- 1Department of Veterinary Biomedical Sciences, Western College of Veterinary Medicine, University of Saskatchewan, Saskatoon, SK, Canada
- 2Department of Animal and Poultry Science, College of Agriculture and Bioresources, University of Saskatchewan, Saskatoon, SK, Canada
- 3Prairie Swine Centre, Inc., Saskatoon, SK, Canada
Fava bean, which is available in high- and low-tannin varieties, is not an approved pet food ingredient and was not included in the “assumed to be safe” category based on its ability to cause favism and hemolytic anemia in susceptible humans. The effects of 7-day feeding of test canine diets containing moderate protein (~27%) were compared with two control commercial diets with normal (NP, grain-containing, ~25% protein) or high protein (HP, grain-free, ~41% protein). Fava bean diets were formulated either with or without Candida utilis fermentation processing to reduce antinutritional factors. Glucose tolerance, body weight, cardiovascular function, and blood parameters were investigated in beagles fed the NP or HP diets or a randomized, crossover, 2 × 2 Latin square design of the fava bean diets: unfermented high-tannin (UF-HT), fermented high-tannin (FM-HT), unfermented low-tannin (UF-LT), and fermented low-tannin (FM-LT). After 7 days, HP decreased red blood cells (RBC) (P < 0.05) compared with NP, while FM increased RBC compared with UF. HP increased blood bicarbonate, calcium, phosphorus, urea, cholesterol, and albumin:globulin ratio while decreasing bilirubin, liver enzymes, and total protein. Sodium:potassium ratio was increased in UF-HT, decreased in FM-HT, and intermediate in LT regardless of fermentation. Blood phosphorus was increased in HT. Blood amylase was increased in FM-HT and decreased in FM-LT, being intermediate in UF regardless of fava bean variety. Blood direct bilirubin was decreased in HT regardless of fermentation. Of note, left ventricular end-systolic volume and cardiac output were increased in NP compared with HP-fed dogs, but were normal and had no significant differences among the fava bean diets. As expected, plasma taurine, cystine, and cysteine levels were increased in HP- compared with NP-fed dogs. Plasma cysteine levels were increased in HT- compared with LT-fed dogs and in FM- compared with UF-fed dogs. Taken together, these results show that fava bean appears to be safe as a dog food ingredient at least in the short term, and its nutritional value appears improved by fermentation. Moreover, blood chemistry parameters and cardiovascular function were impacted by protein content which merits further investigation with longer term feeding trials.
Introduction
Fava bean (Vicia faba L.) has been regarded as a healthy, sustainable alternative for partially replacing animal protein sources in human diets (1). The varieties of fava bean are divided by their tannin levels such as low or normal/high tannin content which affects taste (2). Because pet owners are increasingly matching their own nutritional choices with that of their pets, incorporation of fava beans in pet diets as a source of carbohydrates and protein has been considered. Fava bean safety in dogs has been scarcely explored and is not approved as a feed ingredient for pet food by the American Association of Feed Control Organization (AAFCO) yet due to concerns about potential toxicity from antinutritional factors (3). Further complicating the approval of a novel pulse ingredient such as fava beans for use in pet food, the US Food and Drug Administration (FDA) reported in July 2018, cases in which dilated cardiomyopathy (DCM) was observed in dogs fed grain-free diets, i.e., food formulated with potatoes and pulse ingredients instead of grains (4). DCM is recognized as the second most common type of genetically linked cardiac disease in the dog and is most prevalent in large or giant breeds (5). Dobermans, Boxers, Great Danes, Newfoundlands, Irish Wolfhounds, English Cocker Spaniels, and Portuguese Water Dogs are the breeds with the highest prevalence of DCM (6–9). Moreover, Golden Retrievers and American Cocker Spaniels have recently emerged as being predisposed to taurine deficiency (10, 11). DCM is described as a primary myocardial disorder causing systolic dysfunction with secondary ventricular dilation, regular or decreased wall thickness, and increased cardiac mass due to myocyte enlargement (12). However, while pulse ingredients in grain-free diets have been suggested as causally linked to DCM, the actual link between them has not been definitively demonstrated to date (13–15). Despite an acknowledgment by the FDA in November 2020 that the link between DCM and diet in dogs is multi-factorial and not due solely to pulses, this issue remains unresolved, leaving veterinarians, and pet owners wary of pulse-containing dog foods.
The best evidence so far for a link between DCM and nutrition in dogs is through taurine insufficiency (16, 17). Taurine is important to cardiac health because it participates in the reabsorption of calcium by the sarcoplasmic reticulum and enhances the sensitivity of the myofilaments to calcium (18). Therefore, as reviewed by Mansilla et al. (13), because calcium is a key component of cardiac contraction, in cases of taurine absence due to reduced synthesis or low intake of taurine and/or its precursors, the cardiac muscle tissue is unable to properly contract and presumably develops DCM. Dog diets do not require taurine and taurine is not considered essential since dogs can synthesize taurine from sulfur-containing amino acids such as cysteine and methionine (19). Grain-free and pulse-containing diets are higher in fermentable fiber which has been demonstrated to decrease protein digestibility though either an increased fermentation of the sulfur-containing amino acids leading to decreased bioavailability or to higher fecal excretion (20, 21). Previous studies have reported that high fermentable dietary fiber in dogs increases the need for dietary taurine or taurine precursors (e.g., methionine and cysteine) due to increased fecal excretion of taurocholate (the predominant bile acid in dogs), leading to fecal loss of taurine (22, 23).
Fava beans have numerous antinutritional factors such as condensed tannins, trypsin inhibitor activity, lectins, and pyrimidine glucosides (24). Tannins are known to cause reduction of protein and energy digestibility (3). The pyrimidine glucosides (vicine and convicine) lead to favism which is a blood disorder caused when fava beans are eaten by humans with genetic mutations of glucose-6-phosphate dehydrogenase (G6PD), leading to decreased G6PD activity and reduced ability of red blood cells (RBCs) to produce ATP and regenerate glutathione (25). This then makes the red blood cell susceptible to oxidative damage, leading to rapid RBC death and acute anemia when uncooked fava beans are consumed. There are no reports of favism in dogs to the best of our knowledge. However, because of this concern, fava beans cannot be placed in the “generally assumed to be safe” category by AAFCO and thus is not currently used in pet food until proven otherwise.
Fermentation is a processing technique well-known for improving health, functional, and nutraceutical effects of foods (26). Fermentation also has been positively associated with enhanced nutritional quality of pulses by reducing their levels of anti-nutritional factors (27–29). For example, fermentation of fava bean with Lactobacillus plantarum reduced the content of the antinutritional factors vicine and convicine by more than 90% while increasing the amount of free amino acids and enhancing protein digestibility (30). Likewise, Rizzello et al. (31) demonstrated a complete degradation of the pyrimidine glycosides in L. plantarum–fermented fava bean flour after 48 h, which indicates the usefulness of bioprocessing techniques for industrial fava bean detoxification. In comparison, some yeast organisms in the Candida clade have the potential to synthesize and increase taurine content while other yeasts and bacteria do not (32). This current study explored the use of fermentation with the yeast Candida utilis to both reduce antinutritional factors and increase taurine content.
The objective of this study was to determine if short-term (7-day) feeding of beagles with a moderate protein diet that has 30% inclusion of fava bean flour would show altered glucose tolerance, body weight, cardiovascular function, and blood parameters when contrasted to commercial diets with normal vs. high protein. Two commercial diets with normal or high protein content and four different fava bean-containing diets with low- (LT) or high-tannin (HT) content were fed, both varieties with and without fermentation. We hypothesized that pulse-based diets would impair cardiovascular health due to the low taurine, cysteine, or methionine levels and high fiber content. Moreover, fermentation of fava bean flour with C. utilis would enhance diet quality and, consequently, health of dogs.
Materials and Methods
Fava Bean Ingredients and Fermentation Protocol
Low-tannin (Snowdrop) and HT (Florent) fava bean varieties, genotypes grown in Saskatchewan (2), were dehulled and ground into flour using a 400-μm screen. Fermentation of each variety was adapted from methodology used previously in pea flour in our laboratory (33). Briefly, C. utilis (ATCC 9950) was maintained in sterile 80% (v/v) glycerol solution at −80°C, then reactivated on YGC agar plates when needed (Yeast Extract Glucose Chloramphenicol Agar; catalogue number 95765; Sigma Aldrich, St Louis, MO). Seeded plates were incubated at 30°C for 72 h. Two loops of colonies were transferred using a flame-sterilized platinum needle to a 250-ml sterile conical flask containing 100 ml of YPD liquid medium (Yeast Peptone Dextrose—A1374501; ThermoFisher, Waltham, MA). The flask was incubated in a horizontal shaker incubator (30°C) at 120 rpm for 12 to 15 h. After this period, 10 ml of the cultured yeast mass was transferred into a 500-ml sterile conical flask containing 250 ml of YPD liquid medium. The medium containing the yeast was then incubated on a horizontal shaker (30°C) at 120 rpm for an additional 12 to 15 h. Twenty-kilogram batches of each fava bean variety were mixed with the yeast broth, ammonia, and sterile water to form a soft dough, then fermented in an adapted cement mixer with temperature maintained at 30°C. The fermentation slurry was mixed for 3 min every hour for 72 h. Samples were collected every 24 h, and serial dilutions of mixture plated on sterile agar plates, then incubated at 30°C for 72 h to verify yeast viability and count throughout the process. The fermented fava bean flour was subsequently dried in an oven (60°C) for 48 h at the WCVM before transport to the University of Saskatchewan Canadian Feed Research Centre (North Battleford, Canada) for grinding of the fermented flours, followed by mixing of all test diets (both fermented and unfermented fava bean flours), extruding, and vacuum coating with fat to produce the final dry kibble format of the diets to be used in the feeding trials.
