- 1The Innovation Centre of Ruminant Precision Nutrition and Smart and Ecological Farming, Jilin Agricultural Science and Technology University, Jilin City, China
- 2Jilin Inter-regional Cooperation Centre for the Scientific and Technological Innovation of Ruminant Precision Nutrition and Smart and Ecological Farming, Jilin City, China
- 3Portal Agri-Industries Co., Ltd., Nanjing, China
- 4Angel Yeast Co., Ltd., Yichang, China
- 5School of Agriculture and Environment, Massey University, Palmerston North, New Zealand
- 6College of Animal Science and Technology, Yangzhou University, Yangzhou, China
There is a growing interest in the use of yeast (Saccharomyces cerevisiae) culture (YC) for the enhancement of growth performance and general animal health. Grain-based pelleted total mixed rations (TMR) are emerging in intensive sheep farming systems, but it is uncertain if the process of pelleting results in YC becoming ineffective. This study aimed to examine the effects of YC supplemented to pelleted TMR at two proportions of corn in the diet on animal performance, feed digestion, blood parameters, rumen fermentation, and microbial community in fattening lambs. A 2 × 2 factorial design was adopted with two experimental factors and two levels in each factor, resulting in four treatments: (1) low proportion of corn in the diet (LC; 350 g corn/kg diet) without YC, (2) LC with YC (5 g/kg diet), (3) high proportion of corn in the diet (HC; 600 g corn/kg diet) without YC, and (4) HC with YC. Fifty-six 3-month-old male F2 hybrids of thin-tailed sheep and Northeast fine-wool sheep with a liveweight of 19.9 ± 2.7 kg were randomly assigned to the four treatment groups with an equal number of animals in each group. The results showed that live yeast cells could not survive during pelleting, and thus, any biological effects of the YC were the result of feeding dead yeast and the metabolites of yeast fermentation rather than live yeast cells. The supplementation of YC resulted in 31.1 g/day more average daily gain regardless of the proportion of corn in the diet with unchanged feed intake during the 56-day growth measurement period. The digestibility of neutral detergent fibre and acid detergent fibre was increased, but the digestibility of dry matter, organic matter, and crude protein was not affected by YC. The supplementation of YC altered the rumen bacterial population and species, but the most abundant phyla Bacteroidetes, Firmicutes, and Proteobacteria remained unchanged. This study indicates that YC products can be supplemented to pelleted TMR for improved lamb growth performance, although live yeast cells are inactive after pelleting. The improved performance could be attributed to improved fibre digestibility.
Introduction
Sheep is of economic importance in Northeast China (1). Sheep farming in the area is shifting from grazing or grazing plus supplements to intensive indoor feeding with a high proportion of grain included in the diet. The indoor feeding system enables feed additives to be extensively used for the manipulation of rumen fermentation and improved animal performance. There has been increasing pressure from both consumers and governments to remove antibiotics and synthetic chemical compounds from animal industries. This has led to increased research in the use of other feed additives as alternative growth promoters in sheep (2).
One of the common feed additives used by farmers and researchers is yeast culture (YC) (3), which is a natural product produced from yeast fermentation. YC contains, in addition to live and dead yeast cells, culture media and the products of metabolism, including proteins, polysaccharides (mainly β-glucans and mannan-oligosaccharides), lipids, vitamins, peptides, amino acids, nucleotides, organic acids, and antioxidants (4). YC supplementation has a range of beneficial effects, including increased feed intake (5), stabilisation of rumen pH (6, 7), increased feed digestion (8), enhancement of overall immunity (9, 10), and consequently improved animal performance (3, 5).
To increase growth rates in sheep production, farmers tend to increase the proportion of cereal grains in the diet (11). However, a high-grain diet can affect the rumen environment by reducing rumen pH, consequently resulting in disorders of the digestive system in ruminants (12, 13). Thus, one of the key goals when feeding sheep is to maintain the optimal rumen environment for growth. High-yielding dairy cows fed a large amount of grain in their diets can suffer the same problem. To solve the problem, YC is supplemented to the diet of dairy cows because of its role in the stabilisation of rumen pH (6, 7). YC may have a similar effect on the rumen pH of sheep when fed high-grain diets (14).
Feeding total mixed rations (TMR) has been popular due to its advantages of providing balanced nutrients in the diet, the relatively stable rumen environment, reduced feed sorting, and better animal performance in dairy cows (15). TMR feeding has been further developed by pelleting the TMR and fed to cattle (16–18) and sheep (19–23). Feeding of a pelleted TMR results in almost no feed selection by animals, non-palatable ingredients can be included in the diet, minimisation of feed loss during storage and feeding, and improved ease of feeding management and labour efficiency (11).
Pelleting is a critical step in the manufacture of pelleted TMR and involves steam conditioning, high temperature, and high pressure. These processes could cause changes to the nutritional value of the diet through chemical alterations (24) and could potentially eliminate the beneficial effects of YC. However, pelleting does not result in the disappearance of the beneficial effects of YC in non-ruminant animals (25, 26). Therefore, whether or not the effects of YC remain after pelleting when supplemented to TMR is uncertain.
We hypothesise that YC supplemented to pelleted TMR still has beneficial effects on fattening lambs. The objective of the study was to examine the effects of supplementing YC to pelleted TMR containing two proportions of corn in the diet on animal performance, digestibility, blood metabolites, rumen fermentation parameters, and ruminal microbial communities of fattening lambs.
Materials and Methods
Experimental Design and Animals
The experiment was conducted in a 2 × 2 factorial design with four treatments in total. The four treatments were (1) low proportion of corn in the diet (LC; 350 g corn/kg diet) without yeast (Saccharomyces cerevisiae) culture (YC) supplemented (CON), (2) LC with YC supplemented at the 5 g/kg diet, (3) high proportion of corn in the diet (HC; 600 g corn/kg diet) without YC, and (4) HC with YC supplemented at the 5 g/kg diet. The dose of YC at the 5 g/kg diet was recommended by the YC producer. The proportion of corn at the 350 g/kg diet was recommended by researchers (23), and the high proportion (600 g corn/kg diet) is used by farmers in the region.
Sixty male F2 hybrid lambs of thin-tailed sheep and Northeast fine-wool sheep, the most popular breed in the region (1), aged 3 months with a liveweight of 19.9 ± 2.7 kg, were used in the experiment. They were de-wormed, examined twice for brucellosis using the Rose Bengal plate test (Bruceila spp.) (27), and then adapted gradually from hay to pelleted TMR for 1 week and fed completely with pelleted TMR for another 2 weeks. All lambs were brucellosis negative. After the adaption period, the well-adapted and healthy lambs were randomly allocated to one of the four treatments after stratification for liveweight (n = 14 per treatment).
All lambs were individually weighed fortnightly for 8 weeks. The weighing was conducted at the same time of the day before the morning feeding each time using an electronic scale for livestock with a precision of 0.05 kg (Dahe Electronics Co., Ltd., Wuyi, Zhejiang, China). Average daily gain (ADG) was estimated using a plot of liveweight against time.
On day 56 after weighing, blood samples were collected randomly from six lambs from each treatment before the morning feeding. On day 70, rumen samples were randomly collected before the morning feed from 12 lambs to characterise the rumen microbial profile. On the same day, six lambs were randomly removed from each treatment for a digestibility trial and another six lambs were removed for slaughter. At the end of the digestibility trial, rumen samples were taken on day 82 at 3 h after morning feeding on these lambs for the determination of fermentation characteristics. Slaughter was performed on days 71 and 72 with three lambs each day for each treatment group. The remaining two lambs were released from the experiment on day 70.
Feed and Feeding
The ingredients and nutrient contents of the experimental diets are presented in Table 1. According to the Chinese Feeding Standard of Meat-producing Sheep and Goats (NY/T 816-2004) (28), the diets were formulated with nitrogen content adjusted to be similar for the LC and HC diets. YC (®Bailike) specified for ruminants contained 200 g/kg mannan, 200 g/kg β-glucan, 200 g/kg crude protein (CP), and 80 g/kg moisture and was obtained from Angel Yeast Co., Ltd., Hubei, China. Premix, mineral supplements, and YC product were not ground, but other ingredients were ground to pass a 4/6 mm screen (a half part of the screen with holes having a diameter of 4 mm and another half with holes with a diameter of 6 mm). All ingredients were mixed thoroughly and steam conditioned for 10 s first, and then pelleting was performed at 85°C using a pelleting press (model YPM508E, Jiangsu Yongli Machinery Co., Ltd., Liyang, Jiangsu, China). Pelleted TMR was 3.2 mm in diameter. All the diets used in the experiment were produced at the Shanxi Subsidiary Company of Jiangsu Portal Agri-Industries Co., Ltd., China, in a single batch for each diet. The experimental diets were bagged and transferred to the experimental site and stored in a well-ventilated room for a month before use. At the beginning of the formal experimental period, feed samples were collected in triplicate for the count of live yeast.
