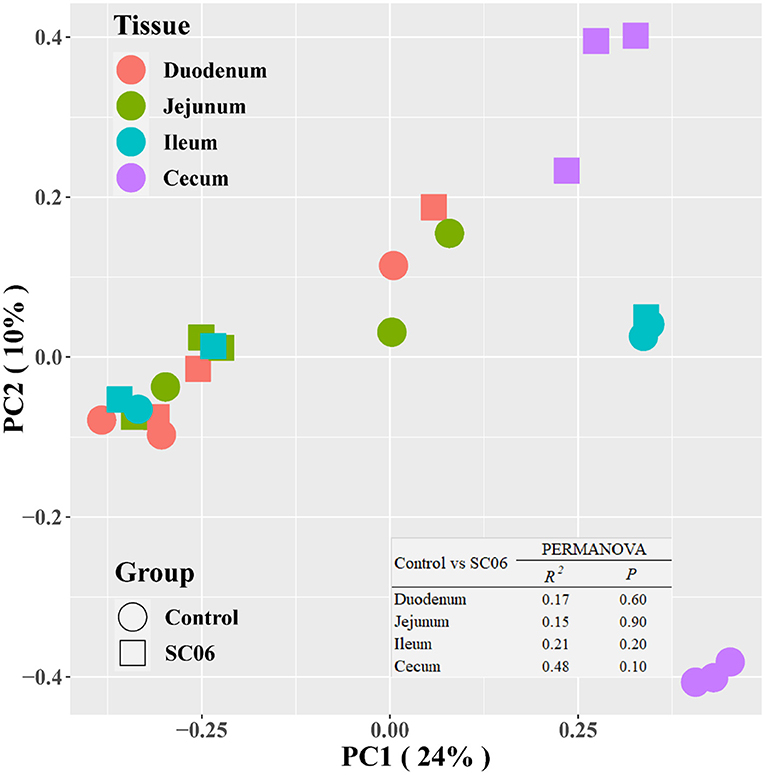
Figure 3. Microbial community analyzed by principal coordinates analysis (PCoA) based on Bray–Curtis distance. PERMANOVA, permutational multivariate analysis of variance (n = 3 birds/group).
LEfSe analysis showed that 68 biomarkers were identified with LDA scores >2, of which 61 biomarkers were identified in cecal microbiota (Figure 4). The SC06 group was enriched with Proteobacteria in the duodenum and Facklamia in the jejunum, while the control group was enriched with Planococcaceae in the duodenum, Microbacteriaceae in the jejunum, and Cyanobacteria, Chloroplast, and Streptophyta in the ileum. In the cecum, SC06 group was enriched with 49 biomarkers, most of which belonged to Firmicutes, Proteobacteria, and Bacteroidetes, while the control group was enriched with 12 biomarkers that belonged to Firmicutes. Welch's t-test was further employed to explore the differences in the microbial composition between the two groups (Figure 5). The results showed that the SC06 treatment significantly (P < 0.05) increased the abundances of Bacteroidetes, Bacteroidales, Bacteroides, Fusobacteria, Clostridiaceae, and Veillonellaceae in the cecum and simultaneously (P < 0.05) decreased the abundances of Planococcaceae in the duodenum, Microbacteriaceae in the jejunum, and Lachnospiraceae, [Ruminococcus] and Ruminococcus in cecum.
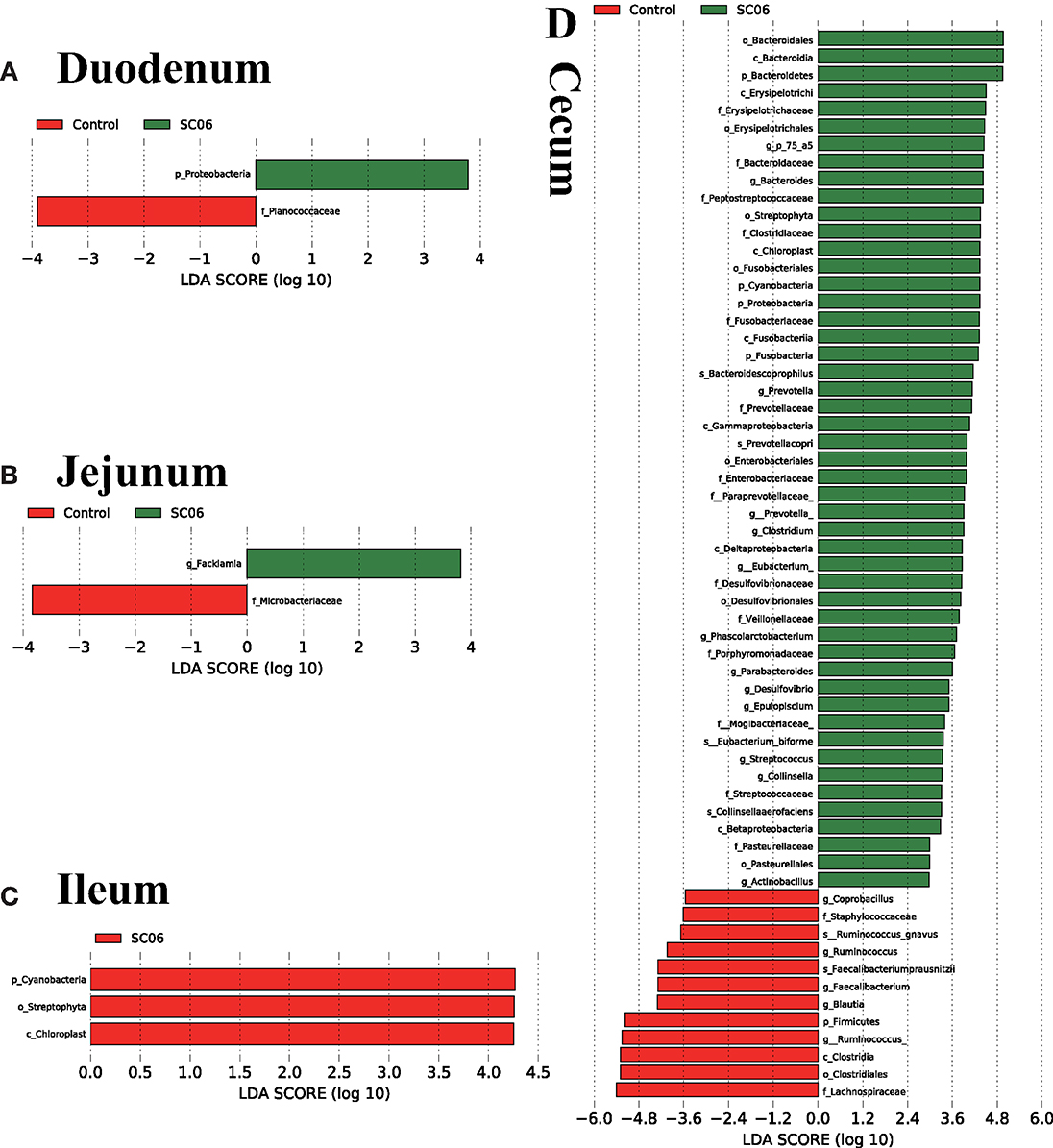
