- 1Oxford University Clinical Research Unit, Ho Chi Minh City, Vietnam
- 2Faculty of Animal Science and Veterinary Medicine, Nong Lam University, Ho Chi Minh City, Vietnam
- 3Sub-Department of Animal Health and Production, Cao Lãnh City, Vietnam
- 4Department of Veterinary Medicine, Can Tho University, Can Tho, Vietnam
- 5Faculty of Agriculture and Aquaculture, Dong Thap Community College, Cao Lãnh City, Vietnam
- 6Food and Agriculture Organization of the United Nations, Hanoi, Vietnam
- 7Food and Agriculture Organization of the United Nations, Jakarta, Indonesia
- 8Centre for Tropical Medicine and Global Health, University of Oxford, Oxford, United Kingdom
- 9Institute of Infection and Global Health, University of Liverpool, Liverpool, United Kingdom
Indiscriminate antimicrobial use (AMU) in animal production is a driver of antimicrobial resistance globally. There is a need to define sustainable interventions to reduce AMU in small-scale production systems, which currently represent the most widespread farming systems in South East Asia and many low- and middle-income countries. We conducted a before-and-after intervention study on a random sample of small-scale chicken farms in the Mekong Delta of Vietnam from 2016 to 2019. The study included a baseline followed by an intervention phase where farmers were provided with regular veterinary advice on flock health and husbandry, as well as antimicrobial replacement products. Of 102 recruited farms (raising >100 chickens per flock cycle), thirty-five (34.2%) entered the intervention phase, whilst the rest stopped raising chickens, mainly due to suboptimal flock performance. Through the implementation of our intervention, chicken flocks reduced levels of AMU by 66% [adjusted hazard ratio (HR) = 0.34; p = 0.002) from a baseline of 343.4 Animal Daily Doses per 1,000 chicken-days and decreased weekly mortality by 40% (adjusted HR = 0.60; p = 0.005) from a baseline mortality of 1.60 per 100 birds. Chicken bodyweight increased by 100 g (p = 0.002) in intervention flocks. Our findings demonstrate that the provision of veterinary advice can achieve substantial reductions in AMU in small-scale production systems without compromising flock health and productivity.
Introduction
In many low- and middle-income countries (LMICs) small-scale poultry farming plays a crucial role in supporting the livelihoods of rural communities (1). Compared with other species, poultry production has relatively low investment and production costs (2). Globally, poultry (mainly chicken) is the second most consumed type of meat (117 million tons in 2017), and by 2026 it is expected to surpass pork (3).
Antimicrobial use (AMU) in animal production has been recognized as a driver of antimicrobial resistance (AMR) globally (4, 5). In terms of frequency, chickens are the target of the highest AMU levels of all animal food species (6). In addition, many antimicrobial active ingredients (AAI) regarded as critically important for human medicine by the World Health Organization (7) are often used in chicken production (8).
In Vietnam, it has been estimated that three quarters (72%) of all AMU (3,842 tons in 2015) are aimed at animal production (9). Studies in the Mekong Delta region of Vietnam have described very high amounts of antimicrobial to small-scale chicken flocks (8, 10–12). The high levels of disease in flocks in the area is a major driver of AMU in such systems (13). In chicken farms, antimicrobials are used primarily for disease prevention (10), since farmers regard them as a cheaper alternative to other disease control measures (14). Recent studies have shown that some of the most commonly used AAIs in small-scale chicken flocks in the area also belong to the WHO highest priority, critically important antimicrobial classes such as polymyxins and fluoroquinolones (8, 12, 15). This situation is aggravated by a general lack of awareness about antimicrobials and the negative consequences of AMR among farmers (16). In addition, the ease of access to antimicrobials over-the-counter in veterinary drug shops (17) and their affordability (18) are factors that contribute to excessive AMU in Vietnam.
There is a pressing need to identify sustainable interventions that reduce AMU in food animal production systems. Such interventions will need to overcome the diversity of production systems and value chains they depend on and the patterns of AMU in these systems and their policy contexts. A number of interventions have already taken place in developed countries based on improvements in biosecurity and husbandry practices aiming at reducing AMU in pigs (19–21) and broilers (22). However, no intervention studies targetting AMU in small-scale farming systems from LMICs have been published. We conducted a “before-and-after” randomized intervention study on small-scale chicken farms in the Mekong Delta region of Vietnam. The intervention consisted of providing farmers with regular veterinary advice, alongside antimicrobial replacement products (23). The aim was to investigate the impact of this intervention on AMU, as well as on flock disease and productivity. Results and the lessons from this study can be adapted to comparable animal production systems in Vietnam and more generally, to other LMICs.
Materials and Methods
Study Design
The intervention was designed as a randomized “before-and-after” controlled study on small-scale farms raising chickens for meat in two districts (Cao Lanh and Thap Muoi) within Dong Thap province (Mekong Delta, Vietnam) (Figure 1 and Supplementary Figure 1). We aimed to recruit farmers raising chicken flocks (defined as a group of birds raised together in the same building) meeting the criterion “>100 meat chickens raised as single age.”
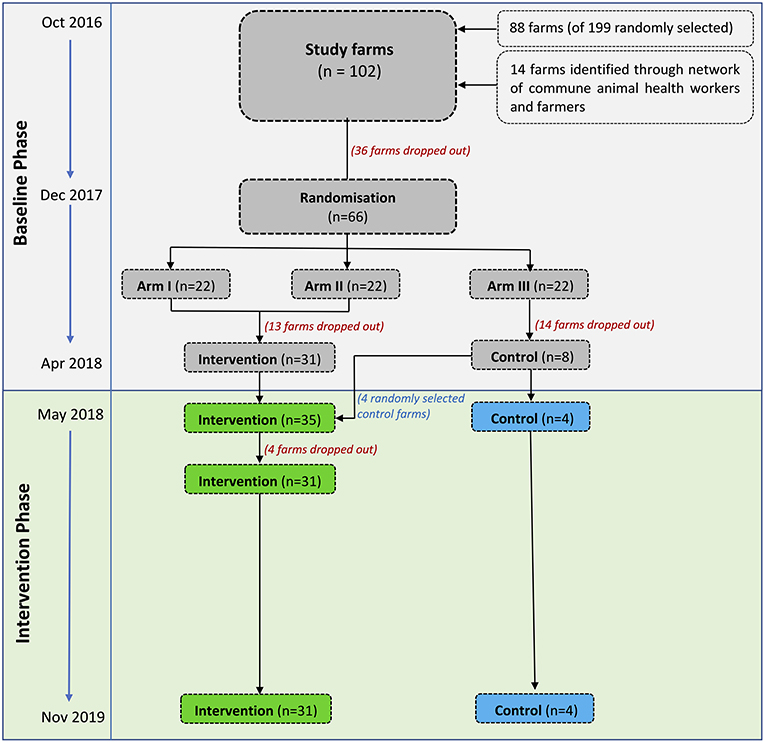
Figure 1. Recruitment and follow-up of study farms. Modified from design of project named ViParc published in 2017 (23).