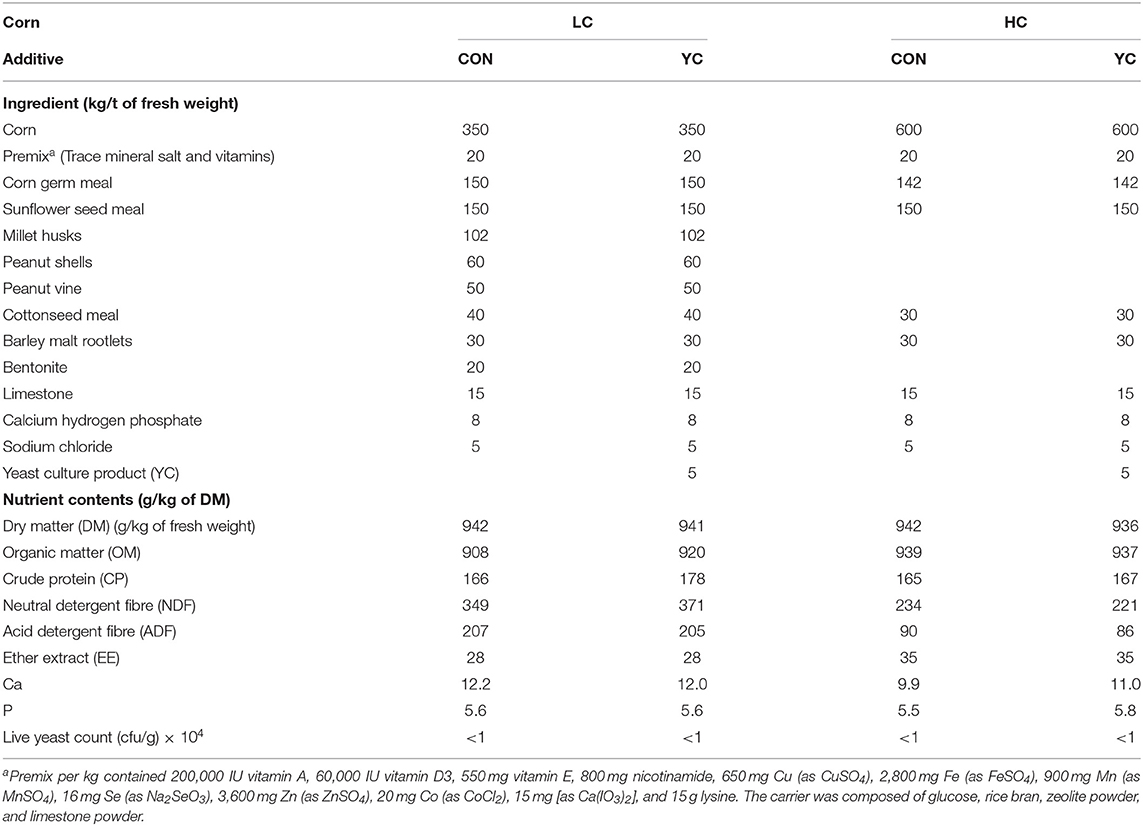
Table 1. Ingredients and nutrient contents of experimental diets containing a low (LC) or high (HC) proportion of corn and supplemented with nil (CON) or yeast culture (YC).
All lambs were kept in the same room with a ventilation fan that operated for 24 h a day. No bedding was provided, but the pens were cleaned every morning before feeding. Lambs were offered feed twice a day with equal proportions at 08:00 and 16:00 h. Feed allowance was such that 10–20% of the feed offered was not eaten. Feed offered and refused was quantified daily for the estimation of feed intake. Lambs were group fed with 14 in each pen, except during the digestibility measurement period when lambs were individually fed in metabolic crates. Water was available at all times.
Apparent Total Tract Digestibility Measurement
The digestibility trial began on day 70 and was carried out over 12 days. Lambs were given 6 days for adaption and 6 days for sample collection. Feed offered and refused, and the outputs of faeces and urine were collected and quantified daily using the total faeces collection technique (29). One-fifth of faeces was subsampled each day with half acidified for CP analysis only and another half not acidified for the analysis of other nutrients. The subsamples were pooled for each animal over the duration of the 6-day sampling period. Urine was collected in a bucket containing 100 ml of 6 M H2SO4 and stirred twice daily to homogenise urine and acid. Urine volume was measured, and one-tenth was subsampled and pooled for each animal. Faeces and urine subsamples were stored at −20°C. Feed refusals were not subsampled daily but pooled for each animal. At the end of the trial, daily feed samples were pooled for each dietary treatment. Feed, refusal, and faeces were dried at 65°C for 48 h, ground to pass a 1-mm sieve and sealed in bottles for chemical composition analysis.
Rumen and Blood Sampling
Rumen samples (ca. 50 ml) for the analysis of rumen fermentation parameters were taken using an oesophageal tube with the first 50 ml discarded to minimise saliva contamination in a procedure described by Huo et al. (21). pH value was immediately measured using a pH metre (LICHEN pH-100 A, Shanghai Lichen Scientific Laboratory Instrument Ltd., Shanghai, China). The samples for the rumen microbial community analysis were kept in 10-ml tubes, immediately placed in liquid nitrogen, and then stored at −80°C. Rumen samples for the analysis of fermentation parameters were kept on ice in a chilly bin and transferred to the laboratory within 0.5 h. Subsamples were taken and stored in 2-ml cryogenic vials (Corning Inc., New York, USA) at −20°C until further analysis. Blood was sampled from the jugular vein and placed in a coagulation promoting tube (5 mL) with separating gel (Sanli Industrial Co., Ltd., Huizhou, China). Serum was separated by centrifuging at 1,000 × g for 5 min (Model TDL-80-2B; Anting Scientific Instrument Factory, Shanghai, China) and then stored at −20°C.
Slaughter
After fasting for 24 h, lambs were weighed and slaughtered, and hot carcass weight (HCW), backfat thickness, loin eye area, and fresh organs were quantified. HCW was recorded with kidneys included. The dressing percentage was calculated as HCW divided by live body (BW) ×100. Backfat thickness was measured in the method of Kirton et al. (30). The loin eye area was estimated using the equation that eye muscle area = loin eye width and loin eye height × 0.7 (31), where the width and height were measured from the cut surface of longissimus dorsi muscle between the 12th and 13th ribs using a digital Vernier calliper with a precision of 0.01 mm (DL91150; Deli Group Ltd, Linbo, Zhejiang, China). Fresh organs were measured using an electronic scale with a precision of 1 g (DH-2012; Diheng Electronic Co., Ltd, Shenzhen, Guangdong, China). Organ indexes were calculated as organ weights divided by live BW.
Laboratory Analysis
The live yeast cells in the YC products and diets were counted under a microscope after staining with alkaline methylene blue (32).
Feed samples were determined for dry matter (DM) (33), ash (34), CP [method no. 968.06; (35)], neutral detergent fibre (NDF) (36), acid detergent fibre (ADF) (36), ether extract (EE) (37), Ca (38), and P (39). Organic matter (OM) was calculated as 1,000 – ash. The NDF was assayed with a heat-stable amylase and expressed exclusive of residual ash. The ADF was consecutively determined after NDF determination and expressed exclusive of residual ash. Refusal and faeces samples were determined for DM, ash, CP, NDF and ADF, and acidified faeces samples for CP only. Urine samples were determined only for CP.
Rumen samples were determined for ammonia concentration in a modified Indigo phenol blue-spectrophotometry method (40). Short-chain fatty acids (SCFA) were identified and quantified using gas chromatography (Model GC-9A; Shimadzu Co., Japan) coupled with a flame ionisation detector (FID) and a polar capillary column (CP-WAX, 30 m × 0.53 mm × 1 μm) in a procedure described by Wang et al. (41). Microbial protein (MCP) was quantified using the spectrophotometric and HPLC methods of Zinn and Owens (42) as modified by Makkar and Becker (43) with yeast RNA as a standard.
Serum metabolites were analysed after thawing in a fridge overnight using an automatic biochemical analyser (Model 7160; Hitachi Ltd., Tokyo, Japan) with reagents from Mairui Biomedical Electronics Co., Ltd. (Shenzhen, China).
Rumen Microbial Community Analysis
After the extraction of total genome DNA, bacterial 16S rRNA gene regions were amplified using PCR primers (341F: 5′-CCTAYGGGRBGCASCAG-3′; 806R: 5′-GGACTACNNGGGTATCTAAT-3′) as described previously (21). After sequencing libraries were generated and quality ensured, sequencing was conducted on the Illumina MiSeq platform (Illumina, Inc., San Diego, CA, USA) to generate 250 bp/300 bp paired-end reads and merged using FLASH (44). Sequence analysis was performed by the UPARSE software package using the UPARSE-OTU and UPARSE-OTU ref algorithms (45). Sequences with a similarity over 97% were treated as the same operational taxonomic unit (OTU). The classification and annotation of each OTU were performed by the RDP classifier (http://rdp.cme.msu.edu) against the Silva 123 (www.arb-silva.de) 16S rRNA database updated for ruminal bacteria (46). The relative abundances of taxa and diversity indices, including Chao, Shannon and Simpson, were estimated using the Mothur software package v.1.21.1 (47).
Statistical Analysis
A two-way ANOVA was performed with the proportion of corn in the diet and the supplementation of YC as the experimental factors using the GenStat 19th edition statistical software (48). The multiple comparisons among treatments were performed using the Duncan method. The effect was declared as a significant difference if P < 0.05 and a trend if P < 0.10.
Results
Live Yeast Counts and Growth Performance
The number of live yeast cells was 2 × 108 colony-forming units (cfu)/g in the YC product, and <10 × 103 cfu/g in the diets supplemented either with or without YC.