Figure 4. Linear discriminant analysis (LDA) effect size (LEfSe) analysis (P < 0.05, LDA >2.0) showing the biomarker taxa (n = 3 birds/group). The prefixes “p,” “c,” “o,” “f,” “g,” and “s” represent the annotated levels of phylum, class, order, family, genus, and species. (A) duodenum, (B) jejunum, (C) ileum, (D) cecum.
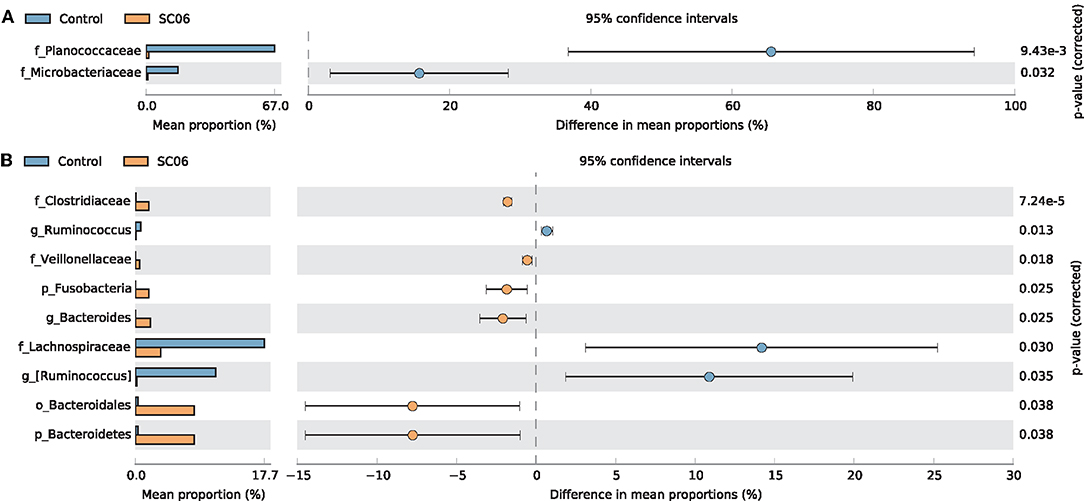
Figure 5. Comparison of the intestinal microbiota between the two groups by statistical analysis of taxonomic and functional profiles (STAMP). (A) Differences of Planococcaceae in the duodenum and Microbacteriaceae in the jejunum. (B) Differences of intestinal microbiota in the cecum.
To determine the co-occurrence patterns of intestinal microbiota in all groups, eight networks were constructed based on OTU levels (Figure 6, Table 6). The results showed that the microbial networks of SC06 group had more nodes (OTUs) and edges than those of the control group in the duodenum, jejunum, and ileum, except in the cecum. The values of average degree of SC06 group were higher than those of the control group in the duodenum, jejunum, ileum, and cecum. The values of graph density of SC06 group were lower than those of the control group in the duodenum and jejunum, whereas these were higher than those of the control group in the ileum and cecum. The modularity values of all the co-occurrence networks in the two groups were higher than 0.4. Additionally, a negative correlation of the network of the SC06 group was more than those of the control group in the jejunum and ileum, whereas it higher than that of the control group in the duodenum.
