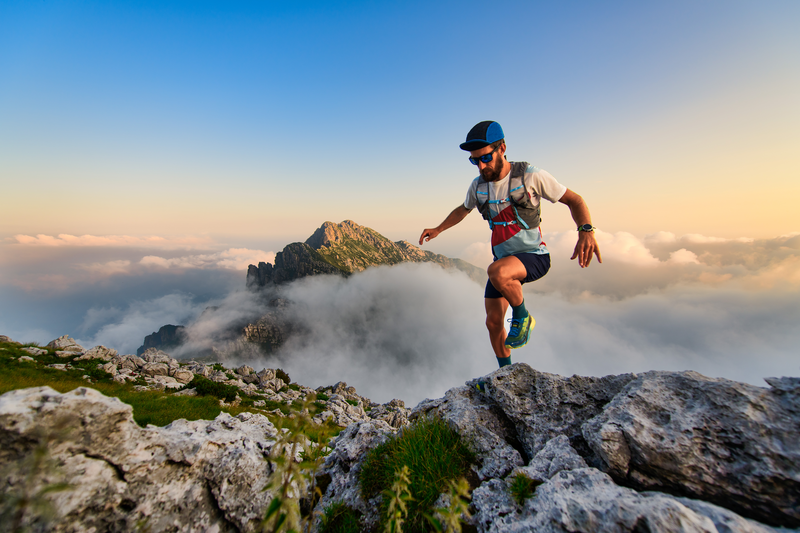
94% of researchers rate our articles as excellent or good
Learn more about the work of our research integrity team to safeguard the quality of each article we publish.
Find out more
ORIGINAL RESEARCH article
Front. Vet. Sci. , 04 March 2021
Sec. Veterinary Regenerative Medicine
Volume 8 - 2021 | https://doi.org/10.3389/fvets.2021.646087
Background: Chronic tubulointerstitial fibrosis is a common final pathway leading to end stage kidney disease in cats and has no effective treatment. The use of cell-based molecules to treat kidney fibrosis may be a promising approach. The objectives were to test the effects of intra-renal chemokine CXCL12 injection in a pre-clinical cat model of unilateral ischemia/reperfusion (I/R)-induced kidney fibrosis and then, within a clinical pilot study, test the safety/feasibility of CXCL12 injection in cats that might have early chronic kidney disease (CKD).
Methods: Pre-clinical: Thirty cats received intra-renal injection of 100, 200, or 400 ng of recombinant human CXCL12, or sterile saline, into the I/R kidney 70 days post-injury, or were non-injured, non-injected controls (n = 6/group). Kidney collagen content was quantified 4 months post-treatment using Masson's Trichrome and Picrosirius Red (PSR) stained tissues. In a separate study (n = 2) exploring short-term effects of CXCL12, 200 ng CXCL12 was injected into I/R kidneys and then harvested either 30 min (n = 1) or 1 month (n = 1) post-injection. Kidney concentrations of CXCL12, matrix metalloproteinase 1 (MMP-1), and lysyl oxidase-like enzyme 2 (LOXL-2) were quantified via ELISA. Clinical Pilot: 14 client-owned cats with potential early kidney disease received a single-treatment, bilateral intra-renal injection of 200 ng CXCL12 (n = 7), or received no injection (n = 7). Blood/urine samples were collected monthly for 9 months to assess renal function and CKD staging.
Results: Pre-clinical: I/R increased the affected kidney collagen content, which both mid and high doses of CXCL12 restored to normal (ps < 0.05 vs. untreated). I/R increased collagen fiber width, which both mid and high doses of CXCL12 restored to normal (p < 0.001 vs. untreated). Early changes in kidney MMP-1, associated with collagen breakdown, and subsequent decreases in LOXL-2, associated with collagen cross-linking, in response to CXCL12 treatment may contribute to these findings. Clinical Pilot: Bilateral intra-renal injection of CXCL12 using ultrasound guidance in cats with CKD was feasible and safe in a general practice clinical setting with no obvious side effects noted during the 9-month follow-up period.
Conclusions: Intra-renal injection of CXCL12 may prove to be an effective treatment for kidney fibrosis in cats with CKD. Additional mechanistic and clinical evaluations are needed.
Chronic kidney disease (CKD) affects ~30% of cats age 15 years or older with the prevalence increasing with age (1–5). Current treatments include pharmaceutical therapies and dietary management to slow disease progression, increase longevity, and improve quality of life, but alternatives are needed.
The underlying pathology leading to End Stage Renal Disease (ESRD) is chronic tubulointerstitial fibrosis (6–16). Kidney tissue regenerative therapies designed to restore functional kidney units have been tested in several studies. These include various combinations of bone marrow and adipose-derived mesenchymal stem cells and stromal vascular fraction therapies and different routes of administration (intravenous, intra-renal, and intra-arterial) (17–23). While modestly effective, the clinical applicability of these cell therapies are limited because they are expensive, time consuming, and require advanced cell processing capabilities not available in a general practice setting.
As an alternative, we tested a chemokine (CXCL12), produced by cells to promote tissue regeneration in damaged tissues (24–32). The recombinant human form is commercially available and inexpensive. Injection of recombinant human CXCL12 protein has been shown to reduce fibrosis in rodent models of chronic kidney disease (28), uterine fibrosis (30), stress urinary incontinence (33, 34), and non-human primate models of intrinsic sphincter deficiency (35, 36) and erectile and urinary dysfunction post-radical prostatectomy (37), but its effects on collagen content and collagen fiber histomorphometry have not been tested in cat models of chronic renal fibrosis.
Thus, the goal of this study was to test the effects of the chemokine CXCL12 in chronic renal fibrosis in cats. We first tested the dose-effects of CXCL12 in cats with unilateral ischemia/reperfusion (I/R)-induced interstitial fibrosis (38). Then in a pilot study, we tested the feasibility of ultrasound-guided intra-renal CXCL12 injection in a general practice setting in cats with potential early CKD (Figure 1: Visual Abstract Summary Diagram).
Cats with surgically-produced ischemia/reperfusion (I/R)-induced renal fibrosis were used to test the effects of intra-renal injection of 100, 200, or 400 ng (n = 6/dose) of CXCL12, or saline carrier (n=6), on renal structure in the affected kidney (Figure 2A). Six cats in a control group were anesthetized but uninjured and not injected. They were all fed maintenance diets (Laboratory Feline Diet, LabDiet, St. Louis, MO). Baseline blood/urine renal function tests and ultrasounds were performed at the time of I/R Injury, day 42, day 70 (Treatment injection), and then monthly for 4 months post-injection. At 4 months post-injection, necropsies were performed and tissues harvested for histological evaluation.
Figure 2. (A) Schematic of pre-clinical study design and timeline using 5 groups of cats to test the effects of intra-renal injection of 100, 200, or 400 ng (n = 6/dose) of CXCL12, or sterile saline carrier (n = 6), administered 70 days after I/R-induced renal fibrosis. Six cats in a control group were uninjured and not injected. Baseline blood/urine renal function tests and ultrasounds were performed at the time of I/R Injury, Day 42, Day 70 (Treatment injection), and then monthly for 4 months post-injection. (B) Intraoperative images during I/R injury surgery with vascular clamps occluding left renal artery and left renal vein and purple discoloration of the kidney. Clamps remained in place for 65 min and then were released restoring renal blood flow that was confirmed using a Doppler probe to detect renal artery pulsation.
Two cats were used in a small, separate study exploring early changes in local kidney concentrations of CXCL12, collagenase, and a collagen cross-linking enzyme. Using the Mid dose (200 ng) of CXCL12, one cat with a 5-month I/R injury was necropsied 30 min post-injection. The other cat with a 4-month I/R injury was necropsied 1 month post-injection. Tissues were harvested and flash frozen in cryotubes with liquid nitrogen then stored at −80°C for ELISA assay.