Corn proportion and its interaction with YC supplementation had no significant effects on ADG and the final BW at the end of the growth performance measurement period, but YC supplementation tended to increase the final BW by 2.6 kg (P = 0.063) and increased ADG by 31 g/day (9.6%; P = 0.019) compared with CON (Table 2).

Table 2. Growth performance of fattening lambs fed experimental diets containing a low (LC) or high (HC) proportion of corn and supplemented with nil (CON) or yeast culture (YC) (n = 14 per treatment).
Apparent Total Tract Digestibility
There were no significant interactions between the corn proportion and YC supplementation on DM and nutrient intake, digestibility, and N balance (Table 3). The supplementation of YC did not affect DM and OM intake and the digestibility of DM, OM, and CP, but resulted in an increase in NDF digestibility by 46 g/kg (P = 0.031) and in ADF digestibility by 71 g/kg (P = 0.014). The YC supplementation tended to reduce N output from urine (P = 0.067) and total N output (P = 0.045) per unit of N intake and consequently increased the proportion of retained N (P = 0.045). The increase in the proportion of corn in the diet decreased nutrient intake (P ≤ 0.004) and increased nutrient digestibility (P ≤ 0.016). The HC reduced daily N intake (P < 0.001) and daily faecal (P < 0.001) and urinary (P = 0.008) N outputs and did not change daily N retention overall. In terms of per unit of N intake, HC resulted in a decrease in faecal N output (P = 0.002), although there was no significant change in urinary N output and N retention was similar between LC and HC.
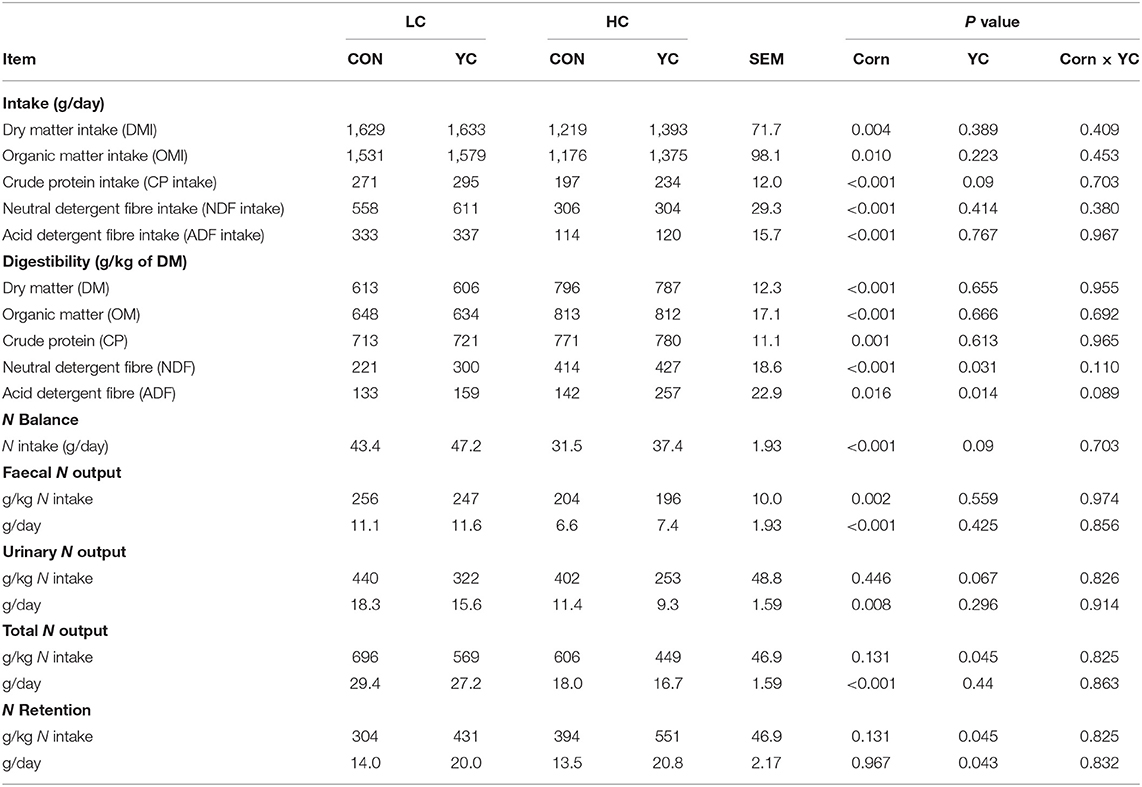
Table 3. Intake, total tract apparent nutrient digestibility, and nitrogen balance of fattening lambs fed experimental diets containing a low (LC) or high (HC) proportion of corn and supplemented with nil (CON) or yeast culture (YC) (n = 6 per treatment).
Rumen Fermentation Parameters
The rumen fermentation parameters (Table 4), including pH value, ammonia, MCP, total SCFA concentration, the molar proportions of acetate, propionate, and iso-butyrate in the total SCFA, and the ratio of acetate to propionate, were affected only by the proportion of corn in the diet (P ≤ 0.043), not by YC supplementation and its interaction with corn proportion. The molar proportions of butyrate, valerate, and iso-valerate in the total SCFA were not significantly affected by the two experimental factors.
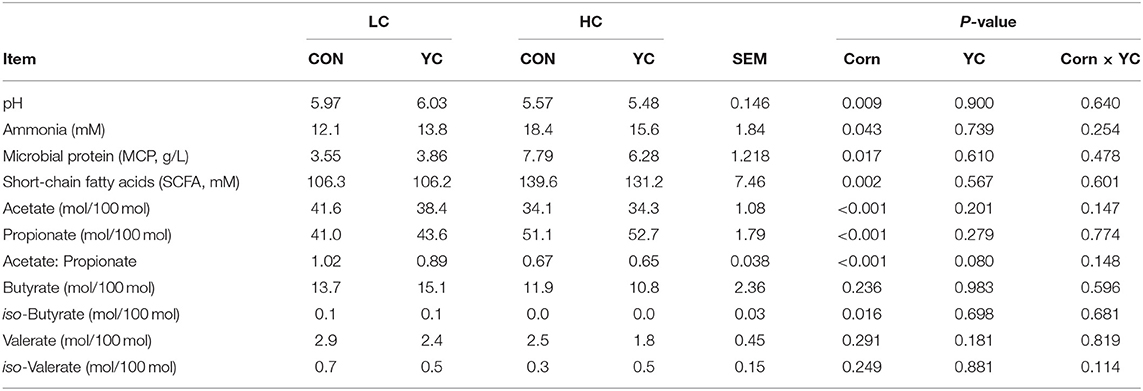
Table 4. Rumen fermentation parameters of fattening lambs fed experimental diets containing a low (LC) or high (HC) proportion of corn and supplemented with nil (CON) or yeast culture (YC), sampled 3 h after morning feeding (n = 6 per treatment).
Serum Parameters
The supplementation of YC did not interact with the proportion of corn in the diet for all serum biochemical parameters assayed (Table 5). The supplementation of YC resulted in a decrease in the contents of total protein (TP; P = 0.002), albumin (ALB; P = 0.016), and globulin (GLOB; P = 0.017) in serum and tended to decrease the content of glucose (P = 0.098). The increase in the proportion of corn in the diet tended to reduce alkaline transaminase (ALT; P = 0.061).
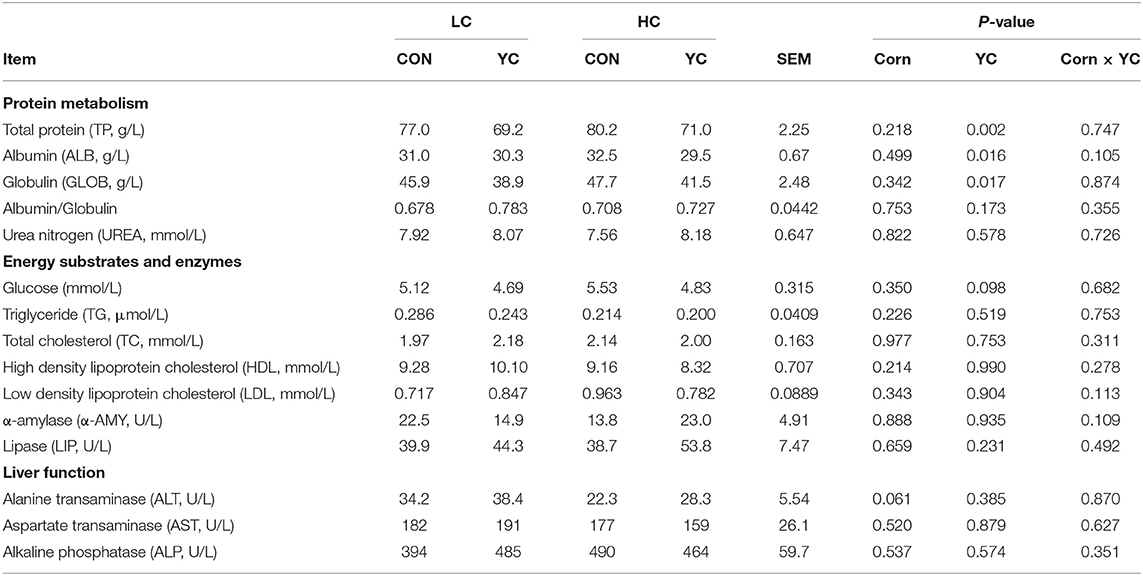
Table 5. Serum biochemical parameters of fattening lambs fed experimental diets containing a low (LC) or high (HC) proportion of corn and supplemented with nil (CON) or yeast culture (YC) (n = 6 per treatment).
