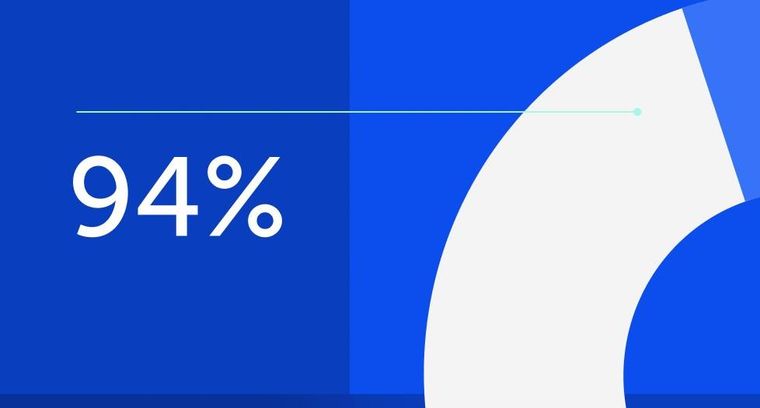
94% of researchers rate our articles as excellent or good
Learn more about the work of our research integrity team to safeguard the quality of each article we publish.
Find out more
ORIGINAL RESEARCH article
Front. Vet. Sci., 18 January 2021
Sec. Parasitology
Volume 7 - 2020 | https://doi.org/10.3389/fvets.2020.625641
This article is part of the Research TopicStowaways of a Stowaway: Parasites of Invasive RodentsView all 9 articles
Most Bartonella spp. are transmitted by fleas and harbored by small mammals which serve as reservoirs. However, little is known about the composition of fleas and their Bartonella spp. from small mammals in Central Europe. Therefore, the aims of this study were to investigate flea communities on small mammals from three differently structured sites (urban, sylvatic, renatured) in Germany as well as the prevalence of Bartonella spp. in small mammals and their parasitizing fleas. In total, 623 small mammals belonging to 10 different species (the majority were Myodes glareolus and Apodemus flavicollis) were available. Fleas were removed from the small mammals' fur, morphologically identified and DNA was extracted. To detect Bartonella spp., two conventional PCRs targeting the gltA gene and the 16S−23S rRNA intergenic spacer were carried out followed by sequencing. Obtained sequences were compared to those in GenBank. In total, 1,156 fleas were collected from 456 small mammals. Altogether, 12 different flea species (the majority were Ctenophthalmus agyrtes, Nosopsyllus fasciatus, and Megabothris turbidus) were detected. At the urban site mostly Leptopsylla segnis and N. fasciatus were collected which may be vectors of zoonotic pathogens to companion animals. The overall prevalence for Bartonella in small mammals was 43.3% and in fleas 49.1%. Five different Bartonella spp. were detected in small mammals namely B. grahamii, B. taylorii, B. doshiae, Bartonella sp. N40 and uncultured Bartonella sp. whereas in fleas four Bartonella spp. were found which were with the exception of B. doshiae identical to the Bartonella species detected in their small mammal hosts. While B. grahamii was the only zoonotic Bartonella sp. most Bartonella strains found in fleas and small mammals belonged to uncultured Bartonella spp. with unknown zoonotic potential. This study showed a high diversity of flea species on small mammals from Germany. Further, high prevalence rates of Bartonella species were detected both in fleas and in their mammalian hosts. Several different Bartonella species with a high genetic variability were discovered. Especially at the urban study sites, this may pose a risk for Bartonella transmission to companion animals and humans.
Bartonellosis, which can result in severe clinical symptoms in humans and their companion animals, is caused by the facultative intracellular alpha-proteobacteria Bartonella spp. (order Rhizobiales, family Bartonellaceae) (1). Bartonella spp. are arthropod-borne bacteria and mainly transmitted by fleas, lice, deer keds, and sandflies (2–5). Bartonellae are highly adapted to one specific or few closely related mammalian reservoir hosts in which they can cause long-lasting bacteremia. In contrast, infections in incidental hosts may evoke disease with a broad range of symptoms (6, 7). Amongst the most common reservoir hosts are cats, rodents and other small mammals. Phylogenetic analyses based on sequence data from rpoB, gltA, ribC, and groEL genes revealed four different deep-branching Eubartonellae lineages and additionally Bartonella australis (8). Bartonella tamiae and Bartonella apis could build two additional separate lineages, which is however not yet confirmed. Lineage 4 is the most diverse group regarding the variety of Bartonella spp. as well as reservoir host species. Thus far, the highest prevalence and highest diversity of Bartonella spp. were described in rodents. Five of these rodent-associated Bartonella spp. are known to be hazardous to human health (Bartonella grahamii, Bartonella elizabethae, Bartonella vinsonii subsp. arupensis, Bartonella washoensis, and B. tamiae) (9). In studies from Poland (10, 11), Sweden (12), France (13), and the UK (14) the prevalence of Bartonella spp. in rodents ranged from 0 to 72.2%. Fleas are suggested to serve as main vectors for Bartonella spp. which are associated with rodents (15, 16). Previous studies showed that fleas may transmit Bartonella spp. experimentally to their mammalian hosts (17, 18). Moreover, there are several epidemiologic studies based on the molecular analysis showing that rodent-associated fleas are also infected with Bartonella spp. in nature (19–21). Studies from the USA, Afghanistan, and Israel reported prevalences between 15.5 and 95% for Bartonella spp. in fleas collected from rodents and small mammals (19–22). The knowledge on the species diversity of fleas on small mammals and the Bartonella prevalence are very scarce in Central Europe. Small mammal species build the vast majority of hosts for over 50 different flea species (23). In Germany, there are only four reports about small mammal fleas from the last century and only one report which is more recent (19). Recently, our group reported high prevalences of Bartonella spp. in rodents (65.8%) and their associated fleas (54.1%) in Germany (24). Further studies on the prevalence and species diversity of Bartonella in rodents and especially their parasitizing flea species are scarce in Germany. The previous study by our group showed results from one location and the sample size examined did not allow statistical associations. Thus, the objectives of the present study were: (1) detection of flea species parasitizing small mammals and (2) detection of Bartonella spp. in small mammals and their fleas and (3) detection of associations between small mammals, fleas and Bartonella species.