Study design and animal procedures were approved by the Institutional Animal Care and Use Committee (IACUC) at the Wake Forest University School of Medicine. The pre-clinical study used 32 healthy, purpose-bred, 8-month-old female cats acquired from Liberty Research, Inc. (Waverly, NY). Cats were examined by the Wake Forest University Animal Resources Program (ARP) veterinary staff within 3 days of arrival, screened for intestinal parasites, microchipped, and vaccinated with a Rabies vaccine. Cats were vaccinated by the vendor with core vaccines (Feline Rhinotracheitis Virus/Herpesvirus 1, Feline Calicivirus, Feline Panleukopenia) prior to arrival. Cats were quarantined for 3 weeks and deemed healthy by the ARP staff veterinarian prior to release for this study.
A slight modification to the protocol for producing interstitial renal fibrosis described by Schmiedt et al. (38) was used for these studies (the duration of ischemia was increased to 65 min, instead of 60 min, before reperfusion). Cats were fasted and pre-medicated with buprenorphine, ketamine, midazolam, and glycopyrrolate. Intravenous (IV) catheter and endotracheal tube were placed, and Lactated Ringers Solution (LRS) was administered IV. Anesthesia was maintained using isoflurane in oxygen. Famotidine and maropitant citrate were also administered parenterally. Cats were shaved for renal ultrasound and surgically prepared for sterile ventral laparotomy. Both kidneys were exposed and visually inspected, and vasculature of the left kidney was isolated. Vascular clamps were placed across the renal artery first and renal vein second, and purple discoloration of the kidney was observed as visual evidence of hypoxia (Figure 2B). Clamps remained in place for 65 min and then were released restoring renal blood flow that was confirmed using a Doppler probe to detect pulsation of the renal artery. The incision was closed in three layers, cats were recovered in their cages, and appropriate analgesics (e.g., robenacoxib, buprenorphine) were administered as described in the approved IACUC Protocol.
At day 70 after the I/R procedure, cats were fasted and pre-medicated with buprenorphine, ketamine, and midazolam. Anesthesia was maintained using isoflurane in oxygen via mask. LRS was administered subcutaneously. Bilateral flanks were shaved, and renal ultrasound was performed in lateral recumbency for each kidney to assess baseline in vivo kidney size (Acclarix AX8 Portable Ultrasound System, Edan USA, San Diego, CA). The left flank was prepared for aseptic ultrasound-guided intra-renal injection. Using a sterile-covered linear probe (L10-4Q) to obtain a longitudinal view of the left kidney, a single injection was administered into the middle of the left kidney mid-cortex level with a sterile 27-gauge needle to deliver .25ml total volume of sterile saline or saline plus CXCL12 (Recombinant Human SDF-1α (CXCL12), Catalog #300-28A, PeproTech Inc., Rocky Hill, NJ) that was reconstituted and aliquoted sterilely using a 0.45 um filter. All injections were performed by one investigator using ultrasound guidance.
In the separate, small study (n = 2) exploring early changes in local kidney concentrations of CXCL12, collagenase, and a collagen cross-linking enzyme, similar procedures were used to administer a single, intra-renal 200 ng CXCL12 injection at 5 months post-I/R injury into the affected kidney in one cat, and at 4 months post-I/R injury into the affected kidney in the other cat.
Four months after treatment, cats were sedated, heparinized, and euthanized using sodium pentobarbital in accordance with American Veterinary Medical Association Guidelines. One liter of saline was infused into the left cardiac ventricle exiting the lanced caudal vena cava to remove residual blood. Gross pathology was documented and photographed. Both kidneys were removed, weighed, photographed, and measured using calipers with renal capsules intact. Both kidneys were incised transversely into four quarter sections. Two sections were immersed in 4% paraformaldehyde for 72 h then transferred to 70% ethanol prior to paraffin embedding. The remaining two sections were incised transversely into three sections and flash frozen in 3 cryotubes with liquid nitrogen then stored at −80°C. Remaining harvested tissues were immersed in 10% neutral buffered formalin (NBF) for 48 h then transferred to 70% ethanol prior to paraffin embedding. Paraffinized left kidney tissue was sectioned into 5 um slices using a microtome, and histology slides were stained with Masson's Trichrome stain (#HT15, Sigma-Aldrich Inc., St. Louis, MO) and Picrosirius Red (PSR) (#24901, PolySciences, Warrington, PA) for collagen content analyses.
In the small study exploring changes in local kidney concentrations of CXCL12, collagenase, and a collagen cross-linking enzyme, similar procedures were followed except that cats were not heparinized, infusion with one liter of saline was not performed, and necropsy was performed 30 min post-treatment in one cat and 1 month post-treatment in the other cat.
Images of histology sections were taken using the Olympus BX63 microscope (Olympus, Center Valley, PA) with an Olympus DP80 camera (Olympus). Ten randomly selected, evenly spaced images were taken at 200× magnification (for Masson's Trichrome stain) and 100× magnification under the polarized light filter (for PSR stain) of each left kidney renal cortico-medullary junction (CMJ) that included at least one glomerulus but excluded large blood vessels and processing artifacts (e.g., wrinkles, tears). For tissues stained with Masson's Trichrome stain, quantitative analysis of fibrosis was performed using the Olympus cellSens dimension software (v.1.16), Count and Measure application, to calculate Area % of Collagen (Figure 3C). The results of 10 images were averaged for each cat (N = 30) for further analysis. For tissues stained with Picrosirius Red under polarized light (PSR-POL), two collagen fiber analyses were performed: (1) Collagen fiber quantification for length and width using the segmentation software CT-FIRE (Laboratory for Optical and Computation Instrumentation, University of Wisconsin) (39–45) (Figure 4B); and (2) Hue analysis for level of collagen bundling and total collagen quantification using a MATLAB script (R2020a, MathWorks, Natick, MA) (43, 44, 46). Results of 10 images were averaged for each cat (N = 30) for further analysis.
Figure 3. (A) Gross necropsy of left kidney for Control, I/R injured, and CXCL12 treated. I/R resulted in renal fibrosis (white discoloration) that was restored with treatment. (B) Histology of left kidney CMJ stained with Masson's Trichrome under 200× magnification for each study group. I/R (Carrier) increased collagen accumulation, stained blue. CXCL12 Treatment restored I/R-induced changes in collagen in a dose-dependent manner. (C) Masson's Trichrome stain image and cellSens Imaging Software-generated image used to calculate Area % of collagen (yellow). (D) One-way ANOVA for Area % of positive staining for collagen for each group on Masson's Trichrome stain showed a significant group effect, F(4,25) = 8.408, p < 0.01. n = 6/group. Values are individual dot plot and mean ± SD. *p < 0.05.
Figure 4. (A) Histology of left kidney CMJ stained with PSR and PSR-POL under 100× magnification for each study group. I/R (Carrier) increased birefringent collagen fiber signal. CXCL12 Treatment restored I/R-induced changes in birefringent collagen fiber signal in a dose-dependent manner. (B) PSR-POL image used to calculate total collagen in pixels and segmentation software CT-FIRE-generated image used to quantify collagen fiber length and width. (C) One-way ANOVA for collagen fiber width for each group on PSR-POL showed a significant group effect, F(4,25) = 36.634, p < 0.001; and (D) One-way ANOVA for total birefringent collagen signal in pixels for each group on PSR-POL showed a significant group effect, F(4,25) = 15.165, p < 0.001. n = 6/group. Values are individual dot plot and mean ± SD. *p < 0.05; **p < 0.01; ***p < 0.001.
In the small study exploring early changes in local kidney concentrations of CXCL12, collagenase, and a collagen cross-linking enzyme, ELISAs were performed in duplicate using the supernatant collected from 50 mg frozen kidney tissue homogenized in Pierce RIPA Lysis and Extraction Buffer (Catalog #89900, ThermoFisher Scientific, Waltham, MA) to detect and quantify concentrations of human CXCL12 (Human SDF-1α Standard ABTS ELISA Development Kit, Catalog #900-K92, Lot #0717092, PeproTech Inc., Cranbury, NJ), matrix metalloproteinase 1 (MMP-1) (Nori Feline MMP-1 ELISA Kit, Catalog #GR188162, Genorise Scientific, Inc., Glen Mills, PA), and lysyl oxidase-like enzyme 2 (LOXL-2) (Human LOXL-2 ELISA Kit, Catalog #ab213808, Abcam, Cambridge, MA).