Slaughter Performance, Organ Weights and Indexes, and Digestion Tract pH Values
The proportion of corn in the diet, the supplementation of YC, and their interaction did not affect body weight at slaughter, HCW, and dressing out percentage (Table 6). The proportion of corn in the diet and its interaction with YC supplementation had no effect on the dorsal fat thickness and loin eye area, whereas YC supplementation increased dorsal fat thickness (P = 0.039) and tended to reduce eye muscle area (P = 0.062).
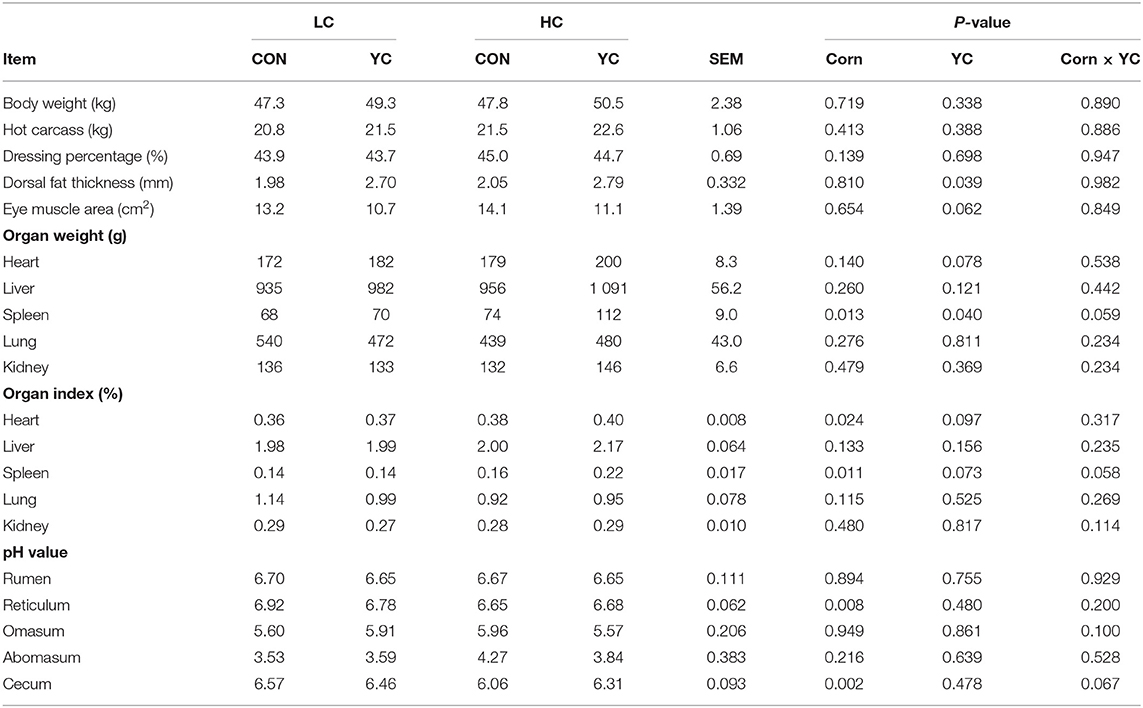
Table 6. Slaughter performance, organ weights and indexes, and digestive tract pH values of fattening lambs fed experimental diets containing a low (LC) or high (HC) proportion of corn and supplemented with nil (CON) or yeast culture (YC) (n = 6 per treatment).
The supplementation of YC had no effect on spleen weight and the proportion of spleen in the whole body at LC; however, at HC, the effects were significant (P < 0.05). The heart tended to be heavier, and the proportion of heart in the whole body tended to be higher after supplementation with YC (P < 0.010). The pH values of the contents of the reticulum and cecum were lower for HC than for LC (P < 0.05), but the supplementation of YC did not affect digestion tract pH values.
Rumen Microbial Communities
The number of OTUs was not affected by either the supplementation of YC or the proportion of corn in the diet (Table 7) and averaged 947. The community richness index Chao was not significantly affected by the proportion of corn in the diet, the supplementation of YC, and their interaction. The Shannon index is one of the indexes for estimating microbial diversity in samples. The index was affected by both experimental factors. The index dropped from 4.25 to 3.98 when the proportion of corn in the diet increased from 350 to 600 g/kg (P < 0.001) and the supplementation of YC also reduced the index from 4.19 to 4.04 (P = 0.036).
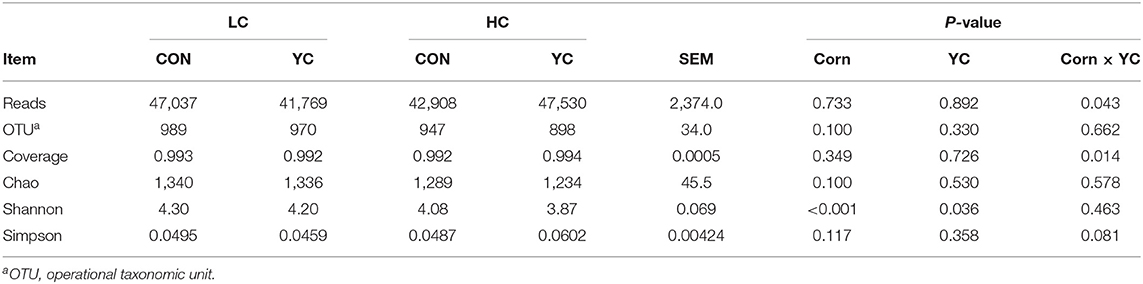
Table 7. The rumen bacterial diversity of fattening lambs fed experimental diets containing a low (LC) or high (HC) proportion of corn and supplemented with nil (CON) or yeast culture (YC) (n = 12 per treatment).
Bacteroidetes, Firmicutes, and Proteobacteria were the three most abundant phyla, accounting for 55, 29, and 14% of the total bacterial sequences analysed, respectively, and summing up to 98% (Table 8). The proportion of corn in the diet and the supplementation of YC did not affect the abundance of these phyla with an exception that HC tended to increase the abundance of Proteobacteria compared with LC (P = 0.090). The fourth and fifth abundant phyla were Fibrobacteres and Spirochaetes, and the remainder of the phyla identified were <1% in any one of the four treatment groups. Increases in the proportion of corn in the diet decreased the abundance of Fibrobacteres (P = 0.017) and Spirochaetes (P = 0.005) from 1.3 to 0.4% and from 0.7 to 0.1%, respectively. The supplementation of YC or increasing corn proportion increased the abundance of Cyanobacteria (P ≤ 0.030). The interaction between corn proportion and YC supplementation affected the abundance of Tenericutes. At LC, YC supplementation reduced the abundance from 0.2 to 0.1% (P < 0.01). At HC, Tenericutes disappeared from the rumen (P = 0.030). The supplementation of YC resulted in the disappearance of Patescibacteria from the rumen (P = 0.022).
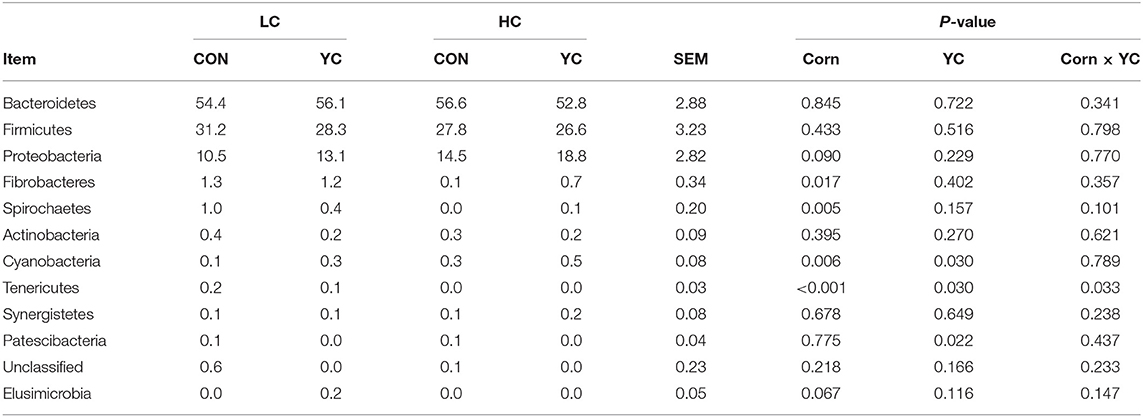
Table 8. Ruminal bacterial phyla (being higher than 0.1% of the total bacteria at least in one of the four treatments) in fattening lambs fed experimental diets containing a low (LC) or high (HC) proportion of corn and supplemented with nil (CON) or yeast culture (YC) (n = 12 per treatment), expressed as a percentage of the total.
The interaction between the proportion of corn in the diet and the supplementation of YC affected the abundance of the Rikenellaceae RC9 gut group (P = 0.023), Prevotellaceae_Unclassified (P = 0.026), Alloprevotella (P = 0.028), Oribacterium (P = 0.037), and Megasphaera (P = 0.065) and tended to affect the abundance of Prevotellaceae UCG-001 (P = 0.092) but had no effect on the abundance of other genera listed in Table 9 and Supplementary Table 1. At LC, the supplementation of YC decreased the abundance of the Rikenellaceae RC9 gut group, Alloprevotella, and Prevotellaceae UCG-001 (P < 0.05) and increased the abundance of Prevotellaceae_Unclassified (P < 0.05). At HC, the supplementation of YC did not result in significant differences for these genera.