Animals and Diets
All animal use and procedures were approved by the University of Saskatchewan Animal Care Committee (Animal Utilization Protocol #20190055) and adhered to the Canadian Council on Animal Care. Eight neutered beagles, four males and four females, at ideal body weight (8.8 ± 1.9 kg) and a mean age of 2.6 ± 1.0 years, were obtained from King Fisher International (Toronto, ON, Canada) or Marshall Bioresources (North York, NY, USA) and housed at the Western College of Veterinary Medicine (Saskatoon, SK, Canada). The animals were housed individually in 1.1 × 2.7-m kennels with outdoor kennel access at night and during feeding but kept in a group kennel area during the day. Dogs were walked or socialized with volunteers for at least 1 h every day. When not on trial, dogs were fed a commercial adult maintenance dry diet (Purina Dog Chow; Ralston Purina Co, St Louis, MI) in amounts that maintained each individual dog at ideal condition (score of 4–6 on a 9-point scale) in conjunction with energy requirements stated in the National Research Council guidelines (34). All dogs were acclimated to procedures with rewards before the start of experiments to minimize stress, thus no anesthetics or sedatives were used in this study.
In total, six diets were tested. Low- or high-tannin fava bean flours were used at 30% inclusion, in either fermented or unfermented formats with diet formulations indicated in Supplementary Table 1. The four fava bean test diets were unfermented low-tannin [UF-LT; 27% crude protein (CP)], fermented low-tannin (FM-LT; 28% CP), unfermented high-tannin (UF-HT; 27% CP), and fermented high-tannin (FM-HT; 28% CP). Insoluble ash (Celite) was included at 1% as a non-digestible marker. Diets were formulated in accordance with the nutrient guidelines for adult dog maintenance set by AAFCO to be nutritionally balanced before being extruded under identical process conditions (35). The two additional diets tested were commercial diets containing normal-protein (NP; Purina Dog Chow, St. Louis, MI; 24% CP) or high-protein content (HP; GO! Solutions Carnivore, Richmond, BC; 41% CP). Ingredient lists for the commercial diets are shown in Supplementary Table 2. All six diets were randomly sub-sampled and sent for proximate analysis (Central Testing, Winnipeg, MB, Canada) (Supplementary Table 3). The analyzed content of crude fiber, non-fiber carbohydrates, metabolizable energy, vicine, and convicine of UF-LT, UF-HT, FM-LT, and FM-HT diets are 1.1, 0.5, 0.5, and 0.5%; 60.7, 62.1, 61.6, and 61.8%; 3.7, 3.8, 3.8, and 3.8 kcal/g; 1.8, 1.8, 0.4, and 0.6 mg/g; and 0.5, 0.6, 0.1, and 0.2 mg/g, respectively. The analyzed content of crude fiber, non-fiber carbohydrates, and metabolizable energy of NP and HP diets are 0.8 and 1.1%, 53.0 and 29.8%, and 4.0 and 4.1 kcal/g, respectively. Dogs were fed each diet for 7 days, with the NP or HP diets fed during the first and sixth weeks, respectively. From weeks 2 to 5, using a randomized, crossover, 2 × 2 Latin square design, the UF-LT, FM-LT, UF-HT, and FM-HT diets were fed. Commercial diets were not included in the crossover design because the fava bean–based diets were formulated to contain the same nutrient profile to enable a reasonable comparison. In contrast, NP and HP diets have different ingredients at unknown inclusion levels (specific formulation not available on label), making direct comparisons difficult. Dogs were weighed at the beginning of the experiment and after each feeding week. The amount of diet allotted per dog per day was calculated to be isocaloric with the daily requirement for that dog using standard equations that determine the energy requirements for individual dog maintenance [maintenance energy (ME in kcal)] = [(70 × BW0.7) × 1.6], daily portions divided, and dogs fed twice daily (at 08:30 and 16:30 h). Bowls were removed before the next meal and any uneaten food was weighed and recorded. All dogs generally consumed all food portioned in each meal within 5–10 min, with no palatability issues noted (Morris, Reis & Weber, unpublished).
Digestibility Protocol
After a 5-day feeding period on each fava bean–based diet, feces were collected during the subsequent 2 days of each period (total of 7 days on each diet). Collected feces were labeled and frozen at −20°C until analysis. Feces were thawed, homogenized, and pooled by dog (i.e., two samples from each dog pooled per dietary treatment). Before laboratory testing, feces were dried in a forced air oven at 55°C for 72 h and ground in a cutting mill with a 1-mm sieve. Diets and feces were analyzed (Central Testing, Winnipeg, MB) according to AOAC standards (36) for dry matter by oven-drying the sample, non-fiber carbohydrates, crude protein applying the Kjeldahl method, and acid-hydrolyzed fat. Gross energy (GE) content of diets was determined using a bomb calorimeter. The equation below was used to calculate the apparent digestibility coefficients of dry matter, crude protein, non-fiber carbohydrates, fat, gross energy, methionine, cysteine, and taurine for each fava bean–based diet using Celite as a non-digestible marker (37):
Where M feed and M feces represent concentrations of index compound in feed and feces, respectively; C feed and C feces represent concentrations of components of interest in feed and feces, respectively.
Oral Glucose Tolerance Test and Blood Analysis
After 7 days of feeding each diet, dogs were fasted for 8 h then a syringe with 10 ml/kg body weight of a 10% glucose solution (1 g/kg BW glucose) fed to each dog by placing in the back of the mouth for an oral glucose tolerance test conducted at the same time each day. Before glucose feeding, the fasted dogs were aseptically catheterized using a peripheral intravenous catheter equipped with an extension tube inserted into the cephalic vein. Blood samples (~0.2 ml) were taken before feeding glucose (time 0) and at 15, 30, 60, and 90 min after feeding. Blood glucose was measured using a glucometer (OneTouch Ultra 2; LifeScan, Johnson & Johnson, New Brunswick, NJ) with a minimum of duplicate readings for each time or until two consistent readings were obtained. The extension tube was filled with blood and initial blood discarded before glucometer reading to ensure no contamination of blood with anticoagulant solution. Subsequently, after each blood sample was obtained, the catheter was flushed with a sterile citrate solution to prevent clotting. The trapezoidal method was used to determine the incremental area under the curve (AUC) for the glucose response (38). Peak concentration and time to peak concentration for glucose were also calculated.
Additional fasting blood samples (8 ml) were taken using collection tubes with and without EDTA. Complete blood cell count [red (RBC) and white (WBC) blood cell counts] and chemistry panel [cholesterol; total (TB), direct (DB), and indirect bilirubin (IB); alkaline phosphatase (ALP); alanine aminotransferase (ALT); creatine kinase (CK); gamma-glutamyltransferase (GGP); glutamate dehydrogenase (GLDH); total protein, albumin (A), globulin (G), and A:G] were analyzed at Prairie Diagnostic Services (Saskatoon, SK). Moreover, 3-ml subsamples of EDTA-tube blood from fasted animals were centrifuged at 2,000 rpm for 10 min for plasma collection. Plasma samples were kept at −80°C until further analyses of methionine, cystine, cysteine, and taurine content (UC Davis Amino Acid Lab, Davis, CA). Plasma amino acid concentrations were analyzed in an automated amino acid analyzer via cation-exchange high-pressure liquid chromatography separation and ninhydrin-reactive colorimetric detection (39–42).
Cardiac Function, Blood Pressure, and Vascular Health
After 7 days of feeding each diet, dogs were tested for cardiovascular health. All ultrasound measurements were performed and analyzed by one individual. Before ultrasound, blood pressure was taken using a high-definition canine/feline oscillometer (VET HDO High Definition Oscillometer, Babenhausen, Germany). An average of two readings with good agreement was used to determine diastolic and systolic pressures. Endpoints of flow-mediated dilation were used as an indicator of vascular health and included brachial artery diameter during baseline, during inflation of a blood pressure cuff placed distal to the brachial artery, and at the time of peak dilation (30 s) after cuff release, as previously determined by our research group in dogs (43, 44). Echocardiography endpoints were used to assess cardiac function included heart rate (HR), stroke volume (SV), and cardiac output (CO) (44, 45). Moreover, left ventricular end-diastolic volume (EDV), left ventricular end-systolic volume (ESV), ejection fraction (EF), left ventricular diastolic free wall thickness (DWT), left ventricle systolic free wall thickness (SWT), systolic blood pressure (SBP), diastolic blood pressure (DBP), velocity time integral for bloodflow through the mitral valve (VTI), and maximum velocity of bloodflow through the mitral valve (MV) were also obtained. Flow-mediated dilation and echocardiography were measured using a SonoSite Edge II ultrasound (Fujifilm SonoSite, Bothell, WA) with detection using a P10x transducer (8–4 Hz) to detect cardiac endpoints and the L38xi (10–5 Hz) transducer for vascular imaging. Flow-mediated dilation was calculated using the following equation:
Two-dimensional ultrasonography was used to measure left ventricular volume using the left parasternal apical two- and four-chamber views in diastole and systole (46). Two-dimensional guided M-mode echocardiography was used to obtain a right parasternal short-axis view of the heart at the level of the papillary muscles (46). Measurements of left ventricular end-diastolic inner diameter (LVIDd) and left ventricular end-systolic inner diameter (LVIDs) were also compiled from all dogs and normalized to body weight according to methodology described by Cornell et al. (47).
