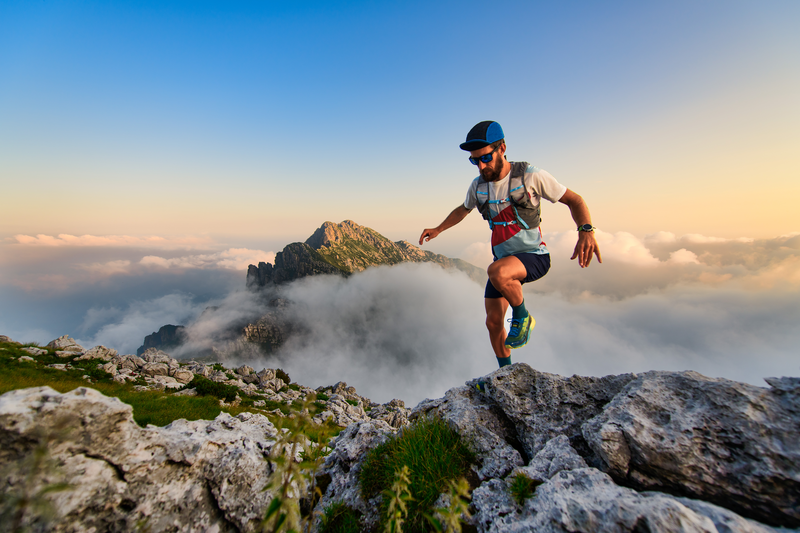
94% of researchers rate our articles as excellent or good
Learn more about the work of our research integrity team to safeguard the quality of each article we publish.
Find out more
BRIEF RESEARCH REPORT article
Front. Vet. Sci. , 08 July 2021
Sec. Veterinary Epidemiology and Economics
Volume 8 - 2021 | https://doi.org/10.3389/fvets.2021.618497
This article is part of the Research Topic Bridging Science and Policy for Surveillance, Economics and Social Sciences: ICAHS & ISESSAH 2020 View all 17 articles
Antimicrobial use (AMU) and antimicrobial resistance (AMR) are a growing public health and economic threat in Vietnam. We conducted a pilot surveillance programme in five provinces of Vietnam, two in the south and three in the north, to identify antimicrobial resistance (AMR) in rectal swab samples from pigs and fecal samples from chickens at slaughter points during three different points in time from 2017 to 2019. Escherichia coli (E. coli) and non-typhoidal Salmonella (NTS) isolates were tested for antimicrobial susceptibility using disk diffusion assay for 19 antimicrobial agents belonging to nine antimicrobial classes and Etest for colistin (polymyxin). Almost all E. coli (99%; 1029/1042) and NTS (96%; 208/216) isolates were resistant to at least one antimicrobial agent; 94% (981/1042) of E. coli and 89% (193/216) of NTS isolates were multidrug-resistant (MDR). Higher proportions of E. coli and NTS isolated from chickens were resistant to all antimicrobial classes than those isolates from pigs. There was a significantly higher proportion of MDR NTS isolates from the southern provinces of Ho Chi Minh City and Long An (p = 0.008). Although there were increasing trends of NTS in proportion of resistance to fluoroquinolone over the three surveillance rounds, there was a significant decreasing trend of NTS in proportion of resistance to polymyxin (p = 0.002). It is important to establish an annual AMR surveillance program for livestock in Vietnam to assess the impact of interventions, observe trends and drive decision making that ultimately contributes to reducing AMR public health threat.
Antimicrobial use (AMU) and antimicrobial resistance (AMR) are a growing public health and economic threat in Vietnam and countries in southeast Asia (1). Escherichia coli (E. coli) isolated from pig and chicken farms in Vietnam had extremely high resistance to ampicillin and ciprofloxacin, and high resistance to gentamycin and colistin (2). A similar situation has also been observed in Cambodia (3). A very high proportion of non-typhoidal Salmonella (NTS), isolated from meat in the market in Vietnam was resistant to quinolone and beta-lactams (4). Overall, the use of antimicrobials in livestock production and poor biosecurity, especially on small farms, were associated with high levels of AMR in Vietnam (2, 5).