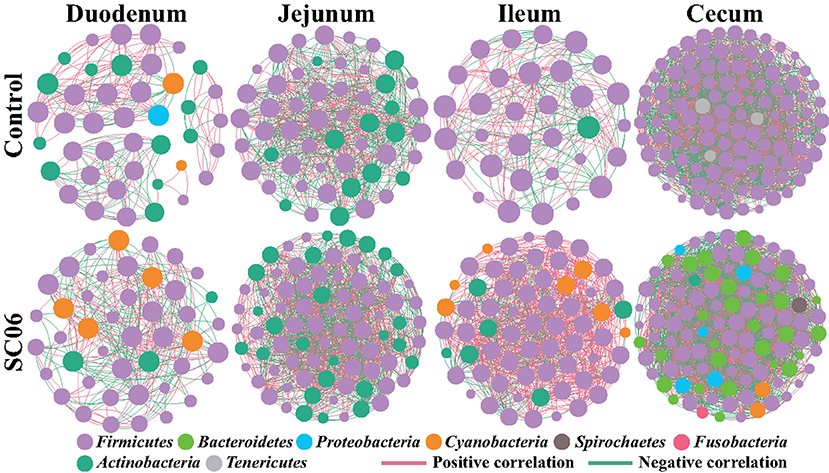
Figure 6. Co-occurrence networks of microbial communities based on Spearman correlation analysis sorted in color by operational taxonomic unit (OTU) level. A connection stands for a very strong (Spearman's R > 0.6) and significant [false discovery rate (FDR)-adjusted P < 0.05] correlation. The size of each node is proportional to the relative abundance; the thickness of each connection between two nodes is proportional to the value of Spearman's correlation coefficients. Red lines represent significant positive correlations, and green lines denote negative correlations.
Discussion
Because of the issues of antibiotic-resistant bacteria and antibiotic-residual animal products, many countries have forbidden the use of antibiotics in animal husbandry (4). As an emerging green antibiotic substitute, probiotics are thought to be beneficial for the animals' health by maintaining the presence of beneficial microorganisms, enhancing digestive capacity, improving the mucosal immunity, and inhibiting pathogen adherence in the intestine (21, 22). Previous studies had reported that probiotics administration achieved a better effect on broilers' growth-related matrices than antibiotic administration (23–27). However, the beneficial effects of probiotics instead of antibiotics on broilers' growth performance are not always consistent, which are dependent on specific probiotic strains, the concentrations used, and the feeding stage of animals (28, 29). Many scientific literatures also documented that as an AGP alternative, probiotics had a similar effect on improving growth performance and gut health (8, 9, 21). In the current study, antibiotic-added basal diet was used as a control group to simulate the commercial intensive rearing mode, and a similar effect on the growth-related matrices of birds was observed in the two groups, which might imply that SC06 administration instead of antibiotics could bring similar economic benefits in the industrial farming of broilers.
Oxidative stress reflects an imbalance between the production of ROS (free radicals) and antioxidants, which leads to cellular dysfunctions and cell death (30). The major antioxidant defense system of the host is composed of antioxidant enzymes and biological antioxidants, of which T-AOC and SOD are the most important (31, 32). The elevated T-AOC capacity and SOD activity reflects the increase of antioxidant capacity (33). Liver redox environment is critical for the functions of the organ in nutrient digestion, so the redox status of the liver is vital for host health (34). In poultry husbandry, oxidative stress is ubiquitous, which restricts the growth performance of broilers (35, 36). There were several researches that demonstrated that probiotics could serve as a natural antioxidant to protect the host against oxidative stress-induced damage (37–39). Our results found that the T-AOC capacity and T-SOD activity in liver were significantly enhanced in the SC06 group, which is consistent with the previous findings that probiotics played a beneficial role in oxidative defenses (9, 40, 41).
Digestive enzymes play an important role in the digestion of nutrients into smaller nutrient molecules to facilitate the absorption by the host. Previous studies showed that probiotics had a positive effect in promoting intestinal digestive enzyme activities of broilers (42, 43). However, the positive results are not always consistent. Some literatures also found that probiotics did not affect the activities of intestinal digestive enzymes of broilers (44). The current study showed that probiotic SC06 significantly enhanced the activities of trypsin, AMS, and Na+K+-ATPase, while it markedly decreased lipase, γ-GT, and maltase activities in the ileum of broilers. These findings indicated that SC06 might play a biased beneficial role in degrading feed proteins and long-chain starch into smaller peptides, amino acids, short-chain dextrin, maltose, or glucose in order to be easily absorbed by the intestinal mucosa (45).
In the poultry industry, the immunosuppression induced by overcrowding, stress, and pathogenic infections can result in serious reductions in birds' growth performance, quality of poultry products, and economic benefits (46–48). Therefore, induction and maintenance of a proper level of immunologic function are vital for broiler healthy growth (23). Inflammatory cytokines secreted by the immune system play a key role in preventing against bacterial or viral infectious diseases and balancing the immune homeostasis (49). The pro-inflammatory cytokines (IL-6, TNF-α) and anti-inflammatory cytokines (IL-10, TGF-β) are key inflammatory cytokines that endorse cell-mediated immunity and regulate cytokine secretion homeostasis (50, 51). Many documents reported that probiotics could modulate host immune function (52, 53) and have been widely used in animal and human in order to enhance the disease resistance capacity (54, 55). As a natural immune modulator, probiotics could improve the immune response of broilers by increasing inflammatory cytokines and thus protect broilers against pathogens, coccidia, viruses, and stress (56, 57). In the present study, probiotic SC06 significantly increased the anti-inflammatory cytokine (IL-10) and decreased the pro-inflammatory cytokines (IL-6 and TNF-α) in the ileum, which implies that probiotic SC06 could be used as a potential immune modulator to regulate the intestinal immune function of broilers.