Our small-scale commercial flocks lie between “backyard” flocks and intensively managed “industrial” systems, roughly corresponding to FAO Sectors 2 and 3 (between 50 and 2,000 birds, with feed and water supplied to the birds) (24). The study was designed in two stages, a “baseline” followed by an “intervention” phase. Two intervention arms (Arm 1 and Arm 2) were initially planned, both including the provision of training and advice to farmers, as well as a control arm (Arm 3) (no training or advice). The difference between both intervention arms was that Arm 2 also included the withdrawal of medicated commercial feed. This aimed at investigating whether restriction of medicated feed might have affected disease outcomes, therefore contributing to changes in levels of AMU (23).
Farmers registered in the official SDAH census (2014) were contacted by post and were invited to participate in an introductory meeting held in October 2016 in each of the two study districts. In these meetings, the project aims and methods were outlined. Farmers willing to enroll in the study were asked to contact project staff as soon as they restocked with day-old chicks.
Description of the Baseline and the Intervention
During the baseline phase of the study, routine AMU and productivity data were collected from enrolled farms without the provision of any advice. Using a random number generator, we allocated enrolled farms to either an intervention or a control arm. All farms allocated to the intervention arm were supported with a Farmer Training Programme (FTP), where farm owners were invited to participate in six workshops where a poultry veterinarian instructed them on the principles of chicken husbandry, prevention, control of infectious diseases and waste management and a Farm Health Plan (FHP), where each farm was assigned to a Project Veterinarian (PV) who was responsible for providing specific advice to farmers. The PV visited each farm on three different occasions for each flock cycle: (i) early-brooding (weeks 1–2), (ii) late brooding (weeks 3–4), and (iii) grow-out (>2 months) periods. Prior to each visit, the PV reviewed records of productivity and disease over previous cycles, inspected the flock and house/pen, reviewed farmers' records, discussed with farm owner about current production/health issues, and then drafted a list of recommendations to address them. In addition, the PV recommended the farm owner to use an antimicrobial replacement product, either a liquid phytogenic solution containing essential oils (Product A) or a yeast fraction-containing product (Product B) for 3 days a week over the first 10 weeks of the production cycle. Product A was recommended to flocks with a history of diarrhoeal disease in previous cycles; for all other flocks the PV recommended Product B. By providing these products we aimed at allaying the farmers' anxiety about reducing or eliminating antimicrobials during the early phase of production, which is critical in terms of disease and mortality. In all visits, the PVs reminded the farmers that healthy birds should not be given any antimicrobials.
Data Collection
Each farmer was provided by project staff with a diary to weekly record data on farming practices, including number of chickens purchased, number of chickens in and out of the flock (number of dead and sold chickens), as well as the types and quantities of antimicrobial products used. The average bodyweight of slaughter-age chickens was also measured by average of total bodyweight of chickens divided for total number of chickens sold. Project staff visited study farms four times (different from PV visits) to verify the data collected, which was subsequently transferred to validated questionnaires and double-entered into a web-based database.
Statistical Analyses
The initially proposed sample size was based on previous quantitative data on AMU in Mekong Delta chicken farms (10). We aimed at recruiting 120 farms and estimated a total of 40 farms for each arm. A sample size of 40 farms per arm, each contributing with 2 cycles investigated during baseline and 2 during the intervention, and a two-sided significance level of 5%, will have 82% power to detect a ~33% reduction, and a 91% power to detect a 50% reduction. Since the study design exploited within-farm correlation of unknown magnitude, the true power was expected to be higher.
The primary outcome was the weekly number doses of antimicrobial active ingredient (AAI) corresponding to 1 kg of live chicken administered to a flock (Weekly ADDkg). Secondary outcomes were “Weekly mortality,” calculated by dividing the number of chickens dying over the week by the total chicken present at the beginning of each week (%), and “Weight of the birds (in units of 100 g) at the time of sale.” The latter was calculated by dividing the total flock weight by the number of chickens sold at the end of the cycle. The correlation between all three outcomes at flock and at week level was investigated using the Spearman's rank correlation coefficient.
Weekly antimicrobial consumption in each flock was expressed as No. of Animal Daily Doses-kilogram (ADDkg). ADDkg was calculated for each antimicrobial contained in each product based on the preparation instructions included in its technical dossier/label. The amounts of antimicrobial product administered each week were multiplied by a dilution factor [for water-administered products, Volume of water (ml)/Weight of product (g); for feed feed administered products, Weight of feed (g)/Weight of product (g)]. The obtained amounts were then divided by the estimated weekly water of feed consumption corresponding to a 1 kg chicken (7*0.225 l of water, 7*0.063 kg of feed). The number of ADDkg per 1,000 kg chicken-days on any given week was calculated by dividing the total of number of ADDkg by the estimated weight of the flock at a given week, and then multiplied by 1,000. The estimated total weight of the flock by week was calculated by multiplying the total number of chickens at the beginning of the week by their estimated weight. The No. ADDkg per 1,000 chicken-days can be interpreted as the number of days (per 1,000) when one chicken is treated or the number of treated chickens daily (per 1,000) (8).
We built Poisson regression models with for “Weekly ADDkg” and “Weekly mortality.” For the former the offset was the (weekly) total number of chicken-kg days (log); for the latter it was the number of chickens at the beginning of the week (log). In addition, a linear regression model was developed with bodyweight of chickens at the point of sale (kg) as outcome. In all cases, “Farm,” “Flock cycle” and “Week” were modeled as random effects, where “Week” was nested within “Flock cycle,” and the latter was nested within “Farm.” The main variable of interest was the impact of the intervention delivered; therefore, we investigated “Status” (baseline, transition, and intervention) as an explanatory variable in Intervention Arm and “Status” (baseline, intervention calendar time) as an explanatory variable in the Control Arm. “Status = transition” was assigned to those flocks that were not exposed to all three advisory visits for Intervention Arm farms. This occurred to a number of flocks at the beginning of the intervention phase, given that some advisory visits (typically the first and second) were missed. In order to account for the potential confounding effects of “District” and “Flock size” these were forced into a multivariable model; we tested the interactions between “Status = intervention” with “District” and “Flock size” to investigate whether the observed effects were dependent on the geographical location or the size of the flock. Moreover, we investigated whether subsequent cycles over the intervention resulted in improved outcomes by splitting “Status = intervention” into “Status = first intervention cycle” and “Status = subsequent intervention cycle.” The presence of overly influential observations was investigated by testing the model with and without those observations yielding the largest residuals. We used the “survey” package to calculate (farm-flock-week) adjusted estimates and “lme4” package to build statistical models (http://www.r-project.org).