Blood and urine samples were submitted to IDEXX Laboratories for complete blood count, serum biochemical analysis, including renal biomarker symmetric dimethylarginine (SDMA) (47–50), and urinalysis.
The effect of treatment on total collagen and collagen fiber width was evaluated for each group using a one-way ANOVA for approximately normally distributed data. Differences between groups were considered statistically significant at p < 0.05. Bonferroni-corrected post-hoc-tests were used to evaluate multiple comparisons. Results are shown as Mean ± SD. The following assumptions were checked for a one-way ANOVA: no significant outliers on visualization of boxplots, normality using the Shapiro-Wilk test for normality, and homogeneity of variances using Levene's-test of equality of variances. Winsorization was used to retain any extreme outliers. For violations of normality on Shapiro-Wilk, z-scores for skewness and kurtosis were checked to be within ± 2.58 standard deviations. All statistical analyses were performed using IBM SPSS Statistics (IBM Corp., Armonk, NY).
Given the small sample size of the study exploring early changes in local kidney concentrations of CXCL12, collagenase, and a collagen cross-linking enzyme, only descriptive summary statistics were performed.
The pilot study was done in collaboration with veterinarians at the PDHB Joint Veterinary Practice in Indonesia. Fourteen geriatric, client-owned cats with potential early kidney disease, based on physical exam, history of weight loss, polyuria/polydipsia with urine specific (USG) gravity <1.035, were divided into Control (no injection) vs. Treatment (n = 7/group). The inclusion criteria included: (1) Age 7 years or older; (2) Negative Feline Leukemia Virus (FeLV) and Feline Immunodeficiency Virus (FIV) test; and (3) Overall good health and mobility. Cats who met these inclusion criteria were placed in the Treatment group early in the study and, as such, were not randomized. Exclusion criteria were the presence or history of: (1) Polycystic kidney disease; (2) Urolithiasis (renal and ureteral); and (3) Respiratory disease requiring treatment (in Indonesia, feline upper respiratory infections are oftentimes highly virulent, pathogenic, and debilitating). All cats were treated with selamectin and praziquantel, and significant comorbidities (e.g., flea allergy dermatitis and stomatitis) were treated and resolved prior to treatment injections. Two cats in the Control group (Cats 5 and 6) were previously diagnosed with cardiomegaly (suspected hypertrophic cardiomyopathy) and considered stable with diltiazem and amlodipine. Baseline blood/urine renal function tests and ultrasounds were performed prior to injections and repeated monthly (blood/urine) and quarterly (renal ultrasound) for 12 months. All cats were provided with Royal Canin Renal Special prescription diet, and two Treatment group cats (Cats 1 and 2), residents of the clinic, were fed the prescription diet exclusively with no other therapies. All other cats were permitted to receive subcutaneous fluid therapy and amlodipine, if needed. Anti-proteinuric treatment, such as benazepril, is not readily available in Indonesia; human formulations of telmisartan and enalapril are available but were not needed. Although some cats were indoor only, it is common for cats in Indonesia to roam freely indoor and outdoor. Owners of the participating cats were thoroughly informed of the scope, objectives, expectations, and potential risks and benefits of the procedures to be performed in this pilot study. All clients were willing participants, signed consent forms, and were not blinded to treatment group.
Before injections, Treatment group cats were fasted and pre-medicated with diazepam IV. IV catheters were placed, and LRS was administered IV. Propofol was used for anesthesia induction, and anesthetic plane was maintained using isoflurane in oxygen via mask or endotracheal tube. Bilateral flanks were shaved, and renal ultrasound was performed in lateral recumbency for each kidney. Each flank was prepared for aseptic ultrasound-guided intra-renal injection. Using a sterile-covered convex probe to obtain a longitudinal view of the kidney, a single injection was administered into the middle of each kidney mid-cortex level using a sterile 27-gauge needle to deliver 0.25 ml of sterile saline plus 200 ng CXCL12 (Recombinant Human SDF-1α (CXCL12), Catalog #300-28A, PeproTech Inc., Rocky Hill, NJ) that was reconstituted and aliquoted sterilely using a 0.45 um filter. All treatment injections were performed by the same two investigators who were trained in the technique used in the I/R study.
Blood and urine samples for complete blood count, serum biochemical analysis, and urinalysis were submitted to the Animal Laboratory Sunter, Indonesia for evaluation. Blood samples were also submitted to the Microbiology and Immunology Laboratory, Primate Research Center, Institut Pertanian Bogor, Bogor Agricultural University, Indonesia for SDMA (symmetric dimethylarginine) evaluation using a canine/feline SDMA ELISA kit in duplicate (#TRM-594, Biovet, Inc., St-Hyacinthe, QC, Canada). SDMA was measured over the length of the experiment but not used to determine CKD Stage. For the purpose of this study, CKD Stage was defined as follows: 1) Stage 1: urine specific gravity (USG) <1.035 and serum Creatinine <1.6 mg/dL; 2) Stage 2: USG <1.035 and serum Creatinine 1.6–2.8 mg/dL; and 3) Stage 3: USG <1.035 and serum Creatinine 2.9–5 mg/dL. A theoretical Stage 0 was formulated to denote USG 1.035 or higher and serum Creatinine <1.6 mg/dL.
Given the small sample size and missing data points, only descriptive and summary statistics were calculated, to include median and range.
I/R resulted in renal fibrosis, tubulointerstitial collagen accumulation, and widening of collagen fibers in the affected kidney. I/R significantly increased collagen content (p < 0.05 vs. Control) and collagen fiber width (p < 0.001 vs. Control) in the affected kidney. Injection of recombinant CXCL12 treatment attenuated these injury-induced changes in collagen (Figure 3A: Gross necropsy) in a dose-dependent manner (Figure 3B: Masson's Trichrome stain and Figure 4A: PSR-POL). Both the Mid and High doses of CXCL12 restored kidney collagen in I/R kidneys (p < 0.05 vs. untreated) as measured with Masson's Trichrome stained tissue sections (Figure 3D). This treatment effect on kidney collagen was also validated on Picrosirius Red under polarized light (p < 0.01 vs. untreated) (Figure 4C). Furthermore, CXCL12 treatment also restored (reduced) collagen fiber width (p < 0.001 vs. untreated) (Figure 4D).
Analysis of renal function clinical pathology endpoints over time detected no significant differences among groups. Due to the unilateral injury of our model (and subsequent compensation by the uninjured kidney), no significant differences in renal function were detected among the groups (Figures 5A–F). Changes in body weight and urine specific gravity (USG) for all groups were measured by calculating Average % change from Baseline evaluated at 1, 2, 3, and 4 months post-Treatment injection (Figures 5G,H), using Day 70 post-injury as Baseline (maximum injury), with no significant differences among groups. Supplementary Tables with raw data are also provided in the Pre-clinical Supplementary Material section.
Figure 5. Pre-clinical study clinical pathology results of renal function over time (Baseline, Day 70, Month 1, Month 2, Month 3, Month 4) for each group depicted as dot plot and mean ± SD showing: USG (A), serum creatinine (B), body weight (C), SDMA (D), serum BUN (E), and serum phosphorus (F). Average % change in body weight (kg) (G) and USG (H) over time (Month 1, Month 2, Month 3, Month 4) for each group when compared to day 70 post-injury (i.e., maximum injury), just prior to treatment injection. n = 6/group. Values are mean ± SD.
Ultrasound-guided intra-renal injection of chemokine therapy was safe and feasible in this model. The procedure duration for bilateral renal ultrasound and unilateral intra-renal injection was <1 h. No adverse reactions (e.g., anesthetic complication, infection, immune reaction) were observed during or after the procedure. Route of administration was minimally invasive and required general practitioner-level training and equipment.