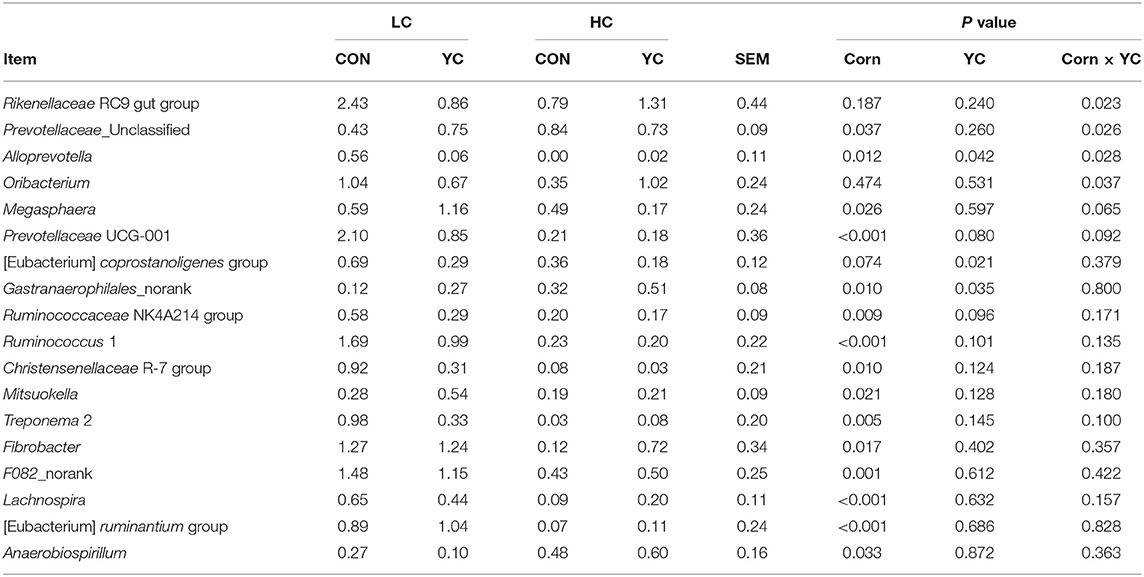
Table 9. The relative abundance of ruminal bacterial genera (being higher than 0.5% of the total bacteria at least in one of the four treatments and statistically different among treatments) in fattening lambs fed experimental diets containing a low (LC) or high (HC) proportion of corn and supplemented with nil (CON) or yeast culture (YC) (n = 12 per treatment), expressed as a percentage of the total.
Increases in the corn proportion resulted in decreases in the abundance of the [Eubacterium] coprostanoligenes group (P = 0.074), Ruminococcaceae NK4A214 group (P = 0.009), Ruminococcus 1 (P < 0.001), Christensenellaceae R-7 group (P = 0.010), Mitsuokella (P = 0.021), Treponema 2 (P = 0.005), Fibrobacter (P = 0.017), F082_norank (P = 0.001), Lachnospira (P < 0.001), and [Eubacterium] ruminantium group (P < 0.001), and increases in Gastranaerophilales_norank (P = 0.010) and Anaerobiospirillum (P = 0.033). Among these genera, the [Eubacterium] coprostanoligenes group (P = 0.021) and Ruminococcaceae NK4A214 group (P = 0.096) decreased, and Gastranaerophilales_norank (P = 0.035) increased with the supplementation of YC, cellulolytic bacteria Fibrobacter, Ruminococcus 1, and Butyrivibrio 2, and other bacteria were not significantly affected. Lactate-producing bacteria Lactobacillus, Streptococcus, and Bifidobacterium were < 0.1% in total bacteria and thus not listed in Supplementary Table 1. Lactate-consuming bacteria Megasphaera and Selenomonas 3 were < 1.2% and 0.5% in any one of the four treatment groups and not affected by YC supplementation.
Discussion
The YC product used in this study contained 2 × 108 cfu/g live yeast cells and was supplemented at 5 g/kg feed, which was expected to have 1 × 106 cfu/g live cells in the feed if all live yeast cells survived. However, the results from the microscopical evaluation showed that the number of live yeast cells was <10 × 103 cfu/g with a survival rate of <1%, which was the same as the CON, indicating that live yeast in the YC product was inactivated during the process of pelleting. Yeast is sensitive to high temperatures (49) and would not survive during pelleting without being protected using special techniques such as agglomeration (50, 51) or micro-encapsulation (52). The loss of yeast viability during pelleting in the present study suggests that the effects of the YC supplementation, if they occur, are from dead yeast, the metabolites of yeast fermentation, and culture media rather than from live yeast. Thus, we can only compare our results with other YC studies in which live yeast does not play a significant role.
In the present study, 31.1 g/day more ADG resulted from the supplementation of YC during the 56-day animal growth performance measurement period. This result is consistent with the finding of more N retained in lambs supplemented with YC and indicated beneficial biological effects of YC on animal growth. The increase of 9.6% in ADG with YC supplementation is consistent with the report by Ghoneem and Mahmoud (53) who provided each lamb 5 g of inactivated yeast per day, which is equivalent to 4.7 g/kg feed and similar to the dose we had in this study but is contrary to the findings of Soliman et al. (54). However, in the study by Soliman et al. (54), each lamb was given 10 g of prebiotics per day containing on a per-kilogramme basis, 100 g of inactive yeast product (50% mannan + 50% β-glucan), 2 g of MnSO4.H2O, 0.12 g of vitamin A, 0.13 g of vitamin E, and 500 g of bentonite, and the feed intake was 1,197 g per day. The real dose of yeast product was only 0.84 g/kg feed, which was largely lower than the dose of 5 g/kg feed in our study. The low dose might be a reason for the lack of improvement in animal growth performance in their study. Our results are in agreement with the studies on other ruminants; for example, Lei et al. (55) fed 2 g/kg yeast cell walls to beef cattle, resulting in an increase of 12.8% ADG, and Peng et al. (56) found an increase of 9.5% ADG when beef cattle were supplemented with yeast cell walls at 20 g/day/head.
Animal growth performance reflects the feeding value of a feed, which is the function of feed intake and feed nutritional value. Feed intake depends on the physical capacity of the digestive system, the physical and chemical properties of the diet, and the digestion and utilisation of nutrients in ruminant animals (57). The supplementation of YC does not change the dietary chemical composition and physical characteristics. Thus, we would not expect an increase in feed intake with YC supplementation. This is confirmed with our result that feed intake was similar between the presence and absence of YC supplementation in the diet observed during the digestibility measurement period, and this result is consistent with the results of Ghoneem and Mahmoud (53) and Soliman et al. (54), suggesting that the improved growth performance with the supplementation of YC may not be related to feed intake.
The fibre digestibility increased with the supplementation of YC, although DM, OM, and CP digestibility did not change in this study. These findings are in agreement with a summary by Bakory (58) who reviewed a range of studies with YC supplemented to ruminants and with a study where beef cattle were fed a diet containing 2 g of yeast cell walls per kg of diet (55). In a study on the supplementation of YC to growing lambs, the digestibility of DM, OM, CP, NDF, and ADF all improved and the extent of increase in fibre digestibility was much higher than our study (59). Our results are also consistent with a dairy cow study where the supplementation of killed yeast tended to increase fibre digestibility, although lactation performance was not improved (60). Although some discrepancies exist among these studies, the common finding is an increase in fibre digestibility with the supplementation of YC. The abundance of fibre-degrading bacteria would be expected to increase for the increased fibre digestibility as found by Abou Elenin et al. (59). It is proposed that metabolites in YC stimulate cellulolytic bacteria (61). However, we did not find an increased abundance of fibre-degrading bacteria in the rumen with the supplementation of YC. This agrees with studies by Dawson et al. (62) and Jiang et al. (60) who found that inactive yeast was unable to stimulate the growth of cellulolytic bacteria. There would be other explanations for increased fibre digestibility with unchanged cellulolytic bacteria. One possibility is that YC may stimulate anaerobic fungi colonising fibre to make the fibre more accessible to fibre-degrading bacteria rather than having a direct effect on the proliferation of these bacteria (58). Live yeast can stimulate the growth of fibre-degrading fungus Neocallimastix frontalis (63). Inactive yeast might have the same effect, but this needs experimental evidence to support.
The contents of TP, ALB, and GLOB in serum decreased with the supplementation of YC in this study. These findings were contradictory to the results obtained from lambs (10, 59, 64) and from dairy cows (7). Even the results among these studies were inconsistent. The heterogeneity in response was claimed to be a result of the delivery method of YC (top-dressing vs. mixing with the TMR) (7). The major difference between our study and these studies was that pelleting was conducted for the YC-supplemented TMR and resulted in the loss of live yeast activity, which might be a reason for the difference. Blood urea nitrogen is also related to protein metabolism and is an indirect indicator of dietary protein composition and/or utilisation. The supplementation of YC did not change blood urine nitrogen concentration in our study and in the literature (7, 64), suggesting nitrogen status was unchanged with the supplementation of YC. Energy-related parameters such as serum glucose, triglyceride, and total cholesterol were not affected by YC supplementation. These results are consistent with studies on lambs (64) and on dairy cows (7). Parameters related to liver function such as ALT, AST, and ALP were also not changed with YC in this study and in the study by Abou Elenin et al. (59), suggesting that YC has no adverse or beneficial effects on liver metabolism.