One of the most important functions of the intestinal mucosa is to act as an intestinal epithelial barrier that consists of the tight junction, adhesion belt, and desmosomes (58). The intestinal epithelial barriers play a vital role in resisting invasion of pathogens and the maintenance of mucosal homeostasis (59, 60). Many studies have reported that supplementation with probiotics improved the intestinal ultrastructure of broilers (35, 61). Similarly, we also found that SC06 treatment enhanced the intercellular connectivity in ileal mucosa, as evidenced by longer tight junctions and adhesion belts, indicating that SC06 could improve the intestinal epithelial barriers of broilers.
Gut microbiota plays an important role in the digestive tract of animals (62). The composition of the intestinal microbial community is greatly influenced by dietary interaction (63). Previous studies have demonstrated that dietary probiotics have a positive effect in modulating the intestinal microbiota (21, 23, 64). Supplementation with probiotics could promote the presence of beneficial bacteria and reduce potential harmful bacteria populations in the intestinal tract of chickens (65, 66) and reestablish pathogen-induced intestinal microbial dysfunction (67). In the present study, dietary SC06 mainly increased the alpha diversity indices in the jejunum, ileum, and cecum, indicating that SC06 improved the microbial diversity of the above intestinal segments. LefSe results showed that SC06 induced differentially enriched bacterial species at different taxonomic levels, especially in the cecum. The SC06 group was totally enriched with 51 biomarkers (microbial taxa) in the duodenum, jejunum, and cecum, most of which belonged to Firmicutes, Proteobacteria, and Bacteroidetes. We further found that among the identified 68 biomarkers (microbial taxa) in the two groups, 11 biomarkers were calculated to be significantly different between the two groups by Welch's t-test analysis. Dietary SC06 significantly increased the abundances of Bacteroidetes, Bacteroidales, Bacteroides, Fusobacteria, Clostridiaceae, and Veillonellaceae in the cecum. Bacteroides genus can metabolize a variety of plant- and animal-derived glycans and improve the immune function and mucosal barrier function of animals (68, 69). It is reported that Fusobacteria activate the inflammatory responses of the host to protect against pathogens that promote tumor growth (70). Clostridiaceae mediates starch breakdown and lactic acid fermentation (71) and is one of the three key families in dogs to digest the intestinal protein and energy (72). Veillonellaceae was reported to produce high levels of the short-chain fatty acids (acetate and propionate) (73). Simultaneously, SC06 treatment decreased the abundances of Planococcaceae in the duodenum, Microbacteriaceae in the jejunum, and Lachnospiraceae and Ruminococcus in the cecum. Planococcaceae family previously was described in association with vertebrate carrion (74). Lachnospiraceae was reported to increase in patients with non-alcoholic fatty liver disease (75). It was reported that antibiotic (zinc bacitracin) treatment increased the relative abundance of Ruminococcus, but the role of this genus in broilers remains to be further investigated (76, 77). The above results implied that SC06 supplementation may exert beneficial effects on modulating the intestinal microbiota of broilers.
Finally, the co-occurrence network analysis was employed to investigate microbial interactions. In this study, we found that the values of edges and average degree of the microbial networks in the SC06 group were higher than those in the control group in the different intestinal segments, suggesting that SC06 treatment increased the connection among the intestinal microbiota (78). The modularity values of all the co-occurrence networks in the two groups in different intestinal segments were higher than 0.4, suggesting that these microbial networks had a modular structure (79). Additionally, negative connection of the network in the SC06 group was less than that in the control group in the jejunum and ileum, which could be interpreted as a reduction in competitive relationships within the intestinal microbiota (80).
Conclusion
In conclusion, B. amyloliquefaciens SC06 instead of antibiotics is beneficial for the health of broilers by improving the antioxidant capacity of the liver, digestive function and immune response of the intestinal mucosa, and intestinal epithelial barrier and modulating the intestinal microbiota. However, further investigations about the involved and interacted roles of gut microbiota in SC06-mediated benefits are needed.
Data Availability Statement
The datasets presented in this study can be found in online repositories. The names of the repository/repositories and accession number(s) can be found at: NCBI SRA BioProject, accession no: PRJNA720313.
Ethics Statement
The animal study was reviewed and approved by the Institutional Animal Care and Use Committee of Zhejiang University, P. R. China.
Author Contributions
W-FL contributed to the conceptualization, supervision, and project administration. BW and YZ contributed to the microbial analysis, writing of the original draft, and writing, review, and editing. BW, YZ, and LT contributed to the animal experiments. ZZ, LG, and YW assisted with the experiments and manuscript preparation. All authors have read and agreed to the published version of the manuscript.
Funding
This study was supported by the Natural Science Foundation of Zhejiang province, China (Nos. 2006C12086 and 2015C02022) and the Education Foundation of Da Bei Nong Group.
Conflict of Interest
The authors declare that the research was conducted in the absence of any commercial or financial relationships that could be construed as a potential conflict of interest.