Results
Recruitment of Study Farms
The study took place between October 2016 and November 2019. A meeting with 199 randomly selected farmers from the farm census registered as owners of chickens was held in October 2016. Eighty-eight participating farmers indicating their willingness to restock within 6 months were enrolled. The remaining 14 farms were identified by commune animal health workers or through contact with farmers that had already been enrolled in the study. Therefore, a total of 102 farms were enrolled over the period October 2016 to October 2017. The baseline phase spanned October 2016 to April 2018. The intervention was delivered from May 2018 to November 2019.
The flow of participating farms was complicated by many (n = 63) that stopped farming during the study for financial reasons unrelated to the study. The recruitment and allocation to arms is summarized in Figure 1. Their location is presented in Supplementary Figure 1.
In December 2017 farms that remained in production at the time (n = 66) were randomized to either intervention Arm 1 (n = 22), intervention Arm 2 (n = 22) or a Control arm (n = 22). Following discussion with the farmers, it became apparent that replacement of medicated feed as initially planned for Arm 2 would not be acceptable; therefore, the two intervention arms were merged into one single arm. At the time of the onset of the intervention (May 2018), of 44 farms initially allocated to the intervention, only 31 remained in business; of the 22 allocated to the control, only 8 were still raising chickens. To compensate for the reduced sample size and associated loss in study power, we further allocated four randomly-selected control farms to the intervention arm. Therefore, a total of 35 and 4 farms allocated to the intervention and control arm, respectively, proceeded to the intervention phase (Figure 1).
The intervention commenced with the delivery of the Farmer Training Programme (FTP) in May 2018 to owners of the 35 intervention farms; however, at that time 18 had already restocked with day-olds. Since flocks (n = 22) in these farms were not exposed to all four advisory visits, they were therefore analyzed as “transition” flocks. Four farms assigned to the intervention arm stopped raising chickens shortly after having attended the FTP modules, and were classified as “Baseline-Transition-Stop” farms.
Data collected from 35 farms (31 intervention, 4 control) were eligible for the final analyses. One hundred flock cycles were analyzed as baseline phase (87 in intervention; 13 in control arms) and 89 flock cycles corresponded to the intervention phase (77 intervention farms; 12 in control farms). Of the 77 flocks, 28 (14 farms) were given Product A (an essential oil); and 43 (14 farms) were given Product B (a yeast fraction-based product). Six flocks (3 farms) did not agree with the supplementation of either Product A or Product B.
The median number of chickens restocked per flock was 303 [IQR (inter-quartile range) 200–500], and the median duration of one production cycle was 18 weeks (IQR 16.0–20.0). Each farm raised a median of 5 flocks (IQR 4.0–7.0), 2 (IQR 1.0–2.2) during the baseline and 2 (IQR 1.0–2.5) during the intervention phase. Details of number of flocks per farm and status are shown in Table 1 and Figure 2. Descriptive characteristics of chicken farms by total farms, flocks and weeks were presented in Supplementary Table 1.
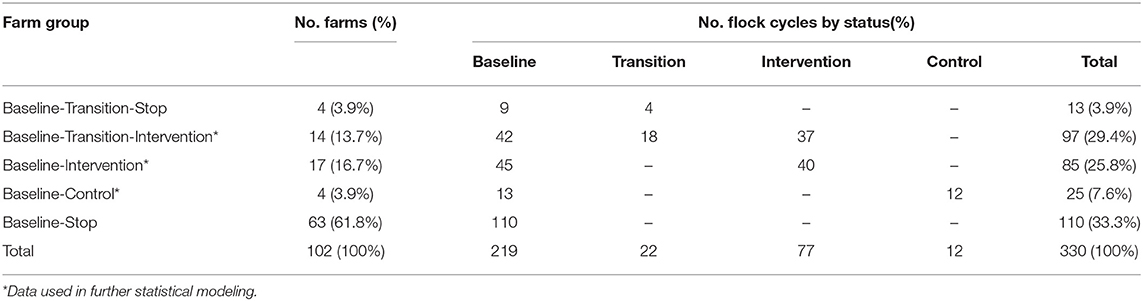
Table 1. Categorization of farms based on number of flocks investigated during the baseline phase, transition period, and intervention phase.
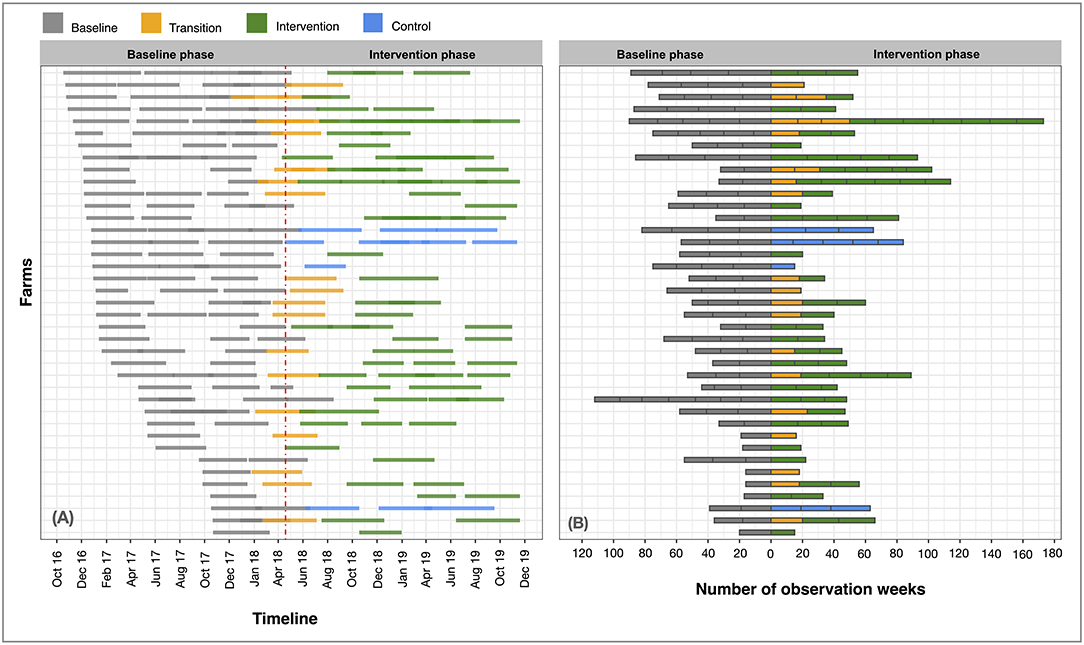
Figure 2. Calendar and observation time of the 39 farms recruited during the baseline and intervention phases. These included: Baseline-Transition-Stop farms (n = 4), Baseline-Control farm (n = 4), Baseline-Transition-Intervention (n = 14) and Baseline-Intervention farms (n = 17). A total of 63 farms (61.8% of recruited farms) stopped raising chickens and are not displayed in the graphs.