Resistance genes, resistant bacteria and antibiotic residues can spread to humans via direct contact with animals, wastewater, slurry, vegetables, and animal by-products (6, 7). Before the ban of antimicrobial growth promoters in 2018 in Vietnam, the most widely used in-feed antibiotics in chicken production were bacitracin, chlortetracycline and enramycin. In pig production the most used antibiotics were bacitracin, chlortetracycline and florfenicol (1).
The World Health Organization (WHO) has listed five antimicrobial classes of highest priority among the critically important antimicrobials (CIAs); cephalosporins (3rd, 4th, and 5th generations), glycopeptides, macrolides and ketolides, polymyxins, and quinolones (8). A 2019 study in Indonesia, Thailand, and Vietnam identified neomycin, colistin and amoxicillin in pig and chicken feeds, all of which are antimicrobials classified as critically important or highly important in treatment of human infections (9).
In 2017, Vietnam's Ministry of Agriculture and Rural Development (MARD) issued a national action plan for AMR and AMU in livestock and aquaculture in line with the Food and Agriculture Organization of the United Nations (FAO) Action Plan on AMR (10). One of the main activities under this plan was to establish and implement a national programme for the surveillance of AMR in animals and food (11). MARD assigned the Department of Animal Health as the focal agency for AMU and AMR management in livestock. In collaboration with Department of Animal Health, FAO, conducted a pilot surveillance to support the development of the national AMR surveillance programme and establish a baseline that could be used to track AMR level changes. This pilot surveillance was designed to estimate AMR in two commensal bacteria, E. coli and non-typhoid Salmonella (NTS), in pigs and chickens. We expected that the surveillance would also allow comparison of AMR spatially (between geographical regions) and temporally (between rounds of sampling) in pigs and chickens produced in Vietnam.
The pilot surveillance was conducted in five provinces in Vietnam: three in the north, Hanoi, Hai Phong, Quang Ninh; and two in the south, Ho Chi Minh City and Long An. The first round of surveillance was conducted in August 2017, the second round in February 2019 and the third round in August 2019. The target population was chickens sold at wet markets and pigs at slaughter points.
The National Center for Veterinary Hygiene and Inspection (NCVHI) No.1 in Hanoi, which is an assigned laboratory for AMR surveillance, was assessed for technical capacity and biological material management before conducting the first round of the pilot surveillance using FAO Assessment Tool for Laboratory and AMR Surveillance Systems (ATLASS) in April 2017. NCVHI is also accredited by the ISO/IEC 17025 standard for conducting isolation and identification of E. coli and NTS.
For the sample size, we followed the European Food Safety Authority's target sample size of 170 isolates tested for each species (12). Seven pigs and seven chickens slaughter points (either at slaughterhouses or wet markets) with the highest number of animals processed in each region (north and south) were selected. NCVHI staff conveniently selected 25 pig rectal swabs from all holding pens, or 25 chicken droppings from all chicken cages from each slaughter points for sample collection. The total sample size of 350 for each species collected each round was assumed to yield at least 170 NTS isolates if the prevalence of NTS was 50% in pigs and chickens (13). All laboratory tests were performed at NCVHI No. 1 were based on protocols, including reference strains established by Department of Animal Health following FAO regional antimicrobial resistance surveillance and monitoring guidelines (14).