References
1. M'Sadeq SA, Wu S, Swick RA, Choct M. Towards the control of necrotic enteritis in broiler chickens with in-feed antibiotics phasing-out worldwide. Anim Nutr. (2015) 1:1–11. doi: 10.1016/j.aninu.2015.02.004
2. Tellez G, Latorre JD. Editorial: alternatives to antimicrobial growth promoters and their impact in gut microbiota, health and disease. Front Vet Sci. (2017) 4:196. doi: 10.3389/fvets.2017.00196
3. Laxminarayan R, Van Boeckel T, Teillant A. The economic costs of withdrawing antimicrobial growth promoters from the livestock sector. In: OECD Food, Agriculture and Fisheries Papers. Paris (2015). p. 75. doi: 10.1787/5js64kst5wvl-en
4. Martin MJ, Thottathil SE, Newman TB. Antibiotics overuse in animal agriculture: a call to action for health care providers. Am Public Health Assoc. (2015). 105:2409–10. doi: 10.2105/AJPH.2015.302870
5. Walsh TR, Wu Y. China bans colistin as a feed additive for animals. Lancet Infect Dis. (2016) 16:1102–3. doi: 10.1016/S1473-3099(16)30329-2
6. Marshall BM, Levy SB. Food animals and antimicrobials: impacts on human health. Clin Microbiol Rev. (2011) 24:718–33. doi: 10.1128/Cmr.00002-11
7. Teillant A, Brower CH, Laxminarayan R. Economics of antibiotic growth promoters in livestock. Annu Rev Resour Econ. (2015) 7:349–74. doi: 10.1146/annurev-resource-100814-125015
8. Giang HH, Viet TQ, Ogle B, Lindberg JE. Effects of different probiotic complexes of lactic acid bacteria on growth performance and gut environment of weaned piglets. Livestock Sci. (2010) 133:182–4. doi: 10.1016/j.livsci.2010.06.059
9. Wang Y, Wu Y, Wang B, Cao X, Fu A, Li Y, et al. Effects of probiotic Bacillus as a substitute for antibiotics on antioxidant capacity and intestinal autophagy of piglets. AMB Express. (2017) 7:52. doi: 10.1186/s13568-017-0353-x
10. Rehman A, Arif M, Sajjad N, Al-Ghadi M, Alagawany M, Abd El-Hack M, et al. Dietary effect of probiotic and prebiotic on broiler performance, carcass and immunity. Poultry Sci. (2020) 99: 6946–53. doi: 10.1016/j.psj.2020.09.043
11. Dec M, Puchalski A, Nowaczek A, Wernicki A. Antimicrobial activity of Lactobacillus strains of chicken origin against bacterial pathogenss. Int Microbiol. (2016) 19:57–67. doi: 10.2436/20.1501.01.264
12. Zhou M, Zeng D, Ni X, Tu T, Yin Z, Pan K, et al. Effects of Bacillus licheniformis on the growth performance and expression of lipid metabolism-related genes in broiler chickens challenged with Clostridium perfringens-induced necrotic enteritis. Lipids Health Dis. (2016) 15:48. doi: 10.1186/s12944-016-0219-2
13. Allen SJ. The potential of probiotics to prevent Clostridium difficile infection. Infect Dis Clin. (2015) 29:135–44. doi: 10.1016/j.idc.2014.11.002
14. Ji J, Hu S, Zheng M, Du W, Shang Q, Li W. Bacillus amyloliquefaciens SC06 inhibits ETEC-induced pro-inflammatory responses by suppression of MAPK signaling pathways in IPEC-1 cells and diarrhea in weaned piglets. Livestock Sci. (2013) 158:206–14. doi: 10.1016/j.livsci.2013.09.017
15. Wang Y, Wu Y, Wang Y, Fu A, Gong L, Li W, et al. Bacillus amyloliquefaciens SC06 alleviates the oxidative stress of IPEC-1 via modulating Nrf2/Keap1 signaling pathway and decreasing ROS production. Appl Microbiol Biotechnol. (2017) 101:3015–26. doi: 10.1007/s00253-016-8032-4
16. Nikoskelainen S, Ouwehand AC, Bylund G, Salminen S, Lilius E-M. Immune enhancement in rainbow trout (Oncorhynchus mykiss) by potential probiotic bacteria (Lactobacillus rhamnosus). Fish Shellfish Immunol. (2003) 15:443–52. doi: 10.1016/S1050-4648(03)00023-8
18. Qin C, Gong L, Zhang X, Wang Y, Wang Y, Wang B, et al. Effect of Saccharomyces boulardii and Bacillus subtilis B10 on gut microbiota modulation in broilers. Anim Nutr. (2018) 4:358–66. doi: 10.1016/j.aninu.2018.03.004
19. Parks DH, Tyson GW, Hugenholtz P, Beiko RG. STAMP: statistical analysis of taxonomic and functional profiles. Bioinformatics. (2014) 30:3123–4. doi: 10.1093/bioinformatics/btu494
20. Jiao S, Liu Z, Lin Y, Yang J, Chen W, Wei G. Bacterial communities in oil contaminated soils: biogeography and co-occurrence patterns. Soil Biol Biochem. (2016) 98:64–73. doi: 10.1016/j.soilbio.2016.04.005
21. Wang Y, Du W, Fu A, Zhang X, Huang Y, Lee K, et al. Intestinal microbiota and oral administration of Enterococcus faecium associated with the growth performance of new-born piglets. Beneficial Microbes. (2016) 7:529–38. doi: 10.3920/BM2015.0099
22. Callaway T, Edrington T, Anderson R, Harvey R, Genovese K, Kennedy C, et al. Probiotics, prebiotics and competitive exclusion for prophylaxis against bacterial disease. Anim Health Res Rev. (2008) 9:217–25. doi: 10.1017/S1466252308001540