AMU, Mortality, and Bodyweight of Chicken Flocks
We collected data over 5,872 production-weeks for all study flocks combined; of which 3,899 (66.4%) corresponded to the baseline and 1,973 (33.6%) to the intervention phase. The latter included 396 (6.7%) weeks from transition flocks, 1,350 (23.0%) weeks of full intervention flocks, and 277 (3.9%) weeks from flocks allocated to the control arm. Data on AMU, mortality and bodyweight in these flocks over the baseline, transition and intervention cycles are presented in Table 2.
During the baseline phase, flocks (n = 110) raised in the 63 farms that dropped out prior to the implementation of the intervention phase had a higher mortality (weekly average 3.18 per 100 birds; SE ± 0.3), than flocks (n = 109) in 39 farms that proceeded to the intervention (1.52 per 100 birds; SE ± 0.1) (Wilcoxon Test, p = 0.020).
The weekly summary data of the outcome variables and the distribution of flocks concerning these in flocks during the baseline (n = 87) and intervention phases (n = 77) are displayed in Figure 3. Weekly AMU in these flocks was reduced from 343.4 (SE ± 33.5) (baseline) to 223.9 (SE ± 30.0) (intervention) Animal Daily Doses (ADDkg) per 1,000 kg chicken-days (−34.8%) (one-sided Wilcoxon test, p < 0.001). The bodyweight at slaughter-age of chickens of intervention flocks was 1,670 g (SE ± 30), compared with 1,560 g (SE ± 20) during baseline (+7.1%) (one-sided Wilcoxon test, p = 0.006). However, weekly mortality increased from 1.60 (per 100 birds) (SE ± 0.2) to 1.64 (SE ± 0.2) (+2.4%), although the difference was not significant (one-sided Wilcoxon test, p = 0.999).
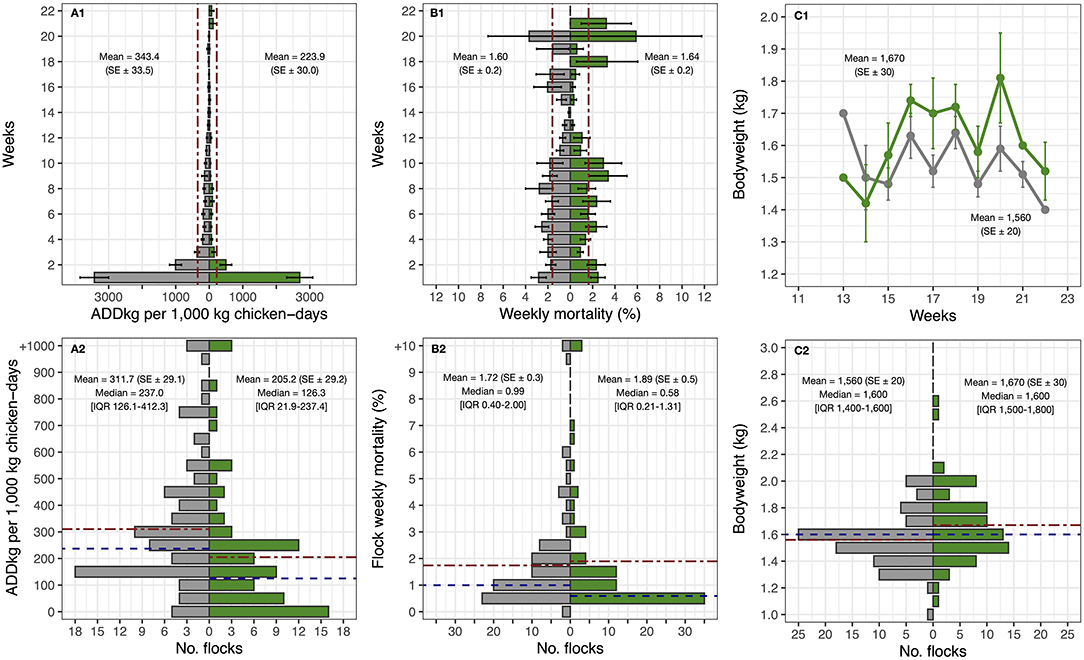
Figure 3. Comparisons between AMU, mortality and bodyweight by weeks and flocks between baseline (in gray color) and intervention phase (in green color) in 31 eligible farms. Dot-dash red line: Mean. Dashed blue line: Median.
The unadjusted overall mortality increased slightly during the intervention. However, the number of farms that experienced a reduction in mortality exceeded (19/31) than those that increasing it (12/31). The changes in (flock average) values of ADDkg per 1,000 kg chicken-days, mortality and bodyweight between the baseline and intervention phases are displayed in Figure 4. Among intervention flocks, there were 3/77 (3.9%) with an average weekly mortality greater than 12% (12.8, 24.8 and to 26.0%) and a cumulative mortality of >98%; two of these flocks were detected with Highly Pathogenic Avian Influenza (HPAI) and one with Avibacterium paragallinarum, compared with 2/87 flocks experiencing >10% weekly mortality among baseline flocks (one 12.4% and one 12.8%) and cumulative mortality of 100% in these two baseline flocks.
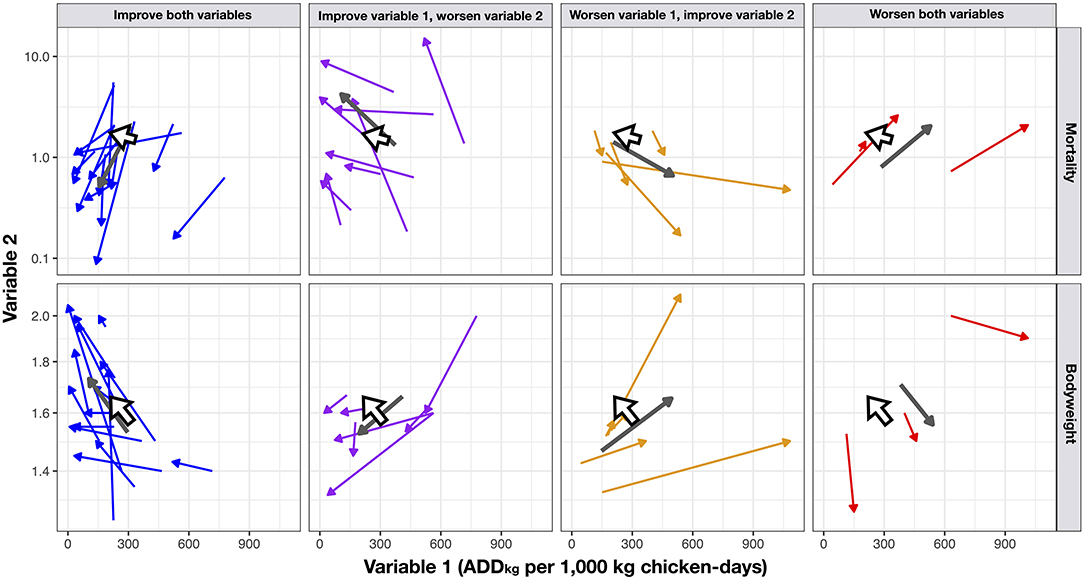
Figure 4. Bi-dimensional arrow charts showing crude (unadjusted) changes between the baseline and the intervention phases with regards to Variable 1 (No. ADDkg per 1,000 kg chicken-days) and Variable 2 (mortality and bodyweight). The arrow indicates the direction of change. Large gray arrows indicate summary result for each pair of variables. Large white arrows indicate overall results. The log10-transformed scale for easier visualization.