To collect small mammals, traps were placed at three sites of urban, sylvatic or recultivated character. These locations were previously selected for field studies by our group (25, 26). The urban area (R1) “Dörnbergpark” (7.4 ha, 49°00′55.72″N, 12°05′08.89″E) is situated in the city centre of Regensburg, Bavaria, Southern Germany. It is a small well-tended park which was described in detail before (26, 27). The sylvatic area (T) “Angelberger Forst” (641 ha, 48°06′36.42″N, 10°34′33.40″E) is a large forest located in Bavaria, Southern Germany (28). The recultivated site (S) consisted of three trapping localizations (51°15′32.2″N, 12°21′02.5″E; 51°17′01.3″N, 12°21′00.6″E; 51°26′97.2″ N, 12°32′25.6″ E) which were previously examined by our group and named as sites “E,” “F,” and “G” (25). This area is surrounding a lake which was a former open pit brown coal mining region near Leipzig, Saxony, Eastern Germany (436 ha).
Altogether, 50 Sherman© live animal traps (H. B. Sherman Traps, Inc., Tallahassee, Fla., U.S.A.) were placed at each Bavarian site between July and October in 2012 and between April and September in 2013. In Saxony, 60 traps were placed between March and October in 2012 and between January and September in 2013 (official permit Site S: AZ 36.11–36.45.12/4/12-001, Site R1: 55.1-8646.4-140, Site T: 55.1-8646-2/30). Traps were placed for two consecutive nights per month and site and checked twice a day. Collected small mammals were anesthetized with CO2, then euthanized by cervical dislocation and stored at −80°C. Small mammals were morphologically identified using taxonomic keys (29). Additionally, randomly selected rodents (15 Apodemus sylvaticus, 14 Myodes glareolus, and 23 A. flavicollis, 5 Microtus arvalis, 1 Mi. agrestis) as well as all shrews (Sorex spp.; n = 5) (by-catch found dead in traps) and least weasels (Mustela nivalis; n = 2) were identified by conventional PCR targeting the cytochrome b gene (354 bp) (30). A complete necropsy was performed with the collection of spleen samples. Cross contamination may be ruled out during dissection as each small mammal was handled with its own set of dissection instruments. Disinfection of working surfaces was performed after each individual and gloves were changed. Fleas were collected with tweezers from the fur during small mammal dissection. Fleas were stored individually in 100 μl RNALater (Qiagen, Hilden Germany) until morphological identification under a stereomicroscope (31, 32). Detailed information about trapping procedures and sampling sites have been given before (25–28).
In a previous study by our group, 623 small mammals belonging to 10 different species (395 My. glareolus, 172 A. flavicollis, 6 A. agrarius, 35 A. sylvaticus, 6 Mi. arvalis, 1 Mi. agrestis, 2 M. nivalis, 4 Sorex coronatus, one Sorex araneus, and one Talpa europaea) were captured (26, 33).
DNA was extracted from all collected rodents' spleens and from a preselected number of fleas (n = 450) which were collected from the small mammals. The DNA extraction was carried out for each sample individually with the QIAamp DNA Mini Kit (Qiagen, Hilden, Germany) as previously described (20). The quality and quantity of the extracted DNA samples were measured spectrophotometrically (NanoDrop ND-1000, Erlangen, Germany). DNA samples exceeding a concentration of 40 ng/μl were additionally diluted with elution buffer in order to avoid false negative results.
DNA samples were tested for the presence of Bartonella spp. via conventional polymerase chain reaction (PCR) targeting the gltA gene with BhCS.1137n (5′-AATGCAAAAAGAACAGTAAACA-3′) as forward and BhCS.781p (5′-GGGGaCCaGCTCATGGTGG-3′) as reverse primer (34). All samples were further processed by an additional PCR targeting 453–780 base pairs (bp) of the 16S−23S rRNA intergenic spacer (ITS) region with the forward primer Ba325s (5′-CTTCAGATGATGATCCCAAGCCTTCTGGCG-3′) and the reverse primer Ba1100as (5′-GAACCGACGACCCCCTGCTTGCAAAGCA-3′) (35, 36). Visualization of PCR products followed under UV-light on 2% agarose gel dyed with GelRedTM (Biotium, Hayward CA, USA). As the gltA gene is considered to be more sensitive, only samples which were positive in both genes were considered positive and further processed by sequencing. Purification of PCR products of the samples positive in ITS was carried out with the QIAquick PCR purification Kit (Qiagen) according to the manufacturer's recommendations. Purified amplicons were sequenced by Eurofins MWG Operon (Martinsried, Germany) with both primers and sequences were analyzed with Chromas Lite® (Technelysium Pty Ltd, South Brisbane, Australia) as formerly described (35). Obtained sequences were aligned with sequences from GenBank using BLASTn (National Center for Biotechnology Information, Bethesda MD, USA) and deposited in GenBank under following Acc. No.: MT551048-MT551101 and MT913158-MT913206. In total 33% of the positive rodent samples and 29% of the positive flea samples were sequenced. Sequences were considered as a matching result in GenBank with at least a similarity of 97.7%.