23. Gao P, Ma C, Sun Z, Wang L, Huang S, Su X, et al. Feed-additive probiotics accelerate yet antibiotics delay intestinal microbiota maturation in broiler chicken. Microbiome. (2017) 5:91. doi: 10.1186/s40168-017-0315-1
24. Gadde U, Oh S, Lee Y, Davis E, Zimmerman N, Rehberger T, et al. The effects of direct-fed microbial supplementation, as an alternative to antibiotics, on growth performance, intestinal immune status, and epithelial barrier gene expression in broiler chickens. Probiot Antimicrob Prot. (2017) 9:397–405. doi: 10.1007/s12602-017-9275-9
25. Yang J, Zhan K, Zhang M. Effects of the use of a combination of two bacillus species on performance, egg quality, small intestinal mucosal morphology, and cecal microbiota profile in aging laying hens. Probiot Antimicrob Proteins. (2019) 12:204–13. doi: 10.1007/s12602-019-09532-x
26. Li X, Wu S, Yan T, Duan Y, Yang X, Duan Y, et al. Simultaneous supplementation of Bacillus subtilis and antibiotic growth promoters by stages improved intestinal function of pullets by altering gut microbiota. Front Microbiol. (2018) 9:2328. doi: 10.3389/fmicb.2018.02328
27. Wealleans A, Sirukhi M, Egorov I. Performance, gut morphology and microbiology effects of a Bacillus probiotic, avilamycin and their combination in mixed grain broiler diets. Br Poult Sci. (2017) 58:523–9. doi: 10.1080/00071668.2017.1349298
28. Behnsen J, Deriu E, Sassone-Corsi M, Raffatellu M. Probiotics: properties, examples, and specific applications. Cold Spring Harb Perspect Med. (2013) 3:a010074. doi: 10.1101/cshperspect.a010074
29. Bai S, Wu A, Ding X, Lei Y, Bai J, Zhang K, et al. Effects of probiotic-supplemented diets on growth performance and intestinal immune characteristics of broiler chickens. Poult Sci. (2013) 92:663–70. doi: 10.3382/ps.2012-02813
30. Finkel T. Oxidant signals and oxidative stress. Curr Opin Cell Biol. (2003) 15:247–54. doi: 10.1016/S0955-0674(03)00002-4
31. Riedl MA, Saxon A, Diaz-Sanchez D. Oral sulforaphane increases Phase II antioxidant enzymes in the human upper airway. Clin Immunol. (2009) 130:244–51. doi: 10.1016/j.clim.2008.10.007
32. Cho H-Y, Reddy SP, Kleeberger SR. Nrf2 defends the lung from oxidative stress. Antioxid Redox Signal. (2006) 8:76–87. doi: 10.1089/ars.2006.8.76
33. Wang Y, Wang Y, Wang B, Mei X, Jiang S, Li W. Protocatechuic acid improved growth performance, meat quality, and intestinal health of Chinese yellow-feathered broilers. Poult Sci. (2019) 98:3138–49. doi: 10.3382/ps/pez124
34. Mari M, Colell A, Morales A, von Montfort C, Garcia-Ruiz C, Fernandez-Checa JC. Redox control of liver function in health and disease. Antioxid Redox Signal. (2010) 12:1295–331. doi: 10.1089/ars.2009.2634
35. Wang Y, Wang Y, Xu H, Mei X, Gong L, Wang B, et al. Direct-fed glucose oxidase and its combination with B. amyloliquefaciens SC06 on growth performance, meat quality, intestinal barrier, antioxidative status, and immunity of yellow-feathered broilers. Poult Sci. (2018) 97:3540–9. doi: 10.3382/ps/pey216
36. Bai K, Huang Q, Zhang J, He J, Zhang L, Wang T. Supplemental effects of probiotic Bacillus subtilis fmbJ on growth performance, antioxidant capacity, and meat quality of broiler chickens. Poult Sci. (2016) 96:74–82. doi: 10.3382/ps/pew246
37. Gong L, Wang B, Mei X, Xu H, Qin Y, Li W, et al. Effects of three probiotic Bacillus on growth performance, digestive enzyme activities, antioxidative capacity, serum immunity, and biochemical parameters in broilers. Anim Sci J. (2018) 89:1561–71. doi: 10.1111/asj.13089
38. Wu Y, Wang B, Zeng Z, Liu R, Tang L, Gong L, et al. Effects of probiotics Lactobacillus plantarum 16 and Paenibacillus polymyxa 10 on intestinal barrier function, antioxidative capacity, apoptosis, immune response, and biochemical parameters in broilers. Poult Sci. (2019) 98:5028–39. doi: 10.3382/ps/pez226
39. Wang Y, Wu Y, Wang Y, Xu H, Mei X, Yu D, et al. Antioxidant properties of probiotic bacteria. Nutrients. (2017) 9:521. doi: 10.3390/nu9050521
40. Tang W, Xing Z, Hu W, Li C, Wang J, Wang Y. Antioxidative effects in vivo and colonization of Lactobacillus plantarum MA2 in the murine intestinal tract. Appl Microbiol Biotechnol. (2016) 100:7193–202. doi: 10.1007/s00253-016-7581-x
41. Rajput IR, Li YL, Xu X, Huang Y, ZhiWC, Yu DY, et al. Supplementary effects of Saccharomyces boulardii and Bacillus subtilis B10 on digestive enzyme activities, antioxidation capacity and blood homeostasis in broiler. Int J Agric Biol. (2013) 15:231–7. doi: 10.1071/CP13079