Samples for NTS were stored in a falcon tube containing 10 ml buffered peptone water at room temperature (25°C). Samples for E. coli were stored in a falcon tubes and kept cool (4°C) immediately after sampling. All E. coli or Salmonella isolates were stored at the laboratory, preserved in appropriate condition before conducting the antimicrobial susceptibility testing. Isolates to be tested and quality control strains were revived by using a nutrient agar medium. NTS isolates were not further characterized (serogrouping and serotyping). Antimicrobial susceptibility testing of E. coli and NTS isolated from pig and chicken fecal samples were conducted using the Kirby-Bauer disk diffusion test for 19 agents belonging to nine antimicrobial classes. Etest was used to determine resistance to colistin. E. coli and NTS isolates were classified as resistant using Clinical Laboratory Standard Institute (15) and European Committee on Antimicrobial Susceptibility Testing (www.EUCAST.org) breakpoints. Reference strains for testing quality control were used, including: Salmonella typhimurium American Type Culture Collection (ATCC) 14020 for identification of NTS; ATCC E. coli 25922 for identification of E. coli; P. Aeruginosa ATCC 27853 for cat-ion control; Enterococcus faecalis ATCC 29212 for thymidine control in the medium; the ATCC E. coli 25922 and the K. pneumoniae ATCC 700603 for antimicrobial susceptibility testing. A multidrug resistant (MDR) strain is defined as a strain's non-susceptibility to at least one agent in three or more antimicrobial classes (16). Enrofloxacin was classified as resistant if the zone diameter was ≤ 16 mm. For colistin, an Etest (AB Biodisk, Solna, Sweden), a minimum inhibitory concentration (MIC) assay, was used with the criteria that isolates with MIC > 4 were classified as resistant. Proportions of resistance were compared using the chi-square test.
Significant levels of increasing or decreasing trends over the three rounds of sampling were determined using the score test for trend of resistant odds from round one to round three. A multivariable logistic regression model with slaughter points as a random effect was used to estimate odds ratio (OR) of resistance isolated from different species (pig/chicken), regions (north/south) and rounds (August 2017/February 2019/August 2019). We include rounds in the model to clarify the direction of the trend and differences between sampling rounds. Resistance to at least one agent in each antimicrobial class was used as an outcome variable. All variables were kept in the model to control for each other. All statistical tests were conducted at 0.05 significant level. All statistical analysis was performed using STATA (College Station, TX).
Samples and data were collected by the Department of Animal Health in Vietnam. Information on specific animals was collected from slaughterhouses and wet markets without identifying the owners' personal information.
A total of 1,050 samples were tested (Table 1). The overall E. coli isolation rate was 99% (1042/1050) and 21% (216/1050) for NTS. There was significantly (p < 0.001) more NTS isolated from pigs (26%, 136/525) than chickens (15%, 80/525) while E. coli isolation rate in both pigs (100%, 523/525) and chickens (99%, 519/525) were not significantly different (p = 0.156). The isolation rates for E. coli (345/350, 350/350, 347/350; p = 0.091) and NTS (64/349, 80/350, 72/350; p = 0.336) were not significantly different when comparing the three surveillance rounds.
Table 1. Collection of pig and chicken samples during three rounds of antimicrobial resistance surveillance in five provinces in Vietnam, 2017–2019.
Resistance to at least one antimicrobial agent was found in 99% (1042/1050) of E. coli and 96% (216/1050) of NTS isolates. MDR was found in 94% (981/1042) of E. coli and 89% (193/216) of NTS isolates. Many of the E. coli isolates showed extremely high proportions of resistant with more than 70% of isolates being resistance to six antimicrobials tested (Figure 1). A high proportion of E. coli isolated from both chickens (95%, 494/519) and pigs (93%, 487/523) were MDR. A higher proportion of E. coli isolates from chicken were resistant to all antimicrobial classes, than those isolated from pigs. The proportion of MDR E. coli was not significantly different over the three surveillance rounds. The decreasing trend of E. coli resistance to macrolide (p = 0.001) and polymyxin (p < 0.001) is shown in Figure 2. When comparing the two southern and three northern provinces, there was a significantly lower proportion of E. coli with resistance to cephalosporin (OR = 0.6, p = 0.001) and polymyxin (OR = 0.5, p = 0.001) in the south compared to the north (Table 2).
Figure 1. Percentages of antimicrobial resistance in Escherichia coli in pig and chicken samples collected from five provinces in Vietnam, 2017–2019.
Figure 2. Decreasing trend of Escherichia coli resistance to macrolide and polymyxin in pig and chicken samples collected from five provinces in Vietnam, 2017–2019.