Confidence intervals (95%CI) for the prevalence of Bartonella spp. in small mammals and fleas were determined by the Clopper and Pearson method using Graph Pad Software (Graph Pad Software Inc., San Diego, Ca., USA). Independence of compared small sample sizes (n < 30) was tested with Fisher's exact test, respectively with the chi-squared test for sample sizes n > 30. The t-test was used to test significant differences of flea infestation on My. glareolus and A. flavicollis. The significance threshold was set at p ≤ 0.05.
In total, 1,156 fleas were collected from 456 small mammals. Altogether, twelve different flea species were detected (873 Ctenophthalmus agyrtes, 3 Ctenophthalmus bisoctodentatus, 62 Ctenophthalmus congener, 98 Megabothris turbidus, 8 Megabothris walkeri, 9 Hystrichopsylla talpae talpae, 25 Peromyscopsylla silvatica, 3 Paleopsylla soricis, 44 Nosopsyllus fasciatus, 11 Typhloceras poppei, 17 Leptopsylla segnis) (Table 1). Except for individuals belonging to the insectivore species T. europaea and Sorex spp., all other small mammal species were infested with fleas. The infestation prevalence ranged from 20 to 100% per small mammal species. The most prevalent species was C. agyrtes, which was found on all infested small mammal species. The flea burden was significantly higher on A. flavicollis compared to My. glareolus (t = −91.32; p < 0.0001). Megabothris turbidus was significantly more often collected from My. glareolus than from all other small mammal species (t = −5.65; p < 0.0001). Nosopsyllus fasciatus was significantly more frequently collected from specimens belonging to the family Muridae (Apodemus spp.) than from those belonging to the family Cricetidae (Microtus spp.; Myodes spp.) (t = −4.16; p = 0.00021). Leptopsylla segnis was exclusively found on A. sylvaticus, which were trapped only in the urban habitat. Peromyscopsylla silvatica were significantly more often collected from My. glareolus compared to all other small mammal species (t = −3.23; p = 0.0006).
Only samples yielding a positive result in both PCR approaches were considered positive in the following analysis. In total, 43.3% (95%CI: 39.5–47.3) of all small mammals were positive for Bartonella spp. Though not infested with fleas, positive T. europaea (100%; 95%CI: 16.75–100) and Sorex spp. (20%; 95%CI: 0–11.5) were detected. The prevalences were quite high in all captured small mammal species (20–100%) with the exception of Microtus spp. which were all negative and thus significantly less often infected than other species (p = 0.007). Considering the two most frequently captured species, the prevalence was significantly higher in A. flavicollis compared to My. glareolus (p < 0.0001; Table 2).
Table 2. Bartonella spp. detection based on the gltA gene and the 16S−23S rRNA ITS and species determination based on the 16S−23S rRNA ITS in spleen samples from different small mammal species.
A total of 88 out of 270 (33%) PCR products were selected by small mammal species and location to be further processed in order to determine the Bartonella species via sequence analysis. Altogether five different Bartonella species were detected in rodents and Sorex araneus (Table 2). The most prevalent species group (n = 56) which was detected in small mammals were uncultured Bartonella species. Altogether 56 sequences obtained in this study showed 100% identity to altogether 16 different uncultured Bartonella spp. sequences deposited in GenBank (Table 3), and these sequences showed 27–99% homology to one another. Bartonella grahamii was significantly more often detected in My. glareolus compared to A. flavicollis (p = 0.0370). Bartonella doshiae was mainly detected in My. glareolus. There were four very short sequences (below 430 base pairs) which were not considered as a positive sequencing result and therefore not taken into consideration for Bartonella species identification.
Table 3. Number of Bartonella sp. sequences based on the 16S−23S rRNA ITS of Bartonella found in small mammals and fleas in this study in comparison to sequences from GenBank.
Overall, 221 out of 450 fleas were tested positive for Bartonella spp. [49.1% (95%CI: 44.5–53.7)]. Every tested flea species was positive for Bartonella spp. with a prevalence ranging from 18.8 to 100% (Table 3). The prevalence levels of Bartonella spp. did not differ significantly comparing the most prevalent flea species (C. agyrtes, M. turbidus, N. fasciatus, C. congener; χ2 = 1.8; p = 0.6121). Comparing small mammals and fleas, the prevalence with Bartonella spp. was almost identical and thus not significantly different (p = 0.9018). Positive fleas derived from 53 negative and 54 positive small mammals.