Statistical Analysis
Analyses were performed using SAS (version 9.4; SAS Institute, Cary, NC). Before performing all analyses, the data were explored for normality and outliers using the PROC UNIVARIATE model in SAS and the Shapiro–Wilk test. One-way ANOVA was used to compare differences among diets among normal vs. high protein commercial diets and two-way ANOVA was used to compare parameters for fava bean–based diets (fixed effects being fava bean variety and fermentation). In the present study, we used mixed-sex dogs to control the variation possibly associated with this factor. Previous studies from our group have not detected sex-related differences among (spayed/neutered) dogs (44) and because sex-related differences are not related to the purposes of the present study, we did not include this factor in the model. All post-hoc analyses were performed using the Fisher least significant difference (LSD) method. Differences were considered significant at P < 0.05.
Results
Body Weight, Meal Portion, and Body Condition Score
Body weight (BW), BCS, and meal portion data are presented in Table 1. No significant effect (P > 0.05) of dietary protein content (NP vs. HP) was observed on BCS or BW after 7 days of feeding in beagles. Within commercial diets, meal portion (163–187 g/day) was significantly smaller (P < 0.05) in HP compared with NP-fed dogs to restrict meal size to an isocaloric amount among diets. Within fava bean–based diets, there was no effect of either FM, FB, or the interaction between FM and FB on body weight, BCS, or meal portion (P > 0.10).
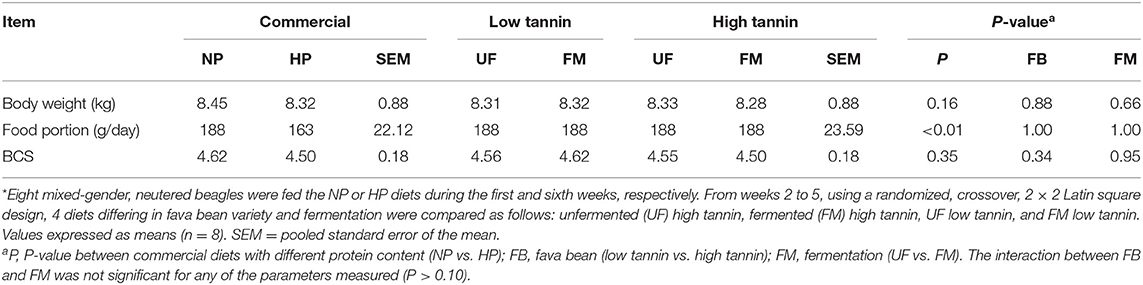
Table 1. Body weight, food portion, and body condition score (BCS) of dogs fed diets formulated with either low or high tannin fava beans without (UF) or with (FM) fermentation, or normal (NP) vs. high (HP) protein commercial diets for 7 days each*.
Glucose Tolerance
Time course of blood glucose responses to the oral glucose tolerance test are shown in Figure 1 with fasting and peak glucose levels (mmol/L), time to peak (min), and area under the curve (mmol/L × min) data shown in Table 2. There was no effect (P > 0.10) of dietary protein content (NP vs. HP) and no effect of FM, FB, or the interaction between FM and FB on fasting and peak glucose levels, time to peak, or AUC.
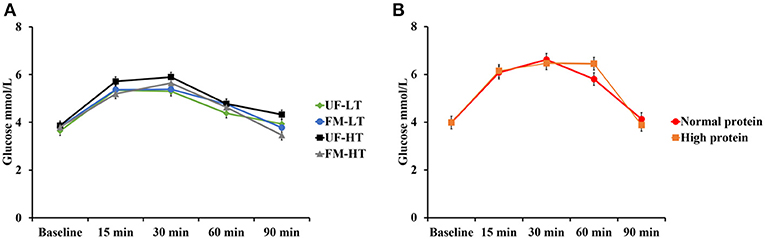
Figure 1. Blood glucose responses to the oral glucose tolerance test in dogs fed either low (LT) or high (HT) tannin fava bean–based diets without (UF) or with (FM) fermentation (A), or normal vs. high protein commercial diets (B). Eight mixed-gender, neutered beagles were fed the NP, or HP diets during the first and sixth weeks, respectively. From weeks 2 to 5, using a randomized, crossover, 2 × 2 Latin square design, 4 diets differing in fava bean variety and fermentation were compared as follows: UF-HT, FM-HT, UF-LT, and FM-LT. Fasting and peak glucose levels, time to peak, and area under the curve (AUC) were not influenced by dietary treatments (P > 0.10).
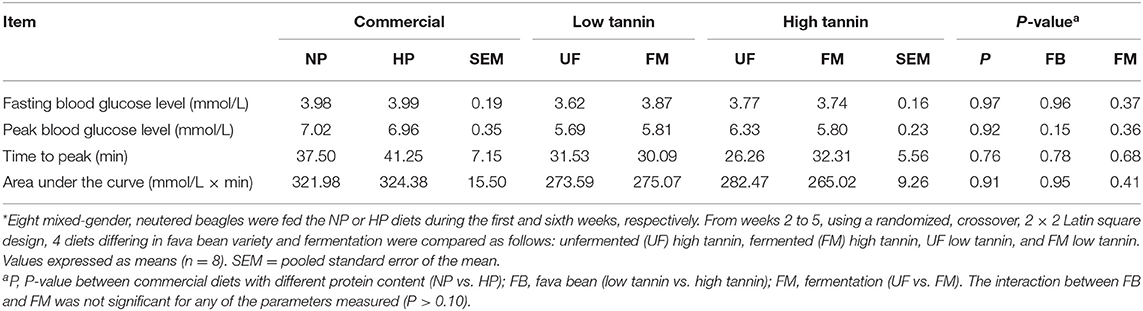
Table 2. Fasting and peak blood glucose levels, time to peak, and area under the curve of dogs fed diets formulated with either low or high tannin fava beans without (UF) or with (FM) fermentation, or normal (NP) vs. high (HP) protein commercial diets for 7 days each*.
Red and White Blood Cell Count
White blood cell and RBC of beagles after feeding each diet for 7 days are shown in Figure 2. Dogs fed HP diets showed decreased RBC compared with NP-fed dogs (P < 0.05). Conversely, WBC tended to increase in dogs fed HP diets compared with NP-fed dogs (P < 0.10). Within fava bean–based diets, FM increased RBC regardless of FB (P < 0.05) and no effect (P > 0.10) of either FM, FB, or the interaction between FM and FB was observed on WBC.
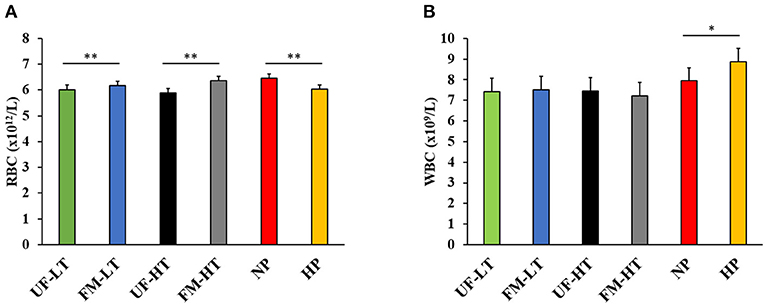
Figure 2. Red [RBC; (A)] and white [WBC; (B)] blood cell counts in dogs fed either low (LT) or high (HT) tannin fava bean–based diets without (UF) or with (FM) fermentation, or normal vs. high protein commercial diets. Eight mixed-gender, neutered beagles were fed the NP, or HP diets during the first and sixth weeks, respectively. From weeks 2 to 5, using a randomized, crossover, 2 × 2 Latin square design, 4 diets differing in fava bean variety and fermentation were compared as follows: UF-HT, FM-HT, UF-LT, and FM-LT *P < 0.10; **P < 0.05.
Blood Parameters of Hepatic Function
Blood parameters indicative of hepatic function in beagles after feeding test diets for 7 days are shown in Table 3. Within commercial diets, serum cholesterol was increased, while bilirubin parameters (TB, DB, and IB) were decreased in dogs fed HP diets compared with NP-fed dogs (P < 0.05). Furthermore, serum ALP and ALT were decreased in dogs fed HP diets compared with NP-fed dogs (P < 0.05). Moreover, total serum protein was decreased in dogs fed HP diets compared with NP-fed dogs, primarily due to a decrease in serum globulin, which elevated the albumin:globulin ratio (P < 0.05). There was no effect of dietary protein content on GGT, GLDH, CK, and albumin (P > 0.10). Among fava bean–based diets, DB was higher in dogs fed LT compared to HT-fed dogs regardless of FM (P < 0.05). There was no significant effect (P > 0.10) of either FM, FB, or the interaction between FM and FB on any other blood parameter of hepatic function measured.