Early changes in kidney concentrations of MMP-1, associated with collagen breakdown, and LOXL-2 concentrations, associated with collagen cross-linking, were measured in the I/R-injured kidneys in response to CXCL12 Treatment (Supplementary Figure 1 in Pre-clinical Supplementary Material section).
Descriptive statistics of renal function clinical pathology endpoints are provided in Supplementary Table 1 in the Pilot Supplementary Material section. Prior to treatment, the Control group included 6 Stage 2 and 1 Stage 1 cats, and the Treatment group 5 Stage 2 and 2 Stage 1 cats. At the pilot study end, 3 Stage 2 cats and 1 Stage 1 cat in the Control group increased in disease stage (Worse), and 3 Stage 2 cats decreased (Improved) (Table 1). In the Treatment group, 3 Stage 2 cats decreased in disease stage (Improved) at the pilot study end, and 2 Stage 2 cats and 2 Stage 1 cats maintained the same stage (No Change) (Table 1). None of the treated cats advanced to a higher stage of disease at the end of the pilot study. The sum of the changes in CKD stage compared to baseline was calculated monthly for each group (Supplementary Figure 1A, Pilot Supplementary Material).
Changes in urine specific gravity, serum creatinine, and SDMA with CXCL12 treatment were measured. Number of cats able to concentrate urine to 1.035 or higher is shown in Supplementary Figure 1B, Pilot Supplementary Material, with n specified for each group, Control (C) and Treatment (T), at each time point. Number of cats with creatinine <1.6 mg/dl (Supplementary Figure 1C, Pilot Supplementary Material) and % change in serum creatinine when compared to baseline (Supplemental Figure 1D, Pilot Supplementary Material) were calculated for both groups. Number of cats with SDMA 14 ug/dl or less (Supplementary Figure 1E, Pilot Supplementary Material) and % change in SDMA when compared to baseline (Supplementary Figure 1F, Pilot Supplementary Material) were calculated for both groups. Supplementary Tables with raw data are also provided in the Pilot Supplementary Material section.
We were unable to perform statistical analyses with significance due to missing data points resulting from the inherent challenges of collecting urine samples from client-owned cats and ending the pilot study 3 months early due to inconsistent adherence to study parameters. One owner from the Control group cats moved to a different country after 6 months. One cat from each group also developed severe respiratory symptoms requiring treatment near the end of the study, and subsequent data points were excluded.
The major findings of this study were: (1) Injection of recombinant CXCL12 reduced the formation of collagen and reduced collagen fiber width in a pre-clinical cat model of I/R-induced tubulointerstitial fibrosis; and (2) Kidney injection of recombinant CXCL12 was safe and feasible as local treatment in cats with early stages of CKD.
Tubulointerstitial fibrosis is the underling tissue pathology that best correlates with declining renal function (10, 11, 16, 51). Therefore, the main goal of this study was to utilize a treatment that would reduce the amount of interstitial fibrosis and then test feasibility of this treatment in a pilot study. We chose intra-renal injection of recombinant CXCL12 as our treatment. CXC motif chemokine 12 (CXCL12) is expressed in many tissues and cells. It is released mainly by mesenchymal stem cells and damaged tissues in the regenerative microenvironment (52, 53). Through a receptor (CXCR4) mechanism, CXCL12 has a major role in cell trafficking and homing of progenitor cells to sites of injury and in enhancing cell survival once at the injury site. There are numerous reports of this regenerative capacity in various tissues (28, 30, 33–37). We have previously used CXCL12 as a local treatment to stimulate regeneration of urogenital tissues (34, 36, 37).
There are published studies that seem to contradict our results and dispute the beneficial effects of CXCL12 on kidney regeneration (54), renal function and fibrosis (55, 56), and collagen synthesis in different tissues and organs. These studies report inflammatory effects of serum CXCL12 in various disease states (57, 58), to include malignancy (59), and that the CXCL12/CXCR4 mechanism may be directly involved in renal carcinoma (59). Additionally, other in vitro cell culture studies report CXCL12/CXCR4 axis-mediated kidney pathology and activation of collagen synthesis (56, 60, 61). However, these studies focus on the secretion of physiologic CXCL12 that Ray et al. showed to form dimers in physiologic conditions and high concentrations that more efficiently activate pathways leading to pathology and malignancy and inhibit chemotaxis (32, 62, 63). Recombinant CXCL12, specifically purified monomeric CXCL12 (32, 62, 64), has demonstrated its protective effects against myocardial infarction during ischemia/reperfusion injury (27, 31, 32, 65–69). Veldkamp et al. also suggested that low concentrations of monomeric CXCL12 stimulates chemotaxis (63) and is the active form for chemotaxis that confers cardioprotection during I/R myocardial infarction (32). Monomeric CXCL12 is secreted by both mammalian cells and bacteria (62) and commercially available in recombinant, purified form, as used in our treatment injections.
Because of the high risk of coagulopathy and mortality described in the bilateral I/R model (38, 70), we chose to replicate the 60-min unilateral renal I/R model, with a minor increase to 65 min. Since only one kidney was damaged, minimal changes could be detected in renal function clinical pathology endpoints (p > 0.05) (Figure 5) due to compensation by the untouched contralateral kidney. More recently, a 90-min unilateral renal I/R model has been described (71) as well as other protocols using various combinations of I/R and delayed contralateral nephrectomy (72) that may better detect significant changes in renal function in blood and urine samples for future pre-clinical models. Interestingly, elevation of the renal biomarker SDMA >14 ug/dL was observed in 11/30 cats at baseline (with 4/30 cats having SDMA ≥18 ug/dL at baseline) and fluctuated regardless of treatment group, and 3/6 cats in the uninjured Control group had SDMA >14 at the end of the study (Pre-clinical Supplementary Table 4). Recently, Brans et al. suggested that SDMA readings using the cut off 18 ug/dL rather than 14 ug/dL may be a more appropriate indicator of early kidney disease that could avoid false positives (50). We also measured changes in body weight (Figure 5G) and USG (Figure 5H) for all groups when compared to Day 70 post-injury (i.e., maximum injury), just prior to Treatment injection, but there was no significant difference among groups.
We focused our evaluation on the quantitative analysis of treatment effects on fibrosis in the injured kidney cortico-medullary junction (CMJ), where lesions should be most severe (38). Our I/R model significantly increased tubulointerstitial collagen content in the injured renal CMJ as quantified on Masson's Trichrome stain and validated with PSR-POL. Both the Mid and High doses of CXCL12 injected 70 days post-injury significantly decreased tubulointerstitial collagen content using kidney tissues stained with both Masson's Trichrome (p < 0.05 vs. untreated) and PSR-POL (p < 0.01 vs. untreated) 4 months post-injection. Although statistical results of pairwise comparisons were slightly different between Masson's Trichrome stain and PSR-POL, the overall CXCL12 Mid and High dose treatment effects on fibrosis were consistent despite using the two different quantification methods and analyses for collagen.
Normal tissue homeostasis is maintained through a delicate balance of collagen production, deposition, and degradation in the extracellular matrix (ECM) (73). Irreversible fibrosis results when the tissue microenvironment favors ECM production and deposition over degradation and remodeling. Analysis of collagen fiber parameters, such as length, width, angularity, and straightness, in normal and pathologic disease states may help unlock the conditions that favor irreversible fibrosis (40, 43, 44, 46), and this method of evaluating collagen has been validated by Caetano et al. as comparable to the gold standard hydroxyproline assay (45). Increased collagen fiber width in the tumor microenvironment is a powerful negative prognostic indicator associated with reduced 5-year survival that was validated in two separate gastric cancer cohorts of 225 and 151 cancer patients (42). Our I/R model significantly increased collagen fiber width in the injured renal CMJ as analyzed with PSR-POL. Both the Mid and High doses of recombinant CXCL12 injected 70 days post-injury significantly decreased collagen fiber width (p < 0.001 vs. untreated) at 4 months post-injection. Incorporating segmentation software-generated analyses of collagen fiber histomorphometry, such as CT-FIRE, and in particular, evaluating the collagen fiber parameter of width, could more accurately depict which microenvironments may favor irreversible fibrosis over collagen degradation and remodeling that may impact prognosis.