In total, 74 positive samples (29%) were further determined to species level by sequencing which revealed four different Bartonella species in the examined fleas. All confirmed Bartonella species detected in fleas were the same Bartonella species as described for their small mammal hosts. However, most samples were positive for uncultured Bartonella spp. which showed 100% identity to 13 different sequences deposited in GenBank (Table 3). Almost all strains found in fleas were identical to those already found in their small mammal hosts (Table 4). Even though the distribution of the Bartonella species found in fleas was not completely identical compared to Bartonella spp. in small mammals, the prevalence of each Bartonella species did not differ significantly between small mammals and fleas (p = 0.2418–0.7631). Due to very small sample sizes of most flea species, statistical comparisons between the flea species were not carried out.
Table 4. Bartonella spp. detection based on the gltA gene and the 16S−23S rRNA ITS and species determination based on the 16S−23S rRNA ITS in fleas collected from small mammals.
This study reports high prevalence rates of different Bartonella species in small mammals (43.3%) and their fleas (49.1%) from Germany. The Bartonella species detected in the current study were the same as earlier described by our group in small mammals from one of the investigated study sites (urban, renatured, and sylvatic) (24). The prevalences in small mammals from the current study as well as the detected Bartonella spp. are in line with those from Poland, France, the Netherlands, Slovenia, and Germany (11–72%) (10, 13, 24, 37, 38). Small mammals are known to be the main reservoirs for over 22 different Bartonella species (7, 8). The current study reports four Bartonella species belonging to lineage four in small mammals (B. grahamii, B. doshiae, B. taylorii, Bartonella sp. N40;). Of the detected Bartonella spp., only B. grahamii is yet known to be zoonotic. Bartonella grahamii was isolated from My. glareolus from the UK for the first time (39). Since, it was found in rodents from almost all over the world and also caused disease in humans (40). Bartonellosis caused by B. grahamii displays similar symptoms as cat scratch disease such as enlarged lymph nodes, fever and fatigue. Even though cats are not considered competent reservoirs for B. grahamii, reports showed that they may still transmit the pathogen to humans via cat scratches when carrying infected rodent tissue on their claws (40). Most cases of bartonellosis caused by B. grahamii are likely to remain undiagnosed due to the mild unspecific symptoms, insufficient diagnostic measures and the lack of awareness of practitioners (40). Further, 16 different uncultured Bartonella spp. strains of yet unknown pathogenic potential were found in small mammals from the current study. This finding makes it obvious why clinical and public awareness of this zoonotic threat have to be increased.
Regarding the investigated small mammal species, Apodemus spp. showed a significantly higher infection rate compared to My. glareolus providing evidence that My. glareolus is able to resolve Bartonella infection after a certain time, while resolving a Bartonella infection has not been observed in Apodemus spp. yet (10). Moreover, it has been described that the re-infection rate in Apodemus spp. is higher than in My. glareolus which could also explain the higher prevalence in Apodemus spp.
The infestation rate with fleas may also influence the Bartonella prevalence in small mammals. In the current study, Apodemus spp. were more often infested with fleas and the infestation rate of fleas was higher compared to My. glareolus, which was also described in earlier studies from Germany and explained by the larger body size of Apodemus spp. (41). This higher infestation rate may have resulted in a higher Bartonella prevalence in Apodemus spp. High Bartonella prevalence rates (36–42%) were reported in Mi. arvalis and Mi. agrestis from Finland and Poland, respectively (9, 42). Further, another study by our group showed moderate to high prevalence rates in Microtus spp. from the Czech Republic and Germany (43). In the current study, both Microtus species (n = 8) were the only rodent species found negative for Bartonella spp. However, the sample size tested was rather low. Although no fleas were found on the insectivores analyzed in this study, all insectivore species were positive for Bartonella spp. In Sweden, it has been reported that insectivores may serve as reservoirs for certain Bartonella species (37). Future studies need to be conducted in order to confirm this observation. The urban study site was the only site where A. sylvaticus were trapped and L. segnis were detected. Further L. segnis is known to be a vector of Rickettsia felis and Rickettsia typhi and to occur mainly on small mammals which live synanthropic such as Mus musculus and Rattus norvegicus (44). The name “A. sylvaticus” is misleading as this species is likewise synanthropic and a well-known host for L. segnis (45). As the urban study site is a small park surrounded by walls and a high-traffic road, this study suggests it has basically a small ecological niche on its own. The proximity of small mammals to human settlements may pose a risk thus to the health of companion animals and humans.
The flea species may vary in their host specificity of being highly host-specific to being only host-opportunistic (46). The variety of flea species found in this study was high with twelve identified species. This high diversity is quite unexpected as previous studies from Poland, the UK and Germany found only 4–10 different flea species on the mentioned small mammal species (24, 41, 47, 48). However, one should consider that the current study covered three completely differently structured study sites which may have led to a higher variety of flea species. The flea burden was higher on Apodemus spp. than on all other small mammal species. It is known that there is a higher immune resistance against flea burden in Microtus spp. compared to Apodemus spp. and My. glareolus (49) which could also explain why the Microtus spp. in the current study were all negative for Bartonella spp. In our study, M. turbidus and P. silvatica were found significantly more often on My. glareolus compared to A. flavicollis which confirms that My. glareolus is the main reservoir host for M. turbidus (50). Moreover, the occurrence of P. silvatica is quite rare and known to occur on My. glareolus suggesting host specificity (51).