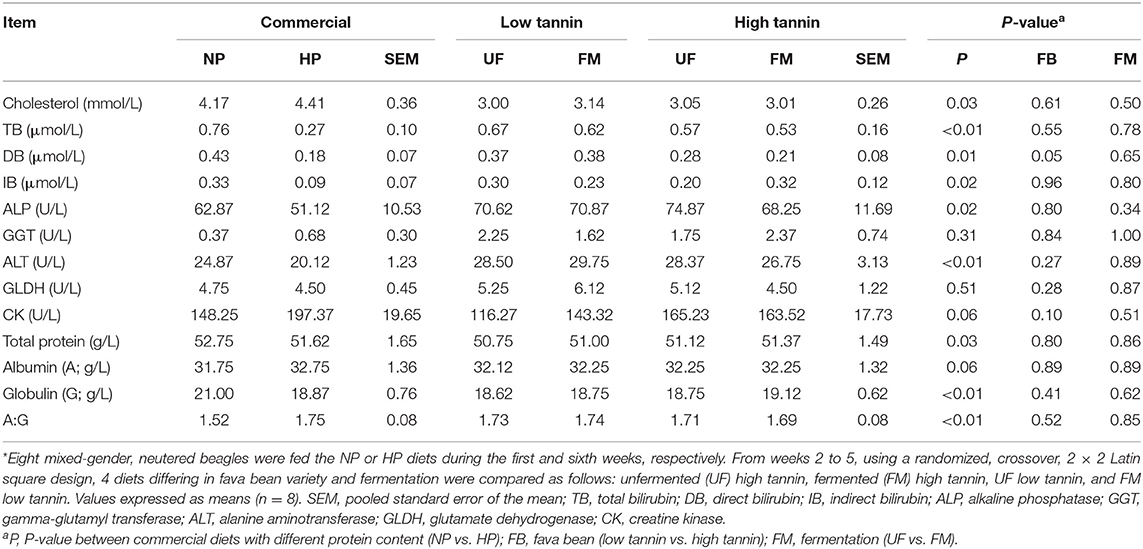
Table 3. Blood parameters of hepatic function in dogs fed diets formulated with either low or high tannin fava beans without (UF) or with (FM) fermentation, or normal (NP) vs. high (HP) protein commercial diets for 7 days each*.
Blood Electrolytes
Blood electrolytes from beagles after feeding each test diet for 7 days are shown in Table 4. Within commercial diets, serum bicarbonate, Ca, and P were increased in dogs fed HP compared with NP-fed dogs (P < 0.05). There was no effect (P > 0.10) of serum protein content on Na, K, Na:K, Cl, anion gap, or Mg. Among fava bean–based diets, serum P was increased in dogs fed HT compared with LT-fed dogs, regardless of FM (P < 0.05). Furthermore, FM tended to increase serum P compared with UF, regardless of FB (P < 0.05). There was an interaction between FB and FM for serum K and Na:K (P < 0.05). Dogs fed FM-HT diets showed the highest serum K, UF-HT the lowest, with FM-LT and UF-LT being intermediate. Consequently, dogs fed UF-HT diets showed the highest serum Na:K, and FM-HT the lowest, with FM-LT and UF-LT being intermediate. There was no significant effect (P > 0.10) of either FM, FB, or the interaction between FM and FB on serum Na, Cl, bicarbonate, anion gap, Ca, and Mg.
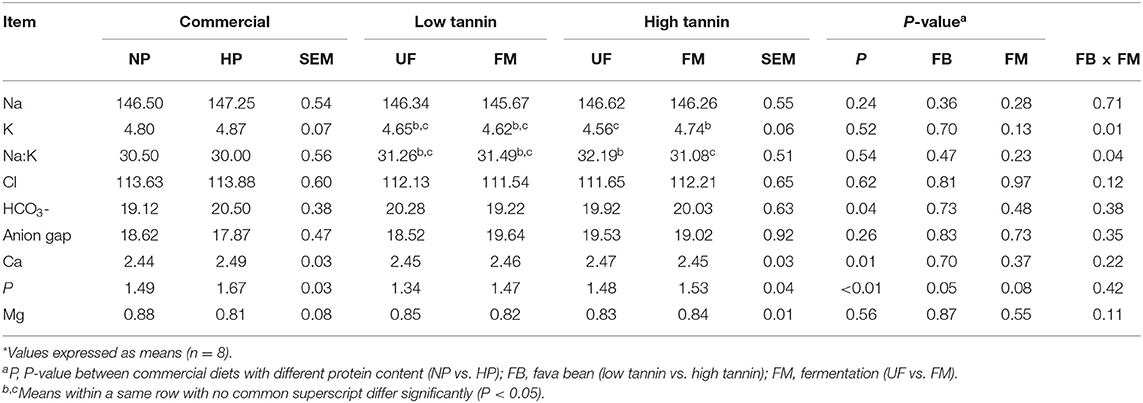
Table 4. Blood electrolytes (mmol/L) of dogs fed diets formulated with either low or high tannin fava beans without (UF) or with (FM) fermentation, or normal (NP) vs. high (HP) protein commercial diets for 7 days each*.
Blood Urea and Creatinine
Blood urea and creatinine of beagles after feeding each test diet for 7 days are shown in Figure 3. Serum urea was increased (P < 0.05) while creatinine tended to increase (P < 0.10) in dogs fed HP compared with NP-fed dogs. There was no significant effect (P > 0.10) of either FM, FB, or the interaction between FM and FB on serum urea and creatinine.
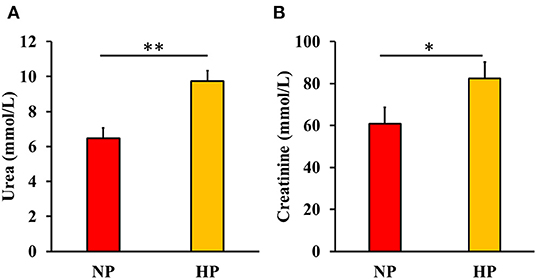
Figure 3. Blood urea (A) and creatinine (B) content in dogs fed either normal (NP) or high protein (HP) commercial diets. Eight mixed-gender, neutered beagles were fed the NP, or HP diets during the first and sixth weeks, respectively *P < 0.10; **P < 0.05. There was no effect of either fava bean (FB), fermentation (FM), or the interaction between FB and FM on urea and creatinine content (P > 0.05).
Digestive Enzymes in Blood
After 7 days of feeding each test diet to dogs, there was an interaction between FB and FM, where serum amylase was highest in FM-HT, lowest in FM-LT, but intermediate in UF-LT and UF-HT (P < 0.05; Figure 4). However, there was no significant effect of either FM or FB on serum amylase (P > 0.10). Moreover, dietary protein content did not significantly impact serum amylase (P > 0.10).
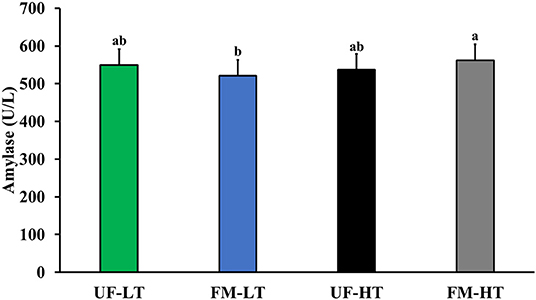
Figure 4. Blood amylase content in dogs fed either low (LT) or high (HT) tannin fava bean–based diets without (UN) or with (FM) fermentation. From weeks 2 to 5, using a randomized, crossover, 2 × 2 Latin square design, 8 mixed-gender, neutered beagles were fed 4 diets differing in fava bean variety and fermentation as follows: UF-HT, FM-HT, UF-LT, and FM-LT. Bars with no common letter (a, b) differ significantly (P < 0.05). There was no effect of dietary protein content on serum amylase content (P > 0.05).
Cardiovascular Function
Cardiovascular function parameters of beagles after 7 days of feeding each test diet are shown in Table 5. Dogs fed HP had decreased left ventricular end-systolic volume (ESV) and cardiac output (CO) compared with NP-fed dogs (P < 0.05). Dogs fed FM diets tended to have decreased maximum velocity (MV) of passive ventricular filling (the early or E wave) compared with UF-fed dogs regardless of FB (P < 0.10). There was no significant effect of protein content or either FM, FB, or the interaction between FM and FB on left ventricular end-diastolic volume (EDV), stroke volume (SV), heart rate (HR), ejection fraction (EF), left ventricular diastolic wall thickness (DWT), left ventricular systolic wall thickness (SWT), systolic blood pressure (SBP), diastolic blood pressure (DBP), flow-mediated dilation (FMD), velocity time integral for ventricular filling (VTI) (E and A wave combined), and MV (A wave) (P > 0.10). Moreover, there was no significant effect of dietary treatments on LVIDd and LVIDs (P > 0.10).
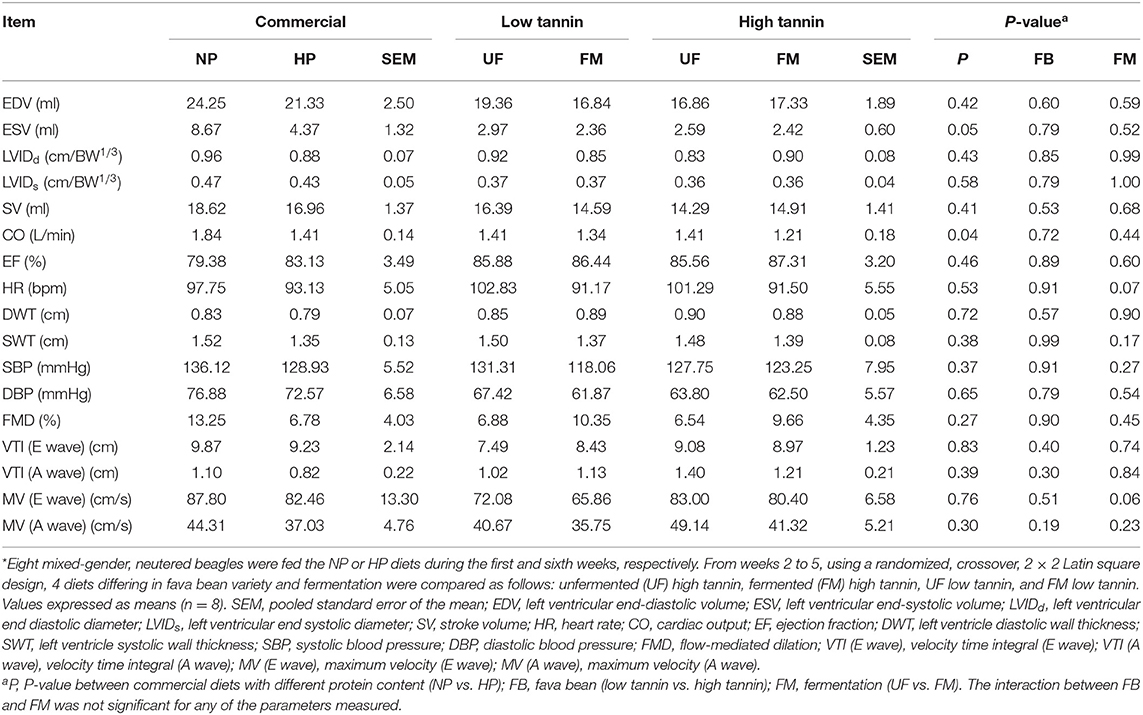
Table 5. Cardiovascular function parameters of dogs fed diets formulated with either low or high tannin fava beans without (UF) or with (FM) fermentation, or normal (NP) vs. high (HP) protein commercial diets for 7 days each*.