A proposed mechanism for the observed attenuation in I/R-induced renal fibrosis and collagen fiber widening may be early, short-term CXCL12 treatment effects on collagenases and collagen cross-linking enzymes as suggested by our small study findings. MMP-1 is a collagenase that degrades fibrillar collagen and disrupts its 3-dimensional triple-helical structure to increase exposure and susceptibility to further degradation by other proteases (74). CXCL12 stimulation has been shown to have early, dose-dependent increases in MMP-1 production (75–77), and CXCL12 signaling and crosstalk with MMP-1 both stimulate stem cell migration (78). Early changes (increases) in MMP-1 concentrations post-CXCL12 injection may play a role in the attenuation of renal fibrosis with CXCL12 treatment, but our small sample size (n = 1) precludes us from making this conclusion. LOXL-2 crosslinks collagens and elastin resulting in increased ECM stiffness (79) and has been associated with renal fibrosis and pathology (80, 81). ECM scaffold treatment with anti-LOXL2 antibodies demonstrated a decrease in average collagen fiber width (82). We suspect that early changes (decreases) in LOXL-2 concentrations post-CXCL12 injection may also play a role in re-establishing a more normal ECM environment favoring collagen fibers of normal width that are potentially more susceptible to remodeling. However, the small sample size of our study evaluating early changes in MMP-1 and LOXL-2 concentrations (n = 1/early time point) is a major limitation. More studies that include local concentrations measured at baseline (just prior to treatment injection), more time points, and larger sample sizes are needed to investigate these and other possible mechanisms with statistical significance.
In the pilot study, recombinant CXCL12 treatment for cats with early CKD was safe and feasible in a general practice setting, and we measured changes in kidney disease stage progression, USG, serum creatinine, and SDMA in both groups. At the study end, there were no treated cats that advanced to a higher stage of CKD. Most cats who successfully met our inclusion criteria and requirements were placed into the Treatment group early in the study, thus, were not randomized. All cats who were enrolled in the study were treated for any pre-existing conditions. The 2 cats in the Control group with suspected hypertrophic cardiomyopathy (Cats 5 and 6) were considered stable with their instituted therapy. SDMA ELISA kits were used as an alternative to the proprietary IDEXX Laboratory assay due to geographic inaccessibility in Indonesia. Despite the aforementioned limitations, small sample size without randomization, and missing data points, our study supports future studies to evaluate the potential use and efficacy of CXCL12 therapy in a clinical setting.
All cats in both pilot study groups were fed dry Royal Canin Renal Special prescription diet at the beginning and throughout the duration of the study, but similar to what veterinarians experience in other countries, we cannot guarantee that was the only food the cats ate, with the exception of 2 clinic cats in the Treatment Group (Cats 1 and 2). These 2 cats received no other standard of care treatment other than prescription renal food, similar to some cat owners who are unable to medicate their cats. Although the consumption of prescription renal food has been shown to slow the increase in serum creatinine and help maintain lean muscle mass, no effects on urine concentration ability or SDMA have been reported (83).
The combined data from our pre-clinical and pilot studies suggest that intra-renal injection of recombinant CXCL12 may be a novel treatment for chronic renal fibrosis in cats with the potential for future use in other veterinary species and translational applications in humans (Figure 1). However, more controlled studies with larger sample sizes and randomization will be needed to evaluate treatment efficacy with statistical significance in a clinical setting. The ideal candidates for CXCL12 therapy may be in the early stages of kidney disease, which can have subtle or no clinical signs, therefore, elevating the value of routine wellness screening and early disease detection. Further studies are needed to identify CXCL12 formulations that may increase the efficacy of this therapy to treat more advanced kidney disease and to fully elucidate the mechanism, tissue and systemic dispersion, and duration of effect.
The original contributions generated in the study are included in the article/Supplementary Material, further inquiries can be directed to the corresponding author.
The animal study was reviewed and approved by Institutional Animal Care and Use Committee (IACUC) at the Wake Forest University School of Medicine. Written informed consent was obtained from the owners for the participation of their animals in this study.
JB, SL, RM, DS, CK, IS, WK, MP, SM, GB, and JW contributed to the conception and design of the study. JB, SL, RM, DS, JF, CK, IS, WK, MP, SM, and JW contributed to the data acquisition, analysis, and interpretation of the work. JB and JF performed the statistical analyses. JB and JW wrote the first draft of the manuscript, and all authors contributed to revisions, read and approved the submitted version, and agreed to be accountable for the work. All authors contributed to the article and approved the submitted version.
This work was funded by Piedmont Animal Health, Greensboro, NC. JB was supported by NIH Post-doctoral T32 Institutional Training Grant Laboratory Animal and Comparative Medicine Research (NIH T32 OD010957).
JW is an inventor on patent rights related to this work owned by Wake Forest University Health Sciences. The patents, whose value may be affected by publication, have the potential to generate royalty income in which the inventors would share.
The remaining authors declare that the research was conducted in the absence of any commercial or financial relationships that could be construed as a potential conflict of interest.
We express gratitude to the families in Indonesia whose beloved cats participated in our pilot study. We also thank our colleagues in Indonesia: Dr. Erni Sulistiawati, Director of the Animal Laboratory Sunter, for analyzing clinical pathology samples; Dr. Kevin Tan from the PDHB Joint Veterinary Clinic, for conducting renal ultrasounds and treatment injections; and Rachmitasari Noviana from the Primate Research Center, Institut Pertanian Bogor, for conducting SDMA ELISA tests. We also thank Dr. Mahesh Devarasetty and Anthony Dominijanni from the Wake Forest Institute for Regenerative Medicine for providing valuable guidance on using the CT-FIRE software and MATLAB scripts for the PSR-POL collagen fiber analyses.
The Supplementary Material for this article can be found online at: https://www.frontiersin.org/articles/10.3389/fvets.2021.646087/full#supplementary-material
1. DiBartola SP, Rutgers HC, Zack PM, Tarr MJ. Clinicopathologic findings associated with chronic renal disease in cats: 74 cases (1973-1984). J Am Vet Med Assoc. (1987) 190:1196–202.
2. Elliott J, Barber PJ. Feline chronic renal failure: clinical findings in 80 cases diagnosed between 1992 and 1995. J Small Anim Pract. (1998) 39:78–85. doi: 10.1111/j.1748-5827.1998.tb03598.x
3. Marino CL, Lascelles BDX, Vaden SL, Gruen ME, Marks SL. Prevalence and classification of chronic kidney disease in cats randomly selected from four age groups and in cats recruited for degenerative joint disease studies. J Feline Med Surg. (2014) 16:465–72. doi: 10.1177/1098612X13511446