Table 2. Association between Escherichia coli resistance, species, region and round, Vietnam, 2017–2019.
Many of the NTS isolates showed high proportions of resistance (Figure 3). In NTS isolated from chickens, 95% (76/80) were MDR, while 86% (117/136) of NTS isolated from pigs were MDR. Higher proportions of NTS isolated from chickens were resistant to all antimicrobial classes tested than those isolates from pigs (Table 3). The decreasing trend of NTS resistance to macrolide (p < 0.001) and polymyxin (p = 0.002) is shown in Figure 4.
Figure 3. Antimicrobial resistance in non-typhoidal Salmonella in pig and chicken samples collected from five provinces in Vietnam for 20 antimicrobials, 2017–2019.
Table 3. Association between non-typhoidal Salmonella resistance, species, region and round, Vietnam, 2017–2019.
Figure 4. Decreasing trend of non-typhoidal Salmonella resistance to macrolide and polymyxin in pig and chicken samples collected from five provinces in Vietnam, 2017–2019.
The proportion of MDR NTS isolated from pigs and chickens in the southern provinces was significantly higher (OR = 3.7, p = 0.008) than in the northern provinces (Table 3). When comparing the two provinces in the south to the three provinces in the north, there was a significantly higher proportion of resistant NTS to fluoroquinolones (OR = 3.0, p = 0.001), but a significantly lower proportion of resistance to cephalosporin (OR = 0.4, p = 0.010) (Table 3).
The median number of resistant antimicrobial classes in E. coli and NTS isolates from chickens was significantly (p < 0.001) higher than the median number in pigs (Table 4). There was a significantly higher proportion of MDR NTS isolates from the south (Ho Chi Minh city, Long An; p = 0.008) compared to the north (Hanoi, Hai Phong, Quang Ninh). Although there was an increasing trend of NTS in proportion of resistance to fluoroquinolone over the three surveillance rounds, there was a significant decreasing trend of NTS in proportion of resistance to polymyxin. Similar patterns were also observed in E. coli.
Table 4. Distribution of multidrug resistant isolates collected from pig and chicken samples during three rounds of antimicrobial resistance surveillance in five provinces in Vietnam, 2017–2019.
Both E. coli and NTS detected in this study are commensals bacteria that do not cause disease in animals, although some of these strains can cause diseases in human. Overall, this pilot surveillance found almost all E. coli and NTS with resistant to at least one antimicrobial tested. A high proportion of E. coli and NTS were MDR. Considering resistance to the WHO's CIA, there was a low proportion of E. coli and NTS with resistance to third generation cephalosporin (ceftazidime); low to moderate with resistance to polymixin (colistin) and fluoroquinolone (ciprofloxacin); and moderate to high with resistance to macrolide (azithromycin). However, there was a decreasing trend of E. coli and NTS resistance to macrolide and polymyxin, shown by both regression and trend analysis, after the 2018 ban of antimicrobial growth promoters. A variety of resistance characteristics between E. coli and NTS were identified between the northern and southern provinces of Vietnam.
The prevalence of NTS isolated from pigs (26%, 136/525) and chickens (15%, 80/525) were much lower than the NTS prevalence identified at pig (65%) and chicken (31%) farms (13), and in pork (70%) and chicken meat (70%) sold at the market (4). However, the lower prevalence may have resulted from using individual samples collected from many different sources of animals at slaughter points as opposed to using environmental samples from farms or markets. Despite the lower prevalence, NTS was found in this study and could be potentially be transmitted to humans and cause disease via under cooked food. The proportion of MDR Salmonella in Vietnam detected in this study was much higher than those reported earlier in Vietnam (17), Thailand and Cambodia (18). We currently do not have any explanation for this difference, but further analysis comparing antimicrobial consumption among these countries may shed some light on this issue. Salmonella, with resistance to third generation cephalosporin, one of WHO highest priority CIAs, was reported earlier in Vietnam with an increasing proportion of resistance from <1% in 2015 to 4% in 2018 and up to 22% (48/216) in this study. Similarly, resistance to fluoroquinolone, another WHO priority CIA, in this and more recent studies, is much higher than earlier reported (4, 13).