The Bartonella species detected in small mammals were almost identical compared to those obtained from fleas. However, half of the positive fleas were collected from negative small mammals. This observation indicates that the infection status of fleas can be independent from that of the current small mammal host. A previous study reported the vertical transmission in fleas which could explain how Bartonellae maintain in flea populations independently from a mammalian reservoir (52). Furthermore, frequent host changes by the fleas may have led to high infection levels. Only a few other studies report Bartonella prevalence in the examined flea species (15, 17). However, it should be considered that some of these flea species may parasitize companion animals such as cats and dogs (53) and thus may pose a health threat as direct vectors of zoonotic pathogens such as Bartonella spp. and Rickettsia spp.
To conclude, this study shows a high diversity of flea species on small mammal hosts from Germany. Though none of the detected vector-host species combinations was unusual, the number of flea species found was unexpectedly high. In addition to small mammals, some of them especially the ones collected at the urban site also parasitize companion animals such as dogs and cats and may pose a risk for the transmission of zoonotic Bartonella spp. Though B. grahamii was the only confirmed zoonotic Bartonella in this study, a very high variety of uncultured Bartonella spp. of yet unknown zoonotic potential was also detected. Especially at the urban study site, a health risk in encountering Bartonella infections is possible as infested rodents live there in close proximity to human settlements.
The datasets presented in this study can be found in online repositories. The names of the repository/repositories and accession number(s) can be found at: https://www.ncbi.nlm.nih.gov/genbank/, MT551048-MT551101; MT913158-MT913206.
CS and MP organized and planned the study. AO and MP organized and participated in the fieldwork for the collection of wildlife samples. MK and DK carried out the morphologic determination of fleas. AO prepared the samples in the laboratory. AO and NK tested the samples for Bartonella spp. AO, NK, and CS performed the sequence analysis. AO, NK, CS, and MP drafted the manuscript and wrote the final version. All authors read and approved the final manuscript.
This study was partially funded by EUgrant FP7-261504 EDENext (http://www.edenext.eu/). The Federal Environment Agency of Germany (FKZ 371148) also funded part of this project.
The contents of this publication are the sole responsibility of the authors and do not necessarily reflect the views of the European Commission.
The authors declare that the research was conducted in the absence of any commercial or financial relationships that could be construed as a potential conflict of interest.
We dedicate this work to the memory of Dr. Matthias Kiefer. Authors wish to thank Dana Rüster and Sarah Wagner for excellent technical assistance. Furthermore, the authors wish to thank Tim Tiedemann, Claudia Thiel, Dietlinde Woll, Anneliese Balling, Uwe Birett, Rayan Ababneh, Jennifer Krieg, Caroline Oltersdorf, Daniela Sum, Tessa Foerster, Franziska Eller, and Claudia Kehler for help in rodent trapping. The authors acknowledge support from the German Research Foundation (DFG) and Universität Leipzig within the program of Open Access Publishing.
1. Prutsky G, Domecq JP, Mori L, Bebko S, Matzumura M, Sabouni A, et al. Treatment outcomes of human bartonellosis: a systematic review and meta-analysis. Int J Infect Dis. (2013) 17:e811-9. doi: 10.1016/j.ijid.2013.02.016
2. Battisti JM, Lawyer PG, Minnick MF. Colonization of Lutzomyia verrucarum and Lutzomyia longipalpis Sand Flies (Diptera: Psychodidae) by Bartonella bacilliformis, the Etiologic Agent of Carrión's Disease. PLoS Negl Trop Dis. (2015) 9:e0004128. doi: 10.1371/journal.pntd.0004128
3. Bonilla DL, Kabeya H, Henn J, Kramer VL, Kosoy MY. Bartonella quintana in body lice and head lice from homeless persons, San Francisco, California, USA. Emerg Infect Dis. (2009) 15:912–5. doi: 10.3201/eid1506.090054
4. Dehio C, Sauder U, Hiestand R. Isolation of Bartonella schoenbuchensis from Lipoptena cervi, a blood-sucking arthropod causing deer ked dermatitis. J Clin Microbiol. (2004) 42:5320–3. doi: 10.1128/JCM.42.11.5320-5323.2004
5. Rolain J-M, Franc M, Davoust B, Raoult D. Molecular detection of Bartonella quintana, B. koehlerae, B. henselae, B. clarridgeiae, Rickettsia felis, and Wolbachia pipientis in cat fleas, France. Emerg Infect Dis. (2003) 9:339–42. doi: 10.3201/eid0903.020278
6. Harms A, Dehio C. Intruders below the radar: molecular pathogenesis of Bartonella spp. Clin Microbiol Rev. (2012) 25:42–78. doi: 10.1128/CMR.05009-11
7. Deng H, Le Rhun D, Buffet J-PR, Cotté V, Read A, Birtles RJ, et al. Strategies of exploitation of mammalian reservoirs by Bartonella species. Vet Res. (2012) 43:15. doi: 10.1186/1297-9716-43-15