4. Lulich JP. Feline renal failure, questions, answers, questions. Comp Cont Educ Pract Vet. (1992) 14:127–53.
5. Brown CA, Elliott J, Schmiedt CW, Brown SA. Chronic kidney disease in aged cats: clinical features, morphology, and proposed pathogeneses. Vet Pathol. (2016) 53:309–26. doi: 10.1177/0300985815622975
6. Maeshima A, Nakasatomi M, Nojima Y. Regenerative medicine for the kidney: renotropic factors, renal stem/progenitor cells, and stem cell therapy. BioMed Res Int. (2014) 2014:595493. doi: 10.1155/2014/595493
7. Lawson JS, Liu HH, Syme HM, Purcell R, Wheeler-Jones CPD, Elliott J. The cat as a naturally occurring model of renal interstitial fibrosis: characterisation of primary feline proximal tubular epithelial cells and comparative pro-fibrotic effects of TGF-beta1. PLoS ONE. (2018) 13:e0202577. doi: 10.1371/journal.pone.0202577
8. Farris AB, Colvin RB. Renal interstitial fibrosis: mechanisms and evaluation. Curr Opin Nephrol Hypertens. (2012) 21:289–300. doi: 10.1097/MNH.0b013e3283521cfa
9. Chakrabarti S, Syme HM, Brown CA, Elliott J. Histomorphometry of feline chronic kidney disease and correlation with markers of renal dysfunction. Vet Pathol. (2013) 50:147–55. doi: 10.1177/0300985812453176
10. McLeland SM, Cianciolo RE, Duncan CG, Quimby JM. A comparison of biochemical and histopathologic staging in cats with chronic kidney disease. Vet Pathol. (2014) 52:524–34. doi: 10.1177/0300985814561095
11. Lawson J, Elliott J, Wheeler-Jones C, Syme H, Jepson R. Renal fibrosis in feline chronic kidney disease: known mediators and mechanisms of injury. Vet J (London, England: 1997). (2015) 203:18–26. doi: 10.1016/j.tvjl.2014.10.009
12. Prunotto M, Ghiggeri G, Bruschi M, Gabbiani G, Lescuyer P, Hocher B, et al. Renal fibrosis and proteomics: current knowledge and still key open questions for proteomic investigation. J Proteomics. (2011) 74:1855–70. doi: 10.1016/j.jprot.2011.05.031
13. Nangaku M. Chronic hypoxia and tubulointerstitial injury: a final common pathway to end-stage renal failure. J Am Soc Nephrol. (2006) 17:17–25. doi: 10.1681/ASN.2005070757
14. Djudjaj S, Boor P. Cellular and molecular mechanisms of kidney fibrosis. Mol Aspects Med. (2019) 65:16–36. doi: 10.1016/j.mam.2018.06.002
15. Liu B-C, Tang T-T, Lv L-L, Lan H-Y. Renal tubule injury: a driving force toward chronic kidney disease. Kidney Int. (2018) 93:568–79. doi: 10.1016/j.kint.2017.09.033
16. Nath KA. Tubulointerstitial changes as a major determinant in the progression of renal damage. Am J Kidney Dis. (1992) 20:1–17. doi: 10.1016/S0272-6386(12)80312-X
17. Quimby JM, Webb TL, Randall E, Marolf A, Valdes-Martinez A, Dow SW. Assessment of intravenous adipose-derived allogeneic mesenchymal stem cells for the treatment of feline chronic kidney disease: a randomized, placebo-controlled clinical trial in eight cats. J Feline Med Surg. (2016) 18:165–71. doi: 10.1177/1098612X15576980
18. Rosselli DD, Mumaw JL, Dickerson V, Brown CA, Brown SA, Schmiedt CW. Efficacy of allogeneic mesenchymal stem cell administration in a model of acute ischemic kidney injury in cats. Res Vet Sci. (2016) 108:18–24. doi: 10.1016/j.rvsc.2016.07.003
19. Quimby JM, Webb TL, Gibbons DS, Dow SW. Evaluation of intrarenal mesenchymal stem cell injection for treatment of chronic kidney disease in cats: a pilot study. J Feline Med Surg. (2011) 13:418–26. doi: 10.1016/j.jfms.2011.01.005
20. Webb TL, Quimby JM, Dow SW. In vitro comparison of feline bone marrow-derived and adipose tissue-derived mesenchymal stem cells. J Feline Med Surg. (2012) 14:165–8. doi: 10.1177/1098612X11429224
21. Quimby JM, Borjesson DL. Mesenchymal stem cell therapy in cats: current knowledge and future potential. J Feline Med Surg. (2018) 20:208–16. doi: 10.1177/1098612X18758590
22. Quimby JM, Dow SW. Novel treatment strategies for feline chronic kidney disease: a critical look at the potential of mesenchymal stem cell therapy. Vet J (London, England: 1997). (2015) 204:241–6. doi: 10.1016/j.tvjl.2015.04.007
23. Thomson AL, Berent AC, Weisse C, Langston CE. Intra-arterial renal infusion of autologous mesenchymal stem cells for treatment of chronic kidney disease in cats: phase I clinical trial. J Vet Int Med. (2019) 33:1353–61. doi: 10.1111/jvim.15486
24. Sun Z, Li X, Zheng X, Cao P, Yu B, Wang W. Stromal cell-derived factor-1/CXC chemokine receptor 4 axis in injury repair and renal transplantation. J Int Med Res. (2019) 47:5426–40. doi: 10.1177/0300060519876138
25. Takabatake Y, Sugiyama T, Kohara H, Matsusaka T, Kurihara H, Koni PA, et al. The CXCL12 (SDF-1)/CXCR4 axis is essential for the development of renal vasculature. J Am Soc Nephrol. (2009) 20:1714–23. doi: 10.1681/ASN.2008060640
26. Janssens R, Struyf S, Proost P. The unique structural and functional features of CXCL12. Cell Mol Immunol. (2018) 15:299–311. doi: 10.1038/cmi.2017.107
27. Bromage DI, Taferner S, He Z, Ziff OJ, Yellon DM, Davidson SM. Stromal cell-derived factor-1α signals via the endothelium to protect the heart against ischaemia-reperfusion injury. J Mol Cell Cardiol. (2019) 128:187–97. doi: 10.1016/j.yjmcc.2019.02.002
28. Chen L-H, Advani SL, Thai K, Kabir MG, Sood MM, Gibson IW, et al. SDF-1/CXCR4 signaling preserves microvascular integrity and renal function in chronic kidney disease. PLoS ONE. (2014) 9:e92227. doi: 10.1371/journal.pone.0092227
29. Ohnishi H, Mizuno S, Mizuno-Horikawa Y, Kato T. Stromal cell-derived factor-1 (SDF1)-dependent recruitment of bone marrow-derived renal endothelium-like cells in a mouse model of acute kidney injury. J Vet Med Sci. (2015) 77:313–9. doi: 10.1292/jvms.14-0562
30. Ersoy SG, Zolbin MM, Cosar E, Moridi I, Mamillapalli R, Taylor HS. CXCL12 promotes stem cell recruitment and uterine repair after injury in Asherman's syndrome. Mol Ther Methods Clin Dev. (2017) 4:169–77. doi: 10.1016/j.omtm.2017.01.001
31. Bogoslovsky T, Spatz M, Chaudhry A, Maric D, Luby M, Frank J, et al. Stromal-derived factor-1[alpha] correlates with circulating endothelial progenitor cells and with acute lesion volume in stroke patients. Stroke. (2011) 42:618–25. doi: 10.1161/STROKEAHA.110.596007
32. Veldkamp CT, Ziarek JJ, Su J, Basnet H, Lennertz R, Weiner JJ, et al. Monomeric structure of the cardioprotective chemokine SDF-1/CXCL12. Protein Sci. (2009) 18:1359–69. doi: 10.1002/pro.167
33. Khalifa AO, Kavran M, Mahran A, Isali I, Woda J, Flask CA, et al. Stromal derived factor-1 plasmid as a novel injection for treatment of stress urinary incontinence in a rat model. Int Urogynecol J. (2020) 31:107–15. doi: 10.1007/s00192-019-03867-3
34. Williams JK, Dean A, Lankford S, Andersson KE. Efficacy and initial safety profile of CXCL12 treatment in a rodent model of urinary sphincter deficiency. Stem Cells Transl Med. (2017) 6:1740–6. doi: 10.1002/sctm.16-0497