In the four farms that were allocated to the control arm, a total of 13 flocks were investigated during the baseline phase, and 12 during the intervention phase. AMU in these decreased from 216.8 (SE ± 71.8) to 182.5 (SE ± 56.3) (Wilcoxon Test, p = 0.857); weekly mortality changed from 1.17 to 1.29 per 100 birds (Wilcoxon test, p = 0.493) and bodyweight changed from 1,680 to 1,600 g (Wilcoxon test, p = 0.511).
Correlation Between AMU and Mortality, Bodyweight
There were significant correlations between weekly AMU (ADDkg per 1,000 kg chicken-days) and mortality (Spearman's rank correlation R = 0.26; p < 0.001). There were, however, no correlations between average bodyweight and AMU (R = −0.06, p = 0.292) or mortality (R = −0.09, p = 0.128) at flock level. The details of these calculations are provided in Supplementary Figure 2.
Modeling
The statistical models investigating the effectiveness of the intervention on AMU, mortality and chicken bodyweight are presented in Table 3. In the univariable models for the Intervention Arm, “Status = intervention” was associated with an overall decreased AMU (HR = 0.33; 95% CI = 0.17–0.65; p = 0.001) (−67%), decreased mortality (HR = 0.57; 95% CI = 0.40–0.82; p = 0.002) (−43%) and increased bodyweight (+100 g; 95% CI 37–164 p = 0.002). The size of the flock was negatively associated with AMU (HR = 0.55, 95% CI 0.36–0.85, p = 0.007), but positively associated with mortality (HR = 1.34; 95% CI 1.02–1.47; p = 0.032). Adjustment for flock size resulted in minimal change in the estimates of AMU (−66%) (HR = 0.34; 95% CI 0.18–0.66; p = 0.002), mortality (−40%) (HR = 0.60; 95% CI 0.41–0.86; p = 0.005) and bodyweight (+100 g; 95% CI 37–164 g; p = 0.002). When the variable level “status = intervention” was replaced by two new variables (first, subsequent cycles), greater reductions in AMU were seen in the subsequent (HR = 0.26, p = 0.003) compared with the first cycle (HR = 0.46; p = 0.066), although the difference between both was not statistically significant (p = 0.298). Similarly, chicken bodyweight further increased during subsequent intervention cycles (+119 g per chicken sold, p = 0.003) compared with the first intervention cycle (+77 g, p = 0.068). However, there was no statistical significance in chicken bodyweight between first and subsequent intervention cycles (p = 0.378). Levels of mortality did not change between first and subsequent cycles (p = 0.967). There were no significant interactions between either “flock size” and “district” and “Status = intervention.” There was no statistical difference in AMU and mortality between flocks using Product A, Product B or those given no additional product. However, flocks that were administered with either Product A and B had increased bodyweight compared with flocks not given any supplementary product (data not shown). Data from these flocks were kept in the final models after confirming that their removal did not change model coefficients to a large degree: AMU reduced from HR = 0.34 including them compared with HR = 0.33 when excluded. With regards to mortality, the removal of these observations resulted in HR = 0.53 compared with HR = 0.60 obtained with the whole dataset.
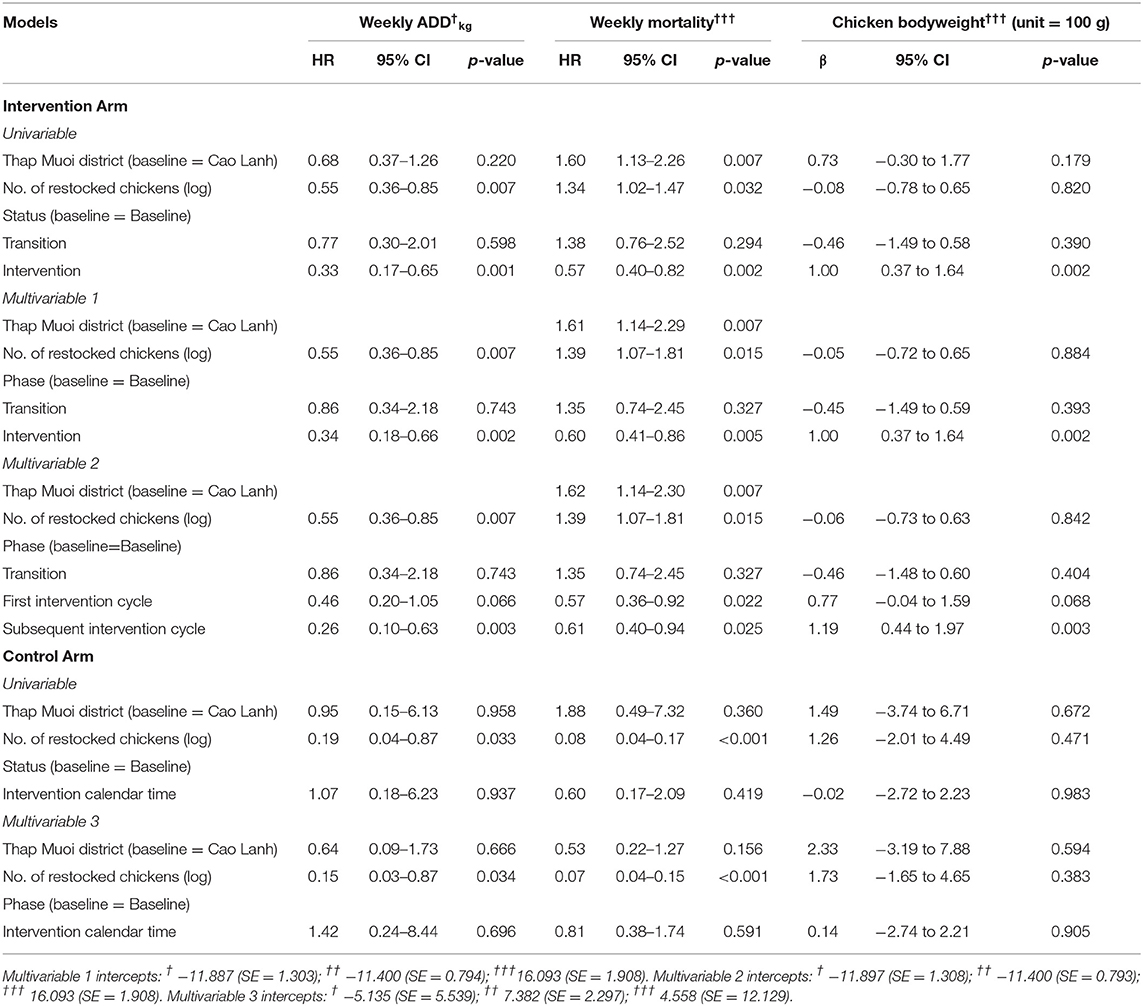
Table 3. Mixed regression models investigating the effectiveness of the intervention on AMU, mortality, and chicken bodyweight.