Although HCW was 3.7–5.1% higher when YC was supplemented to both the LC and HC diets than CON, the differences were not statistically significant. In most YC supplement studies where even yeast is active, slaughter performance does not change (5, 65). An increased ADG, as occurred in this study, does not necessarily translate to improved slaughter performance (66, 67). Another reason is that in most slaughter trials, six to eight lambs are applied to detect treatment differences, which do not have enough power to detect a moderate response. With our own HCW data in this study, a power analysis indicated that seven lambs are needed to detect a 20% difference in HCW, 23 lambs for a 10% difference, and 87 lambs for a 5% difference when power is set at 80% and the significance level is set at 5%.
It is well-known that the ruminal microbial community is associated with animal performance, health, and immunity (68). Both the supplementation of YC and the proportion of corn in the diet altered ruminal microbial community in lambs in our study. However, the most abundant three phyla Bacteroidetes, Firmicutes, and Proteobacteria were predominant in the rumen, being over 98% of the total bacteria, and were not affected by the two experimental treatments, which is consistent with the findings by Li et al. (69) and supports the existence of a core rumen microbiota claimed by Henderson et al. (70). The relative abundance of Prevotella was around half of the total bacteria, reflecting the importance of this microbe in the rumen for its roles in carbohydrate utilisation, nitrogen metabolism, and fibre degradation (69).
Our results showed that the relative abundances of the [Eubacterium] coprostanoligenes group and Ruminococcaceae NK4A214 group decreased with the supplementation of YC. These two genera were found to have higher abundances in the high-yielding dairy cows than in the low-yielding cows (71). The [Eubacterium] coprostanoligenes group was reported to have a negative correlation with milk fat content (72), suggesting that lipid metabolism might be modulated via the bacteria, and this could be a reason for the increased dorsal fat thickness with the supplementation of YC in our study. Ruminococcaceae could have a critical role in biohydrogenation pathways in the rumen (73). Knowledge of the biological functions of these two genera in the rumen reported in the literature so far is too little to explain the altered abundance of the genera in lambs with the supplementation of YC and thus warrants further studies. In contrast to the above genera, Gastranaerophilales_norank increased with the supplementation of YC. The relative abundance of the order Gastranaerophilales in the rumen had a negative correlation with milk yield in dairy cows (74), and lambs with low feed efficiency had a higher relative abundance of Gastranaerophilales than those with high feed efficiency (75). These results are contradictory to our results that found that Gastranaerophilales was associated with higher ADG with the supplementation of YC. This discrepancy is difficult to explain based on the knowledge we have from the literature.
Lambs in the HC diet had lower relative abundances of Ruminococcus 1, Christensenellaceae R-7 group, Mitsuokella, Treponema 2, Fibrobacter, F082_norank, Lachnospira, and [Eubacterium] ruminantium group. Some of these microbes are fibre-degrading bacteria, such as Ruminococcus 1, Fibrobacter (76), and Treponema (77). It is not surprising that fibre-degrading bacteria decline under a high-grain diet. There were several genera, such as the Rikenellaceae RC9 gut group and Prevotellaceae UCG-001, with their relative abundances affected by the interaction between the proportion of corn in the diet and YC supplementation, which is a challenge for the interpretation of these data.
We did not find reportable amounts of typical lactate-producing bacteria Lactobacillus, Streptococcus, and Bifidobacterium, and lactate-consuming bacteria Megasphaera and Selenomonas 3 (14) were in the minimum amount for reporting. No rumen pH values were significantly lower than 5.5. These results suggested that even lambs fed the HC diet did not suffer from acidosis and the role of YC in the stabilisation of rumen pH did not appear.
Conclusions
In conclusion, live yeast cells in the YC product could not survive during TMR pelleting. The supplementation of YC to pelleted TMR at a dose of 5 g/kg improved male lamb growth performance regardless of the proportion of corn in the diet. The improvement might not be attributed to feed intake but could be related to increased fibre digestibility, although the relative abundance of fibre-degrading bacteria in the rumen did not increase. Dorsal fat thickness increased with the supplementation of YC, and eye muscle area tended to decrease. The supplementation of YC altered rumen bacterial community although the core rumen microbiota remained unchanged. Although pelleting inactivates live yeast, bioactive substances in the YC product supplemented to pelleted TMR still improved lamb growth performance and thus YC as a feed additive can be feasibly included in pelleted TMR for sheep.
Data Availability Statement
Sequencing data are available in the NCBI Sequence Read Archive (http://www.ncbi.nlm.nih.gov/bioproject/694322), as project PRJNA694322, submission ID SUB8925435. All the rest of the relevant data are within the paper and its supporting information file.
Ethics Statement
The animal study was reviewed and approved by the Animal Ethics and Welfare Committee of Jilin Agricultural Science and Technology University (Approval number 2018005).
Author Contributions
XS, PY, HW, and KK conceived and planned the study. XS acquired funding, supervised all research, analysed and interpreted data, prepared the tables, prepared the figures, and wrote the manuscript. BS, TW, WY, MW, BL, YH, QH, CL, WT, RL, JL, CW, and XS collected data. XS and JB revised the manuscript. All authors contributed to the article and approved the submitted version.
Funding
This study was supported by the Development of Science and Technology of Jilin Province (Grant number 20180201041NY) and Jilin Agricultural Science and Technology University (Grant number 2018:5001).
Conflict of Interest
PY and WY were employed by Portal Agri-Industries Co., Ltd. HW and KK were employed by Angel Yeast Co., Ltd.
The remaining authors declare that the research was conducted in the absence of any commercial or financial relationships that could be construed as a potential conflict of interest.
The funders of the study were not involved in the study design, the collection, analysis, and interpretation of data, the writing of this article or the decision to submit it for publication.
Acknowledgments
The authors would like to thank the staff at the Animal Experimental Station of Jilin Agricultural Science and Technology University for technical assistance and care of the experimental animals. Yiyong Li is acknowledged for formulating the diets; the staff at the Shanxi Subsidiary Company of Jiangsu Portal Agri-Industries Co., Ltd. for manufacturing the diets used in the experiment; Lixian Yang for the chemical composition analysis of feed, faeces, and urine samples; and Chuang Li for the determination of rumen fermentation parameters.
Supplementary Material
The Supplementary Material for this article can be found online at: https://www.frontiersin.org/articles/10.3389/fvets.2021.657816/full#supplementary-material
References
1. Ma N. Introduction of breeding stock and protection and utilisation of genetic resources of sheep. J Jilin Agric Univ. (2004) 26:77–82 (in Chinese). doi: 10.13327/j.jlau.2004.01.021
2. Salem AFZM. Nutritional Strategies of Animal Feed Additives. Hauppauge, NY: Nova Science Publishers, Inc. (2013).
3. Liu YZ, Lang M, Zhen YG, Chen X, Sun Z, Zhao W, et al. Effects of yeast culture supplementation and the ratio of non-structural carbohydrate to fat on growth performance, carcass traits and the fatty acid profile of the longissimus dorsi muscle in lambs. J Anim Physiol Anim Nutr. (2019) 103:1274–82. doi: 10.1111/jpn.13128
4. Alugongo GM, Xiao J, Wu Z, Li S, Wang Y, Cao Z. Review: Utilization of yeast of Saccharomyces cerevisiae origin in artificially raised calves. J Anim Sci Biotechnol. (2017) 8:34. doi: 10.1186/s40104-017-0165-5
5. Tripathi MK, Karim SA. Effect of yeast cultures supplementation on live weight change, rumen fermentation, ciliate protozoa population, microbial hydrolytic enzymes status and slaughtering performance of growing lamb. Livestock Sci. (2011) 135:17–25. doi: 10.1016/j.livsci.2010.06.007
6. Li S, Yoon I, Scott M, Khafipour E, Plaizier JC. Impact of Saccharomyces cerevisiae fermentation product and subacute ruminal acidosis on production, inflammation, and fermentation in the rumen and hindgut of dairy cows. Anim Feed Sci Technol. (2016) 211:50–60. doi: 10.1016/j.anifeedsci.2015.10.010
7. Dias ALG, Freitas JA, Micai B, Azevedo RA, Greco LF, Santos JEP. Effects of supplementing yeast culture to diets differing in starch content on performance and feeding behavior of dairy cows. J Dairy Sci. (2018) 101:186–200. doi: 10.3168/jds.2017-13240
8. Titi HH, Dmour RO, Abdullah AY. Growth performance and carcass characteristics of Awassi lambs and Shami goat kids fed yeast culture in their finishing diet. Anim Feed Sci Technol. (2008) 142:33–43. doi: 10.1016/j.anifeedsci.2007.06.034
9. Broadway PR, Carroll JA, Sanchez NCB. Live yeast and yeast cell wall supplements enhance immune function and performance in food-producing livestock: a review. Microorganisms. (2015) 3:417–27. doi: 10.3390/microorganisms3030417
10. Mahmoud MM, Youssef IMI, Abd El-Tawab MM, Bakr HA, Eissa NA, Hassan MS, et al. Influence of probiotic and yeast culture supplementation on selected biochemical and immunological parameters of growing lambs. Polish J Vet Sci. (2020) 23:5–12. doi: 10.24425/pjvs.2019.131413
11. Sun X, Song B, He Y, You P. A review on pelleted complete feed for sheep and goats. Mod J Anim Husbandry Vet Med. (2017) 46:162–5 (in Chinese).