35. Williams JK, Dean A, Badra S, Lankford S, Poppante K, Badlani G, et al. Cell versus chemokine therapy in a nonhuman primate model of chronic intrinsic urinary sphincter deficiency. J Urol. (2016) 196:1809–15. doi: 10.1016/j.juro.2016.05.106
36. Williams JK, Mariya S, Suparto I, Lankford SS, Andersson KE. Cell versus chemokine therapy effects on cell mobilization to chronically dysfunctional urinary sphincters of nonhuman primates. Int Neurourol J. (2018) 22:260–7. doi: 10.5213/inj.1836126.063
37. Zambon JP, Patel M, Hemal A, Badlani G, Andersson KE, Magalhaes RS, et al. Nonhuman primate model of persistent erectile and urinary dysfunction following radical prostatectomy: feasibility of minimally invasive therapy. Neurourol Urodyn. (2018) 37:2141–50. doi: 10.1002/nau.23536
38. Schmiedt CW, Brainard BM, Hinson W, Brown SA, Brown CA. Unilateral renal ischemia as a model of acute kidney injury and renal fibrosis in cats. Vet Pathol. (2016) 53:87–101. doi: 10.1177/0300985815600500
39. Bredfeldt J, Liu Y, Pehlke C, Conklin M, Szulczewski J, Inman D, et al. Computational segmentation of collagen fibers from second-harmonic generation images of breast cancer. J Biomed Optics. (2014) 19:016007. doi: 10.1117/1.JBO.19.1.016007
40. Drifka CR, Loeffler AG, Mathewson K, Mehta G, Keikhosravi A, Liu Y, et al. Comparison of picrosirius red staining with second harmonic generation imaging for the quantification of clinically relevant collagen fiber features in histopathology samples. J Histochem Cytochem. (2016) 64:519–29. doi: 10.1369/0022155416659249
41. Pointer KB, Clark PA, Schroeder AB, Salamat MS, Eliceiri KW, Kuo JS. Association of collagen architecture with glioblastoma patient survival. J Neurosurg. (2017) 126:1812–21. doi: 10.3171/2016.6.JNS152797
42. Zhou Z-H, Ji C-D, Xiao H-L, Zhao H-B, Cui Y-H, Bian X-W. Reorganized collagen in the tumor microenvironment of gastric cancer and its association with prognosis. J Cancer. (2017) 8:1466–76. doi: 10.7150/jca.18466
43. Devarasetty M, Dominijanni A, Herberg S, Shelkey E, Skardal A, Soker S. Simulating the human tumor microenvironment in colorectal cancer organoids in vitro and in vivo. bioRxiv [Preprint]. (2019). doi: 10.1101/822684
44. Devarasetty M, Dominijanni A, Herberg S, Shelkey E, Skardal A, Soker S. Simulating the human colorectal cancer microenvironment in 3D tumor-stroma co-cultures in vitro and in vivo. Sci Rep. (2020) 10:9832. doi: 10.1038/s41598-020-66785-1
45. Caetano GF, Fronza M, Leite MN, Gomes A, Frade MAC. Comparison of collagen content in skin wounds evaluated by biochemical assay and by computer-aided histomorphometric analysis. Pharm Biol. (2016) 54:2555–9. doi: 10.3109/13880209.2016.1170861
46. Brovold M, Keller D, Soker S. Differential fibrotic phenotypes of hepatic stellate cells within 3D liver organoids. Biotechnol Bioeng. (2020) 117:2516–26. doi: 10.1002/bit.27379
47. Bode-Böger SM, Scalera F, Kielstein JT, Martens-Lobenhoffer J, Breithardt G, Fobker M, et al. Symmetrical dimethylarginine: a new combined parameter for renal function and extent of coronary artery disease. J Am Soc Nephrol. (2006) 17:1128–34. doi: 10.1681/ASN.2005101119
48. Hall JA, Yerramilli M, Obare E, Yerramilli M, Jewell DE. Comparison of serum concentrations of symmetric dimethylarginine and creatinine as kidney function biomarkers in cats with chronic kidney disease. J Vet Int Med. (2014) 28:1676–83. doi: 10.1111/jvim.12445
49. Ernst R, Ogeer J, McCrann D, Cross J, Strong-Townsend M, Friis H, et al. Comparative performance of IDEXX SDMA Test and the DLD SDMA ELISA for the measurement of SDMA in canine and feline serum. PLoS ONE. (2018) 13:e0205030. doi: 10.1371/journal.pone.0205030
50. Brans M, Daminet S, Mortier F, Duchateau L, Lefebvre HP, Paepe D. Plasma symmetric dimethylarginine and creatinine concentrations and glomerular filtration rate in cats with normal and decreased renal function. J Vet Int Med. (2021) 35:303–11. doi: 10.1111/jvim.15975
51. Chakrabarti S, Syme HM, Elliott J. Clinicopathological variables predicting progression of azotemia in cats with chronic kidney disease. J Vet Int Med. (2012) 26:275–81. doi: 10.1111/j.1939-1676.2011.00874.x
52. Lau TT, Wang DA. Stromal cell-derived factor-1 (SDF-1): homing factor for engineered regenerative medicine. Expert Opin Biol Ther. (2011) 11:189–97. doi: 10.1517/14712598.2011.546338
53. Zisa D, Shabbir A, Mastri M, Taylor T, Aleksic I, McDaniel M, et al. Intramuscular VEGF activates an SDF1-dependent progenitor cell cascade and an SDF1-independent muscle paracrine cascade for cardiac repair. Am J Physiol Heart Circ Physiol. (2011) 301:H2422–32. doi: 10.1152/ajpheart.00343.2011
54. Romoli S, Angelotti ML, Antonelli G, Kumar Vr S, Mulay SR, Desai J, et al. CXCL12 blockade preferentially regenerates lost podocytes in cortical nephrons by targeting an intrinsic podocyte-progenitor feedback mechanism. Kidney Int. (2018) 94:1111–26. doi: 10.1016/j.kint.2018.08.013
55. Mo H, Wu Q, Miao J, Luo C, Hong X, Wang Y, et al. C-X-C chemokine receptor type 4 plays a crucial role in mediating oxidative stress-induced podocyte injury. Antioxid Redox Signal. (2017) 27:345–62. doi: 10.1089/ars.2016.6758
56. Liu Y, Feng Q, Miao J, Wu Q, Zhou S, Shen W, et al. C-X-C motif chemokine receptor 4 aggravates renal fibrosis through activating JAK/STAT/GSK3β/β-catenin pathway. J Cell Mol Med. (2020) 24:3837–55. doi: 10.1111/jcmm.14973
57. Klimczak-Tomaniak D, Pilecki T, Zochowska D, Sieńko D, Janiszewski M, Paczek L, et al. CXCL12 in patients with chronic kidney disease and healthy controls: relationships to ambulatory 24-hour blood pressure and echocardiographic measures. Cardiorenal Med. (2018) 8:249–58. doi: 10.1159/000490396
58. Gao J-H, Yu X-H, Tang C-K. CXC chemokine ligand 12 (CXCL12) in atherosclerosis: an underlying therapeutic target. Clinica Chimica Acta. (2019) 495:538–44. doi: 10.1016/j.cca.2019.05.022
59. Floranović MP, Veličković LJ. Effect of CXCL12 and its receptors on unpredictable renal cell carcinoma. Clin Genitourin Cancer. (2020) 18:e337–42. doi: 10.1016/j.clgc.2019.11.004
60. Patalano S, Rodríguez-Nieves J, Colaneri C, Cotellessa J, Almanza D, Zhilin-Roth A, et al. CXCL12/CXCR4-mediated procollagen secretion is coupled to cullin-RING ubiquitin ligase activation. Sci Rep. (2018) 8:3499. doi: 10.1038/s41598-018-21506-7
61. Rodríguez-Nieves JA, Patalano SC, Almanza D, Gharaee-Kermani M, Macoska JA. CXCL12/CXCR4 axis activation mediates prostate myofibroblast phenoconversion through non-canonical EGFR/MEK/ERK signaling. PLoS ONE. (2016) 11:e0159490. doi: 10.1371/journal.pone.0159490
62. Ray P, Lewin SA, Mihalko LA, Lesher-Perez SC, Takayama S, Luker KE, et al. Secreted CXCL12 (SDF-1) forms dimers under physiological conditions. Biochem J. (2012) 442:433–42. doi: 10.1042/BJ20111341