We found the proportion of E. coli with resistance to colistin in both pigs and chicken (11%, 112/1,042) was lower than other studies (22–24%) had reported earlier (2), corresponding to the lower proportion of Extended-Spectrum Beta-Lactamases (ESBL) producing E. coli also found in this study. A study in Vietnam published in 2018 showed a high occurrence of ESBL producing E. coli in both pig farmers and pigs that could pose a risk of transmission of these bacteria from pig farms to the community (19).
This pilot surveillance study had several limitations. The model for the sample size was from the European Food Safety Authority that stated the optimal sample size should be 170 positive isolates of E. coli and NTS for each bacterium in each animal species. However, we only found 80 positive NTS isolates in chicken, which is arguably not a strong enough sample size to evaluate resistance in NTS. We also assumed that the 25 samples from each slaughter point would represent animals from 25 different locations. However, animals could not be traced back to the farm of origin; this could have led to clustering of samples from the same farms. Fecal samples were collected from conveniently selected droppings from cages in the wet markets. The microbial status of the bird cages may have impacted the results due to inadequate cleaning and disinfection. Although using the disk diffusion test is an affordable method to assess resistance, this method does not provide the MIC to allow for subsequent analysis, especially when different interpretive criteria are used or the criteria change (14). Further typing of NTS found in this study can clarify the risk poses.
In conclusion, the observed dynamic of resistance of E. coli and NTS to many antimicrobials warrant the development of a national AMR surveillance programme to monitor the situation and support AMU management policy development. As our findings differed between the two geographical areas, management may need to be customized for the northern and southern areas of the country. The decreasing trend of resistance to WHO's highest priority critically important antimicrobials detected by the surveillance programme can also be used to strengthen the implementation of existing AMU management policy such as banning of antimicrobial growth promoters and advocate for further usage reduction policy such as banning of prophylaxis use. AMR is a public health concern, requiring cross-sectoral collaboration. From a public health perspective, it is especially important to monitor WHO's highest priority critically important antimicrobial classes in animals as well as in humans. We recommend that a national AMR surveillance program for livestock be established in Vietnam. Annual surveillance makes it possible to assess the impact of interventions, observe trends and drive decision making that ultimately contributes to reducing the public health threat from AMR. This antimicrobial resistance data in livestock, generated by the Vietnam Department of Animal Health, has contributed to the global antimicrobial resistance surveillance database and can be used for joint analysis with human health surveillance data under the One Health approach. Future One Health surveillance design, involving human health, animal health and the private sector can be developed through the identification of appropriate levels of collaboration, depending on the expected positive impacts on the value of surveillance (20). The multi-sectoral AMR surveillance, leading to the harmonization and combination of data from different sources, could improve knowledge on transmission routes and risk factors related to AMR in human, animal and environment (21). Specifically, the AMR surveillance programme can be included under the next phase of the national action plan for the management of AMU and monitoring of AMR 2021–2025. The national action plan would provide a legal basis for mobilizing resource from central and provincial authorities to support the surveillance activities and ensure sustainability of the surveillance programme.
The raw data supporting the conclusions of this article will be made available by the authors, without undue reservation.
Ethical review and approval was not required for the animal study because The surveillance was conducted by a national competent authority for animal health.
CT, NT, LH, VG, and PP conceived and designed study. CT, PH, and NL conducted field sample collection and laboratory testing. CT, PH, NL, VE, and PP contributed to data analysis. CT, VE, and PP contributed to writing and editing the manuscript. All authors contributed to the article revision and approved the submitted version.
This work was conducted with financial support from the United States Agency for International Development (USAID) grant number GHA-G-00-06-00001, through the Food and Agriculture Organization of the United Nations.
The authors declare that the research was conducted in the absence of any commercial or financial relationships that could be construed as a potential conflict of interest.