In the control arm, there were no significantly associations between “Status = intervention calendar time” and any of the three outcome variables in either univariable or multivariable models (all p > 0.419). After adjustment of flock size and study district in multivariable models, estimates of AMU and bodyweight increased (+42% and +14 g, respectively) and mortality was reduced (−19%).
Discussion
Through a locally delivered veterinary intervention, we achieved a 66% reduction in antimicrobials (quantified as daily doses) administered to small-scale commercial chicken flocks, alongside a reduction in mortality (−40%). In our crude (unadjusted) analyses AMU reductions were, however, modest (−35%), since our analysis implicitly adjust for week of use and most AMU took place during the early weeks (i.e., the brooding period). Similarly, the crude data indicated a slightly higher mortality during the intervention (+2.4%). However, the adjusted analysis indicated a ~40% reduction in mortality, and weekly mortality was reduced in a majority (19/31, 61.3%) of farms. This discrepancy was explained by unusually high mortality in three intervention flocks.
Unlike other studies involving the delivery of a uniform treatment (i.e., vaccination) (25), our intervention consisted of providing farmers with veterinary advice. The nature of this advice was variable across farms, and was based on specific observations and information collected by project veterinarians from their flocks. This advice included measures to improve flock health and productivity, whilst emphasizing the message that “antimicrobials should not be admnistered to healthy chickens.”
In addition to providing antimicrobial replacement products, the main advice given to farmers focused on biosecurity, cleaning and disinfection, vaccination, litter management, and administration of medicines (including antimicrobials, antiparasitic drugs and other health-enhancing products). The detail of this advice provided and its uptake will be presented elsewhere. The advice provided was based on a persuasive, rather than a restrictive advice. We believe this approach is likely to be more sustainable in the mid-to-long term (26). A similar holistic approach was adopted on a study on pig farms in Belgium, resulting in 52% AMU reduction in pigs raised from birth to slaughter, and by 32% among breeding animals; furthermore, the study resulted in additional productivity gains (20). Similarly, a study conducted in four European Union (EU) countries reported AMU reductions of 3 and 54% in fattening and weaned pigs, respectively, following improvements of herd management practices (19). However, reductions in AMU were not seen in breeding pigs, and the authors attributed it to the concurrent incursion of Porcine Epidemic Diarrhea (PED) in Germany. A study in 20 industrial-scale broiler farms in Europe using a holistic approach resulted in 20% reductions in levels of AMU and 14% increase in gross margins (22).
After consultation with participating farmers during the baseline phase, we were compelled to modify our original protocol by offering selected health-enhancing, antimicrobial replacement products (27) to chicks during the brooding phase. This aimed at allaying the farmers' anxiety about reducing or eliminating antimicrobials during this critical phase of production. Administration of antimicrobials during the brooding phase is standard practice and many antimicrobial-containing commercial formulations are marketed as “brooding medicine” (13). Similarly, many of our study farmers expressed their opposition about changing the feed and therefore we consolidated the two intervention arms into only one arm. Often the advice provided by project veterinarians to farmers was overrun by that given at local veterinary drug shops. Farmers often visit these shops to buy animal feed and other supplies (17). In addition, the antimicrobial product labels often include indications for prophylactic use at a lower dose (28).
Small-scale commercial chicken production using native breeds is widespread in the Mekong Delta of Vietnam, and often represents an upgrade from backyard production. The popularity of this system resides in the preference of the Vietnamese consumer for meat of long-cycle native birds. Native chicken meat reaches a considerably higher price compared with broiler meat (29). However native chickens (and their crosses) are slow growing (>4 months), and preventing disease over such a prolonged period requires sustained efforts (13).
In our study, the identification and enrollment of study farms was challenging due to the fluidity of this type of production system, with many households setting up chicken farms as well as stopping raising chickens altogether. Because of this, a large number of farms did not remain in business over the extended duration of the study. Indeed, flock mortality was an important predictor for farmers giving up raising chickens (data not shown) and a large fraction of our study farms (61.8%) had gone out of business even before the start of the planned intervention phase.
In addition to their previous experience with disease, farmers may start or stop raising chickens depending on circumstances, such as market price of day-olds, commercial feed and poultry meat, income from the sale of the previous flocks or other rural activities. Furthermore, many farmers raised one cycle per year, but not necessarily every year. This was reflected in the lack of experience in chicken husbandry of many farmers (and farm workers). This represents a hurdle for the implementation of correct management practices. This contrasts with a recent study in Belgium, where pig farmers had on average 22.6 years of experience (20). In this context, often antimicrobials are used as replacement of other, most costly, but demanding husbandry practices (14). The incursion of African Swine Fever (ASF) in Vietnam in January 2019 and its spread within the country (30) coincided with the intervention phase in this study. This may have exerted additional pressures over our study farmers. During this time, many farms in ASF-affected provinces switched to chicken production, resulting in increased market availability of low-cost chicken meat, therefore reducing the value of chicken production in our area.
The changes to the initial study design are a testament to the challenges of conducting intervention studies in small-scale farming systems. Initially, we planned to allocate one third of all recruited farms to the control arm in order to measure any environmental influences on AMU, for example, due to public engagement initiatives (television campaigns, work in schools, etc.) that took place in the province under the umbrella of this project. Exposure to these may have inadvertently had an influence on the farmers' decision on AMU beyond the intervention. Given the high number of farms that stopped chicken production, we opted for reducing the size of the control arm to a minimum of four, thus reducing the statistical power of any analysis in that group. However, the descriptive data from this small control group suggests no change between baseline and intervention, and gives additional validity of the observed findings.