Digestibility
Apparent total tract digestibility in beagles fed fava bean–based diets are shown in Table 6. Dogs fed FM diets had decreased fat digestibility and increased non-fiber carbohydrates digestibility compared with UF diets regardless of FB (P < 0.05). There was no significant effect of FM, FB, or the interaction between FM and FB on digestibility of crude protein, gross energy, methionine, cysteine, or taurine (P > 0.10).
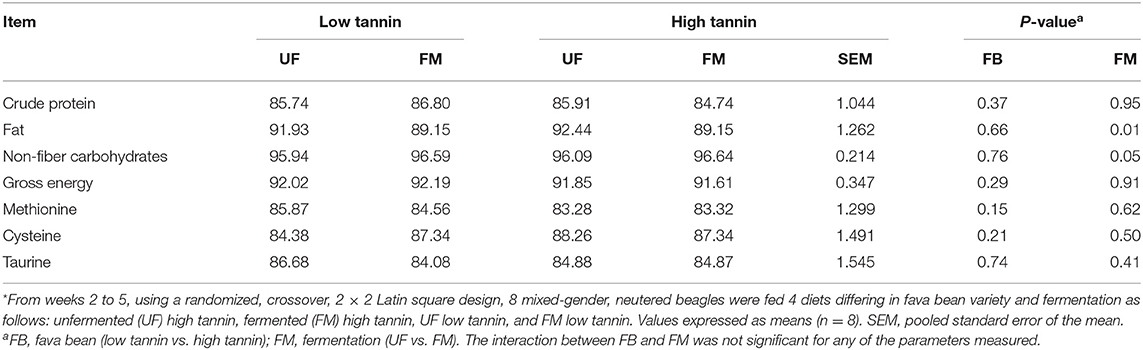
Table 6. Apparent total tract digestibility of dogs fed diets formulated with either low or high tannin fava beans without (UF) or with (FM) fermentation for 7 days each*.
Plasma Amino Acid Levels
Plasma amino acid concentrations in beagles after 7 days of feeding each test diet are shown in Table 7. Dogs fed HP diets had increased taurine, cystine, and cysteine concentrations compared with NP-fed dogs (P < 0.05). There was no significant effect of dietary protein content on plasma methionine concentration (P > 0.10). Plasma cysteine was increased in HT-fed dogs compared with LT-fed dogs regardless of fermentation (P < 0.05). There was no significant effect of fava bean variety on plasma taurine, cystine, and methionine levels (P > 0.05). However, fermentation increased plasma cysteine (P < 0.05) and tended to decrease plasma cystine (P < 0.10) regardless of fava bean variety. There were no significant interactions between fava bean variety and fermentation on plasma amino acid levels (P > 0.10).
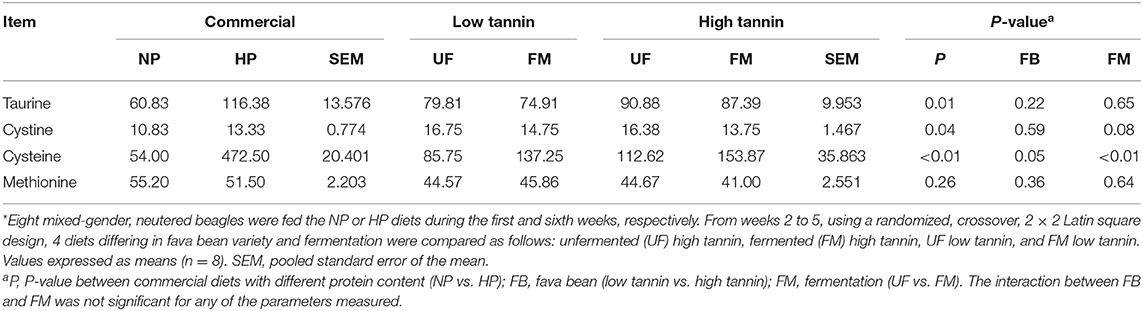
Table 7. Plasma amino acid levels (nmol/ml) of dogs fed diets formulated with either low or high tannin fava beans without (UF) or with (FM) fermentation, or normal (NP) vs. high (HP) protein commercial diets for 7 days each*.
Discussion
The objective of this study was to determine if neutered, mixed-gender, adult beagles fed diets with 30% inclusion of fava bean flour would show altered nutrient digestibility, glucose tolerance, overall health, cardiovascular function, and plasma amino acid levels when contrasted to commercial diets with normal vs. high protein. Fava bean diets had moderate protein levels, but were formulated to be near the AAFCO dietary minimums for methionine (0.33% inclusion) or cystine + methionine (0.65% inclusion) (35). This was done intentionally to cause more rapid changes in sulfur-containing amino acid levels in the dogs and potentially cause early, reversible impairments in cardiac function despite using only a 7-day feeding period for each test diet. All fava bean diets in this study met the cystine + methionine AAFCO minimum, but all were slightly below the minimum for methionine alone due to variations in methionine content of the fava beans from reported literature values that were used for diet formulation (26).
Lack of Evidence for Toxicity From Fava Beans, Digestibility, Glucose Tolerance, and Antinutritional Factors
Fava beans are pulses, a subset of legumes. Other legumes such as peas have been increasingly included in dog diets as a protein and fiber source (48, 49). Pulse ingredients have been controversially associated with grain-free diets and the occurrence of DCM in dogs (13). Specific to fava beans, however, is the additional association from vicine and convicine antinutritional factors with hemolytic anemia in susceptible humans (50). Worries about potential dog toxicity have prevented its AAFCO approval as a dog food ingredient thus far. Fermentation has been used as a valuable approach to reduce anti-nutritional factors in pulses, including trypsin inhibitors, hemagglutinins, and saponins. Moreover, Candida species have the potential to synthesize and increase taurine content (32) as well as improve protein digestibility through its breakdown into amino acids by fermentative microorganisms (51). This current study is the first to show that fermentation with C. utilis can successfully reduce the vicine/convicine content in fava bean flour, but had no effect on taurine content, protein digestibility, or amino acid digestibility. Of interest, however, is the observation that fermented fava bean diets both caused increases in plasma cysteine after 7 days of feeding, an effect that does not seem to relate to dietary levels and has no current explanation that should be explored in future studies.
The low-tannin variety used in the present study, Snowdrop, is known to have tannin levels as low as 1% (52), while Florent, which was the high tannin variety used in the present study, has been classified as a normal (higher) tannin genotype in Canada (2). Generally, other antinutritional factors tend to be high in varieties with high tannins, but both fava bean varieties used in the present study had high concentration of the antinutritional factors vicine and convicine. The main issue associated with high tannin content in the diet is related to reduced bioavailability of nutrients in the gastrointestinal tract (53, 54). Despite not evaluating bioavailability in the present study, there was no effect of fava bean variety on digestibility values of any nutrient measured. This implies that despite the potential negative effects of tannins on, specially, protein digestibility (55), we do not have evidence to show a clear detrimental effect of tannins. However, within-animal variation in digestive response to tannins and low sample size may have prevented detection of an impact on digestibility (56). Interestingly, fermentation was able to dramatically reduce both the vicine and convicine content in fava bean–based diets, regardless of variety. In a recent study, fermentation with L. plantarum degraded the pyrimidine glycosides in fava bean flour within 48 h, which reduced the toxicity of the fermented fava bean as assessed through ex vivo assays on human blood (31). In the present study, there was no impairment in glucose tolerance in dogs fed fermented fava bean (FM) diets as revealed by the lack of effect on glucose baseline and peak levels, time to peak, and AUC. This leads to the conclusion either that fava beans do not have any effect on glucose utilization in dogs or that 7 days is not sufficient to alter glucose utilization. However, it should be noted that the four fava bean test diets in this study were fed sequentially in a crossover design. By the end of these four feeding periods, beagles had been fed fava bean–based diets for a month, with no change in glucose utilization from the previous NP period. Moreover, RBC content was unchanged in dogs fed fava beans compared with the NP diet. Taken together, because anemia is a primary sign of favism and none of the fava bean–based diets caused anemia in the dogs in the current study, it can be concluded that fava beans are not toxic in dogs. While AAFCO requires a 6-month feeding study for fava beans to be approved as a pet food ingredient, this study provides initial indications that they are a safe dog food ingredient.