42. Zhang L, Zhang L, Zeng X, Zhou L, Cao G, Yang C. Effects of dietary supplementation of probiotic, Clostridium butyricum, on growth performance, immune response, intestinal barrier function, and digestive enzyme activity in broiler chickens challenged with Escherichia coli K88. J Anim Sci Biotechnol. (2016) 7:3. doi: 10.1186/s40104-016-0061-4
43. Jin L, Ho Y, Abdullah N, Jalaludin S. Digestive and bacterial enzyme activities in broilers fed diets supplemented with Lactobacillus cultures. Poult Sci. (2000) 79:886–91. doi: 10.1093/ps/79.6.886
44. Lima ACFd, Pizauro Júnior JM, Macari M, Malheiros EB. Effect of probiotic supplementation on performance and digestive enzymes activity of broiler chickens. Rev Brasil Zoot. (2003) 32:200–7. doi: 10.1590/S1516-35982003000100025
45. Hu S, Cao X, Wu Y, Mei X, Xu H, Wang Y, et al. Effects of probiotic Bacillus as an alternative of antibiotics on digestive enzymes activity and intestinal integrity of piglets. Front Microbiol. (2018) 9:2427. doi: 10.3389/fmicb.2018.02427
46. Gomes A, Quinteiro-Filho WM, Ribeiro A, Ferraz-de-Paula V, Pinheiro M, Baskeville E, et al. Overcrowding stress decreases macrophage activity and increases Salmonella enteritidis invasion in broiler chickens. Avian Pathol. (2014) 43:82–90. doi: 10.1080/03079457.2013.874006
47. Gimeno I, Schat K. Virus-induced immunosuppression in chickens. Avian Dis. (2018) 62:272–85. doi: 10.1637/11841-041318-Review.1
48. Shini S, Huff G, Shini A, Kaiser P. Understanding stress-induced immunosuppression: exploration of cytokine and chemokine gene profiles in chicken peripheral leukocytes. Poult Sci. (2010) 89:841–51. doi: 10.3382/ps.2009-00483
50. Boden EK, Snapper SB. Regulatory T cells in inflammatory bowel disease. Curr Opin Gastroenterol. (2008) 24:733–41. doi: 10.1097/MOG.0b013e328311f26e
51. Rajput IR, Li WF. Potential role of probiotics in mechanism of intestinal immunity. Pak Vet J. (2012) 32:2531. doi: 10.1501/Vetfak_0000002531
52. Isolauri E, Sütas Y, Kankaanpää P, Arvilommi H, Salminen S. Probiotics: effects on immunity. Am J Clin Nutr. (2001) 73:444s−50. doi: 10.1093/ajcn/73.2.444s
53. Borchers AT, Selmi C, Meyers FJ, Keen CL, Gershwin ME. Probiotics and immunity. J Gastroenterol. (2009) 44:26–46. doi: 10.1007/s00535-008-2296-0
54. Tanoue T, Morita S, Plichta DR, Skelly AN, Suda W, Sugiura Y, et al. A defined commensal consortium elicits CD8 T cells and anti-cancer immunity. Nature. (2019) 565:600. doi: 10.1038/s41586-019-0878-z
55. Li J, Sung CYJ, Lee N, Ni Y, Pihlajamäki J, Panagiotou G, et al. Probiotics modulated gut microbiota suppresses hepatocellular carcinoma growth in mice. Proc Natl Acad Sci. (2016) 113:E1306–15. doi: 10.1073/pnas.1518189113
56. Smith JM. A review of avian probiotics. J Avian Med Surg. (2014) 28:87–95. doi: 10.1647/2012-031
57. Dhama K, Verma V, Sawant P, Tiwari R, Vaid R, Chauhan R. Applications of probiotics in poultry: Enhancing immunity and beneficial effects on production performances and health-a review. J Immunol Immunopathol. (2011) 13:1–19.
58. Odenwald MA, Turner JR. The intestinal epithelial barrier: a therapeutic target? Nature reviews Gastroenterol Hepatol. (2017) 14:9. doi: 10.1038/nrgastro.2016.169
59. Choi W, Yeruva S, Turner JR. Contributions of intestinal epithelial barriers to health and disease. Exp Cell Res. (2017) 358:71–7. doi: 10.1016/j.yexcr.2017.03.036
60. Turner JR. Intestinal mucosal barrier function in health and disease. Nat Rev Immunol. (2009) 9:799–809. doi: 10.1038/nri2653
61. Rajput I, Li L, Xin X, Wu B, Juan Z, Cui Z, et al. Effect of Saccharomyces boulardii and Bacillus subtilis B10 on intestinal ultrastructure modulation and mucosal immunity development mechanism in broiler chickens. Poult Sci. (2013) 92:956–65. doi: 10.3382/ps.2012-02845
62. Marchesi JR, Adams DH, Fava F, Hermes GD, Hirschfield GM, Hold G, et al. The gut microbiota and host health: a new clinical frontier. Gut. (2016) 65:330–9. doi: 10.1136/gutjnl-2015-309990
63. Power SE, O'Toole PW, Stanton C, Ross RP, Fitzgerald GF. Intestinal microbiota, diet and health. Br J Nutr. (2014) 111:387–402. doi: 10.1017/S0007114513002560
64. Kristensen NB, Bryrup T, Allin KH, Nielsen T, Hansen TH, Pedersen O. Alterations in fecal microbiota composition by probiotic supplementation in healthy adults: a systematic review of randomized controlled trials. Genome Med. (2016) 8:52. doi: 10.1186/s13073-016-0300-5
65. Forte C, Acuti G, Manuali E, Casagrande Proietti P, Pavone S, Trabalza-Marinucci M, et al. Effects of two different probiotics on microflora, morphology, and morphometry of gut in organic laying hens. Poult Sci. (2016) 95:2528–35. doi: 10.3382/ps/pew164