12. Silberberg M, Chaucheyras-Durand F, Commun L, Mialon MM, Monteils V, Mosoni P, et al. Repeated acidosis challenges and live yeast supplementation shape rumen microbiota and fermentations and modulate inflammatory status in sheep. Animal. (2013) 7:1910–20. doi: 10.1017/S1751731113001705
13. Plaizier JC, Mesgaran MD, Derakhshani H, Golder H, Khafipour E, Kleen JL, et al. Review: enhancing gastrointestinal health in dairy cows. Animal. (2018) 12:S399–418. doi: 10.1017/S1751731118001921
14. Amin AB, Mao S. Influence of yeast on rumen fermentation, growth performance and quality of products in ruminants: a review. Anim Nutr. (2021) 7:31–41. doi: 10.1016/j.aninu.2020.10.005
15. Schingoethe DJ. A 100-year review: total mixed ration feeding of dairy cows. J Dairy Sci. (2017) 100:10143–50. doi: 10.3168/jds.2017-12967
16. Bonfante E, Palmonari A, Mammi L, Canestrari G, Fustini M, Formigoni A. Effects of a completely pelleted diet on growth performance in Holstein heifers. J Dairy Sci. (2016) 99:9724–31. doi: 10.3168/jds.2016-11033
17. He YH, Zhong RZ, Cheng L, You PH, Li YY, Sun XZ. Effects of the supplementation of lysophospholipids through pelleted total mixed rations on blood biochemical parameters and milk production and composition of mid-lactation dairy cows. Animals. (2020) 10:215. doi: 10.3390/ani10020215
18. Zhong R, Zhao C, Feng P, Wang Y, Zhao X, Luo D, et al. Effects of feeding ground versus pelleted total mixed ration on digestion, rumen function and milk production performance of dairy cows. Int J Dairy Technol. (2020) 73:22–30. doi: 10.1111/1471-0307.12656
19. Islam R, Redoy M, Shuvo A, Sarker M, Akbar M, Al-Mamun M. Effect of pellet from total mixed ration on growth performance, blood metabolomics, carcass and meat characteristics of Bangladeshi garole sheep. Progr Agric. (2017) 28:222–9. doi: 10.3329/pa.v28i3.34659
20. Zhong RZ, Fang Y, Zhou DW, Sun XZ, Zhou CS, He YQ. Pelleted total mixed ration improves growth performance of fattening lambs. Anim Feed Sci Technol. (2018) 242:127–34. doi: 10.1016/j.anifeedsci.2018.06.008
21. Huo Q, Li B, Cheng L, Wu T, You P, Shen S, et al. Dietary supplementation of lysophospholipids affects feed digestion in lambs. Animals. (2019) 9:805. doi: 10.3390/ani9100805
22. Zhang C, Li MM, Al-Marashdeh O, Gan LP, Zhang CY, Zhang GG. Performance, rumen fermentation, and gastrointestinal microflora of lambs fed pelleted or unpelleted total mixed ration. Anim Feed Sci Technol. (2019) 253:22–31. doi: 10.1016/j.anifeedsci.2019.05.003
23. Li B, Sun XZ, Huo Q, Zhang GG, Wu TT, You PH, et al. Pelleting of a total mixed ration affects growth performance of fattening lambs. Front Vet Sci. (2021) 8:629016. doi: 10.3389/fvets.2021.629016
24. Svihus B, Zimonja O. Chemical alterations with nutritional consequences due to pelleting animal feeds: a review. Anim Prod Sci. (2011) 51:590–6. doi: 10.1071/AN11004
25. Burgents JE, Burnett KG, Burnett LE. Disease resistance of Pacific white shrimp, Litopenaeus vannamei, following the dietary administration of a yeast culture food supplement. Aquaculture. (2004) 231:1–8. doi: 10.1016/j.aquaculture.2003.09.003
26. Ayiku S, Shen JF, Tan BP, Dong XH, Liu HY. Effects of dietary yeast culture on shrimp growth, immune response, intestinal health and disease resistance against Vibrio harveyi. Fish Shellfish Immunol. (2020) 102:286–95. doi: 10.1016/j.fsi.2020.04.036
27. Alton GG, Jones LM, Pietz DE. Laboratory Techniques in Brucellosis. Monograph Series. Geneva: World Health Organization (1975). p. 1–163.
28. Ministry of Agriculture of China. Feeding Standard of Meat-producing Sheep and Goats (Standard NY/T 816-2004). Beijing: Chinese Agricultural Press (2005).
29. Sun XZ, Krijgsman L, Waghorn GC, Kjestrup H, Koolaard J, Pacheco D. Sheep numbers required for dry matter digestibility evaluations when fed fresh perennial ryegrass or forage rape. Anim Nutr. (2017) 3:61–6. doi: 10.1016/j.aninu.2016.12.001
30. Kirton AH, Woods EG, Duganzich DM. Predicting the fatness of lamb carcasses from carcass wall thickness measured by ruler or by a total depth indicator (TDI) probe. Livestock Prod Sci. (1984) 11:185–94. doi: 10.1016/0301-6226(84)90059-9
31. Luo Y, Zhang X, Zhu Z, Jiao N, Qiu K, Yin J. Surplus dietary isoleucine intake enhanced monounsaturated fatty acid synthesis and fat accumulation in skeletal muscle of finishing pigs. J Anim Sci Biotechnol. (2018) 9:88. doi: 10.1186/s40104-018-0306-5
32. Sami M, Ikeda M, Yabuuchi S. Evaluation of the alkaline methylene blue staining method for yeast activity determination. J Ferment Bioeng. (1994) 78:212–6. doi: 10.1016/0922-338X(94)90292-5
33. ISO 5498. Agricultural Food Products – Determination of Crude fibre Content – General Method. Geneva: International Organization for Stardardization (1999).
34. ISO 5984. Animal Feeding Stuffs – Determination of Crude Ash. Geneva: International Organization for Stardardization (2002).
36. van Soest PJ, Robertson JB, Lewis BA. Methods for dietary fiber, neutral detergent fiber, and nonstarch polysaccharides in relation to animal nutrition. J Dairy Sci. (1991) 74:3583–97. doi: 10.3168/jds.S0022-0302(91)78551-2
37. ISO 6492. Animal Feeding Stuffs – Determination of Fat Content. Geneva: International Organization for Stardardization (1999).
38. ISO 6490–1. Animal Feeding Stuffs – Determination of Calcium Content – Part 1, Titrimetric Method. Geneva: International Organization for Stardardization (1985).
39. ISO 6491. Animal Feeding Stuffs – Determination of Phosphorus Content – Spectrometric Method. Geneva: International Organization for Stardardization (1998).