We would like to acknowledge the technical support from the Oxford University Clinical Research Unit (OUCRU), the Director and staff of the microbiology laboratory of the National Center for Veterinary Hygiene Inspection No I, Sub-Department of Animal Health in Hanoi, Hai Phong, Quang Ninh, Ho Chi Minh City and Long An, and the owners of pig slaughterhouses and chicken vendors who assisted us in sample collection and provided essential information.
The Supplementary Material for this article can be found online at: https://www.frontiersin.org/articles/10.3389/fvets.2021.618497/full#supplementary-material
1. Nhung NT, Cuong NV, Thwaites G, Carrique-Mas J. Antimicrobial usage and antimicrobial resistance in animal production in Southeast Asia: a review. Antibiotics (Basel). (2016) 5:37. doi: 10.3390/antibiotics5040037
2. Nguyen NT, Nguyen HM, Nguyen CV, Nguyen TV, Nguyen MT, Thai HQ, et al. Use of colistin and other critical antimicrobials on pig and chicken farms in Southern Vietnam and its association with resistance in commensal Escherichia coli bacteria. Appl Environ Microbiol. (2016) 82:3727–35. doi: 10.1128/AEM.00337-16
3. Strom G, Boqvist S, Albihn A, Fernstrom LL, Andersson Djurfeldt A, Sokerya S, et al. Antimicrobials in small-scale urban pig farming in a lower middle-income country - arbitrary use and high resistance levels. Antimicrob Resist Infect Control. (2018) 7:35. doi: 10.1186/s13756-018-0328-y
4. Nhung NT, Van NTB, Cuong NV, Duong TTQ, Nhat TT, Hang TTT, et al. Antimicrobial residues and resistance against critically important antimicrobials in non-typhoidal Salmonella from meat sold at wet markets and supermarkets in Vietnam. Int J Food Microbiol. (2018) 266:301–9. doi: 10.1016/j.ijfoodmicro.2017.12.015
5. Nguyen VT, Carrique-Mas JJ, Ngo TH, Ho HM, Ha TT, Campbell JI, et al. Prevalence and risk factors for carriage of antimicrobial-resistant Escherichia coli on household and small-scale chicken farms in the Mekong Delta of Vietnam. J Antimicrob Chemother. (2015) 70:2144–52.
6. Holmes AH, Moore LS, Sundsfjord A, Steinbakk M, Regmi S, Karkey A, et al. Understanding the mechanisms and drivers of antimicrobial resistance. Lancet. (2016) 387:176–87. doi: 10.1016/S0140-6736(15)00473-0
7. Graham DW, Bergeron G, Bourassa MW, Dickson J, Gomes F, Howe A, et al. Complexities in understanding antimicrobial resistance across domesticated animal, human, and environmental systems. Ann N Y Acad Sci USA. (2019)1441:17–30. doi: 10.1111/nyas.14036
8. WHO. Critically Important Antimicrobials for Human Medicine 6th revision2019. Available online at: https://www.who.int/foodsafety/publications/antimicrobials-sixth/en/
9. Coyne L, Arief R, Benigno C, Giang VN, Huong LQ, Jeamsripong S, et al. Characterizing antimicrobial use in the livestock sector in three South East Asian Countries (Indonesia, Thailand, and Vietnam). Antibiotics (Basel). (2019) 8:33. doi: 10.3390/antibiotics8010033
10. FAO. The FAO action plan on antimicrobial resistance 2016-2020. (2016). Available online at: http://www.fao.org/3/a-i5996e.pdf
11. MARD. National action plan for management of antibiotic use and control of antibiotic resistance in livestock production and aquaculture. In: Development MoAaR. Hanoi: Ministry of Agriculture and Rural Development (MARD) (2017).