8. Wagner A, Dehio C. Role of distinct type-IV-secretion systems and secreted effector sets in host adaptation by pathogenic Bartonella species. Cell Microbiol. (2019) 21:e13004. doi: 10.1111/cmi.13004
9. Gutiérrez R Krasnov B Morick D Gottlieb Y Khokhlova IS Harrus S. Bartonella infection in rodents and their flea ectoparasites: an overview. Vector Borne Zoonotic Dis. (2015) 15:27–39. doi: 10.1089/vbz.2014.1606
10. Paziewska A, Harris PD, Zwolińska L, Bajer A, Siński E. Differences in the ecology of Bartonella infections of Apodemus flavicollis and Myodes glareolus in a boreal forest. Parasitology. (2012) 139:881–93. doi: 10.1017/S0031182012000170
11. Welc-Faleciak R, Bajer A, Behnke JM, Siński E. The ecology of Bartonella spp. infections in two rodent communities in the Mazury Lake District region of Poland. Parasitology. (2010) 137:1069–77. doi: 10.1017/S0031182009992058
12. Andersson M, Råberg L. Wild rodents and novel human pathogen candidatus Neoehrlichia mikurensis, Southern Sweden. Emerg Infect Dis. (2011) 17:1716–8. doi: 10.3201/eid1709.101058
13. Buffet J-P, Marsot M, Vaumourin E, Gasqui P, Masséglia S, Marcheteau E, et al. Co-infection of Borrelia afzelii and Bartonella spp. in bank voles from a suburban forest. Compar Immunol Microbiol Infect Dis. (2012) 35:583–9. doi: 10.1016/j.cimid.2012.07.002
14. Telfer S, Clough HE, Birtles RJ, Bennett M, Carslake D, Helyar S, et al. Ecological differences and coexistence in a guild of microparasites: Bartonella in wild rodents. Ecology. (2007) 88:1841–9. doi: 10.1890/06-1004.1
15. Billeter SA, Levy MG, Chomel BB, Breitschwerdt EB. Vector transmission of Bartonella species with emphasis on the potential for tick transmission. Med Vet Entomol. (2008) 22:1–15. doi: 10.1111/j.1365-2915.2008.00713.x
16. Tsai Y-L, Chang C-C, Chuang S-T, Chomel BB. Bartonella species and their ectoparasites: selective host adaptation or strain selection between the vector and the mammalian host? Compar Immunol Microbiol Infect Dis. (2011) 34:299–314. doi: 10.1016/j.cimid.2011.04.005
17. Bown KJ, Bennet M, Begon M. Flea-borne Bartonella grahamii and Bartonella taylorii in bank voles. Emerg Infect Dis. (2004) 10:684–7. doi: 10.3201/eid1004.030455
18. Morick D Krasnov BR Khokhlova IS Gutiérrez R Gottlieb Y Harrus S. Vertical nontransovarial transmission of Bartonella in fleas. Mol Ecol. (2013) 22:4747–52. doi: 10.1111/mec.12408
19. Reeves WK, Rogers TE, Durden LA, Dasch GA. Association of Bartonella with the fleas (Siphonaptera) of rodents and bats using molecular techniques. J Vector Ecol. (2007) 32:118–22. doi: 10.3376/1081-1710(2007)32[118:AOBWTF]2.0.CO;2
20. Morick D Krasnov BR Khokhlova IS Shenbrot GI Kosoy MY Harrus S. Bartonella genotypes in fleas (insecta: siphonaptera) collected from rodents in the negev desert, Israel. Appl Environ Microbiol. (2010) 76:6864–9. doi: 10.1128/AEM.00879-10
21. Billeter SA, Gundi VA, Rood MP, Kosoy MY. Molecular detection and identification of Bartonella species in Xenopsylla cheopis fleas (Siphonaptera: Pulicidae) collected from Rattus norvegicus rats in Los Angeles, California. Appl Environ Microbiol. (2011) 77:7850–2. doi: 10.1128/AEM.06012-11
22. Marié J-L, Fournier P-E, Rolain J-M, Briolant S, Davoust B, Didier R. Molecular detection of Bartonella quintana, B. Elizabethae, B. Koehlerae, B. Doshiae, B. Taylorii, and Rickettsia felis in rodent fleas collected in Kabul, Afghanistan. Am J Trop Med Hyg. (2006) 74:436–9. doi: 10.4269/ajtmh.2006.74.436
23. Lewis RE. Notes on the geographical distribution and host preferences in the order Siphonaptera. 1. Pulicidae. J Med Entomol. (1972) 9:511–20. doi: 10.1093/jmedent/9.6.511
24. Silaghi C, Pfeffer M, Kiefer D, Kiefer M, Obiegala A. Bartonella, rodents, fleas and ticks: a molecular field study on host-vector-pathogen associations in Saxony, Eastern Germany. Microb Ecol. (2016) 72:965–74. doi: 10.1007/s00248-016-0787-8
25. Silaghi C, Woll D, Hamel D, Pfister K, Mahling M, Pfeffer M. Babesia spp. and Anaplasma phagocytophilum in questing ticks, ticks parasitizing rodents and the parasitized rodents–analyzing the host-pathogen-vector interface in a metropolitan area. Parasit Vectors. (2012) 5:191. doi: 10.1186/1756-3305-5-191
26. Obiegala A, Pfeffer M, Pfister K, Tiedemann T, Thiel C, Balling A, et al. Candidatus Neoehrlichia mikurensis and Anaplasma phagocytophilum: prevalences and investigations on a new transmission path in small mammals and ixodid ticks. Parasit Vectors. (2014) 7:563. doi: 10.1186/s13071-014-0563-x
27. Overzier E, Pfister K, Herb I, Mahling M, Böck G, Silaghi C. Detection of tick-borne pathogens in roe deer (Capreolus capreolus), in questing ticks (Ixodes ricinus), and in ticks infesting roe deer in southern Germany. Ticks Tick Borne Dis. (2013) 4:320–8. doi: 10.1016/j.ttbdis.2013.01.004
28. Overzier E, Pfister K, Thiel C, Herb I, Mahling M, Silaghi C. Diversity of Babesia and Rickettsia species in questing Ixodes ricinus: a longitudinal study in urban, pasture, and natural habitats. Vector Borne Zoonotic Dis. (2013) 13:559–64. doi: 10.1089/vbz.2012.1278