63. Veldkamp CT, Seibert C, Peterson FC, De la Cruz NB, Haugner JC III, Basnet H, et al. Structural basis of CXCR4 sulfotyrosine recognition by the chemokine SDF-1/CXCL12. Sci Signal. (2008) 1:ra4-ra. doi: 10.1126/scisignal.1160755
64. Drury LJ, Ziarek JJ, Gravel S, Veldkamp CT, Takekoshi T, Hwang ST, et al. Monomeric and dimeric CXCL12 inhibit metastasis through distinct CXCR4 interactions and signaling pathways. Proc Natl Acad Sci USA. (2011) 108:17655–60. doi: 10.1073/pnas.1101133108
65. Bromage DI, Davidson SM, Yellon DM. Stromal derived factor 1alpha: a chemokine that delivers a two-pronged defence of the myocardium. Pharmacol Ther. (2014) 143:305–15. doi: 10.1016/j.pharmthera.2014.03.009
66. Hu X, Dai S, Wu WJ, Tan W, Zhu X, Mu J, et al. Stromal cell derived factor-1 alpha confers protection against myocardial ischemia/reperfusion injury: role of the cardiac stromal cell derived factor-1 alpha CXCR4 axis. Circulation. (2007) 116:654–63. doi: 10.1161/CIRCULATIONAHA.106.672451
67. Huang C, Gu H, Zhang W, Manukyan MC, Shou W, Wang M. SDF-1/CXCR4 mediates acute protection of cardiac function through myocardial STAT3 signaling following global ischemia/reperfusion injury. Am J Physiol Heart Circ Physiol. (2011) 301:H1496–505. doi: 10.1152/ajpheart.00365.2011
68. Ziff OJ, Bromage DI, Yellon DM, Davidson SM. Therapeutic strategies utilizing SDF-1α in ischaemic cardiomyopathy. Cardiovasc Res. (2018) 114:358–67. doi: 10.1093/cvr/cvx203
69. Ghadge SK, Mühlstedt S, Ozcelik C, Bader M. SDF-1α as a therapeutic stem cell homing factor in myocardial infarction. Pharmacol Ther. (2011) 129:97–108. doi: 10.1016/j.pharmthera.2010.09.011
70. Schmiedt CW, Nelson SA, Brainard BM, Brown CA, Vandenplas M, Hurley DJ. Bilateral renal ischemia as a model of acute kidney injury in cats. Res Vet Sci. (2012) 93:950–9. doi: 10.1016/j.rvsc.2011.12.004
71. Brown CA, Rissi DR, Dickerson VM, Davis AM, Brown SA, Schmiedt CW. Chronic renal changes after a single ischemic event in an experimental model of feline chronic kidney disease. Vet Pathol. (2019) 56:536–43. doi: 10.1177/0300985819837721
72. Dickerson VM, Rissi DR, Brown CA, Brown SA, Schmiedt CW. Assessment of acute kidney injury and renal fibrosis after renal ischemia protocols in cats. Comp Med. (2017) 67:56–66.
73. McKleroy W, Lee T-H, Atabai K. Always cleave up your mess: targeting collagen degradation to treat tissue fibrosis. Am J Physiol Lung Cell Mol Physiol. (2013) 304:L709–21. doi: 10.1152/ajplung.00418.2012
74. Klein T, Bischoff R. Physiology and pathophysiology of matrix metalloproteases. Amino Acids. (2011) 41:271–90. doi: 10.1007/s00726-010-0689-x
75. Inoue H, Goda S, Domae N, Nosaka K, Nakai M, Uchihashi K, et al. Matrix metalloproteinase 1 produced by CXCL12 stimulation on NK92 cells. J Osaka Dental Univ. (2009) 43:163–7. doi: 10.18905/jodu.43.2_163
76. Singh S, Singh UP, Grizzle WE, Lillard JW. CXCL12-CXCR4 interactions modulate prostate cancer cell migration, metalloproteinase expression and invasion. Lab Investig. (2004) 84:1666–76. doi: 10.1038/labinvest.3700181
77. Goda S, Inoue H, Umehara H, Miyaji M, Nagano Y, Harakawa N, et al. Matrix metalloproteinase-1 produced by human CXCL12-stimulated natural killer cells. Am J Pathol. (2006) 169:445–58. doi: 10.2353/ajpath.2006.050676
78. Ho IA, Yulyana Y, Sia KC, Newman JP, Guo CM, Hui KM, et al. Matrix metalloproteinase-1-mediated mesenchymal stem cell tumor tropism is dependent on crosstalk with stromal derived growth factor 1/C-X-C chemokine receptor 4 axis. FASEB J. (2014) 28:4359–68. doi: 10.1096/fj.14-252551
79. Schmelzer CEH, Heinz A, Troilo H, Lockhart-Cairns MP, Jowitt TA, Marchand MF, et al. Lysyl oxidase-like 2 (LOXL2)-mediated cross-linking of tropoelastin. FASEB J. (2019) 33:5468–81. doi: 10.1096/fj.201801860RR
80. Cosgrove D, Dufek B, Meehan DT, Delimont D, Hartnett M, Samuelson G, et al. Lysyl oxidase like-2 contributes to renal fibrosis in Col4α3/Alport mice. Kidney Int. (2018) 94:303–14. doi: 10.1016/j.kint.2018.02.024
81. Choi S-E, Jeon N, Choi HY, Shin JI, Jeong HJ, Lim BJ. Lysyl oxidase-like 2 is expressed in kidney tissue and is associated with the progression of tubulointerstitial fibrosis. Mol Med Rep. (2017) 16:2477–82. doi: 10.3892/mmr.2017.6918
82. Grossman M, Ben-Chetrit N, Zhuravlev A, Afik R, Bassat E, Solomonov I, et al. Tumor cell invasion can be blocked by modulators of collagen fibril alignment that control assembly of the extracellular matrix. Cancer Res. (2016) 76:4249–58. doi: 10.1158/0008-5472.CAN-15-2813
83. Hall JA, Fritsch DA, Jewell DE, Burris PA, Gross KL. Cats with IRIS stage 1 and 2 chronic kidney disease maintain body weight and lean muscle mass when fed food having increased caloric density, and enhanced concentrations of carnitine and essential amino acids. Vet Rec. (2019) 184:190. doi: 10.1136/vr.104865
Keywords: chemokine CXCL12, stromal cell-derived factor (SDF)-1α, local intra-renal injection, tubulo- interstitial fibrosis, ischemia/reperfusion injury, histomorphometry of collagen fibers
Citation: Bennington J, Lankford S, Magalhaes RS, Shankle D, Fanning J, Kartini C, Suparto I, Kusumawardhani W, Putra MA, Mariya S, Badlani G and Williams JK (2021) Chemokine Therapy in Cats With Experimental Renal Fibrosis and in a Kidney Disease Pilot Study. Front. Vet. Sci. 8:646087. doi: 10.3389/fvets.2021.646087
Received: 25 December 2020; Accepted: 12 February 2021;
Published: 04 March 2021.
Edited by:
Jan H. Spaas, Boehringer Ingelheim, GermanyReviewed by:
Dominique Paepe, Ghent University, BelgiumCopyright © 2021 Bennington, Lankford, Magalhaes, Shankle, Fanning, Kartini, Suparto, Kusumawardhani, Putra, Mariya, Badlani and Williams. This is an open-access article distributed under the terms of the Creative Commons Attribution License (CC BY). The use, distribution or reproduction in other forums is permitted, provided the original author(s) and the copyright owner(s) are credited and that the original publication in this journal is cited, in accordance with accepted academic practice. No use, distribution or reproduction is permitted which does not comply with these terms.
*Correspondence: Julie Bennington, amJlbm5pbmdAd2FrZWhlYWx0aC5lZHU=
Disclaimer: All claims expressed in this article are solely those of the authors and do not necessarily represent those of their affiliated organizations, or those of the publisher, the editors and the reviewers. Any product that may be evaluated in this article or claim that may be made by its manufacturer is not guaranteed or endorsed by the publisher.
Research integrity at Frontiers
Learn more about the work of our research integrity team to safeguard the quality of each article we publish.