The present study also provided other indication of fermentation of fava bean flour with C. utilis enhancing diet quality and consequently health in dogs. Fermentation significantly decreased the digestibility of fat and increased the digestibility of non-fiber carbohydrates. It has been shown in fish that the concentration of carbohydrates in the gut are inversely related to fat digestibility (57) and this is exacerbated when large amounts of starch are present (58). Surprisingly, amylase content in blood was increased in FM-HT compared with FM-LT with both unfermented diets being intermediate. This indicates that the fermentability of fava bean varieties may be different, which might be associated with the carbohydrate composition, releasing increased or decreased amounts of starch when fermented (59). Finally, dogs fed fermented diets showed increased RBC levels compared with those fed unfermented fava bean diets. Low RBC in young dogs is a common occurrence as RBC lifespan is shorter and young RBC contain less hemoglobin when compared with aging RBC (60). An increased RBC content in fermented diet–fed dogs could be associated with an accelerated production of blood cells and increased health that should be further explored in future studies.
Effect of High Dietary Protein Diet on Dog Health
The results of the current study using commercial diets also provide indications that high dietary protein can negatively affect overall health of dogs. Previous studies have reported that high protein dog diets may negatively impact gastrointestinal health, pre-disposing dogs to diarrhea (61). While diarrhea was not observed with the HP commercial diet tested in the current study, excessive protein intake has been reported to increase proteinuria and overload kidneys, potentially decreasing the overall health of dogs (62), and this is consistent with the higher serum cholesterol, urea, and creatinine observed with the HP diet in the current study. High serum cholesterol in dogs is consistent with a positive relationship reported between protein intake and cholesterol in humans (63). Moreover, bilirubin measurements (TB, DB, and IB) were decreased in HP-fed dogs compared with NP, which agrees with a recent study showing higher bilirubin concentrations in young pigs fed a protein restricted diet compared with a control diet (64). On the other hand, potential benefits of the HP diet in the current study come from the observed decrease in ALP and ALT in dogs fed HP compared with NP. In dogs, higher ALT content is directly associated with hepatocyte membrane damage and necrosis, whereas ALP is positively associated with biliary stasis (65, 66). Increased ALT and ALP in NP dogs may be interpreted as poor hepatic function (67). It should be noted that while trends for changes in blood parameters can be interpreted as positive or negative, all values for all end-points measured in this study fell within clinical norms and thus all dogs were maintained in a healthy state. Future studies using longer feeding periods are required to determine if trends continue and become clinically significant compared to that observed after 7 days in the current study.
Cardiac Function, Cysteine, Methionine, and Taurine
One of the main hypotheses of the present study was that pulse-based diets with their higher fiber would cause decreases in plasma taurine, cysteine, or methionine levels, subsequently leading to impaired cardiac contractility or enlargement of the heart consistent with DCM. However, after 7 days of feeding each fava bean–based diet, no significant adverse changes were detected in cardiac or vascular function in the current study. Longer feeding trials are needed to confirm that there are no effects. However, what did change in the short term was ESV that was increased in NP-fed dogs compared with HP-fed dogs, but without changes in ventricular chamber size (LVID). Changes in cardiac chamber size would be surprising after only 7 days, thus a functional change such as impaired contractility and reduce cardiac output (CO) is not expected to explain the higher ESV. In fact, the NP-fed dogs instead had higher CO that may be due to a non-significant trend for both HR and SV to increase. This is consistent with a similar non-significant trend for both systolic and diastolic blood pressure to increase in NP-fed dogs compared with HP-fed dogs, suggesting a generalized increase in sympathetic outflow in the NP-fed dogs. Higher sympathetic tone and blood pressure increases afterload and impairs emptying of the left ventricle at the end of systole (i.e., higher ESV), a reliable indicator of impaired systolic function (68).
The proximate analysis of diets showed no major differences in crude fiber content between laboratory-formulated fava bean–based diets and commercial diets. The main differences among diets were observed for cysteine content, which was low in the fava bean diets. Also, methionine and taurine content were highest in HP diets compared with all fava bean–based diets and the NP diet. If reduced dietary taurine levels were driving cardiac changes, then it would have been expected that the NP diet should have led to adverse cardiac changes and this may agree with what we observed. Future studies should explore longer feeding periods and address whether more susceptible dog breeds than beagles produce a stronger relationship between taurine and cardiac impairment.
The fava bean–based diets and the NP commercial diet tested in the current study all had methionine content that was just below the AAFCO minimum. Dogs can synthesize taurine from cysteine or methionine (69), but all three of these amino acids tend to be low or limiting when plant-based protein sources such as pulses are used. Compounding this problem, pulses are high in resistant starch and fiber. Previous studies in dogs have reported that high fiber diets decrease protein digestibility and increase fecal bile acid excretion in feces (70). Because taurocholate is the major bile salt excreted by dogs, the net effect of high fiber has been reported to deplete taurine and impair digestion of protein that contains cysteine and methionine needed to replace it. However, none of the diets tested caused any significant drop in plasma levels of taurine, cysteine, or methionine and levels remained above the reference range throughout the study (27). In fact, fermented fava beans (both varieties) led to significant increases in plasma cysteine, suggesting a potentially beneficial health effect of fermentation processing. In contrast, the high protein (HP) commercial diet led to higher plasma levels of cysteine and taurine, but no change in plasma methionine compared with NP diet, suggesting that 7 days was sufficient time to cause some alterations of blood levels of these amino acids. Cysteine results, however, should be cautiously interpreted due to its unstable nature and an interfering substance during HPLC analysis of this amino acid that could have led to overestimation of cysteine levels (71). Further analysis using a different method would be necessary to confirm the cysteine results.
Strength and Limitations
A strength of this study was that the fava bean–based diets that were made in our laboratory were tested against two popular commercial brands, giving a more realistic context to the results. Another strength was the use of a Latin square, crossover design where the same dogs were tested on each diet, reducing variability and increasing power with the small sample size of this experiment. However, an important limitation of this study was the duration, which is insufficient to cause major structural cardiac changes, but was long enough to change plasma levels of sulfur-containing amino acid levels, at least in response to high dietary protein. Moreover, the results of this study using young, healthy adult beagles may not apply to older, large breed dogs with genetic susceptibility to taurine deficiency. However, we would predict that changes in taurine and sulfur-containing amino acids would be even more pronounced in these breeds or in older dogs. A last limitation of the present study was the experimental design which does not allow the statistical comparison between commercial and fava bean-based diets.
Conclusion and Implications
Most importantly, fava bean–based diets did not cause hemolytic anemia and did not alter glucose handling in dogs after 7 days of feeding, thus fava beans appear safe as a dog food ingredient. In contrast, the high-protein grain-free commercial diet adversely altered blood chemistry compared with the normal protein, grain-containing commercial diet we tested. Moreover, the normal protein, grain-based diet appeared to cause excess sympathetic tone, a trend that if it were to continue with long-term feeding, might lead to adverse changes in cardiac health that are distinct from DCM. On the other hand, fermentation with C. utilis looks promising to reduce antinutritional factors and potentially improve health through improvements in nutrient digestibility and increased RBC levels in dogs. Studies using longer feeding periods are needed to determine whether these short-term changes are sustained to produce clinically significant changes in dogs.
Data Availability Statement
The raw data supporting the conclusions of this article will be made available by the authors, without undue reservation.
Ethics Statement
The animal study was reviewed and approved by University of Saskatchewan Animal Research Ethics Board.
Author Contributions
LGR, ML, and LW designed the study. LGR, TM, and CQ conducted the study. LGR and LAR performed data analysis. LGR and LW wrote the article. LW was responsible for final content of the article. All authors contributed to the interpretation of the results throughout the study and have read and approved the article.
Funding
Funding for this project was provided by Saskatchewan Pulse Growers, Western Grains Research Foundation, and Government of Saskatchewan.
Conflict of Interest
LAR was affiliated with Prairie Swine Centre (Saskatoon, SK, Canada) where he used the facility to do his graduate research. The authors also declare that this study received funding or in-kind support from the Saskatchewan Pulse Growers, Western Grains Research Foundation, Alliance Grain Traders (Saskatoon, SK, Canada) and Horizon Pet Foods (Rosthern, SK, Canada). The funders were not involved in the study design, collection, analysis, interpretation of data, the writing of this article, or the decision to submit it for publication.
Acknowledgments
The authors would like to thank the staff at the Animal Care Unit of the Western College of Veterinary Medicine and Canadian Feed Research Centre for their assistance.
Supplementary Material
The Supplementary Material for this article can be found online at: https://www.frontiersin.org/articles/10.3389/fvets.2021.653771/full#supplementary-material
Abbreviations
ALP, alkaline phosphatase; ALT, alanine aminotransferase; AUC, area under the curve; CK, creatine kinase; CO, cardiac output; CP, crude protein; DCM, dilated cardiomyopathy; DB, direct bilirubin; DBP, diastolic blood pressure; DWT, left ventricular diastolic wall thickness; EDV, left ventricular end-diastolic volume; EF, ejection fraction; ESV, left ventricular end-systolic volume; FM, fermented; FMD, flow-mediated dilation; G6PD, glucose-6-phosphate dehydrogenase; GGP, gamma-glutamyltransferase; GLDH, glutamate dehydrogenase; HP, high protein; HR, heart rate; HT, high-tannin fava bean variety; IB, indirect bilirubin; LT, low-tannin fava bean variety; LVIDd, left ventricular end-diastolic diameter; LVIDs, left ventricular end-systolic diameter; MV, maximum velocity; NP, normal protein; RBC, red blood cell; SBP, systolic blood pressure; SV, stroke volume; SWT, left ventricular systolic wall thickness; TB, total bilirubin; UF, unfermented; VTI, velocity time integral; WBC, white blood cell.
References
1. de Boer J, Aiking H. On the merits of plant-based proteins for global food security: marrying macro and micro perspectives. Ecol Econ. (2011) 70:1259–65. doi: 10.1016/j.ecolecon.2011.03.001
2. Fleury D, Barker B. Faba Bean Variety Report 2015/16. (2015). Available online at: https://saskpulse.com/files/general/151026_Faba_bean_variety_report2.pdf (accessed February 21, 2021).