66. Peng Q, Zeng X, Zhu J, Wang S, Liu X, Hou C, et al. Effects of dietary Lactobacillus plantarum B1 on growth performance, intestinal microbiota, and short chain fatty acid profiles in broiler chickens. Poult Sci. (2016) 95:893–900. doi: 10.3382/ps/pev435
67. Bhat MI, Sowmya K, Kapila S, Kapila R. Potential probiotic Lactobacillus rhamnosus (MTCC-5897) inhibits Escherichia coli impaired intestinal barrier function by modulating the host tight junction gene response. Probiot Antimicrob Prot. (2019) 12:1149–60. doi: 10.1007/s12602-019-09608-8
68. Thomas F, Hehemann JH, Rebuffet E, Czjzek M, Michel G. Environmental and gut bacteroidetes: the food connection. Front Microbiol. (2011) 2:93. doi: 10.3389/fmicb.2011.00093
69. Wexler AG, Goodman AL. An insider's perspective: bacteroides as a window into the microbiome. Nat Microbiol. (2017) 2:17026. doi: 10.1038/nmicrobiol.2017.26
70. Kelly D, Yang L, Pei Z. Gut microbiota, fusobacteria, and colorectal cancer. Diseases. (2018) 6: 109. doi: 10.3390/diseases6040109
71. D'Andreano S, Sanchez Bonastre A, Francino O, Cusco Marti A, Lecchi C, Grilli G, et al. Gastrointestinal microbial population of turkey (Meleagris gallopavo) affected by hemorrhagic enteritis virus. Poult Sci. (2017) 96:3550–8. doi: 10.3382/ps/pex139
72. Bermingham EN, Maclean P, Thomas DG, Cave NJ, Young W. Key bacterial families (Clostridiaceae, Erysipelotrichaceae and Bacteroidaceae) are related to the digestion of protein and energy in dogs. Peerj. (2017) 5:e3019. doi: 10.7717/peerj.3019
73. Lecomte V, Kaakoush NO, Maloney CA, Raipuria M, Huinao KD, Mitchell HM, et al. Changes in gut microbiota in rats fed a high fat diet correlate with obesity-associated metabolic parameters. PLoS ONE. (2015) 10:e0126931. doi: 10.1371/journal.pone.0126931
74. Metcalf JL, Carter DO, Knight R. Microbiome studies of carrion decomposition. In: Benbow M, Tomberlin J, Tarone A, editors. Carrion Ecology, Evolution, and Their Applications. Boca Raton, FL: CRC Press (2020). p. 421–32.
75. Shen F, Zheng RD, Sun XQ, Ding WJ, Wang XY, Fan JG. Gut microbiota dysbiosis in patients with non-alcoholic fatty liver disease. Hepatob Pancreat Dis. (2017) 16:375–81. doi: 10.1016/S1499-3872(17)60019-5
76. Crisol-Martinez E, Stanley D, Geier MS, Hughes RJ, Moore RJ. Understanding the mechanisms of zinc bacitracin and avilamycin on animal production: linking gut microbiota and growth performance in chickens. Appl Microbiol Biot. (2017) 101:4547–59. doi: 10.1007/s00253-017-8193-9
77. Yang C, Kennes YM, Lepp D, Yin X, Wang Q, Yu H, et al. Effects of encapsulated cinnamaldehyde and citral on the performance and cecal microbiota of broilers vaccinated or not vaccinated against coccidiosis. Poult Sci. (2020) 99:936–48. doi: 10.1016/j.psj.2019.10.036
78. Jiao S, Yang Y, Xu Y, Zhang J, Lu Y. Balance between community assembly processes mediates species coexistence in agricultural soil microbiomes across eastern China. ISME Journal. (2020) 14:202–16. doi: 10.1038/s41396-019-0522-9
79. Newman ME. Modularity and community structure in networks. Proc Natl Acad Sci. (2006) 103:8577–82. doi: 10.1073/pnas.0601602103
Keywords: antioxidant capacity, digestive function, immune function, intestinal epithelial barrier, intestinal microbiota, Bacillus amyloliquefaciens
Citation: Wang B, Zhou Y, Tang L, Zeng Z, Gong L, Wu Y and Li W-F (2021) Effects of Bacillus amyloliquefaciens Instead of Antibiotics on Growth Performance, Intestinal Health, and Intestinal Microbiota of Broilers. Front. Vet. Sci. 8:679368. doi: 10.3389/fvets.2021.679368
Received: 11 March 2021; Accepted: 20 April 2021;
Published: 04 June 2021.
Edited by:
Guillermo Tellez, University of Arkansas, United StatesReviewed by:
Anaisa Leyva, National Autonomous University of Mexico, MexicoBruno Vecchi Galenda, Vetanco S.A., Argentina
Copyright © 2021 Wang, Zhou, Tang, Zeng, Gong, Wu and Li. This is an open-access article distributed under the terms of the Creative Commons Attribution License (CC BY). The use, distribution or reproduction in other forums is permitted, provided the original author(s) and the copyright owner(s) are credited and that the original publication in this journal is cited, in accordance with accepted academic practice. No use, distribution or reproduction is permitted which does not comply with these terms.
*Correspondence: Wei-Fen Li, d2ZsaUB6anUuZWR1LmNu
†ORCID: Baikui Wang orcid.org/0000-0001-5283-7337 Wei-Fen Li orcid.org/0000-0001-8159-9876