The study demonstrates that reducing current high levels of AMU through the provision of veterinary advice is achievable in the Vietnamese small-scale commercial farming context. There was an indication that farmers responded to the advice given. Many farmers, especially the larger ones may even be willing to pay for such a service, since labor costs in Vietnam are relatively low (~25 USD for a 2-h visit). We believe that results and lessons from this study can be adapted other LMICs where small-scale animal production systems are common. However, the usefulness of this approach for with regards to intensive farming systems requires further investigation, since such systems already have their own technical advisory services. Sustainable, long-term reductions in AMU could be reached if links between veterinarians/animal health workers and farmers are built and reinforced. Supplementation with health-enhancing products may be beneficial, but this needs to be further explored. We propose to develop a business case for an advisory service targeting the main livestock-producing regions in the country (Mekong River Delta, Southeast, Central region, Red River Delta), with the value proposition that healthy livestock means profitable businesses.
Data Availability Statement
The raw data supporting the conclusions of this article will be made available by the authors, without undue reservation.
Ethics Statement
The studies involving human participants were reviewed and approved by Oxford Tropical Research Ethics Committee (OxTREC). The patients/participants provided their written informed consent to participate in this study. The animal study was reviewed and approved by Oxford Tropical Research Ethics Committee (OxTREC). Written informed consent was obtained from the owners for the participation of their animals in this study. Written informed consent was obtained from the individual(s) for the publication of any potentially identifiable images or data included in this article. The study has been granted ethics approval by the Oxford Tropical Research Ethics Committee (OXTREC) (Ref. 5121/16).
Author Contributions
JC-M, JR, and NC conceived and designed study. DP, NC, and DT conducted field survey. BK and VH designed and aided data collection. HT, LY, NM, and ES aided intervention packages. DP, JC-M, NC, and DT contributed to data analyses. DP, NC, JC-M, PP, JR, and GT contributed to writing up and editing the manuscript. All authors contributed to the article and approved the submitted version.
Funding
This work was funded by the Wellcome Trust through an Intermediate Clinical Fellowship awarded to JC-M (Grant Reference Number 110085/Z/15/Z).
Conflict of Interest
The authors declare that the research was conducted in the absence of any commercial or financial relationships that could be construed as a potential conflict of interest.
Acknowledgments
The authors would like to thank all farmer participants, the staff affiliated to the Dong Thap Sub-Department of Animal Health, Production and Aquaculture, the staff at Agricultural Service Center of Cao Lanh and Thap Muoi district, Dong Thap province for their help and support. This manuscript has been released as a pre-print at: https://www.biorxiv.org/content/10.1101/2020.09.13.295659v1 (31).
Supplementary Material
The Supplementary Material for this article can be found online at: https://www.frontiersin.org/articles/10.3389/fvets.2020.612993/full#supplementary-material
Supplementary Figure 1. Study area in the province of Dong Thap, Mekong Delta of Vietnam.
Supplementary Figure 2. Correlation between AMU, mortality and bodyweight by week (left block) and by flock (right block). The data have been log-transformed scale for easier visualization.
Supplementary Table 1. Descriptive characteristics of chicken farms by total of farms, flocks, and weeks.
Supplementary Data 1. Raw data for calculation of ADDkg per 1,000 kg chicken-days.
Supplementary Data 2. Raw data on AMU, mortality, and bodyweight.
References
1. Wong JT, de Bruyn J, Bagnol B, Grieve H, Li M, Pym R, et al. Small-scale poultry and food security in resource-poor settings: a review. Glob Food Secur. (2017) 1:43–52. doi: 10.1016/j.gfs.2017.04.003
2. Hilmi M, Dolberg F, Clarke B. Products and Profit From Poultry. 2nd ed. Rome: Rural Infrastructure and Agro-Industries Division, Food and Agriculture Organization of the United Nations (2011).
3. OECD Food and Agriculture Organization of the United Nations. OECD-FAO Agricultural Outlook 2017-2026. Paris: OECD (2017).
4. Marshall BM, Levy SB. Food animals and antimicrobials: impacts on human health. Clin Microbiol Rev. (2011) 2:718–33. doi: 10.1128/CMR.00002-11
5. O'neill J. Antimicrobials in Agriculture and the Environment: Reducing Unnecessary Use and Waste. (2015). Available online at: https://amr-review.org/sites/default/files/Antimicrobials%20in%20agriculture%20and%20the%20environment%20-%20Reducing%20unnecessary%20use%20and%20waste.pdf (accessed June 23, 2020).
6. Cuong N, Padungtod P, Thwaites G, Carrique-Mas J. Antimicrobial usage in animal production: a review of the literature with a focus on low- and middle-income countries. Antibiotics. (2018) 7:75. doi: 10.3390/antibiotics7030075
7. WHO. Critically Important Antimicrobials for Human Medicine, 6th Revision. (2019). Available online at: https://www.who.int/foodsafety/publications/antimicrobials-sixth/en/ (accessed April 28, 2020).