12. EFSA. Technical specifications on harmonised monitoring of antimicrobial resistance in zoonotic and indicator bacteria from food-producing animals and food. EFSA J. (2019) 17:5709. doi: 10.2903/j.efsa.2019.5709
13. Tu LT, Hoang NV, Cuong NV, Campbell J, Bryant JE, Hoa NT, et al. High levels of contamination and antimicrobial-resistant non-typhoidal Salmonella serovars on pig and poultry farms in the Mekong Delta of Vietnam. Epidemiol Infect. (2015) 143:3074–86. doi: 10.1017/S0950268815000102
14. FAO. Monitoring and Surveillance of Antimicrobial Resistance in Bacteria From Healthy Food Animals Intended for Consumption. (2019). Available online at: http://www.fao.org/3/ca6897en/CA6897EN.pdf
15. CLSI, editor. Performance Standards for Antimicrobial Disk and Dilution Susceptibility Testd for Bacteria Isolated from Animals. 4th ed. Wayne, PA: Clinical Laboratory Standard Institute (2013).
16. Magiorakos AP, Srinivasan A, Carey RB, Carmeli Y, Falagas ME, Giske CG, et al. Multidrug-resistant, extensively drug-resistant and pandrug-resistant bacteria: an international expert proposal for interim standard definitions for acquired resistance. Clin Microbiol Infect. (2012) 18:268–81. doi: 10.1111/j.1469-0691.2011.03570.x
17. Nguyen DT, Kanki M, Nguyen PD, Le HT, Ngo PT, Tran DN, et al. Prevalence, antibiotic resistance, and extended-spectrum and AmpC beta-lactamase productivity of Salmonella isolates from raw meat and seafood samples in Ho Chi Minh City, Vietnam. Int J Food Microbiol. (2016) 236:115–22. doi: 10.1016/j.ijfoodmicro.2016.07.017
18. Trongjit S, Angkititrakul S, Tuttle RE, Poungseree J, Padungtod P, Chuanchuen R. Prevalence and antimicrobial resistance in Salmonella enterica isolated from broiler chickens, pigs and meat products in Thailand-Cambodia border provinces. Microbiol Immunol. (2017) 61:23–33. doi: 10.1111/1348-0421.12462
19. Dang STT, Bortolaia V, Tran NT, Le HQ, Dalsgaard A. Cephalosporin-resistant Escherichia coli isolated from farm workers and pigs in northern Vietnam. Trop Med Int Health. (2018) 23:415–24. doi: 10.1111/tmi.13054
20. Bordier M, Binot A, Pauchard Q, Nguyen DT, Trung TN, Fortane N, et al. Antibiotic resistance in Vietnam: moving towards a One Health surveillance system. BMC Public Health. (2018) 18:1136. doi: 10.1186/s12889-018-6022-4
Keywords: pig, chicken, Salmonella, Escherichia coli, anti-bacterial agents, drug resistance, public health, livestock
Citation: Tuat CV, Hue PT, Loan NTP, Thuy NT, Hue LT, Giang VN, Erickson VI and Padungtod P (2021) Antimicrobial Resistance Pilot Surveillance of Pigs and Chickens in Vietnam, 2017–2019. Front. Vet. Sci. 8:618497. doi: 10.3389/fvets.2021.618497
Received: 17 October 2020; Accepted: 14 April 2021;
Published: 08 July 2021.
Edited by:
Salome Dürr, University of Bern, SwitzerlandReviewed by:
Agnes Agunos, Public Health Agency of Canada, CanadaCopyright © 2021 Tuat, Hue, Loan, Thuy, Hue, Giang, Erickson and Padungtod. This is an open-access article distributed under the terms of the Creative Commons Attribution License (CC BY). The use, distribution or reproduction in other forums is permitted, provided the original author(s) and the copyright owner(s) are credited and that the original publication in this journal is cited, in accordance with accepted academic practice. No use, distribution or reproduction is permitted which does not comply with these terms.
*Correspondence: Pawin Padungtod, cGF3aW4ucGFkdW5ndG9kQGZhby5vcmc=
Disclaimer: All claims expressed in this article are solely those of the authors and do not necessarily represent those of their affiliated organizations, or those of the publisher, the editors and the reviewers. Any product that may be evaluated in this article or claim that may be made by its manufacturer is not guaranteed or endorsed by the publisher.
Research integrity at Frontiers
Learn more about the work of our research integrity team to safeguard the quality of each article we publish.