40. Feng Z, Gao M. A modified spectrophotometric method for the determination of ammonia concentration in ruminal liquor. Anim Husbandry Feed Sci. (2010) 31:37 (in Chinese). doi: 10.16003/j.cnki.issn1672-5190.2010.z1.027
41. Wang HR, Chen Q, Chen LM, Ge RF, Wang MZ, Yu LH, et al. Effects of dietary physically effective neutral detergent fiber content on the feeding behavior, digestibility, and growth of 8- to 10-month-old Holstein replacement heifers. J Dairy Sci. (2017) 100:1161–9. doi: 10.3168/jds.2016-10924
42. Zinn RA, Owens FN. A rapid procedure for purine measurement and its use for estimating net ruminal protein synthesis. Can J Anim Sci. (1986) 66:157–66. doi: 10.4141/cjas86-017
43. Makkar HPS, Becker K. Purine quantification in digesta from ruminants by spectrophotometric and HPLC methods. Br J Nutr. (1999) 81:107–12. doi: 10.1017/S0007114599000227
44. Mago č T, Salzberg SL. FLASH: fast length adjustment of short reads to improve genome assemblies. Bioinformatics. (2011) 27:2957–63. doi: 10.1093/bioinformatics/btr507
45. Edgar RC. UPARSE: highly accurate OTU sequences from microbial amplicon reads. Nat Methods. (2013) 10:996–8. doi: 10.1038/nmeth.2604
46. Henderson G, Yilmaz P, Kumar S, Forster RJ, Kelly WJ, Leahy SC, et al. Improved taxonomic assignment of rumen bacterial 16S rRNA sequences using a revised SILVA taxonomic framework. PeerJ. (2019) 7:e6496. doi: 10.7717/peerj.6496
47. Schloss PD, Westcott SL, Ryabin T, Hall JR, Hartmann M, Hollister EB, et al. Introducing mothur: open-source, platform-independent, community-supported software for describing and comparing microbial communities. Appl Environ Microbiol. (2009) 75:7537–41. doi: 10.1128/AEM.01541-09
49. Shurson GC. Yeast and yeast derivatives in feed additives and ingredients: sources, characteristics, animal responses, quantification methods. Anim Feed Sci Technol. (2018) 235:60–76. doi: 10.1016/j.anifeedsci.2017.11.010
50. Aguirre-Guzmán G, Ricque-Marie D, Cruz-Suárez LE. Survival of agglomerated Saccharomyces cerevisiae in pelleted shrimp feeds. Aquaculture. (2002) 208:125–35. doi: 10.1016/S0044-8486(01)00711-6
51. Kimsé M, Bayourthe C, Monteils V, Fortun-Lamothe L, Cauquil L, Combes S, et al. Live yeast stability in rabbit digestive tract: consequences on the caecal ecosystem, digestion, growth and digestive health. Anim Feed Sci Technol. (2012) 173:235–43. doi: 10.1016/j.anifeedsci.2012.01.012
52. Rotolo L, Gai F, Peiretti PG, Ortoffi M, Zoccarato I, Gasco L. Live yeast (Saccharomyces cerevisiae var. boulardii) supplementation in fattening rabbit diet: effect on productive performance and meat quality. Livestock Sci. (2014) 162:178–84. doi: 10.1016/j.livsci.2014.01.022
53. Ghoneem WMA, Mahmoud AEM. Effect of in-activated and dried yeast on productive performance of Barki lambs. Asian J Anim Vet Adv. (2014) 9:664–73. doi: 10.3923/ajava.2014.664.673
54. Soliman SM, El-Shinnawy AM, El-Morsy AM. Effect of probiotic or prebiotic supplementation on the productive performance of Barki lambs. J Anim Poultry Prod. (2016) 7:369–46. doi: 10.21608/jappmu.2016.48743
55. Lei CL, Dong GZ, Jin L, Zhang S, Zhou J. Effects of dietary supplementation of montmorillonite and yeast cell wall on lipopolysaccharide adsorption, nutrient digestibility and growth performance in beef cattle. Livestock Sci. (2013) 158:57–63. doi: 10.1016/j.livsci.2013.08.019
56. Peng QH, Cheng L, Kun KA, Gang T, Mohammad AM, Bai XU, et al. Effects of yeast and yeast cell wall polysaccharides supplementation on beef cattle growth performance, rumen microbial populations and lipopolysaccharides production. J Integr Agric. (2020) 19:810–9. doi: 10.1016/S2095-3119(19)62708-5
58. Bakory MTA. Yeast culture in animal nutrition: a review. Biosci Res. (2014) 11:10−9. Available online at: http://www.isisn.org/BR%2011(1-2)%202014/10-19%2011(1)%202014%20BR-1111.pdf
59. Abou Elenin EIM, Abd El-Galil ER, Etman KEI, El-Shabrawy HM. Improvement of rumen fermentation and performance of growing lambs by adding natural microbial resources. Asian J Anim Sci. (2016) 10:202–12. doi: 10.3923/ajas.2016.202.212
60. Jiang Y, Ogunade IM, Arriola KG, Qi M, Vyas D, Staples CR, et al. Effects of the dose and viability of Saccharomyces cerevisiae. 2. Ruminal fermentation, performance of lactating dairy cows, and correlations between ruminal bacteria abundance and performance measures. J Dairy Sci. (2017) 100:8102–18. doi: 10.3168/jds.2016-12371
61. Callaway ES, Martin SA. Effects of a Saccharomyces cerevisiae culture on ruminal bacteria that utilize lactate and digest cellulose. J Dairy Sci. (1997) 80:2035–44. doi: 10.3168/jds.S0022-0302(97)76148-4
62. Dawson KA, Newman KE, Boling JA. Effects of microbial supplements containing yeast and lactobacilli on roughage-fed ruminal microbial activities. J Anim Sci. (1990) 68:3392–8. doi: 10.2527/1990.68103392x
63. Chaucheyras F, Fonty G, Bertin G, Gouet P. Effects of live Saccharomyces cerevisiae cells on zoospore germination, growth, and cellulolytic activity of the rumen anaerobic fungus, Neocallimastix frontalis MCH3. Curr Microbiol. (1995) 31:201–5. doi: 10.1007/BF00298373
64. Malekkhahi M, Tahmasbi AM, Naserian AA, Danesh Mesgaran M, Kleen JL, Parand AA. Effects of essential oils, yeast culture and malate on rumen fermentation, blood metabolites, growth performance and nutrient digestibility of Baluchi lambs fed high-concentrate diets. J Anim Physiol Anim Nutr. (2015) 99:221–9. doi: 10.1111/jpn.12230
65. Ogbuewu IP, Okoro VM, Mbajiorgu EF, Mbajiorgu CA. Yeast (Saccharomyces cerevisiae) and its effect on production indices of livestock and poultry—A review. Comp Clin Pathol. (2019) 28:669–77. doi: 10.1007/s00580-018-2862-7
66. Silva Moura CM, Araújo GGL, Oliveira BYS, Azevêdo JAG, Pimenta Filho EC, Azevedo PS, et al. Different roughage:concentrate ratios and water supplies to feedlot lambs: carcass characteristics and meat chemical composition. J Agric Sci. (2019) 157:643–9. doi: 10.1017/S0021859620000076
67. Bernard M, Cheng L, Chantelauze C, Song Y, Jeanleboeuf A, Sagot L, et al. Nitrogen partitioning and isotopic discrimination are affected by age and dietary protein content in growing lambs. Animal. (2020) 14:942−51. doi: 10.1017/S1751731119002647
68. Huws SA, Creevey CJ, Oyama LB, Mizrahi I, Denman SE, Popova M, et al. Addressing global ruminant agricultural challenges through understanding the rumen microbiome: past, present, and future. Front Microbiol. (2018) 9:02161. doi: 10.3389/fmicb.2018.02161
69. Li Z, Mu C, Xu Y, Shen J, Zhu W. Changes in the solid-, liquid-, and epithelium-associated bacterial communities in the rumen of Hu lambs in response to dietary urea supplementation. Front Microbiol. (2020) 11:00244. doi: 10.3389/fmicb.2020.00244
70. Henderson G, Cox F, Ganesh S, Jonker A, Young W, Janssen PH, et al. Rumen microbial community composition varies with diet and host, but a core microbiome is found across a wide geographical range. Sci Rep. (2015) 5:14567. doi: 10.1038/srep14567
71. Tong J, Zhang H, Yang D, Zhang Y, Xiong B, Jiang L. Illumina sequencing analysis of the ruminal microbiota in high-yield and low-yield lactating dairy cows. PLoS ONE. (2018) 13:e0198225. doi: 10.1371/journal.pone.0198225
72. Sun Z, Yu Z, Wang B. Perilla frutescens leaf alters the rumen microbial community of lactating dairy cows. Microorganisms. (2019) 7:562. doi: 10.3390/microorganisms7110562
73. Huws SA, Kim EJ, Lee MR, Scott MB, Tweed JK, Pinloche E, et al. As yet uncultured bacteria phylogenetically classified as Prevotella, Lachnospiraceae incertae sedis and unclassified Bacteroidales, Clostridiales and Ruminococcaceae may play a predominant role in ruminal biohydrogenation. Environ Microbiol. (2011) 13:1500–12. doi: 10.1111/j.1462-2920.2011.02452.x
74. Bach A, López-García A, González-Recio O, Elcoso G, Fàbregas F, Chaucheyras-Durand F, et al. Changes in the rumen and colon microbiota and effects of live yeast dietary supplementation during the transition from the dry period to lactation of dairy cows. J Dairy Sci. (2019) 102:6180–98. doi: 10.3168/jds.2018-16105
75. Perea K, Perz K, Olivo SK, Williams A, Lachman M, Ishaq SL, et al. Feed efficiency phenotypes in lambs involve changes in ruminal, colonic, small-intestine-located microbiota. J Anim Sci. (2017) 95:2585–92. doi: 10.2527/jas2016.1222
76. Denman SE, Morgavi DP, McSweeney CS. Review: the application of omics to rumen microbiota function. Animal. (2018) 12:S233–45. doi: 10.1017/S175173111800229X
Keywords: animal performance, carcass, digestibility, fattening lamb, high grain diet, pelleted total mixed ration, rumen fermentation parameters, yeast culture
Citation: Song B, Wu T, You P, Wang H, Burke JL, Kang K, Yu W, Wang M, Li B, He Y, Huo Q, Li C, Tian W, Li R, Li J, Wang C and Sun X (2021) Dietary Supplementation of Yeast Culture Into Pelleted Total Mixed Rations Improves the Growth Performance of Fattening Lambs. Front. Vet. Sci. 8:657816. doi: 10.3389/fvets.2021.657816
Received: 24 January 2021; Accepted: 29 March 2021;
Published: 12 May 2021.
Edited by:
Domenico Bergero, University of Turin, ItalyReviewed by:
Anjas Asmara Samsudin, Putra Malaysia University, MalaysiaCarlos Aguilar-Pérez, Universidad Autónoma de Yucatán, Mexico
Manuela Renna, University of Turin, Italy
Copyright © 2021 Song, Wu, You, Wang, Burke, Kang, Yu, Wang, Li, He, Huo, Li, Tian, Li, Li, Wang and Sun. This is an open-access article distributed under the terms of the Creative Commons Attribution License (CC BY). The use, distribution or reproduction in other forums is permitted, provided the original author(s) and the copyright owner(s) are credited and that the original publication in this journal is cited, in accordance with accepted academic practice. No use, distribution or reproduction is permitted which does not comply with these terms.
*Correspondence: Xuezhao Sun, eHVlemhhb3NAaG90bWFpbC5jb20=
†These authors have contributed equally to this work