3. Rahate KA, Madhumita M, Prabhakar PK. Nutritional composition, anti-nutritional factors, pretreatments-cum-processing impact and food formulation potential of faba bean (Vicia faba L.): a comprehensive review. LWT. (2021) 138:110796. doi: 10.1016/j.lwt.2020.110796
4. U.S. Food and Drug Administration. FDA Investigation Into Potential Link Between Certain Diets and Canine Dilated Cardiomyopathy. (2019). Available online at: https://www.fda.gov/animal-veterinary/outbreaks-and-advisories/fda-investigation-potential-link-between-certain-diets-and-canine-dilated-cardiomyopathy (accessed February 21, 2021).
5. Dutton E, López-Alvarez J. An update on canine cardiomyopathies – is it all in the genes? J Small Anim Pract. (2018) 59:455–64. doi: 10.1111/jsap.12841
6. Monnet E, Orton EC, Salman M, Boon J. Idiopathic dilated cardiomyopathy in dogs: survival and prognostic indicators. J Vet Intern Med. (1995) 9:12–7. doi: 10.1111/j.1939-1676.1995.tb03266.x
7. Borgarelli M, Santilli RA, Chiavegato D, D'Agnolo G, Zanatta R, Mannelli A, et al. Prognostic indicators for dogs with dilated cardiomyopathy. J Vet Intern Med. (2006) 20:104–10. doi: 10.1111/j.1939-1676.2006.tb02829.x
8. Werner P, Raducha MG, Prociuk U, Sleeper MM, Van Winkle TJ, Henthorn PS. A novel locus for dilated cardiomyopathy maps to canine chromosome 8. Genomics. (2008) 91:517–21. doi: 10.1016/j.ygeno.2008.03.007
9. Martin MWS, Stafford Johnson MJ, Celona B. Canine dilated cardiomyopathy: a retrospective study of signalment, presentation and clinical findings in 369 cases. J Small Anim Pract. (2009) 50:23–9. doi: 10.1111/j.1748-5827.2008.00659.x
10. Kramer GA, Kittleson MD, Fox PR, Lewis J, Pion PD. Plasma taurine concentrations in normal dogs and in dogs with heart disease. J Vet Intern Med. (1995) 9:253–8. doi: 10.1111/j.1939-1676.1995.tb01076.x
11. Bélanger MC, Ouellet M, Queney G, Moreau M. Taurine-Deficient dilated cardiomyopathy in a family of golden retrievers. J Am Anim Hosp Assoc. (2005) 41:284–91. doi: 10.5326/0410284
12. Maron BJ, Towbin JA, Thiene G, Antzelevitch C, Corrado D, Arnett D, et al. Contemporary definitions and classification of the cardiomyopathies: an American heart association scientific statement from the council on clinical cardiology, heart failure and transplantation committee; quality of care and outcomes research and functional genomics and translational biology interdisciplinary working groups; and council on epidemiology and prevention. Circulation. (2006) 113:1807–16. doi: 10.1161/CIRCULATIONAHA.106.174287
13. Mansilla WD, Marinangeli CPF, Ekenstedt KJ, Larsen JA, Aldrich G, Columbus DA, et al. Special topic: the association between pulse ingredients and canine dilated cardiomyopathy: addressing the knowledge gaps before establishing causation. J Anim Sci. (2019) 97:983–97. doi: 10.1093/jas/sky488
14. McCauley SR, Clark SD, Quest BW, Streeter RM, Oxford EM. Review of canine dilated cardiomyopathy in the wake of diet-associated concerns. J Anim Sci. (2020) 98:skaa155. doi: 10.1093/jas/skaa155
15. Kaplan JL, Stern JA, Fascetti AJ, Larsen JA, Skolnik H, Peddle GD, et al. Taurine deficiency and dilated cardiomyopathy in golden retrievers fed commercial diets. PLoS ONE. (2018) 13:e0209112. doi: 10.1371/journal.pone.0209112
16. Fascetti AJ, Reed JR, Rogers QR, Backus RC. Taurine deficiency in dogs with dilated cardiomyopathy: 12 cases (1997–2001). J Am Vet Med Assoc. (2003) 223:1137–41. doi: 10.2460/javma.2003.223.1137
17. Backus RC, Ko KS, Fascetti AJ, Kittleson MD, MacDonald KA, Maggs DJ, et al. Low plasma taurine concentration in newfoundland dogs is associated with low plasma methionine and cyst(e)ine concentrations and low taurine synthesis. J Nutr. (2006) 136:2525–33. doi: 10.1093/jn/136.10.2525
18. Bakker AJ, Berg HM. Effect of taurine on sarcoplasmic reticulum function and force in skinned fast-twitch skeletal muscle fibres of the rat. J Physiol. (2002) 538:185–94. doi: 10.1113/jphysiol.2001.012872
19. Sanderson SL. Taurine and carnitine in canine cardiomyopathy. Vet Clin Small Anim Pract. (2006) 36:1325–43. doi: 10.1016/j.cvsm.2006.08.010
20. Kim SW, Rogers QR, Morris JG. Dietary antibiotics decrease taurine loss in cats fed a canned heat-processed diet. J Nutr. (1996) 126:509–15. doi: 10.1093/jn/126.2.509
21. Kim SW, Rogers QR, Morris JG. Maillard reaction products in purified diets induce taurine depletion in cats which is reversed by antibiotics. J Nutr. (1996) 126:195–201. doi: 10.1093/jn/126.1.195
22. O'Máille ER, Richards TG, Short AH. Acute taurine depletion and maximal rates of hepatic conjugation and secretion of cholic acid in the dog. J Physiol. (1965) 180:67–79.
23. Story JA, Kritchevsky D. Bile acid metabolism and fiber. Am J Clin Nutr. (1978) 31:S199–202. doi: 10.1093/ajcn/31.10.S199
24. Liener IE. Antinutritional factors in legume seeds: state of the art. Recent Adv Res Antinutritional Factors Legume Seeds. 1st ed. (1989) 6–13.
25. Luzzatto L, Arese P. Favism and glucose-6-phosphate dehydrogenase deficiency. N Engl J Med. (2018) 378:60–71. doi: 10.1056/NEJMra1708111
26. Frias J, Peñas E, Martinez-Villaluenga C. Chapter 16 - ". In: Frias J, Martinez-Villaluenga C, Peñas E, editors. Fermented Foods in Health and Disease Prevention. Cambridge, MA: Academic Press (2017). p. 385–416. doi: 10.1016/B978-0-12-802309-9.00016-9
27. Coda R, Rizzello CG, Gobbetti M. Use of sourdough fermentation and pseudo-cereals and leguminous flours for the making of a functional bread enriched of γ-aminobutyric acid (GABA). Int J Food Microbiol. (2010) 137:236–45. doi: 10.1016/j.ijfoodmicro.2009.12.010
28. Curiel JA, Coda R, Centomani I, Summo C, Gobbetti M, Rizzello CG. Exploitation of the nutritional and functional characteristics of traditional Italian legumes: the potential of sourdough fermentation. Int J Food Microbiol. (2015) 196:51–61. doi: 10.1016/j.ijfoodmicro.2014.11.032
29. Santana FC, Empis J. Bacterial removal of quinolizidine alkaloids from Lupinus albus flours. Eur Food Res Technol. (2001) 212:217–24. doi: 10.1007/s002170000221
30. Coda R, Melama L, Rizzello CG, Curiel JA, Sibakov J, Holopainen U, et al. Effect of air classification and fermentation by Lactobacillus plantarum VTT E-133328 on faba bean (Vicia faba L.) flour nutritional properties. Int J Food Microbiol. (2015) 193:34–42. doi: 10.1016/j.ijfoodmicro.2014.10.012
31. Rizzello CG, Losito I, Facchini L, Katina K, Palmisano F, Gobbetti M, et al. Degradation of vicine, convicine and their aglycones during fermentation of faba bean flour. Sci Rep. (2016) 6:32452. doi: 10.1038/srep32452
32. Hébert A, Forquin-Gomez M-P, Roux A, Aubert J, Junot C, Heilier J-F, et al. New insights into sulfur metabolism in yeasts as revealed by studies of Yarrowia lipolytica. Appl Environ Microbiol. (2013) 79:1200–11. doi: 10.1128/AEM.03259-12
33. Curso Almeida P. Effects of Pea Starch Yeast Fermentation on Glycemic Index, Palatability, Metabolic Status and Intestinal Health of Dogs and Cats Fed a Pea-Based Diet. [dissertation/master's thesis], Saskatoon (SK): University of Saskatchewan (2020).
34. National Research Council. Nutrient Requirements of Dogs and Cats. Washington, DC: The National Academies Press (2006).
35. Association of American Feed Control Officials. Official Publication. Champaign, IL: AAFCO (2017).
36. AOAC. Association of Official Analytical Chemists Official Methods of Analysis. 21st ed. Washington, DC: AOAC (2019).