8. Cuong NV, Phu DH, Van NTB, Dinh Truong B, Kiet BT, Hien BV, et al. High-resolution monitoring of antimicrobial consumption in vietnamese small-scale chicken farms highlights discrepancies between study metrics. Front Vet Sci. (2019) 6:174. doi: 10.3389/fvets.2019.00174
9. Carrique-Mas JJ, Choisy M, Van Cuong N, Thwaites G, Baker S. An estimation of total antimicrobial usage in humans and animals in Vietnam. Antimicrob Resist Infect Control. (2020) 9:16. doi: 10.1186/s13756-019-0671-7
10. Carrique-Mas JJ, Trung NV, Hoa NT, Mai HH, Thanh TH, Campbell JI, et al. Antimicrobial usage in chicken production in the mekong delta of Vietnam. Zoonoses Public Health. (2015) 6:70–8. doi: 10.1111/zph.12165
11. Trung NV, Carrique-Mas JJ, Thi Hoa N, Mai HH, Tuyen HT, Campbell JI, et al. Prevalence and risk factors for carriage of antimicrobial-resistant Escherichia coli on household and small-scale chicken farms in the Mekong Delta of Vietnam. J Antimicrob Chemother. (2015) 70:2144–52. doi: 10.1093/jac/dkv053
12. Nhung N, Cuong N, Thwaites G, Carrique-Mas J. Antimicrobial usage and antimicrobial resistance in animal production in Southeast Asia: a review. Antibiotics. (2016) 5:37. doi: 10.3390/antibiotics5040037
13. Carrique-Mas J, Van NTB, Cuong NV, Truong BD, Kiet BT, Thanh PTH, et al. Mortality, disease and associated antimicrobial use in commercial small-scale chicken flocks in the Mekong Delta of Vietnam. Prevent Vet Med. (2019) 1:15–22. doi: 10.1016/j.prevetmed.2019.02.005
14. Truong DB, Doan HP, Doan Tran VK, Nguyen VC, Bach TK, Rueanghiran C, et al. Assessment of drivers of antimicrobial usage in poultry farms in the Mekong Delta of Vietnam: a combined participatory epidemiology and Q-sorting approach. Front Vet Sci. (2019) 6:84. doi: 10.3389/fvets.2019.00084
15. Nguyen NT, Nguyen HM, Nguyen CV, Nguyen TV, Nguyen MT, Thai HQ, et al. Use of colistin and other critical antimicrobials on pig and chicken farms in Southern Vietnam and its association with resistance in commensal Escherichia coli bacteria. Appl Environ Microbiol. (2016) 8:3727–35. doi: 10.1128/AEM.00337-16
16. Pham-Duc P, Cook MA, Cong-Hong H, Nguyen-Thuy H, Padungtod P, Nguyen-Thi H, et al. Knowledge, attitudes and practices of livestock and aquaculture producers regarding antimicrobial use and resistance in Vietnam. PLoS ONE. (2019) 1:e0223115. doi: 10.1371/journal.pone.0223115
17. Phu DH, Giao VTQ, Truong DB, Cuong NV, Kiet BT, Hien VB, et al. Veterinary drug shops as main sources of supply and advice on antimicrobials for animal use in the Mekong Delta of Vietnam. Antibiotics. (2019) 8:195. doi: 10.3390/antibiotics8040195
18. Dung NT, Truong BD, Cuong NV, Van NT, Phu DH, Kiet BT, et al. A survey of retail prices of antimicrobial products used in small-scale chicken farms in the Mekong Delta of Vietnam. Global Health. (2020) 16:8. doi: 10.1186/s12992-019-0539-x
19. Raasch S, Collineau L, Backhans A, Sjölund M, Belloc C, Emanuelson U, et al. Effectiveness of alternative measures to reduce antimicrobial usage in pig production in four European countries. Porcine Health Manage. (2020) 6:6. doi: 10.1186/s40813-020-0145-6
20. Postma M, Vanderhaeghen W, Sarrazin S, Maes D, Dewulf J. Reducing antimicrobial usage in pig production without jeopardizing production parameters. Zoonoses Public Health. (2017) 6:63–74. doi: 10.1111/zph.12283
21. Rojo-Gimeno C, Postma M, Dewulf J, Hogeveen H, Lauwers L, Wauters E. Farm-economic analysis of reducing antimicrobial use whilst adopting improved management strategies on farrow-to-finish pig farms. Prevent Vet Med. (2016) 1:74–87. doi: 10.1016/j.prevetmed.2016.05.001
22. Roskam JL, Lansink AGJMO, Saatkamp HW. The technical and economic impact of veterinary interventions aimed at reducing antimicrobial use on broiler farms. Poult Sci. (2019) 9:6644–58. doi: 10.3382/ps/pez517
23. Carrique-Mas JJ, Rushton J. Integrated interventions to tackle antimicrobial usage in animal production systems: the ViParc project in Vietnam. Front Microbiol. (2017) 8:1062. doi: 10.3389/fmicb.2017.01062
24. FAO. Smallholder Poultry Production—Livelihoods, Food Security and Sociocultural Significance. Food and Agriculture Organization of the United Nations (2010). Available online at: http://www.fao.org/3/al674e/al674e00.pdf (accessed October 29, 2020).
25. Bessell PR, Kushwaha P, Mosha R, Woolley R, Al-Riyami L, Gammon N. Assessing the impact of a novel strategy for delivering animal health interventions to smallholder farmers. Prevent Vet Med. (2017) 1:108–16. doi: 10.1016/j.prevetmed.2017.08.022
26. Davey P, Brown E, Charani E, Fenelon L, Gould IM, Holmes A, et al. Interventions to improve antibiotic prescribing practices for hospital inpatients. In: The Cochrane Collaboration, editors. Cochrane Database of Systematic Reviews (p. CD003543.pub3). London: John Wiley & Sons, Ltd. (2013).
27. Talkington K, Hoelzer K, Wong N, Thomas J. Alternatives to Antibiotics in Animal Agriculture. (2017). Available online at: https://www.pewtrusts.org/~/media/assets/2017/07/alternatives_to_antibiotics_in_animal_agriculture.pdf (accessed August 28, 2020).
28. Yen NTP, Phu DH, Van Nguyen C, Kiet BT, Hien BV, Padungtod P, et al. Labelling and quality of antimicrobial products used in chicken flocks in the Mekong Delta of Vietnam. Vet Med Sci. (2019) 5:512–6. doi: 10.1002/vms3.189
29. PoultryWorld. Niche Broiler Production in Vietnam, 2018. (2018). Available online at: https://www.poultryworld.net/Home/General/2018/10/Niche-broiler-production-in-Vietnam-347492E/ (accessed July 13, 2020).
30. VASFU. Vietnam: Vietnam African Swine Fever Update. USDA Foreign Agricultural Service (2019). Available online at: https://www.fas.usda.gov/data/vietnam-vietnam-african-swine-fever-update (accessed July 21, 2020).
Keywords: antimicrobial use, disease, smallholder farms, poultry, Vietnam
Citation: Phu DH, Cuong NV, Truong DB, Kiet BT, Hien VB, Thu HTV, Yen LK, Minh NTT, Padungtod P, Setyawan E, Thwaites G, Rushton J and Carrique-Mas J (2021) Reducing Antimicrobial Usage in Small-Scale Chicken Farms in Vietnam: A 3-Year Intervention Study. Front. Vet. Sci. 7:612993. doi: 10.3389/fvets.2020.612993
Received: 09 October 2020; Accepted: 29 December 2020;
Published: 28 January 2021.
Edited by:
Ioannis Magouras, City University of Hong Kong, Hong KongReviewed by:
Agnes Agunos, Public Health Agency of Canada, CanadaLuís Pedro Carmo, University of Bern, Switzerland
Copyright © 2021 Phu, Cuong, Truong, Kiet, Hien, Thu, Yen, Minh, Padungtod, Setyawan, Thwaites, Rushton and Carrique-Mas. This is an open-access article distributed under the terms of the Creative Commons Attribution License (CC BY). The use, distribution or reproduction in other forums is permitted, provided the original author(s) and the copyright owner(s) are credited and that the original publication in this journal is cited, in accordance with accepted academic practice. No use, distribution or reproduction is permitted which does not comply with these terms.
*Correspondence: Doan Hoang Phu, cGh1ZGgmI3gwMDA0MDtvdWNydS5vcmc=