29. Angermann R, Senglaub K, Stresemann E (Editors). Wirbeltiere. Heidelberg: Spektrum Akademischer Verlag (2011).
30. Parson W K., Pegoraro K, Niederstätter H, Föger M, Steinlechner M. Species identification by means of the cytochrome b gene. Int J Leg Med. (2000) 114:23–8. doi: 10.1007/s004140000134
31. Peus F (Editor). Zur Kenntnis der Flöhe Deutschlands. Faunistik und Ökologie der Säugetierflöhe. Insectivora, Lagomorpha, Rodentia. Jena: Zoologische Jahrbücher für Systematik (1970).
32. Peus F (Editor). Zur Kenntnis Flöhe Deutschlands. – IV. Faunistik und Ökologie der Säugetierflöhe. Jena: Zoologische Jahrbücher für Systematik (1972).
33. Obiegala A, Pfeffer M, Pfister K, Karnath C, Silaghi C. Molecular examinations of Babesia microti in rodents and rodent-attached ticks from urban and sylvatic habitats in Germany. Ticks Tick Borne Dis. (2015) 6:445–9. doi: 10.1016/j.ttbdis.2015.03.005
34. Norman AF, Regnery R, Jameson P, Greene C, Krause DC. Differentiation of Bartonella-like isolates at the species level by PCR-restriction fragment length polymorphism in the citrate synthase gene. J Clin Microbiol. (1995) 33:1797–803.
35. Maggi RG, Diniz PP, Cadenas MB, Breitschwerdt EB (Editor). The use of molecular diagnostic techniques to detect Anaplasma, Bartonella and Ehrlichia species in arthropods or patients. The International Canine Vector-Borne Disease Symposium. Billesley: Alcester (2006).
36. Schorn S, Pfister K, Reulen H, Mahling M, Silaghi C. Occurrence of Babesia spp., Rickettsia spp. and Bartonella spp. in Ixodes ricinus in Bavarian public parks, Germany. Parasit Vectors (2011) 4:135. doi: 10.1186/1756-3305-4-135
37. Holmberg M, Mills JN, McGill S, Benjamin G, Ellis BA. Bartonella infection in sylvatic small mammals of central Sweden. Epidemiol Infect. (2003) 130:149–57. doi: 10.1017/s0950268802008075
38. Knap N, Duh D, Birtles R, Trilar T, Petrovec M, Avsic-Zupanc T. Molecular detection of Bartonella species infecting rodents in Slovenia. FEMS Immunol Med Microbiol. (2007) 50:45–50. doi: 10.1111/j.1574-695X.2007.00226.x
39. Birtles RJ, Harrison TG, Saunders NA, Molyneux DH. Proposals to unify the genera Grahamella and Bartonella, with descriptions of Bartonella talpae comb. nov., Bartonella peromysci comb. nov., and three new species, Bartonella grahamii sp. nov., Bartonella taylorii sp. nov., and Bartonella doshiae sp. nov. Int J Syst Bacteriol. (1995) 45:1–8. doi: 10.1099/00207713-45-1-1
40. Oksi J, Rantala S, Kilpinen S, Silvennoinen R, Vornanen M, Veikkolainen V, et al. Cat scratch disease caused by Bartonella grahamii in an immunocompromised patient. J Clin Microbiol. (2013) 51:2781–4. doi: 10.1128/JCM.00910-13
41. Maaz D, Krücken J, Blümke J, Richter D, McKay-Demeler J, Matuschka F-R, et al. Factors associated with diversity, quantity and zoonotic potential of ectoparasites on urban mice and voles. PLoS ONE. (2018) 13:e0199385. doi: 10.1371/journal.pone.0199385
42. Huitu O, Aaltonen K, Henttonen H, Hirvelä-Koski V, Forbes K, Perez-Vera C, et al. Field voles Microtus agrestis as reservoirs of Bartonella spp. EDENext. Montpellier (2015).
43. Obiegala A, Jeske K, Augustin M, Król N, Fischer S, Mertens-Scholz K, et al. Highly prevalent bartonellae and other vector-borne pathogens in small mammal species from the Czech Republic and Germany. Parasit Vectors. (2019) 12:1–8. doi: 10.1186/s13071-019-3576-7
44. Christou C, Psaroulaki A, Antoniou M, Toumazos P, Ioannou I, Mazeris A, et al. Rickettsia typhi and Rickettsia felis in Xenopsylla cheopis and Leptopsylla segnis Parasitizing Rats in Cyprus. Am J Trop Med Hyg. (2010) 83:1301–4. doi: 10.4269/ajtmh.2010.10-0118