37. Zhang F, Adeola O. Techniques for evaluating digestibility of energy, amino acids, phosphorus, and calcium in feed ingredients for pigs. Anim Nutr. (2017) 3:344–52. doi: 10.1016/j.aninu.2017.06.008
38. Wolever TM, Jenkins DJ, Jenkins AL, Josse RG. The glycemic index: methodology and clinical implications. Am J Clin Nutr. (1991) 54:846–54. doi: 10.1093/ajcn/54.5.846
39. Delaney SJ, Kass PH, Rogers QR, Fascetti AJ. Plasma and whole blood taurine in normal dogs of varying size fed commercially prepared food. J Anim Physiol Anim Nutr. (2003) 87:236–44. doi: 10.1046/j.1439-0396.2003.00433.x
40. Tôrres CL, Backus RC, Fascetti AJ, Rogers QR. Taurine status in normal dogs fed a commercial diet associated with taurine deficiency and dilated cardiomyopathy. J Anim Physiol Anim Nutr. (2003) 87:359–72. doi: 10.1046/j.1439-0396.2003.00446.x
41. Spitze AR, Wong DL, Rogers QR, Fascetti AJ. Taurine concentrations in animal feed ingredients; cooking influences taurine content. J Anim Physiol Anim Nutr. (2003) 87:251–62. doi: 10.1046/j.1439-0396.2003.00434.x
42. Heinze CR, Larsen JA, Kass PH, Fascetti AJ. Plasma amino acid and whole blood taurine concentrations in cats eating commercially prepared diets. Am J Vet Res. (2009) 70:1374–82. doi: 10.2460/ajvr.70.11.1374
43. Raitakari OT, Celermajer DS. Research methods in human cardiovascular pharmacology. Br J Clin Pharmacol. (2000) 50:397–404. doi: 10.1046/j.1365-2125.2000.00277.x
44. Adolphe JL, Drew MD, Huang Q, Silver TI, Weber LP. Postprandial impairment of flow-mediated dilation and elevated methylglyoxal after simple but not complex carbohydrate consumption in dogs. Nutr Res. (2012) 32:278–84. doi: 10.1016/j.nutres.2012.03.002
45. Otto CM, Schwaegler RG, Freeman RV, Linefsky J. Echocardiography Review Guide E-Book: Companion to the Textbook of Clinical Echocardiography. Philadelphia, PA: Elsevier Health Sciences. (2019) 456p.
46. Lang RM, Bierig M, Devereux RB, Flachskampf FA, Foster E, Pellikka PA, et al. Recommendations for chamber quantification: a report from the American society of echocardiography's guidelines and standards committee and the chamber quantification writing group, developed in conjunction with the European association of echocardiography, a branch of the European society of cardiology. J Am Soc Echocardiogr. (2005) 18:1440–63. doi: 10.1016/j.echo.2005.10.005
47. Cornell CC, Kittleson MD, Torre PD, Häggström J, Lombard CW, Pedersen HD, et al. Allometric scaling of m-mode cardiac measurements in normal adult dogs. J Vet Intern Med. (2004) 18:311–21. doi: 10.1111/j.1939-1676.2004.tb02551.x
48. Butterwick RF, Markwell PJ, Thorne CJ. Effect of level and source of dietary fiber on food intake in the dog. J Nutr. (1994) 124:2695S−700S. doi: 10.1093/jn/124.suppl_12.2695S
49. Rice JE, Ihle SL. Effects of diet on fecal occult blood testing in healthy dogs. Can J Vet Res. (1994) 58:134–7.
50. Champ MM-J. Non-nutrient bioactive substances of pulses. Br J Nutr. (2002) 88:307–19. doi: 10.1079/BJN2002721
51. Malcolmson L, Han J. pulse processing and utilization of pulse ingredients in foods. In: Dahl WJ, editor. Health Benefits of Pulses. Cham: Springer International Publishing (2019). p. 129–49. doi: 10.1007/978-3-030-12763-3_9
52. Wei X. Effects of Short-Term Germination and Autoclaving on Selected Compounds in Faba Bean and Faba Bean Applications in Low-Fat Pork Bologna. [Dissertation/master's thesis], Saskatoon (SK): University of Saskatchewan (2019).
53. Addisu S. Effect of dietary tannin source feeds on ruminal fermentation and production of cattle; a review. Online J Anim Feed Res. (2016) 6:42–56.
54. Bunglavan SJ, Dutta N. Use of tannins as organic protectants of proteins in digestion of ruminants. J Livest Sci. (2013) 4:67–77.
55. Berard NC, Wang Y, Wittenberg KM, Krause DO, Coulman BE, McAllister TA, et al. Condensed tannin concentrations found in vegetative and mature forage legumes grown in western Canada. Can J Plant Sci. (2011) 91:669–75. doi: 10.4141/cjps10153
56. Hagerman AE, Robbins CT, Weerasuriya Y, Wilson TC, McArthur C. Tannin chemistry in relation to digestion. J Range Manag. (1992) 45:57–62. doi: 10.2307/4002526
57. Storebakken T, Shearer KD, Refstie S, Lagocki S, McCool J. Interactions between salinity, dietary carbohydrate source and carbohydrate concentration on the digestibility of macronutrients and energy in rainbow trout (Oncorhynchus mykiss). Aquaculture. (1998) 163:347–59. doi: 10.1016/S0044-8486(98)00259-2
58. Skrede G, Storebakken T, Skrede A, Sahlstrøm S, Sørensen M, Shearer KD, et al. Lactic acid fermentation of wheat and barley whole meal flours improves digestibility of nutrients and energy in Atlantic salmon (Salmo salar L.) diets. Aquaculture. (2002) 210:305–21. doi: 10.1016/S0044-8486(01)00851-1
59. Çalişkantürk Karataş S, Günay D, Sayar S. In vitro evaluation of whole faba bean and its seed coat as a potential source of functional food components. Food Chem. (2017) 230:182–8. doi: 10.1016/j.foodchem.2017.03.037
60. Bush BM. Interpretation of Laboratory Results for Small Animal Clinicians. Oxford: Blackwell Scientific Publications Ltd (1991) 515p.
61. Hang I, Heilmann RM, Grützner N, Suchodolski JS, Steiner JM, Atroshi F, et al. Impact of diets with a high content of greaves-meal protein or carbohydrates on faecal characteristics, volatile fatty acids and faecal calprotectin concentrations in healthy dogs. BMC Vet Res. (2013) 9:201. doi: 10.1186/1746-6148-9-201
62. Burkholder WJ, Lees GE, LeBlanc AK, Slater MR, Bauer JE, Kashtan CE, et al. Diet modulates proteinuria in heterozygous female dogs with x-linked hereditary nephropathy. J Vet Intern Med. (2004) 18:165–75. doi: 10.1111/j.1939-1676.2004.tb00157.x
63. Pasiakos SM, Lieberman HR, Fulgoni VL. Higher-Protein diets are associated with higher HDL Cholesterol and Lower BMI and waist circumference in US adults. J Nutr. (2015) 145:605–14. doi: 10.3945/jn.114.205203
64. Fisher KD, Scheffler TL, Kasten SC, Reinholt BM, Eyk GR van, Escobar J, et al. Energy dense, protein restricted diet increases adiposity and perturbs metabolism in young, genetically lean pigs. PLoS ONE. (2013) 8:e72320. doi: 10.1371/journal.pone.0072320
65. Willard MD, Twedt DC. Gastrointestinal, pancreatic, and hepatic disorders. Small Anim Clin Diagn Lab Methods. 5th ed. (2012) 191–225. doi: 10.1016/B978-1-4377-0657-4.00009-0
66. Lawrence YA, Dangott LJ, Rodrigues-Hoffmann A, Steiner JM, Suchodolski JS, Lidbury JA. Proteomic analysis of liver tissue from dogs with chronic hepatitis. PLoS ONE. (2018) 13:e0208394. doi: 10.1371/journal.pone.0208394
67. Lucena R, Novales M, Blanco B, Hernández E, Ginel PJ. Effect of probiotic Enterococcus faecium SF68 on liver function in healthy dogs. J Vet Intern Med. (2019) 33:2628–34. doi: 10.1111/jvim.15625
68. Kittleson MD, Kienle RD. Small Animal Cardiovascular Medicine. Milton: Elsevier Canada (1998) 603p.
69. Harrison M, Thomas G, Gilham M, Gray K, Colyer A, Allaway D. Short-term determination and long-term evaluation of the dietary methionine requirement in adult dogs. Br J Nutr. (2020) 123:1333–44. doi: 10.1017/S0007114520000690
70. Pezzali JG, Acuff HL, Henry W, Alexander C, Swanson KS, Aldrich CG. Effects of different carbohydrate sources on taurine status in healthy beagle dogs. J Anim Sci. (2020) 98:1–9. doi: 10.1093/jas/skaa010
Keywords: fava bean (Vicia faba), Candida utilis, fermentation, cardiovascular function, blood chemistry, glucose tolerance, domestic dog (Canis familiaris)
Citation: Reis LG, Morris T, Quilliam C, Rodrigues LA, Loewen ME and Weber LP (2021) The Effect of Fermentation of High- or Low-Tannin Fava Bean on Glucose Tolerance, Body Weight, Cardiovascular Function, and Blood Parameters in Dogs After 7 Days of Feeding: Comparison With Commercial Diets With Normal vs. High Protein. Front. Vet. Sci. 8:653771. doi: 10.3389/fvets.2021.653771
Received: 15 January 2021; Accepted: 11 March 2021;
Published: 11 May 2021.
Edited by:
Luciano Trevizan, Federal University of Rio Grande Do Sul, BrazilReviewed by:
F. Capela e Silva, Universidade de Évora, PortugalElsa Lamy, University of Évora, Portugal
Copyright © 2021 Reis, Morris, Quilliam, Rodrigues, Loewen and Weber. This is an open-access article distributed under the terms of the Creative Commons Attribution License (CC BY). The use, distribution or reproduction in other forums is permitted, provided the original author(s) and the copyright owner(s) are credited and that the original publication in this journal is cited, in accordance with accepted academic practice. No use, distribution or reproduction is permitted which does not comply with these terms.
*Correspondence: Lynn P. Weber, bHlubi53ZWJlciYjeDAwMDQwO3VzYXNrLmNh