45. Fairley JS. Fleas from the Fieldmouse Apodemus sylvaticus (L.) in Co. Down. Irish Nat J. (1963) 14:145–9.
46. Krasnov BR Shenbrot GI Khokhlova IS Allan Degen A. Relationship between host diversity and parasite diversity: flea assemblages on small mammals. J Biogeogr. (2004) 31:1857–66. doi: 10.1111/j.1365-2699.2004.01132.x
47. Noyes HA, Ambrose P, Barker F, Begon M, Bennet M, Bown KJ, et al. Host specificity of Trypanosoma (Herpetosoma) species: evidence that bank voles (Myodes glareolus) carry only one T. (H.) evotomys 18S rRNA genotype but wood mice (Apodemus sylvaticus) carry at least two polyphyletic parasites. Parasitology. (2002) 124:185–90. doi: 10.1017/s0031182001001019
48. Karbowiak G, Solarz K, Asman M, Wróblewski Z, Slivinska K, Werszko J. Phoresy of astigmatic mites on ticks and fleas in Poland. Biol. Lett. (2013) 50:89–96. doi: 10.2478/biolet-2013-0007
49. Krasnov BR, Stanko M, Miklisova D, Morand S. Habitat variation in species composition of flea assemblages on small mammals in central Europe. Ecol Res. (2006) 21:460–9. doi: 10.1007/s11284-005-0142-x
50. Vashchenok VS. Species composition, abundance, and annual cycles of fleas (Siphonaptera) on bank voles (Myodes glareolus) in the western part of Vologda Province (Babaevo District). Entmol Rev. (2014) 94:359–66. doi: 10.1134/S0013873814030087
51. Fairley JS. Epifauna from Irish bank voles Myodes glareolus schreber. Irish Nat J. (1970) 16:342–6.
52. Brinkerhoff RJ, Kabeya H, Inoue K, Bai Y, Maruyama S. Detection of multiple Bartonella species in digestive and reproductive tissues of fleas collected from sympatric mammals. ISME J. (2010) 4:955–8. doi: 10.1038/ismej.2010.22
53. Visser M, Rehbein S, Wiedemann C. Species of flea (siphonaptera) infesting pets and hedgehogs in Germany. J Vet Med B Infect Dis Vet Public Health. (2001) 48:197–202. doi: 10.1046/j.1439-0450.2001.00445.x
54. Birtles RJ, Hazel S, Bown K, Raoult D, Begon M, Bennett M. Subtyping of uncultured bartonellae using sequence comparison of 16 S/23 S rRNA intergenic spacer regions amplified directly from infected blood. Mol Cell Probes. (2000) 14:79–87. doi: 10.1006/mcpr.2000.0289
55. Kraljik J, Paziewska-Harris A, Miklisová D, Blanarová L, Mošanský L, Bona M, et al. Genetic diversity of Bartonella genotypes found in the striped field mouse (Apodemus agrarius) in Central Europe. Parasitology. (2016) 143:1437–42. doi: 10.1017/S0031182016000962
56. Špitalská E, Minichová L, Kocianová E, Škultéty L, Mahríková L, Hamšíková Z, et al. Diversity and prevalence of Bartonella species in small mammals from Slovakia, Central Europe. Parasitol Res. (2017) 116:3087–95. doi: 10.1007/s00436-017-5620-x
57. Mardosaite-Busaitiene D, Radzijevskaja J, Balčiauskas L, Bratchikov M, Jurgelevičius V, Paulauskas A. Prevalence and diversity of Bartonella species in small rodents from coastal and continental areas. Sci Rep. (2019) 9:12349. doi: 10.1038/s41598-019-48715-y
58. Berglund EC, Frank AC, Calteau A, Vinnere Pettersson O, Granberg F, Eriksson A-S, et al. Run-off replication of host-adaptability genes is associated with gene transfer agents in the genome of mouse-infecting Bartonella grahamii. PLoS Genet. (2009) 5:e1000546. doi: 10.1371/journal.pgen.1000546
Keywords: Apodemus flavicollis, Clethrionomys glareolus, Ctenophthalmus agyrtes, Megabothris turbidus, Bartonella grahamii, Europe
Citation: Obiegala A, Pfeffer M, Kiefer D, Kiefer M, Król N and Silaghi C (2021) Bartonella spp. in Small Mammals and Their Fleas in Differently Structured Habitats From Germany. Front. Vet. Sci. 7:625641. doi: 10.3389/fvets.2020.625641
Received: 03 November 2020; Accepted: 21 December 2020;
Published: 18 January 2021.
Edited by:
Serge Morand, Centre National de la Recherche Scientifique (CNRS), FranceReviewed by:
Michael Kosoy, KB One Health LLC, United StatesCopyright © 2021 Obiegala, Pfeffer, Kiefer, Kiefer, Król and Silaghi. This is an open-access article distributed under the terms of the Creative Commons Attribution License (CC BY). The use, distribution or reproduction in other forums is permitted, provided the original author(s) and the copyright owner(s) are credited and that the original publication in this journal is cited, in accordance with accepted academic practice. No use, distribution or reproduction is permitted which does not comply with these terms.
*Correspondence: Anna Obiegala, YW5uYS5vYmllZ2FsYUB2ZXRtZWQudW5pLWxlaXB6aWcuZGU=
Disclaimer: All claims expressed in this article are solely those of the authors and do not necessarily represent those of their affiliated organizations, or those of the publisher, the editors and the reviewers. Any product that may be evaluated in this article or claim that may be made by its manufacturer is not guaranteed or endorsed by the publisher.
Research integrity at Frontiers
Learn more about the work of our research integrity team to safeguard the quality of each article we publish.